ABSTRACT
This report synthesizes all aspects of the taxonomy, distribution, history of introduction and spread, ecological constrains (including preferred climate, substratum and habitats), responses to biotic and abiotic factors, biology (including phenology, vegetative and reproductive biology), economic importance and human uses, ecological impacts, legislation and management of Carpobrotus N.E.Br. (Aizoaceae), a prominent invasive plant in Europe.
Carpobrotus species are mat-forming trailing succulent perennial herbs native from South Africa, introduced in Europe for ornamental and soil stabilization purposes since the beginning of the seventeenth century, now widely naturalized on coastal habitats of southern and western Europe. C. acinaciformis and C. edulis are the main species recognized outside South Africa, together with their hybrids and potential hybrid swarms. Identification conflicts both in the native and invaded areas raise doubts on the taxonomy of these taxa, but hybridization processes may boost adaptive changes in the invaded range.
The release of Carpobrotus in natural environments and protected areas is prohibited in several European countries, but this taxon is not included in the list of invasive species of Union concern. Carpobrotus is a pioneer of disturbed sites and coastal areas including cliffs and sand dune systems, due to its tolerance to stress factors such as salinity, drought and excess of light. Carpobrotus invasion ultimately affects patterns of native species diversity. Moreover, it has been recognized as a major driver of soil conditions shifts and soil geochemical processes disruptions, representing a serious threat for coastal habitats.
Management plans for Carpobrotus must consider its high plasticity for morphological and ecophysiological traits, which may probably explain its tolerance to a wide range of ecological conditions. Its flexible mating systems, which represent an optimal strategy to facilitate local adaptation and habitat colonization, include ability to produce apomictic seeds, self- and cross-pollination, and an intense vegetative clonality. In addition, Carpobrotus produces a large seed bank with a moderate short-term persistence, and fruits are effectively dispersed by mammals. The most efficient control methods are physical removal and herbicide application on leaves, whereas integration of biological control with other conventional management methods are likely to be most effective. A long-term monitoring of control actions and restoration of soil conditions are needed to prevent recovering from clonal parts, seed bank or mammal faeces as well as potential new invasions by other opportunistic species.
Taxonomy
Names and classification
Scientific name: Carpobrotus edulis (L.) N.E.Br., 1926
Basyonym: Mesembryanthemum edule L., 1759
Taxonomic position: Eudicotyledons, Order Caryophyllales Juss. ex Bercht. & J.Presl
Family: Aizoaceae Martinov, subfamily Ruschioideae
Common names: sour-fig, cape-fig, Hottentots-fig, ice plant [EN], fico degli Ottentotti (IT), uña de gato, bálsamo [ES], figue marine, griffes-de-sorcières [FR], hottentottenfeige, pferdefeige [DE], chorão-da-praia [PT].
EPPO code: CBSED
Type: the type of C. edulis is an iconotype, ie the drawing of “Mesembriathemum falcatum majus, flore amplo luteo”, table 212 in Dillenius, Johann Jakob. Hortus Elthamensis […] Tomus alter, 1732 (Wisura and Glen Citation1993).
Scientific name: Carpobrotus acinaciformis (L.) L.Bolus, 1927
Basyonym: Mesembryanthemum acinaciforme L., 1753
Taxonomic position: Eudicotyledons, Order Caryophyllales Juss. ex Bercht. & J.Presl
Family: Aizoaceae Martinov, subfamily Ruschioideae
Common names: sour-fig, cape-fig, Hottentots-fig, ice plant [EN], fico degli ottentotti (IT), uña de gato, bálsamo [ES], figue marine, griffes-de-sorcières [FR], hottentottenfeige, pferdefeige [DE], chorão-da-praia [PT].
EPPO code: CBSAC
Type: the type of C. acinaciformis is an iconotype, ie the drawing of “Mesembriathemum acinaciforme flore amplissimo purpureo”, table 211 in Dillenius, Johann Jakob. Hortus Elthamensis […] Tomus alter, 1732 (Wisura and Glen Citation1993).
Identification conflicts
Aizoaceae sensu Hartmann (Citation1993, Citation2012) is the largest family of leaf succulent plants and consists of about 2,500 species in 127 genera. Members of this family are predominantly perennial shrubs or subshrubs, rarely annual or biennial herbs. The primary centres of diversity are South Africa and Southern Europe, but a few species are native to America, Australia and New Zealand (Hartmann Citation1993; Klak et al. Citation2003). Within the family Aizoaceae, the subfamily Ruschioideae contains about 1,585 species in 112 genera including Carpobrotus N.E.Br. (Hartmann Citation1993). Approximately 20–25 species are recognized in the genus, most from South Africa, four from Australia and New Zealand and one from South America. However, there are several taxonomic uncertainties and a full review of the genus is needed.
The taxonomic identity of the invasive Carpobrotus species in Europe and the Mediterranean has long been a subject of debate. Two species are most often included in the European floras: C. edulis and C. acinaciformis (Cabello Citation2009; Clement and Foster Citation1994; Gonçalves Citation1990; Pastor Citation1987; Tutin Citation1993). According to the original descriptions and floras, the main diagnostic character for these species is petal colour, yellow in C. edulis, purple in C. acinaciformis. However, there are reasonable doubts on the validity of flower colour as a reliable character for identification. Strid and Tan (Citation1997) suggest that records of C. acinaciformis from Greece correspond to C. edulis var. rubescens Druce, a purple-perianth variety of C. edulis. The occurrence of this variety, commonly used in gardening (Cullen Citation2011) has been reported in Europe (eg Crete, Fielding and Turland Citation2005; Ireland, Parnell and Curtis Citation2012). Preston and Sell (Citation1988) also treated the variation in flower colour within the C. edulis complex in the British Isles, recognizing three varieties including C. edulis var. edulis with pure yellow petals, C. edulis var. rubescens with purple flowers, and C. edulis var. chrysophthalmus C.D.Preston & P.D.Sell, with petals yellow at base and purple at apex. They suggest a possible hybrid origin for this variety. More recently, some authors have confirmed the occurrence of another taxon with an unclear identity, generally described as close to C. acinaciformis. This has led to the use of a C. aff. acinaciformis entity in the scientific literature (eg Suehs, Médail, and Affre Citation2003; Ortiz, Lumbreras, and Rosselló Citation2008; Traveset, Moragues, and Valladares Citation2008). According to Suehs, Affre, and Médail (Citation2004a, Citation2004b) this could be a hybrid form between the two species. Intercrosses between C. edulis and C. acinaciformis have been reported in South Africa (Wisura and Glen Citation1993) and in Europe (Ortiz, Lumbreras, and Rosselló Citation2008; Andreu et al. Citation2010; Suehs, Affre, and Médail Citation2004a, Citation2004b) (see reproductive biology section).
Wisura and Glen (Citation1993), in their monograph on South African species of Carpobrotus, used a number of morphological characters for the identification of C. edulis and C. acinaciformis, synthesized in . These characters were partly tested in introduced populations of southern France by Suehs, Affre, and Médail (Citation2004a, Citation2004b), who concluded that both species are present in the area, although a wide hybridization zone has produced a hybrid swarm between C. edulis and C. acinaciformis, hampering identification. Preston and Sell (Citation1988) developed their own key for Carpobrotus but, as noted by Hartmann (Citation2001), discrimination between the different taxa is unclear. In the key of Gonçalves (Citation1990) and in other floras from the Iberian Peninsula (eg Cabello Citation2009) the section of the leaf is considered as a discriminant character (an equilaterous triangle for C. edulis, isosceles for C. acinaciformis), already suggested by Marloth (Citation1913) but not considered by Wisura and Glen (Citation1993).
Table 1. Main diagnostic characters for Carpobrotus edulis and C. acinaciformis from Wisura and Glen (Citation1993) with an addition from Gonçalves (Citation1990).
Several other Carpobrotus species have been recorded as alien to Europe, but many are a result of misidentifications or nomenclature ambiguity (Preston and Sell Citation1988; López González Citation1995; Hartmann Citation2001). Carpobrotus chilensis (Molina) N.E.Br. is a smaller species with an unknown origin that occurs in the Pacific coasts of western South America, and introduced in California, where it is known to hybridise with C. edulis (Vilà, Weber, and D’Antonio Citation1998). Reports in Europe are from the Balearic Islands, but these occurrences have not been confirmed (Akeroyd and Preston Citation1990; López González Citation1995). Carpobrotus glaucescens (Haw.) Schwantes and C. virescens Schwanthes, two species from Australia (Blake Citation1969), have also been reported from the British Isles (Preston and Sell Citation1988). Unless otherwise stated, we refer to the C. edulis – C. acinaciformis complex throughout this monograph, with specific comments for a given taxon (eg C. edulis, C. acinaciformis, C. aff. acinaciformis) reproduced as in the original source ().
Figure 1. The Carpobrotus complex in the invaded range at different development stages. a) Detail view of C. edulis flowers with yellow petals, fading to pink when aging, and leaves with equilateral triangular section; b) Detail view of Carpobrotus sp., tentatively identified as C. acinaciformis with petals rose purple and yellow filaments, and leaves with isosceles triangular section. See section on taxonomy for a more detailed information on identification conflicts of the species.
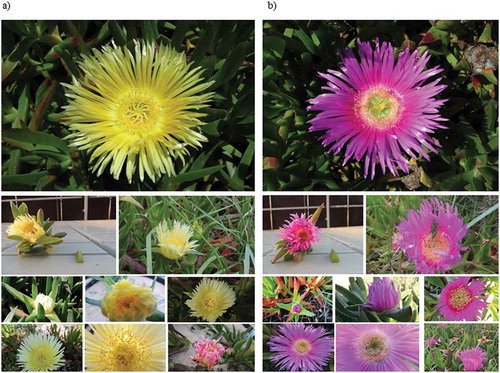
Morphological description
The genus Carpobrotus consists of mat-forming trailing succulent perennial herbs, with opposite leaves, somewhat connate at the base, sharply 3-angled, with a triangular cross-section, ranging from isosceles to equilateral depending on the species (). The chlorenchyma occurs all-round the periphery and colourless water-storage tissue in the core. The water-storage tissue may account for 69% of the leaf fresh weight as opposed to 30% of the leaf dry weight (Earnshaw, Carver, and Charlton Citation1987). The main vascular bundles run through the water-storage tissue with a large bundle occurring in the centre and a smaller bundle near each corner (Earnshaw, Carver, and Charlton Citation1987). The chlorenchyma of C. edulis contains numerous secretory cells with mucilaginous contents, which are released when fresh material is cut. These cells also occur sporadically in the water storage tissue (Earnshaw, Carver, and Charlton Citation1987).
The flowers of Carpobrotus are among the largest in the subfamily Ruschioideae. They are pedicellate, terminal, solitary, up to 120–150 mm in diameter (Wisura and Glen Citation1993). Each pedicel has a pair of leaf-like bracts, usually borne at the midpoint. The calyx in Carpobrotus is almost always composed of five lobes (sepals), which may be sub-equal (C. acinaciformis) or very unequal (C. edulis subsp. edulis). The receptacle is considered a very important character in this genus, turbiniform and tapering into the pedicel in C. edulis, while not tapering into the pedicel in C. acinaciformis (Wisura and Glen Citation1993; ). The multi-locular ovaries are characteristic of the genus Carpobrotus, with the number of locules varying within species and sometimes even on a single plant. The number of styles corresponds with the number of locules (Wisura and Glen Citation1993). The fruit is fleshy, pulpy, indehiscent, without valves and bearing many glossy, brown, obovoid and slightly compressed seeds (Wisura and Glen Citation1993).
Carpobrotus edulis is the only member of the genus to have distinctly yellow flowers, fading to pink when aging (Wisura and Glen Citation1993) (). In its native range, two subspecies have been described, notably C. edulis subsp. edulis and C. edulis subsp. parviflorus Wisura & Glen. The former is the most widespread in both its native and invaded range; the latter has smaller flowers and its habitat seems to be limited to the mountains of the south-western Cape above 800 m a.s.l. (Wisura and Glen Citation1993).
Carpobrotus acinaciformis occurs in abundance on the Cape Peninsula, although conflicts in the identification of Carpobrotus species persist even in its native range (A. Novoa pers. observ.). Its petals are rose purple, filaments pinkish, pallid towards the base. The distinctly scimitar-shaped leaves and the oval fruit distinguish this species from other Carpobrotus species (). However, in the native range, there is a considerable variation in the appearance of the plant in contrasting habitats (A. Novoa pers. observ.).
Distribution and status
Native range
Carpobrotus edulis is native to the Eastern Cape, Northern Cape and Western Cape in South Africa, where it has been reported as growing mainly on coastal and inland slopes at low altitudes (Manning and Paterson-Jones. Citation2007), although it can grow in the mountains of the south-western Cape above 800 m a.s.l. (subsp. parviflorus, Wisura and Glen Citation1993). On the other hand, the native range of C. acinaciformis is restricted to the Western Cape, from Saldanha to Mossel Bay, where it grows in coastal sandy soils near the sea (Wisura and Glen Citation1993; Raimondo et al. Citation2009). Both species (and probably their hybrids) have been intensively planted as ornamental plants all over South Africa, especially in coastal areas. In fact, it is almost impossible to find any large area in the South African coast devoid of Carpobrotus (A. Novoa, pers. observ.) ().
Introduced range
Carpobrotus species have been introduced in all five continents and they are widely naturalized on many coastal habitats outside their native range.
In Oceania, C. edulis was introduced in Australia (Weber Citation2003) where it is naturalized along the southern and eastern coast, being recorded on South Australia, New South Wales, Victoria, and Tasmania. In the northeast of the country, it has also been documented on the south coast of Queensland (Atlas of Living Australia Website Citation2017); in Western Australia, it is naturalized along the west coast from Perth to Albany (Malan and Notten Citation2006). This species was also cultivated and recorded on French Polynesia; in Tahiti Island, it has a restricted distribution (Florence Citation2004) but in Pitcairn Island it occurs in a wider area and is considered as a potential invader in cliffs (Meyer Citation2000). In New Zealand, C. edulis is considered invasive (Howell Citation2008) and it has established on many parts of the coast; it occurs in “cliffs and sand dunes, coastal and inland on railway and roadside cuttings” and it spreads from the North Island (Northland, Auckland, Bay of Plenty, Wellington, Wairarapa) to the South Island (Nelson, Marlborough, Canterbury, Otago, Southland) (Webb, Sykes, and Garnock-Jones Citation1988).
In Northern Africa, C. edulis is naturalized in Algeria and Morocco (USDA National Genetic Resources Program Citation2012; GBIF.org Citation2017b) and it has been introduced in Lybia (Delipetrou Citation2009). It can also be found naturalized along coastal areas of Sousse, Tunisia (Brandes Citation2001; Greuter and Domina Citation2015; GBIF.org Citation2017b).
In North America, it is naturalized in Florida (Wunderlin et al. Citation2018) and is considered invasive in California (CAL-IPC Citation2006) from the Northwest coast (Eureka) to Mexico (Rosarito bay, Lower California) (Albert, D’Antonio, and Schierenbeck Citation1997). In South America, C. edulis has been planted extensively as an ornamental in Argentina (Schmalzer and Hinkle Citation1987). In Chile, C. edulis has naturalized in the Juan Fernández Archipelago and coastal areas of Valparaiso and Biobío regions (Sotes, Cavieres, and Rodríguez Citation2015). It has also been introduced in Bolivia (Jørgensen, Nee., and Beck Citation2014) and Uruguay (Masciadri, Brugnoli, and Muniz Citation2010) where it was only recorded in Punta Ballena, Maldonado (Ríos et al. Citation2010).
In Western Asia, C. edulis has become an invader over large natural areas in Israel (Dufour-Dror Citation2013). It was also recorded in Lebanon, Syria and Cyprus (Delipetrou Citation2009) and it is considered as alien on Turkey (Arslan, Uludag, and Uremis Citation2016).
In Europe, the presence of two hybridizing taxa (C. edulis and “C. aff. acinaciformis”) in the Mediterranean basin is well documented (Suehs, Affre, and Médail Citation2004a, Citation2004b; Verlaque et al. Citation2011). They are widely distributed along the Mediterranean coast from Spain to Greece and along the Atlantic coast from Gibraltar to the United Kingdom ().
Figure 3. Distribution map of C. edulis and C. acinaciformis in Western Europe. The map is given according to GBIF (2017) and the Fédération des Conservatoires botaniques nationaux (Citation2013) with some modifications based on our unpublished data on the species. Species as in the original source, see text for identification problems.
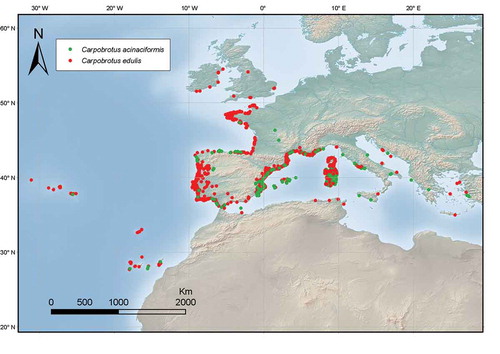
In Spain, Carpobrotus has invaded most coastal regions (Gonçalves Citation1990) along the Cantabrian, Atlantic (eg Campos et al. Citation2004; Campos and Herrera Citation2009; Torre Fernández Citation2003; Fagúndez and Barrada Citation2007) and Mediterranean coast (eg Dana et al. Citation2005) including the Balearic Islands (Moragues and Rita Citation2005). In Mainland Portugal, Carpobrotus is also considered invasive in coastal ecosystems and both taxa occur from the North (Minho) to the South (Algarve) (Marchante et al. Citation2014). In Mediterranean France they are widely spread in the Albères Coast, Provence-Alpes-Côte d’Azur, Bagaud and Porquerolles (Hyères archipelago, Provence) and Corsica, which is particularly invaded by these two taxa (Suehs, Affre, and Médail Citation1999; Suehs, Médail, and Affre Citation2001). In Sardinia, Carpobrotus has also naturalized (Camarda et al. Citation2016; EPPO Citation2006), and in Sicily both C. aff. acinaciformis and C. edulis are reported as frequent on beaches throughout the Island (Giardina, Raimondo, and Spadaro Citation2007). Carpobrotus is one of the most threatening invasive species on Central Italy, where it is particularly widespread along the Tyrrhenian coast (Acosta, Izzi, and Stanisci Citation2006; Carranza et al. Citation2010; Santoro et al. Citation2011). Carpobrotus edulis is also expanded along the coast from Croatia to Albania (Barina et al. Citation2014; Boršić et al. Citation2008). In the alien flora of Greece, only C. edulis was catalogued as naturalized with invasive behaviour (Arianoutsou et al. Citation2010). However, both taxa can be found invading coastal ecosystems including some Aegean Islands as Crete and Lesbos (Vilà et al. Citation2006). Carpobrotus edulis was also introduced into Malta where it has naturalized throughout the Maltese islands (Malta Environment and Planning Authority Citation2013).
In Northwestern Europe, Carpobrotus is present in the British Isles. In England, it is mainly found in Cornwall (SW) and in the Channel Islands it has spread widely on Guernsey Island (Varnham Citation2006), but other occurrences are reported from scattered places along the coast (Preston and Sell Citation1988). In Ireland, C. edulis is locally naturalized and often abundant on coastal cliffs (Reynolds Citation2002). In Belgium, Carpobrotus is not yet naturalized but it is considered to have a high invasion potential in coastal dune ecosystems (Invasive Alien Species in Belgium Website Citation2013). Along the Atlantic coast of France, Carpobrotus is reported from Basque country to Cotentin, with higher densities in Brittany. Schmalzer and Hinkle (Citation1987) reported that C. edulis has been planted extensively as an ornamental plant in Germany.
In the Atlantic islands, Carpobrotus has been reported in the Azores archipelago, although not among the most frequent alien species (Silva and Smith Citation2006), and in Madeira and Porto Santo islands (Jardim et al. Citation2003; Marchante et al. Citation2014; GBIF Citation2017). In the Canary archipelago, C. edulis has been reported as casual in all islands (Gallo, de la Torre, and Rodríguez Citation2008) while C. acinaciformis is locally established in Gran Canaria Island (Sanz-Elorza, Dana, and Sobrino Citation2005). Outside Europe, the Cape Verde, Santo Antão and Fogo Islands have also been colonized by Carpobrotus. In St. Helena Island, C. edulis occupies large areas especially in the north (Ashmole and Ashmole Citation2000).
History of introduction and spread
South African Aizoaceae have been grown in Europe for ornamental purposes since the beginning of the seventeenth century, and a thorough description of the botanists, scientists and plant collectors involved in these early activities can be found elsewhere (Codd and Gunn Citation1985; Gunn and Codd Citation1981). Among the South African Aizoaceae, Carpobrotus is one of the earliest arrivals since its presence in European gardens dates back to the late seventeenth century (Preston and Sell Citation1988). Retracting the history of spread in Europe is challenging. There is evidence of naturalized populations at the mouth of the English Channel (Channel Islands, West Cornwall coast, Isles of Scilly, northern Brittany) by the end of the nineteenth century (McClintock Citation1975; Le Sueur Citation1984; Lousley Citation1971; Davey Citation1909; GBIF.org Citation2017b; Preston and Sell Citation1988). These naturalized populations are very likely the result of propagule escapes from private botanical gardens and from the horticultural industry. For example, Aizoaceae were grown in gardens on the south Cornish coast at least since 1871 (Baker Citation1871). A few decades later, Carpobrotus could be collected from several naturalized populations around the area (Davey Citation1909; Thurston and Vigurs Citation1922). Also, the Isles of Scilly, 28 miles off South-West England, have a reputation for an exotic flora that owes much to a private botanical garden established in the islands by the mid-ninteenth century, and to the flower industry that flourished in the archipelago later in that century. By the early 1920s, naturalized Carpobrotus was common in the islands (Lousley Citation1971). On the french side of the entrance to the English Channel (France), there has been another private botanical garden in the Island of Batz (near Roscoff, Brittany) since 1897 (Clavreul Citation2008). Interestingly, Batz already had a rich exotic flora before the garden was created because sailors from the island had acclimated locally plants from all over the world. Nonetheless, gardening might not be the only origin for the established populations since the plant may have also been deliberately released at some sites for soil and sand dune stabilization (Preston and Sell Citation1988). In other areas of the world (eg California), several Carpobrotus species have been used for soil stabilization since the early twentieth century (Albert, D’Antonio, and Schierenbeck Citation1997).
Given the above, it is unsurprising that the oldest European record of Carpobrotus in GBIF is from the island of Batz (GBIF.org Citation2017b): a preserved specimen collected in 1889 and housed in the MNHN in Paris (Museum National d’Histoire Naturelle Citation2017) (). Records of Carpobrotus from European countries stored in GBIF (> 3000 records as C. edulis and > 350 records as C. acinaciformis; accessed on 1 May 2017) reveal that naturalized populations could be found in regions as far apart as the English Channel and the northern coast of the Western Mediterranean by the beginning of the twentieth century (GBIF.org Citation2017a, Citation2017b). The collection from Batz is nearly contemporaneous with a preserved specimen from the French Mediterranean coast (1903: Toulon, Provence-Aspes-Côte-d’Azur) (Museum National d’Histoire Naturelle Citation2017), and they are closely followed by records from Spain (1911: Barcelona, Catalonia) and Italy (1923: Bordighera, Liguria). Moreover, by the 1930s, the species had been recorded in the Atlantic coasts of Spain (1932: A Lanzada, Galicia) and Portugal (1936: Figueira da Foz, Centro region) while it was collected from Aquitaine by the mid-1940s (1946: Biarritz, Nouvelle-Aquitaine). It seems unlikely that all European naturalized populations of Carpobrotus were the result of dispersal from a single introduction event. Rather, several independent introductions and/or human-aided spread within Europe seem a more plausible explanation (Faulkner et al. Citation2017). Phylogeographic studies using molecular markers with appropriate resolving power are required to elucidate which scenario is most likely.
Table 2. Oldest records for Carpobrotus from Europe stored in GBIF as of 1 May 2017. Only collections dated before 1950 are included. Complete data sets available at http://doi.org/10.15468/dl.hzsn8l (as C. acinaciformis) and at http://doi.org/10.15468/dl.lkoncy (as C. edulis).
Fewer references can be found for Eastern Mediterranean countries, revealing a latter arrival and spread, although absence of records does not necessarily imply non-occurrence in the area. For example, the earliest record in Croatia dates back to 1951 as a cultivated species, but most records along the Croatian coasts as naturalized populations are dated after 2000 (Nikolić Citation2017) while the earliest record in Albania is from 1976 (Barina et al. Citation2014).
An examination of the records stored in GBIF over time provides a rough image of the spread of Carpobrotus across Europe. Any inference derived from this data set must be interpreted with caution, given the limitations of GBIF, a data-gathering platform that combines records from many independent sources with different levels of accuracy. For example, the low number of records gathered in the 1940s should be attributed to a drop in the number of field surveys, probably due to World War II, rather than to actual changes in the occurrence of Carpobrotus across Europe. Nonetheless, the 920 records stored in GBIF that provide a collection/observation date suggest some interesting patterns ( for links to the complete data set). Most records (883 records, 91%) were identified as C. edulis, and an overwhelming majority of them are field observations recorded very recently in the 2010s (808 records, 83%). These very recent records are clearly biased by surveys conducted along the Portuguese coast (698 records, 72%). Nonetheless, even excluding the records from 2010s, Portugal still accounts for 36% of the pre 2010 records, and ranks second in the number of records of Carpobrotus presence, after Spain accounting for 41% of the pre 2010 records. These data reveal that most of the occurrences of Carpobrotus stored in GIBF are from the Atlantic and Mediterranean coasts of the Iberian Peninsula. France (12% of pre-2010 records) and United Kingdom (6%) follow at some distance. As noted above, these four countries (plus Italy) are also the sites where Carpobrotus was detected at an early date, suggesting that Western Europe was the entrance point to the continent and remains the region where this invader is most widely distributed. Before the 2010s, the number of records per decade has remained low for a long time. This may mean that a long phase of acclimatization took place before the invasive phase began. Alternatively, this invader may have gone rather unnoticed due to its restricted habitat. Only from 1980s, a smooth increase is observed in the number of records, mostly from the Iberian Peninsula. Again, the upward trend in the number of records may indicate an actual spread of the invader within the region or the growing interest in the monitoring of alien species.
Western Europe seems the likely source for the introduction of Carpobrotus to North Africa for ornamental and soil stabilization purposes. In Morocco, it was collected at the Atlantic coast (Mehdya) as early as 1934, while there is evidence that it was present on sand dunes in Tunisia at least in 1980 (GBIF.org Citation2017b).
Ecological constraints
Climate
According to the updated Köppen−Geiger Climate Classification (Kottek et al. Citation2006), the Cape Region is under the influence of a cold semi-arid climate or temperate climate with dry winters and hot and dry summers. Rainfall pattern shows dramatic variations in quantity, dropping from 2000 mm per year in the mountains facing the coast to 200 mm in the areas closest to the coast (Goldblatt Citation1997; Goldblatt and Manning Citation2002). Mean annual rainfall is 576 mm, most of which falls in winter (Mostert et al. Citation2017). The driest month of summer receives less than 30 mm and average month temperatures vary from 12.35 ºC (July) to 21.60 ºC (February).
As invasive, Carpobrotus is distributed in the five worldwide Mediterranean climate regions, all located between about 30º and 45º latitude North and South of the Equator and on the Western sides of the continents. In Europe, Carpobrotus occurs both under warm and hot-summer Mediterranean climate (classes Csa and Csb) throughout the Iberian Peninsula except the Cantabrian coasts, and the Mediterranean coasts of all countries, and Oceanic climate (class Cfb) in northern Spain, western France and the British Isles (Kottek et al. Citation2006).
The distribution of C. edulis outside its biogeographical area of origin does not seem to be constrained by the Mediterranean climatic boundaries. In fact, in Europe, C. edulis occurs in Northwestern France, the South of UK and occasionally in Ireland and northern Germany (see section distribution in introduced range). The potential climatic area suitable for C. edulis could increase in the future. According to Thuiller et al. (Citation2005), it icould invade such areas as the North of UK, and the Tejo and Douro river basin in Portugal. The Southwestern coast of America exhibits a very high probability of occurrence including the North coast of Chile, the coast of Perú, Ecuador and Colombia, the entire California and some regions of Mexico. Some biomes of Central East Africa are also suitable to the invasion of C. edulis. The rate of spread of C. edulis in these areas may also depend on non-climatic factors such as propagule pressure.
Substratum
Soils in native/invaded areas
In the Cape region, the native biogeographic area of Carpobrotus, most of the region is covered with a sclerophyllous shrubland called fynbos. Cape Flats Sand Fynbos landscapes consist of predominantly flat plains with acidic, sandy and poor soils (Mostert et al. Citation2017) with a gradient of nutrient status from the coastal dunes to inland (Witkowski and Mitchell Citation1987). Typical soil types for Cape region are Chromic Luvisols and Albic Arenosols along the coast ancient dunes (FAO-Unesco Citation1977).
Out of the Cape region, Carpobrotus is mainly found in the five biogeochemically distinct Mediterranean climate areas. Carpobrotus seems to have adapted to a wide range of soil types, but there is evidence of preferences for Inceptisols and Mollisols soils orders (Eswaran and Reich Citation2005).
In Australia, Carpobrotus has naturalized mainly along the southern and eastern coast, where the soils are highly variable: planosols, ferralsols, arenosols, cambisols and podzols (FAO-Unesco Citation1978), usually, associated to the surrounding granitic areas (Isbell and the National Committee on Soil and Terrain Citation2016). In New Zealand, Carpobrotus invades North and South Islands on cambisols and andosols (FAO-Unesco Citation1978), mainly in dunes and cliffs with soils ranged from sand to sandy loam. In California, C. edulis is widely distributed through the coast in sand dunes and coastal scrub and grasslands. Invaded soils are derived from marine deposits and are low in fertility (Zedler and Scheid Citation1988) with sand and loamy sand soil texture (D’Antonio Citation1993) belonging to luvisols type (FAO-Unesco Citation1978). In Central Chile, C. edulis appears mainly on luvisols (Sotes, Cavieres, and Rodríguez Citation2015; FAO-Unesco Citation1971). In Europe, Carpobrotus can be found in different soil types, mainly on arenosols and eutric, humic and calcic cambisols (FAO-Unesco Citation1981).
Soil pH
Soils invaded by Carpobrotus in New Zealand present a pH from 4.8 to 5.9 (CaCl2) (Liu et al. Citation2014). These are more acidic than the invaded soils in California, in which the pH is highly variable, ranging from 5.6 to 8.2 (Vilà and D’Antonio Citation1998c). The invaded region of Biobío in Central Chile has soil pH values ranging from 5.2 to 6.2 (Berti et al. Citation2011). In Europe, soils with C. edulis are arenosols and diverse types of cambisols with different pH in soil solution ranging from 5.8 to 9.2 (Santoro et al. Citation2011; Novoa et al. Citation2014). The optimal germination pH value for C. edulis is 8. However, plant growth seems to be stimulated at lower pH values (Novoa et al. Citation2012).
Salinity, soil nutrients and moisture
Carpobrotus edulis is a facultative halophyte (Rodrigues et al. Citation2014a). It occurs under soils with a seasonal variation in salt concentration, lower in winter due to higher precipitations and higher in summer, under higher evaporation rates (Callaway et al. Citation1990).
Carpobrotus invades soils with differences in fertility, from the low-nutrient soils of parts of West and South Australia to the nutrient-rich soils of central Chile, California and the Mediterranean Basin (Vilà and D’Antonio Citation1998c; Stock and Verboom Citation2012; Liu et al. Citation2014). Carpobrotus edulis is adapted to different soil conditions, and germination and early growth are not constrained by a lack of nutrients (Novoa et al. Citation2012). Carpobrotus edulis, in its native region, grow in well-drained sandy soils with low soil moisture content. During winter, soil moisture reaches 7% and decreases close to 0 during summer (Yelenik, Stock, and Richardson Citation2004). In the invaded area, C. edulis grows in a wide range of soil types, common to Mediterranean climates, with a moderate to pronounced seasonal moisture deficit (USDA, Citation1999).
Habitats and syntaxonomy
Native range
In its native range, Carpobrotus commonly occurs in coastal habitats that often hold other succulent species such as Drosanthemum, Euphorbia and Tetragonia (Mucina et al. Citation2006). Carpobrotus is also part of the Fynbos vegetation (Manning and Paterson-Jones. Citation2007) where it is commonly associated with Thamnocortus erectus (Thunb.) Mast., Metalasia muricata R.Br., Euclea racemosa L., Rhus laevigata L., Leucadendron coniferum Meisn., Rhus glauca (Thunb.), Eriocephalus africanus L., Agathosma imbricata (L.) Willd. and Diosma hirsuta L. (Cowling, Macdonald, and Simmons Citation1996).
Invaded range
Carpobrotus can grow and establish in embankments, garden margins and disturbed sites under a wide range of environmental conditions. Previous studies report a preferential distribution of Carpobrotus for coastal habitats, both on rocky and sandy coasts and, in the latter, particularly in the central part of the coastal dune vegetation zonation (Carranza et al. Citation2011; Bazzichetto et al. Citation2016; ). This is probably due to the absence of frost and the tolerance of this genus to salinity (Weber and D’Antonio Citation1999). The main invaded communities according to the EUNIS, Habitats directive and phytosociological classifications are shown in . In California, it can likewise be found in dunes, backdunes, sea cliffs, coastal prairies, shrublands and chaparral (D’Antonio Citation1990a, Citation1993; Albert Citation1995a; Albert, D’Antonio, and Schierenbeck Citation1997; Vilà and D’Antonio Citation1998c).
Table 3. Main habitats invaded by Carpobrotus in Europe according to the EUNIS vegetation codes (Moss Citation2008), Habitats Directive habitats codes (Schaminée et al. Citation2012) and main phytosociological classes (Mucina et al. Citation2016).
Figure 4. Coastal areas invaded by Carpobrotus at the Iberian Peninsula (a, b, d, f, g, h), Italy (c), France (e), and Azores (i); (a-d) show the species at sand dunes ecosystems; (e-f) at cliffs; (g-i) at rocky coastal habitats.
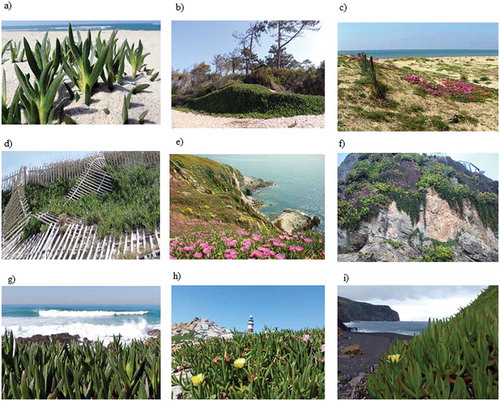
Carpobrotus in Europe occurs in the full mosaic of communities of dune systems, from the early colonizers to the most stabilized woody communities (Santoro et al. Citation2011). In Portugal, it can be found in pre-forest formations of Juniperus thurifera subsp. turbinata (Guss.) Nyman, with other shrubs or trees such as Rhamnus alaternus L. or Pinus pinaster Aiton (Neto Citation2002), as it can effectively compete in shaded and open habitats (Moragues, Traveset, and Valladares Citation2005). It also occurs in coastal dry heaths dominated by Corema album (L.) D.Don., Stauracanthus spp., Thymus spp. and Cistus spp. of the Cisto-Lavanduletea phytosociological class, and dry dune heaths (Calluno-Ulicetea) over podzolic soils with Calluna vulgaris (L.) Hull and Ulex australis Clemente (Neto, Capela, and Costa Citation2004; Neto, Arsénio, and Costa Citation2009). Carpobrotus invades grey dunes, relatively stable communities with high plant cover values and soil development such as the dune pastures of the Koelerio–Corynephoretea class. It also occurs in the foredune vegetation of marram grass (Ammophiletea) of the Mediterranean and Atlantic biogeographic regions (Neto, Arsénio, and Costa Citation2009) and in other communities with low plant cover from the foredune (Cakiletea maritimae). In addition, Carpobrotus can grow on wet soils of dune valleys, where it competes with sedges, rushes and tall grasses of dune slacks (Molinio–Holoschoenion), and even marshes (Bech and Hernández Citation1976), although it seems to prefer well-drained soils.
In cliffs, it grows with many different species at different stages of vegetation development, from the Festuca grasslands of the coastline to the shrub heath or scrub communities of Calluno-Ulicetea and Cisto-Lavanduletea (Costa et al. Citation1997). Mats of Carpobrotus can spread to the vertical cliffs and cover the open vegetation community of adapted species such as Armeria maritima Willd., Limonium spp. or Crithmum maritimum L. (Fernández Prieto and Loidi Citation1984). On the Roussillon coast (Southern France), it has been reported in the cliffs competing with the rare species Armeria ruscinonensis Girard (Rioux, Roux, and Pignatti Citation1955). It also displaces the endemic Limonium emarginatum (Willd.) Kuntze, the dominant species in the cliffs of Algeciras, at the Strait of Gibraltar (Garzón, Castillo, and Figueroa Citation2005).
Responses to environmental factors
Biotic factors
In California, D’Antonio (Citation1993) demonstrated that factors governing C. edulis ability to colonize are community-dependent. In the backdune and coastal scrub sites, C. edulis seedlings become successfully established, despite some reduction in growth due to competition with shrubs. In grasslands, annual grasses inhibit C. edulis seedlings establishment, but C. edulis may succeed if there is any disturbance (such as gophers or ground squirrels). In such cases, C. edulis competes successfully and limits grass development.
Facilitation by co-ocurring exotic or native species can boost the invasion process of Carpobrotus. For example, Pinus halepensis Mill., growing close to the coast in Mediterranean forest, facilitates the inland spreading of C. aff. acinaciformis by protecting it from an excess of light without lessening its growth (Traveset, Moragues, and Valladares Citation2008). The impact of Carpobrotus on native community structure and diversity has been widely studied. In the Tyrrhenian coastal dunes of Italy, diversity patterns of focal species were significantly reduced in C. aff. acinaciformis invaded areas compared to non-invaded. However, taking into account only native species, no differences in diversity parameters were found (Santoro et al. Citation2012a). Maltez-Mouro, Maestre, and Freitas (Citation2010) reported similar results in coastal Portuguese dune systems. On the contrary, Vilà et al. (Citation2006) and Fried et al. (Citation2014) observed a significant decline in diversity patterns when C. acinaciformis and C. edulis invaded coastal habitats of Mediterranean islands or mainlands.
Herbivory
The “natural enemies hypothesis”, an explanation for the success of introduced species (Elton Citation1958; Russo, Mazzeo, and Suma Citation1999) may apply to Carpobrotus either for aboveground herbivores (Maron and Vilà Citation2001) or soil-borne diseases (Van Grunsven et al. Citation2009). Yet, D’Antonio (Citation1993) and Vilà and D’Antonio (Citation1998b, Citation1998c) demonstrated that generalist herbivores can strongly limit C. edulis initial establishment in coastal California.
In its native range, numerous species feed on different parts of Carpobrotus. Leaves are eaten by different tortoise and snail species. Flowers are eaten by diverse antelope species and baboons (Papio sp.), while fruits are eaten by baboons, several rodent species, porcupines (Hystrix africaeaustralis (Peters, 1852)), springbok (Antidorcas marsupialis (Zimmermann, 1780)) and humans (Wisura and Glen Citation1993; Vilà et al. Citation2009).
In Mediterranean islands, Carpobrotus fruits are consumed by introduced mammals as European rabbit and black rat (Bourgeois et al. Citation2005). In California, several rabbit species such as jackrabbit and brush rabbit, as well as mule deer and Californian ground squirrel also feed on C. edulis fruits (Vilà and D’Antonio Citation1998b; D’Antonio Citation1990b) (for more details see fruit/seed dispersal section). Other generalist herbivores can feed occasionally on Carpobrotus fruits and leaves in non-native areas such as small rodents (Bourgeois et al. Citation2005), snails as Theba pisana (Müller, 1774), and Helix aspersa (Müller, 1774) (Rodríguez et al. Citation2017), spittlebugs (Hemiptera) as Aphrophora punctipes (Walley, 1928) (Knapp Citation2014) and Philaenus spumarius (Linnaeus, 1758) (Silva et al. Citation2015; Rodríguez et al. Citation2015, Citation2017). Philaenus spumarius can be found in a variety of terrestrial plant communities and habitats (Rodrigues et al. Citation2014b) including areas invaded by C. aff. acinaciformis and C. edulis but damages on plants are not apparent.
In Europe, insects feeding on Carpobrotus such as the native Aphis fabae (Scopoli, 1763) and the exotic Pulvinariella mesembryanthemi (Vallot, 1829) have been described (Majer Citation1982; Rodríguez et al. Citation2017). Two predatory mites belonging to the family Phytoseiidae, namely Typhlodromus phialatus (Athias-Henriot, 1960) and Euseius stipulatus (Athias-Henriot,1960), are reported as natural enemies feeding on C. edulis (Vilà et al. Citation2008).
The ocurrence of the scale insects (Hemiptera: Coccidae) Pulvinaria delottoi (Gill, 1979) and Pulvinariella mesembryanthemi on Carpobrotus has been reported in native (Delotto Citation1979) and non-native areas including Australia (Collins and Scott Citation1982), America (Washburn and Frankie Citation1981; Washburn, Grace, and Frankie Citation1987) and Europe (Mazzeo, Suma, and Russo Citation2008). They are sap-feeding insects with a range of hosts restricted to the Aizoaceae and Crassulaceae families (Washburn, Grace, and Frankie Citation1987). Few studies have evaluated the impact of these scale insects on Carpobrotus. It has been reported that P. mesembryanthemi retards plant growth and may cause the death of the plant (Collins and Scott Citation1982). According to Washburn, Frankie, and Grace (Citation1985), the survival of insects and host plants is inversely related to scale densities. Therefore, intra-specific scale competition, the decline or death of host plants, and natural enemies pressure, operate regulating the scale insect populations and their impact.
Diaspine scale insect species (Hemiptera, Diaspididae) feeding on C. edulis, namely Aonidiella mesembryanthemi (Vallot, 1829), Aonidia mesembryanthemae (Brain, 1919) and Entaspidiotus lounsburyi (Marlatt, 1908) are reported from South Africa and California (Schmalzer and Hinkle Citation1987) and the later species also occurs in Italy on the non-native Mesembryanthemaceae, Disphyma crassifolium (L.) L.Bolus (Russo, Mazzeo, and Suma Citation1999; Mazzeo et al. Citation2014). Like P. mesembryanthemi, the above mentioned diaspine scale insects are uncommon and highly parasitized in their natural environment in the Cape Provinces, but E. lounsburyi can be very destructive in plantings, even parasitized (S. Neser, pers. comm.). White Spot Moth, Mesocelis monticola (Hübner, 1820) (Lepidoptera, Lasiocampidae) (Mountain White Spot) is a host-specific phytophagous insect reported from South Africa, which defoliates Carpobrotus almost completely (Prinsloo and Uys Citation2015).
Plant parasites and diseases
No severe plant parasite or disease infecting Carpobrotus in its native distribution range has been reported, although it has been shown that high humidity could cause bacterial leaf rot or fungal diseases (ie Botrytis cinerea (Pers., 1974)) in plants growing in shady positions or poorly drained sites (Malan and Notten Citation2006). However, those pathogens have not been isolated from affected tissues of Carpobrotus.
In the Mediterranean area, Van Grunsven et al. (Citation2009) demonstrated that C. edulis and the hybrid are free of soil-borne enemies. Nevertheless, an exhaustive evaluation of diseases affecting C. edulis in California was performed in the 1980s by MacDonald et al. (Citation1983) and MacDonald, Hartman, and Shapiro (Citation1984) and then compiled by Schmalzer and Hinkle (Citation1987). summarizes the information contained in the above-mentioned reports, reflecting disease symptoms, favorable conditions and confirmation by pathogenicity tests. MacDonald et al. (Citation1983) also performed tests for mycoplasma, spiroplasma, rickettsia, virus, and nematode detection, but all of them were negative. McCain, Raabe, and Wilhelm (1981) reported C. edulis as a species susceptible to Verticillium wilt, caused by the microsclerotial form of Verticillium species, known as Verticillium dahliae (Kleb, 1913) or V. albo-atrum, (Reinke and Berthold, 1879). See biological control section for recommendations about their use as control agents.
Table 4. List of pathogens found on Carpobrotus edulis collected along roadsides in California (MacDonald et al. Citation1983; MacDonald, Hartman, and Shapiro Citation1984; Schmalzer and Hinkle Citation1987).
Abiotic factors
Both Carpobrotus edulis and C. acinaciformis, are C3-CAM facultative species (Treichel and Bauer Citation1974; von Willert et al. Citation1977; Sanz-Elorza, Dana, and Sobrino Citation2004). CAM is a specialized photosynthetic CO2 fixation pathway that improves water use efficiency by uptaking part or all of the net atmospheric CO2 at night, when plant evaporative demands are lower. This facultative C3-CAM physiology is an important feature to consider in understanding how C. edulis responds to such environmental factors as light, water, temperature, salinity, and nutrient availability.
Light
Differences between C. edulis and co-occurring native species in their physiological performance in response to varying light conditions may be critical for a mechanistic understanding of its success. Falleh et al. (Citation2012) found that C. edulis from provenances differing in rainfall and length of light periods significantly differed in their antioxidant activity and their polyphenol profiles, with long light period provenances exhibiting stronger antioxidant activity together with higher phenolic content. Based on these data, Falleh et al. (Citation2012) suggested that C. edulis may adapt to environmental stress inducing changes in phenol composition and improving its antioxidant capacities in order to protect plant tissues against oxidative stress. A similar strategy of increased flavonoid production in response to oxidative stress by excessive light, especially at sub- and supra-optimal salinities, has been documented for Carpobrotus rossi (Haw.) Schwantes (Pirie et al. Citation2013). Some photoprotective strategies displayed by C3-CAM facultative species of the Aizoaceae family may be strictly salinity dependent. In C3-CAM facultative species, as C. edulis, the induction of CAM might be not straightforward. For example, Gawronska et al. (Citation2013) have reported that strong light stress-causing oxidative damage may not be enough by itself to activate CAM metabolism in Mesembryanthemum crystallinum L. Furthermore, these authors documented that under high irradiance and in the absence of salinity, C3-CAM species may show an age-dependent increase in photosystem II energy dissipation.
Fenollosa, Munné-Bosch, and Pintó-Marijuan (Citation2017) have described several photoprotective strategies in C. edulis. Under strong irradiance, C. edulis activate the xanthophyll cycle to dissipate the excess of energy, registering a two-fold increase in the VAZ xanthophyll pool. This mechanism is used by plants to mitigate the adverse effects of excessive irradiance (Demmig-Adams and Adams Citation1992, Citation1996). In addition, Fenollosa, Munné-Bosch, and Pintó-Marijuan (Citation2017) also suggested that the antioxidant activity of the lutein (Dall’Osto et al. Citation2006) and the antenna size regulation, mainly through the chlorophyll composition, seem to play a key role in the photoprotective strategy of C. edulis.
The efficiency in the use of light by C. edulis, as estimated by changes in leaf reflectance, has been found to depend on factors as the age of the ramets, the habitat of provenance, and the level of clonal integration (Roiloa et al. Citation2014). It has also been reported that C. edulis respond to high light not only by physiological changes (increasing their photochemical efficiency) but also by morphological variations, increasing the biomass allocated to aboveground structures (Roiloa et al. Citation2014; Roiloa et al. Citation2016). Other studies have documented morphological changes in response to light. Traveset, Moragues, and Valladares (Citation2008) showed that the main and lateral shoots of Carpobrotus aff. acinaciformis differed in response to light availability. Whereas the main shoots grew at similar rates under different light environments, the growth rate of lateral shoots was greater in shaded sites. These authors also documented a high phenotypic plasticity in biomass allocation of individuals located in sun and shade. According to Traveset, Moragues, and Valladares (Citation2008), this highly plastic response to light availabilities greatly contributes to a high photochemical performance, even under moderate shade, and allows the species to rapidly colonize the understorey of Mediterranean scrub and woodlands, well beyond the open sand dune systems. Likewise, Fenollosa, Munné-Bosch, and Pintó-Marijuan (Citation2017), using a new approach to compare phenotypic plasticity, based on the analyses of a combination of different traits, found that C. edulis follows an “all-in” strategy, using a profusion of photoprotection mechanisms to respond to annual climatic variations. They concluded that the greater phenotypic plasticity found in C. edulis, compared to a coexisting native species (Crithmum maritimum), could contribute to its success and be advantageous under a scenario of climate change.
Water
MacDonald, Hartman, and Shapiro (Citation1984) observed that excessive rainfall or watering causes the roots of C. edulis to get rotten in places where poor drainage resulted in extended periods of soil saturation. On the contrary, C. edulis growing in semi-natural, water-stressed conditions may achieve drought tolerance by the induction of Crassulacean Acid Metabolism (CAM) (Earnshaw, Carver, and Charlton Citation1987). As a succulent C3-CAM facultative plant, C. edulis can tolerate severe water restrictions due to its photosynthetic flexibility provided by the optional use of CAM photosynthesis (Winter and Holtum Citation2014), and through leaf water storage (Farrell et al. Citation2012). CAM photosynthesis results in a higher water use efficiency by uptaking CO2 through stomata at night, thus reducing water loss through transpiration and maximizing the rate of carbon assimilation to the rate of transpiration (Sayed Citation2001). Plants with greater succulence (expressed as g water cm−2 leaf area) can generally survive longer periods without water (von Willert Citation1992). Therefore, the water storage in leaves may enable C. edulis plants to survive under dry conditions by providing water that can be used to maintain plant function when water is unavailable for uptake by the roots. Fenollosa, Munné-Bosch, and Pintó-Marijuan (Citation2017) have documented that C. edulis growing in a typical Mediterranean climate, with warm and dry summers, was able to maintain a higher level of hydration (> 9 g H2O g−1 dry mass) in autumn, winter and spring compared to a coexisting native (Crithmum maritimum), but differences disappeared during the summer, when both species exhibited their lowest hydration values. In response to water availability, Fenollosa, Munné-Bosch, and Pintó-Marijuan (Citation2017) also showed some changes in C. edulis leaf morphology, such as a 30% increase in leaf mass per volume (LMV, an estimate of leaf succulence) in the summer. A strong negative correlation between LMV and the relative water content indicated the dependence of leaf morphology on water content in this plant.
The ability of C. edulis to share resources among ramets occupying microhabitats of different quality may be determinant at the time of colonizing water-stressed environments (Lechuga-Lago et al. Citation2016). Another important determinant of the success of this species in colonizing environments with long periods of drought has been suggested by D’Antonio and Mahall (Citation1991) who found that C. edulis reduces the water potential of the native shrubs and changes their rooting profiles.
Temperature
There is a surprising lack of published scientific research on the responses of C. edulis to temperature. Most of the existing information comes from non-systematic observations describing its susceptibility to freezing injury (MacDonald, Hartman, and Shapiro Citation1984), or indirect evidence provided by plant breeders referring to a very general knowledge on the preference of C. edulis for temperate climates. It is considered poorly tolerant to low temperatures (apparently, it can be killed by temperatures below about −2°C), but is resistant to high temperatures. To the best of our knowledge, only two studies have explored the effects of temperature on C. edulis. Vilà et al. (Citation2008), after conducting field sowing tests in more than 200 sites across six Mediterranean Basin islands differing in climatic conditions, concluded that the establishment rates (ie seedling to sown seed ratio) tended to be positively correlated with the cumulative precipitation and negatively with mean temperature. D’Antonio, Odion, and Tyler (Citation1993) provided results of laboratory tests showing that C. edulis seeds in burned soils were killed by exposition to temperatures of 105 ºC or higher for five minutes.
The fact that C. edulis is a succulent plant allows us to infer its tolerance to high temperatures. In fact, succulents have been considered among the most tolerant species to high temperatures, with threshold temperature for heat injury that can well exceed 60 ºC (Larcher Citation1995). The high-water content of succulent plants such as C. edulis has important implications for their thermal economy due to the high-specific heat of water that allows to buffer plant tissues against rapid changes in temperature (Fitter and Hay Citation2002).
Salinity
Carpobrotus edulis is strongly influenced by global and local-scale abiotic factors (climate and shoreline distance) (Maltez-Mouro, Maestre, and Freitas Citation2010). In coastal environments occupied by Carpobrotus, plants are subject to varying levels of substrate salinity and salt spray. It has been demonstrated that germination of C. edulis seeds is reduced by high salt concentrations (1.5% salt content) (Weber and D’Antonio Citation1999). At the lower end of the salinity gradient, salt concentrations do not influence C. edulis germination or seedling establishment, probably because of its relative high tolerance to salinity (Novoa et al. Citation2014), which may determine the extent of establishment and distribution along the salt gradient (Weber and D’Antonio Citation1999).
The inhibition of germination by salt is considered an osmotically enforced dormancy, preventing the establishment of seedlings under unfavorable conditions (Ungar Citation1978; Zia and Khan Citation2008). Carpobrotus edulis fruits ripen from late spring to autumn in Europe and in late summer in California; seeds remain dormant in the soil until winter rains triggers germination. Therefore, salt stimulates germination in a favorable season in Carpobrotus.
Low and moderate salinity causes an enhancement in biomass production with an increase in water use efficiency (Weber and D’Antonio Citation1999) although it does not affect early growth (Novoa et al. Citation2014). Carpobrotus edulis is well adapted to maintaining high photosynthetic rates under high salinity conditions, even though other metabolic processes and growth responses are affected (Madawala, Hartley, and Gould Citation2014). Carpobrotus edulis can modulate its response to salinity showing a high efficiency of the photoprotection mechanism, resulting in a high carotenoid to chlorophyll ratio increase (Varone et al. Citation2017). Chlorophyll a fluorescence measurements revealed no permanent adverse impacts on photosynthetic efficiency resulting from long-term exposure to salinity (Madawala, Hartley, and Gould Citation2014). Seawater submergence of C. edulis stolons significantly reduces the amount of light-harvesting antenna pigments, photochemical activity of PSII and growth. This reduction is dependent on the size of the immersed stolon (Roiloa and Retuerto Citation2016). Despite this damage, C. edulis can grow after an event of seawater submergence indicating its extraordinary capacity to tolerate high salt concentrations. Carpobrotus edulis grown for 24 days in nutrient solution plus 400 mM of NaCl showed the typical CO2 gas exchange reactions observed in CAM plants (Winter Citation1973), demonstrating its C3-CAM facultative metabolism under salt stress.
Carpobrotus edulis is a “transformer” species sensu Richardson et al. (Citation2000). It is capable of modifying soil features, although this depends on the characteristics of the invaded habitats (D’Antonio Citation1990a; Molinari, D’Antonio, and Thomson Citation2007). Parameters such as soil salinity are not always modified in the same way by Carpobrotus invasion as reflected in the different findings by Santoro et al. (Citation2011) and Novoa et al. (Citation2014) and in the variation between sites demonstrated in California (D’Antonio Citation1990a).
Nutrient availability
The germination process of Carpobrotus is influenced by the soil nutrient content. High soil nutrient concentration favours the germination process but does not exert an influence on early growth (Novoa et al. Citation2014). Nutrient scarcity is ameliorated by the plant through clonal reproduction and physiological integration (Campoy, Retuerto, and Roiloa Citation2017) that allow C. edulis to invade new poor areas as incipient dune systems or even beach berm (Lechuga-Lago et al. Citation2016). In Europe, the grow-and-die strategy is used by C. edulis to colonize new habitats. A rapid turnover of plant biomass that results from rapid growth, death, and regrowth creates an organic layer and transforms a hostile habitat into fertile soil for the next generation. Transmission of epigenetic changes may increase phenotypic plasticity, and fast evolution boosts the rate of local adaptation in the invaded range (Fenollosa, Roach, and Munné-Bosch Citation2016).
Biology
Phenology
Flowers emerge between February and June in Europe, and between August and October in South Africa (CEEEI Citation2013; GISD Citation2017; L. González pers. observ.). In the invaded range, fruits remain attached to the plant until late autumn when animals begin to consume them (GISD Citation2017).
Vegetative spread is rapid, and clumps can cover several square meters by the expansion of prostrate stems that take root at each node. Vegetative growth occurs almost all year-round. Roiloa et al. (Citation2010) quantified the expansion rate of C. edulis clones colonizing a coastal sand dune in Mata Nacional das Dunas de Quiaios (Portugal). They reported a constant elongation of the clonal fragments averaging 13.75 cm for the time period from March to September. The greatest stolon increase was recorded during the month of May, with an elongation of 4.5 cm. These results are consistent with those proposed by Sintes et al. (Citation2007), who showed a stolon elongation rate of 29.37 cm per year for Carpobrotus aff. acinaciformis colonizing a coastal habitat in the Balearic Archipelago (Spain), but slower than rates shown for C. edulis in California (53 cm per year) (D’Antonio Citation1993).
Vegetative growth
Carpobrotus shows a radial clonal growth with a structure of nodes and internodes that form dense mats and spreads horizontally by the production of numerous modules or ramets that remain physiologically integrated by stolon connections. This clonal growth allows Carpobrotus to effectively colonize the surrounding area (Roiloa et al. Citation2010). The importance of the clonal traits linked to the invasiveness of C. edulis has been recently tested in a number of greenhouse and field experiments. One of the most striking attributes associated with clonal growth in plants is the capacity for physiological integration (ie the possibility of resources being translocated between connected ramets, Slade and Hutchings Citation1987). Several studies have demonstrated that this capacity for physiological integration, and in particular the transport of essential resources from established basal ramets to developing ramets, generates a growth benefit in clonal plants such as C. edulis (Roiloa, Campoy, and Retuerto Citation2015). Indeed, Roiloa et al. (Citation2010) found that physiological integration significantly increased the growth and survival of developing ramets of C. edulis invading a coastal sand dune in competition with native species. In addition, the benefit of physiological integration in terms of photosynthetic efficiency and growth has been observed in developing ramets of C. edulis under water stress conditions (Lechuga-Lago et al. Citation2016). These results indicate that physiological integration may contribute to the invasiveness of C. edulis in sand dune soils with low water retention. Campoy, Retuerto, and Roiloa (Citation2017) reported similar results, showing that physiological integration improves growth and photosynthetic efficiency in C. edulis. Interestingly, this study demonstrated the presence of local adaptation producing highly integrated ecotypes in the harsher rocky coastal habitats. Differences in the capacity for physiological integration between C. edulis and the co-occurring invader congener C. acinaciformis have recently been detected. Portela and Roiloa (Citation2017) reported in a field experiment a benefit from physiological integration in both species; however, C. acinaciformis was more dependent on integration than C. edulis. This result is consistent with those of Suehs, Affre, and Médail (Citation2004a), confirming that vegetative propagation is an important reproductive alternative for C. acinaciformis. On the other hand, a recent study detected a non-local response to herbivores in C. edulis. In particular, it has been shown that the attack of T. pisana snails to basal ramets induced a non-local compensatory response in un-attacked apical ramets. However, this non-local response was not mediated by physiological integration, but probably due to signals released by root exudates (Rodríguez et al. Citation2018).
Closely related to the concept of physiological integration in clonal plants, is the concept of division of labor. This has traditionally been defined as the capacity of functional specialization of interconnected ramets to acquire locally abundant resources that increases the overall performance of the clone (Alpert and Stuefer Citation1997; Hutchings and Wijesinghe Citation1997). Several studies have demonstrated the capacity of C. edulis to show a developmentally programmed (Roiloa et al. Citation2013) and environmentally induced division of labor (Roiloa et al. Citation2014; Roiloa et al. Citation2016). This division of labor was developed by C. edulis, both at physiological and morphological level, and enhanced the overall performance of the clonal fragment. Interestingly, Roiloa et al. (Citation2014) demonstrated that the ability for division of labor was positively selected, being more accentuated in patchier environments, where the presence of this trait would be more beneficial. On the other hand, in a recent study, Roiloa et al. (Citation2016) compared division of labor between populations of C. edulis from its native and invaded range, demonstrating that the benefit from the division of labor was significantly higher in developing ramets in the invaded area. This finding suggests that C. edulis populations might experience rapid evolutionary adaptation in the invaded area, and that division of labor can be considered an important trait for the invasiveness of this species.
Another interesting consequence associated with the capacity for physiological integration in clonal plants is the ability to discriminate between self and non-self genotypes. This ability could be expected to reduce root competition between genets, and as a consequence to increase the performance of the clone. Roiloa, Rodríguez-Echeverría, and Freitas (Citation2014) found in a greenhouse experiment that physiological integration allowed self/non-self genotype recognition in clones of C. edulis, leading to a form of division of labor, which reduced intra-genotype competition, and enhanced the colonization capacity of the species.
The contribution of storage organs, such as stolon and rhizome internodes, to the success of clonal invaders has been considered only recently (Konlechner, Orlovich, and Hilton Citation2016; Dong et al. Citation2012; Lin, Alpert, and Yu Citation2012). The capacity of clonal modules to survive and re-grow after a process of fragmentation could have important implications for the dispersal of clonal invaders. Storage organs of clonal plants can play a crucial role because the stored carbohydrates may be mobilized under unfavourable conditions, allowing the colonization of new habitats. Roiloa et al. (Citation2017) determined the importance of stolons as reserve organs in the colonization of a coastal sand dune by clones of C. edulis. They showed that stolons can help to buffer stressful conditions after a process of fragmentation, thus allowing expansion of C. edulis. Similarly, Roiloa and Retuerto (Citation2016) simulated in a greenhouse experiment a process of fragmentation and a subsequent event of seawater submergence and de-submergence. Their results suggest the importance of stolons in allowing C. edulis to be transported along the shore by the waves and the tide, to colonize new coastal areas.
To summarize, all these recent studies indicate that traits associated with clonal propagation can contribute to the effective colonization of new habitats by C. edulis, and therefore contribute to its invasiveness.
Reproductive biology
Floral biology
Flower pollinators recorded in Carpobrotus are mostly constituted of Hymenoptera ie, bumblebees, Apis mellifera (Linnaeus, 1758), and solitary bees such as Halictus sp., Anthidium sp. in Provence (France) and Rhodanthidium septemdentatum (Latreille, 1809) in Majorca island (Spain) (Suehs, Affre, and Médail Citation2005; Jakobsson, Padrón, and Traveset Citation2008). In parallel, flowers visitors are represented by other Hymenoptera (ie, social bees and wasps), Coleoptera and Diptera species. The effects of Carpobrotus on plant-pollination networks and on reproduction success of native plants are described in the “Ecological impacts” section.
Reproductive strategies and hybridization potential
Both C. edulis and C. acinaciformis show flexible mating systems through intense clonality and different sexual alternatives (Suehs, Affre, and Médail Citation2004a, Citation2004b; Bartomeus and Vilà Citation2009) which may have facilitated local adaptation and habitat colonization (Brown and Burdon Citation1987; Pyšek Citation1997; Ellstrand and Schierenbeck Citation2000). Indeed, C. edulis shows slight agamospermy (ie asexual seed production, see Vilà, Weber, and D’Antonio Citation1998) and is completely self-fertile without inbreeding depression, while C. acinaciformis is not agamospermic and only slightly self-fertile (Suehs, Affre, and Médail Citation2004a). Furthermore, both species show higher fruit and seed sets in intra-specific outcrossing and even more so when they hybridize with each other (Suehs, Affre, and Médail Citation2004a, Citation2004b). In Australia, hybrids occur between Carpobrotus spp. and Sarcozona spp., and also between C. virescens and C. edulis, and C. rossii and both C. edulis and C. acinaciformis (Blake Citation1969; Biffin et al. Citation2016). Several hybrids are also reported from South Africa (Wisura and Glen Citation1993): C. acinaciformis × C. edulis, C. edulis × C. mellei, C. edulis × C. quadrifidus. Hybridization between C. edulis and C. chilensis occurs in California and contributes to invasion success (Albert, D’Antonio, and Schierenbeck Citation1997; Weber and D’Antonio Citation2000; Schierenbeck et al. Citation2005; Gallagher, Schierenbeck, and D’Antonio Citation1997; Vilà and D’Antonio Citation1998a, Citation1998c). Moreover, C. edulis and C. aequilaterus (Haw.) N.E.Br. have been noted to hybridize with the endemic Disphyma australe (Sol. ex Aiton) J.M.Black (Aizoaceae) in coastal areas throughout New Zealand (Chinnock Citation1972). Carpobrotus acinaciformis shows a strong hybrid vigour and significantly introgressed hybrids within a hybrid swarm (named C. aff. acinaciformis) that can lead to new chromosomal and phenotypic variants (Suehs, Affre, and Médail Citation2004a; Verlaque et al. Citation2011). Hybridization/introgression thus contributes to important evolutionary changes throughout the Carpobrotus invasion dynamics (Suehs et al. Citation2006).
Fruit/seed production
Compared to other representatives of the Rushioideae subfamily, the genus Carpobrotus is distinguished by the presence of fleshy, indeshiscent fruits (Hartmann Citation1993) known as “Hottentot figs”, “sour figs” or “marine figs”. In Provence (France), seed production is roughly 1000–1800 seeds/fruit in C. edulis and 650–750 seeds/fruit in C. aff. acinaciformis (Suehs, Affre, and Médail Citation2004a). Carpobrotus edulis, showing higher seed production and germination than C. aff. acinaciformis, has more opportunities for long-distance dispersal. Similar situations have been found in California, where the invasive C. edulis produces approximately twice the seeds than the less aggressive C. chilensis (Vilà, Weber, and D’Antonio Citation1998) and can be widely dispersed (D’Antonio, Odion, and Tyler Citation1993). Indeed, differences in seed production affect the relative propagule pressure, considered as an important feature linked to invasive plant establishment and success (D’Antonio, Levine, and Thomsen Citation2001; von Holle and Simberloff Citation2005).
Seed bank density and longevity
Few studies have evaluated seed bank densities of C. edulis and C. aff. acinaciformis (D’Antonio Citation1990b, Citation1993; Morzaria-Luna and Zedler Citation2007; Chenot et al. Citation2014). Seed bank density appears to be habitat-dependent and can vary from 556 to 4070 seeds/m2 for C. edulis. Indeed, this species seems to have a much larger seed bank than C. aff. acinaciformis (930 seeds/m2 vs. 4070 seeds/m2 for C. edulis; data gathered on the same island; Chenot et al. Citation2014). According to Gioria, Pyšek, and Moravcová (Citation2012), C. edulis forms a short-term persistent seed bank (1–5 years). Decline in seed germination was observed under Lupinus chamissonis Eschsch. bushes, either due to increased mortality or dormancy (D’Antonio Citation1990b). The same author showed that after two years of storage, 49–80% of C. edulis germinated. Carpobrotus edulis and C. aff. acinaciformis seeds can persist for 5 years after eradication (Ruffino et al. Citation2015). Studies on a longer span of time are needed, and it is likely that they will show that Carpobrotus should be included in the persistent seed bank category (> 5 years).
Fruit/seed dispersal
Carpobrotus fruits are dispersed via endozoochory. All the studied rabbit species (Lepus californicus (Gray, 1837), Sylvilagus bachmani (Waterhouse, 1839), Sylvilagus audubonii (Baird, 1858), Oryctolagus cuniculus (Linnaeus, 1758)) widely consume Carpobrotus fruits and increase seed germination after gut passage, from as low as 15 and 24% to as high as 58 to 100% (D’Antonio Citation1990b; D’Antonio, Odion, and Tyler Citation1993; Bourgeois et al. Citation2005; Morzaria-Luna and Zedler Citation2007; Novoa et al. Citation2012). The maximum distances of seed dispersal by rabbits vary greatly between studies, from 2 to 209 m. Rattus rattus (Linnaeus, 1758), Felis catus (Schreber, 1775) and Odocoileus hemionus (Rafinesque, 1817) also widely consume Carpobrotus fruits and increase seed germination while dispersing seeds to a maximum distance of 96, 500 and 800 m, respectively (D’Antonio Citation1990b; Vilà and D’Antonio Citation1998b; Bourgeois et al. Citation2005). Seeds from baboon’s droppings collected in the Cape Peninsula also germinated in great numbers (R. Retuerto pers. observ.). On the other hand, Otospermophilus beecheyi (Richardson, 1829) also consumes Carpobrotus fruits but damages the seeds leaving only 26% of seeds intact (D’Antonio Citation1990b). In small rodents (Paragnathus, Peromyscus or Dipodomys and Apodemus sylvaticus (Linnaeus, 1758)), fruit consumption is anecdotic. Erinaceus europaeus (Linnaeus, 1758), Sus scrofa (Linnaeus, 1758) and Vulpes vulpes (Linnaeus, 1758) were found not to feed on Carpobrotus fruits (Bourgeois et al. Citation2005).
In its native range, Carpobrotus offers shelter to snails, lizards and skinks. Native snakes, such as puff-adders or Cape Cobras, often hide among Carpobrotus clumps to attack the small rodents that are attracted by its fruits (Malan and Notten Citation2006) (see herbivory section).
Economic importance and human uses
Different species of Carpobrotus have been commonly used worldwide since the early twentieth century as ornamental plants in gardening, and to stabilize sand dunes and prevent soil erosion (Weber Citation2003; Chenot et al. Citation2018). During the last decade, Carpobrotus has also been used to establish green roofs (Razzaghmanesh, Beecham, and Kazemi Citation2014; Vahdati, Tehranifar, and Kazemi Citation2017). Moreover, the Australian species Carpobrotus rossii (Haw.) Schwantes is considered as a promising candidate for the phytoextraction of heavy metals (Zhang et al. Citation2015).
Carpobrotus is also a traditional medicinal plant. Its leaves are used to treat sore throats, oral thrush, stomach and mouth ulcers, painful lungs, diarrhea and skin ailments such as eczema or burn injuries. These reports come mainly from its native area (Matsiliza and Barker Citation2001; Van Wyk, de Wet, and Van Heerden Citation2008), but its use to treat hemorrhoids by local people have also been reported in Campania, southern Italy (Motti, Antignani, and Idolo Citation2009). Carpobrotus edulis has also been credited with anti-cancer (Ordway et al. Citation2003), anti-bacterial (van der Watt and Pretorius Citation2001) and antifungal properties (Omoruyi, Afolayan, and Bradley Citation2014).
Additionally, Carpobrotus is important gastronomically, especially in South Africa. The fruits of C. acinaciformis are used to make jam, pickle or chutney (CABI Website Citation2017), while the fruits of C. edulis and C. deliciosus (L.) L.Bolus are also eaten fresh or dried (Hartmann Citation2001). The leaves of C. edulis are also edible and they can be used as food preservative (Omoruyi, Bradley, and Afolayan Citation2012).
Finally, the pollen of C. edulis is a good food source for several predatory mite species, and therefore may be used as biological control – ie C. edulis may boost the growth of mite predators increasing their effectiveness to control some mite pests (Swirski and Dorzia Citation1969; Swirski, Amitai, and Dorzia Citation1970; Ragusa and Swirski Citation1975; Ragusa, Zedan, and Sciacchitano Citation1986; Ferragut et al. Citation1987; Reuveny, Palevsky, and Gerson Citation1996).
Despite all the uses of Carpobrotus, in the invaded range, stakeholders and the public are aware of the invasiveness and negative impacts of Carpobrotus and have a positive attitude towards its management (Bardsley and Edwards-Jones Citation2007; García-Llorente et al. Citation2008; Dehnen-Schmutz, Chas-Amil, and Touza Citation2010).
Ecological impacts
Much of the literature regarding Carpobrotus report significant changes in the invaded ecosystems at a variety of scales (Vilà et al. Citation2006; Molinari, D’Antonio, and Thomson Citation2007; Conser and Connor Citation2009; Carranza et al. Citation2011; Santoro et al. Citation2012b; Fried et al. Citation2014; Rumlerová et al. Citation2016). Carpobrotus has many indirect negative effects on the invaded native coastal ecosystems (). In fact, it has been recognized as a major driver of soil condition shifts and a disruptor of soil geochemical processes, as seen in Santoro et al. (Citation2011), Novoa et al. (Citation2013) and Vieites-Blanco and González-Prieto (Citation2018). There are many studies supporting that Carpobrotus invasion changes the soil pH, salt content, moisture level, nutrient content and microbial activity, but the significance of such changes depends on the initial characteristics of the invaded ecosystem. Effects of the necromass of C. edulis on soil characteristics in backdune and rocky coastal habitats have been reported to provide some competitive advantages related to the physiology of this invasive plant. In particular, Vieites-Blanco and González-Prieto (Citation2017) have documented that differences in necromass characteristics from invaded and non-invaded areas are linked to the ability of C. edulis to discriminate against Al uptake, while favouring Mg and Ca uptake. It also shows a lower requirement (or higher resorption) of key micronutrients (Co, Cu, Fe, Ni, Zn), when compare to native vegetation. In coastal dune pioneer habitats, Carpobrotus invasion is likely to affect the soil physico-chemical and biological processes (D’Antonio Citation1990a; D’Antonio and Mahall Citation1991; Vilà et al. Citation2006; Conser and Connor Citation2009; Santoro et al. Citation2011; Novoa et al. Citation2012; Vieites-Blanco and González-Prieto Citation2018). These soil modifications could ultimately inhibit germination and affect the survival of the specialized native dune species, which spread only in these particularly poor soils (van den Berg et al. Citation2005; van der Heijden, Bardgett, and van Straalen Citation2008). It has been found that different invasive plant species, including Carpobrotus, may favour the replacement of typical native dune plants by ruderal nitrophilous species through soil nutrient enrichment (Maurel et al. Citation2010; Fried et al. Citation2014; Malavasi et al. Citation2016).
Figure 5. Carpobrotus competing with endemic species: a) Ulex europaeus (A Coruña, Spain), b) Armeria pungens (Pontevedra, Spain), c) Corema album (Moledo, Portugal), d) Cistus salviifolius (Pontevedra, Spain), e) Euphorbia paralias, Aetheorriza bulbosa and Ammophila arenaria (Lugo, Spain), f) Centaurea horrida (Sardinia, Italy), g) Helichrysum picardii (Pontevedra, Spain), h) Medicago marina (Viana do Castelo, Portugal), i) Pancratium maritimum and Artemisia crithmifolia (Pontevedra, Spain).
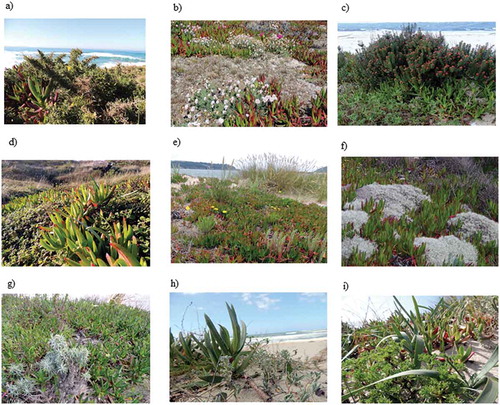
In Californian coastal communities, C. edulis invasion affected water relationships and plant morphology of the shrub species Ericameria ericioides (Less.) Nutt. ex Jeps (= Haplopappus ericioides (Less.) Hook. & Arn.) and Isocoma menziesii var. sedoides (Greene) G.L.Nesom (= Haplopappus venetus var sedoides (Greene) Munz), competing directly for water and light (D’Antonio and Mahall Citation1991). Negative effects of C. edulis decomposed tissues on the germination, survival, growth, and reproduction of annual native Gilia millefoliata Fisch & C.A.Mey in California, by indirect alteration of soil chemistry (decreased soil pH and increased organic matter content) have also been reported (Conser and Connor Citation2009). Also in California, C. edulis has been shown to alter soil nutrients, pH and litter depth (Molinari, D’Antonio, and Thomson Citation2007). Similarly, in the foredunes of Central Italy, C. aff. acinaciformis litter modified significantly soil parameters, increasing nitrogen and organic matter content and decreasing soil pH (Santoro et al. Citation2011). Carpobrotus aff. acinaciformis also affects soil microbial communities, favouring relative increase of fungal with respect to bacterial growth possibly by the increase in total organic carbon and nitrogen (Badalamenti et al. Citation2016). According to these authors, the change in bacteria/fungi ratio can influence the carbon use efficiency, affecting the behaviour of co-ocurring native species and probably favouring the invasion of Carpobrotus. Carpobrotus edulis also lowers Ca and Na content and increases salinity and phosphorus concentration. Those soil modifications have strong negative effects in the early stages of Malcolmia littorea (L.) R.Br. populations, decreasing total germination and survival (Novoa et al. Citation2013). The litter of the invasive C. edulis, which remains on the soil surface for several years, releases allelopathic substances that suppress the native plant germination process and root growth (Novoa et al. Citation2012) depending on plant species and density (Novoa and González Citation2014).
Carpobrotus has undesirable direct impacts on native plants, with negative effects on the germination, survival, growth and reproduction (D’Antonio and Mahall Citation1991; Vilà et al. Citation2006; Conser and Connor Citation2009; Affre et al. Citation2010; Novoa et al. Citation2013). Carpobrotus directly competes with native plants for space, suppressing the growth of mature native shrubs and the establishment of their seedlings (Albert Citation1995a; Conser and Connor Citation2009). Additionally, C. edulis directly competes with native plant species for water, reducing their growth, survival, and reproduction (D’Antonio and Mahall Citation1991; Molinari, D’Antonio, and Thomson Citation2007). Moreover, Carpobrotus may affect the quantitative component of pollination. Its influence on the pollination of native plants can be neutral, facilitative or competitive and it is likely to be species specific, depending on the ecological context (ie the environmental conditions prevailing at a given site), and varying from year to year, along with fluctuations in other factors such as insect abundance, composition, and flower abundance of other native plants (Moragues and Traveset Citation2005; Jakobsson, Padrón, and Traveset Citation2008; Bartomeus, Vilà, and Santamaría Citation2008; Bartomeus, Bosch, and Vilà Citation2008; Morales and Traveset Citation2009; Vilà et al. Citation2009). For instance, Bartomeus, Bosch, and Vilà (Citation2008) reported that although C. aff. acinaciformis is integrated in pollen transport networks, it did not compete for pollination services on coastal Mediterranean ecosystems dominated by shrubs and annual herbs. In fact, it sometimes facilitated the visit of pollinators to native species, affecting the structure of Mediterranean plant-pollinators networks (Bartomeus, Vilà, and Santamaría Citation2008). Similarly, Carpobrotus had no effect on the pollination of the rare plant Dithyrea maritima (Davidson) Davidson in southern California (Aigner Citation2004) or on Cistus monspeliensis L. in the Balearic Islands (Moragues and Traveset Citation2005), but it has a competitive effect (ie, fewer visits) on Lotus cytisoides L. and a facilitative effect (ie, more visits) on Cistus salviifolius L. and Anthyllis cytisoides L. (Moragues and Traveset Citation2005). Likewise, Carpobrotus edulis and C. aff. acinaciformis compete with native plant species by using their pollinators in Southeast France (Suehs, Affre, and Médail Citation2005) and the Balearic Islands (Jakobsson, Padrón, and Traveset Citation2008). If competition dominates, native plant-pollinator networks may be restructured influencing seed production and the dynamics of Carpobrotus invasion.
Several studies have shown that Carpobrotus invasion ultimately affects patterns of native species diversity (Vilà et al. Citation2006; Santoro et al. Citation2012b; Fried et al. Citation2014), confirming that its successful establishment probably operates through the replacement and exclusion of native species, rather than coexistence. For instance, it has been observed that C. aff. acinaciformis represents a serious threat for focal species, characteristic of specific coastal habitats and a major conservation target, representing an early alarm sign of diversity loss (Santoro et al. Citation2012a). Moreover, Carpobrotus may affect community structure and functioning in multiple ways. Molinari, D’Antonio, and Thomson (Citation2007) suggest that C. edulis effectively simplifies communities, through its ability both to reduce vegetation height and to homogenize horizontal vegetative cover. In particular, these authors found that C. edulis differentially altered vegetation height and distribution in backdune and coastal sage scrub communities with the maximum height of vegetation decline in fully invaded sites (Molinari, D’Antonio, and Thomson Citation2007).
Changes in vegetation structure due to Carpobrotus invasions have only been scarcely studied in other organisms. Galán (Citation2008) found a dramatic decrease on the densities of the Western three-toed skink (Chalcides striatus (Cuvier, 1829)) in coastal halophyte grasslands in NW Spain when comparing invaded vs non-invaded spots, and that this decrease correlates with the level of dominance of Carpobrotus. Reptiles, amphibians, insects and other groups are sensitive to environmental changes in coastal habitats, thus potentially affected by the invasion of Carpobrotus.
In conjunction with the decline in taxonomic diversity, Jucker, Carboni, and Acosta (Citation2013) reported a parallel loss in functional diversity as Carpobrotus abundance increased, suggesting that the species is acting as a filter in the process of native community assembly by preferentially excluding species with specific life-history traits. In particular, on the bases of a plant trait analysis, Carpobrotus appears to exclude closely related and ecologically similar taxa (Jucker, Carboni, and Acosta Citation2013). In fact, according to these authors, low-growing species with small leaf surface area-to-weight ratios seemed much more likely to decrease in abundance in response to Carpobrotus invasion. However, Carpobrotus also seem to have a strong impact on wind dispersed species, many of which are ephemeral or characterized by annual life cycles, as already reported by Vilà et al. (Citation2006) and Andreu et al. (Citation2010). Thus, it seems that both weaker competitors and ecologically similar species decline in the presence of Carpobrotus. Ultimately, it has been shown that Carpobrotus invasion in coastal dune plant communities may also affect native assemblages in more subtle and indirect ways, leading to changes in community structure and assembly by disrupting some of the key ecological processes that contribute to determine the pool of plant species present (Santoro et al. Citation2012a). While uninvaded communities were strongly tied to the sea–inland environmental gradient, Carpobrotus invasion may cause a shift to randomness in the species occurrence patterns (Santoro et al. Citation2012a), an effect generally associated to stress factors such as wildfires (Pitzalis, Luiselli, and Bologna Citation2010).
Legislation
Since the Bern ConventionFootnote1 came into force, European and European Union legislations have been concerned with invasive alien species, ie those animals and plants that, after being introduced accidentally or deliberately into a natural environment where they were not normally found, cause serious negative consequences to the new environment. So far, Carpobrotus species have not been specifically taken into account by the Regulation (EU) no. 1143/2014.Footnote2
In Portugal, C. edulis is listed among the 32 species of plants and animals declared invasive (Royal Decree no. 565/99, 21st December) and as such forbidden to be released in the environment (Marchante and Marchante Citation2016). Carpobrotus edulis is listed under Schedule 9 to the Wildlife and Countryside Act 1981 with respect to England, Wales and Scotland. As such, it is an offence to plant or otherwise cause this species to grow in the wild. In Northern Ireland, the Article 15 (2) of The Wildlife (Northern Ireland) Order 1985 (under review) states that if any person plants or otherwise causes to grow in the wild C. edulis or any other species included in Part II of Schedule 9, he shall be guilty of an offence. In the Republic of Ireland, Section 52 (7) of The Wildlife (Amendment) Act 2000 states that if any person who plants or otherwise cause to grow in a wild state any species of flora, or the flowers, roots, seeds or spores of flora except under and in accordance with a licence granted in that behalf by the Minister shall be guilty of an offence. In Spain, the law no. 42/2007 created the Spanish catalogue of Invasive Alien Species which was put into place by the Royal Decree no. 630/2013Footnote3 which lists both C. acinaciformis and C. edulis. As a consequence, a number of management plans to deal with major invasive species have been developed, including for C. edulis and C. acinaciformis on sand dune ecosystems. In Italy (Tuscany), since 2000 the regional law of the 6th of April 2000, no. 56 (afterwards converted into the r.l. 19 March 2015, no. 30 – Gazzetta Uff. 25/03/2015, no.14) explicitly put a ban to the introduction, cultivation and release of Carpobrotus in protected natural habitats (Habitats Directive, HDFootnote4).
Outside Europe, in California, C. edulis is listed as CalEPPC List A-1 (since 1994) and as CDFA-NL (http://www.cal-ipc.org/); on the contrary it is not declared or considered noxious by any state government authorities in Australia. A dedicated search on main legislation databases such as, eg, N-LEX (http://eur-lex.europa.eu/n-lex/index_en) and WorldII (http://www.worldlii.org/) did not return any additional information.
Management
Prevention and early detection
In order to facilitate the prevention of invasive species spread, early detection tools were developed to identify areas more susceptible to invasion, and two of them were specifically tested on Carpobrotus species. Carranza et al. (Citation2011) used a habitat selection function approach combined with a bootstrap test of significance to identify habitat types where the incidence of invasive species is higher or lower than would be expected from a random null model. A different tool for Carpobrotus detection was developed by Calviño-Cancela et al. (Citation2014) consisting in an affordable remote-sensing technique (hyperspectral imaging system operated on board ultralight aircrafts) which allows for accurate spatial and temporal monitoring.
The research program EPIDEMIE (Exotic Plant Invasions: Deleterious Effects on Mediterranean Island Ecosystems) showed that anthropogenic changes in Mediterranean islands increase the invasibility of urban, ruderal and roadside habitats for Carpobrotus (Affre et al. Citation2010; Traveset et al. Citation2008). Avoiding frequent disturbance events may thus be a first prevention step. Early detection is essential, since Carpobrotus can be relatively easy to control if plants are removed when young (D’Antonio and Meyerson Citation2002) (see section control methods for more information).
Eradication and control plans
Eradication and control plans targeting Carpobrotus invasions have been developed in many places around the world (Ruffino et al. Citation2015) and in Europe (Andreu et al. Citation2010; Foxcroft et al. Citation2013; see section on control costs for more information). However, these management actions are carried out mainly by local stakeholders, and precise information on their outcomes is often lacking. In Spain, several plans for control and elimination of invasive plant species in dune systems have been performed in the last 10 years in Andalucía, Asturias, Baleares, Cataluña, Valencia, Galicia and Murcia. In Isla Grosa (Murcia), Cabrera Natural Park (Baleares) and Mondragó Natural Park (Baleares), these actions have been successful (Ministerio de Medio Ambiente y Medio Rural y Marino Citation2011). In Portugal, Carpobrotus control plans were established in Vila Nova de Gaia, in the dune system of Cresmina-Guincho in Madeira Island and in areas of high conservation interest in the Azores archipelago (EPPO Citation2017, Reporting Service no. 08 – 2013 Num. Article: 2013/178). An EPPO survey in 2010 also reported eradication campaigns for Carpobrotus in France (Bagaud Island), Malta (Malta and Gozo islands) and Spain (Andalucía). In Italy, Pontine Archipelago, Tavolara and Carbonara Cape LIFE programs included the eradication of Carpobrotus as one of the objectives. Altogether, the European Commission approved four LIFE Nature proposals in Spain (LIFE00 NAT/E/7339, LIFE00 NAT/E/7355 and LIFE04 NAT/ES/000044, LIFE14 NAT/ES/000699), seven in Italy (LIFE08 NAT/IT/000353, LIFE11 NAT/IT/000093, LIFE12 NAT/IT/000416, LIFE12 NAT/IT/000471, LIFE13/NAT/IT/000433, LIFE14 NAT/IT/000544, LIFE15 NAT/IT/000914) and one LIFE BIO proposal in Portugal (LIFE13 BIO/PT/000386)) in which control and/or eradication of Carpobrotus was involved (Scalera et al. Citation2017).
In all cases, successful control of Carpobrotus, like all other invasive plant species, require long-term management and monitoring to verify the eradication of Carpobrotus re-sprouts and new seedlings, the re-establishment of native plant species, and the restoration of ecosystem-level processes (Ruffino et al. Citation2015; Chenot et al. Citation2018). Moreover, it is fundamental to establish priorities (with respect to the areas more susceptible to Carpobrotus invasion) and to select the most appropriate control methods in each case. Currently the most common methods to control Carpobrotus include physical and chemical control, but these methods are sometimes inadequate because they are costly, labor intensive, or may be harmful for native species. The integration of biological control after taking into account potential unanticipated consequences, along with other control methods could lead to significant cost reductions of invasive plant management (Palmer, Heard, and Sheppard Citation2010).
Chemical control
The IUCN/SSC Invasive Species Specialist Group has listed several broad-spectrum herbicides that can be used to control Carpobrotus, but the standard herbicide is glyphosate. This herbicide has been effective at concentrations of 2% or higher, and the addition of 1% surfactant to break apart the cuticle on the leaves increases plant mortality (Ministerio de Medio Ambiente, y Medio Rural y Marino Citation2011). Moreover, as of 18 July 2017, the CAL-IPC (California Invasive Plant Council) listed on its website that adding an acidifier to water before mixing with glyphosate can increase the effectiveness of the treatment. In Ireland, C. edulis was shown to be also particularly susceptible to the commercial herbicide mix of glyphosate and diquat (Smyth, Jebb, and Booth Citation2011). In Portugal, Guerreiro (Citation1977) also screened other herbicides to control C. edulis. Although glyphosate gave the most rapid and complete control, a mix of paraquat, simazine and benzoylprop-ethyl provided satisfactory results.
In the Natural Park of Albufera (Valencia, Spain), two years after its application, glyphosate persisted in the soil and had a harmful effect on the native flora (Lotus creticus L., Elymus farctus (Viv.) Runemark ex Melderis and Malcolmia littorea). Therefore, despite the effectiveness of glyphosate to control C. edulis, it is not recommended to use it in sandy soils (Hueso Alcaide Citation2017). However, this problem could be solved by adjusting the dose (Fagúndez and Barrada Citation2007) and by treating the target species in early or mid-winter when most native plants are dormant (Sanz-Elorza, Dana, and Sobrino Citation2004). For example, Torre Fernández and Alvarez-Arbesú (Citation1999) sprayed C. edulis with Roundup Plus (glyphosate 36%) in the Xagó dunes (Asturias, Spain). All C. edulis plants died within two or three months while the native species (Ammophila arenaria (L.) Link., Crucianella maritima L., Pancratium maritimum L.) were not affected by the herbicide. In any case, before applying any particular herbicide, it is important to verify that no legislation bans or restricts its use in the area. The current debate over the use of glyphosate in the European Union (Gillam Citation2017) may altogether restrict or forbid its use in the future.
Overall, since the adjacent vegetation may also be negatively affected by herbicides (Fagúndez and Barrada Citation2007; Marchante et al. Citation2014), chemical control may only be useful when Carpobrotus is found in pure clumps (Schmalzer and Hinkle Citation1987). It may also be appropriate to employ chemical control after manual removal of Carpobrotus, in order to avoid re-establishment from seeds (Kelly and Maguire Citation2009). However, physical removal (hand-pulling and buried stem removal) is still considered as the most effective and cost-efficient method to control the invasion of Carpobrotus (Fraga et al. Citation2005).
Physical control
Hand pulling has been shown to increase plant biodiversity in the controlled areas (Andreu et al. Citation2010; Krebs et al. Citation2015). In sandy soils, it does not require a high physical effort. Therefore, it is an effective way for controlling small patches of Carpobrotus (Albert Citation1995b). However, manual pulling can be time consuming when controlling large Carpobrotus patches. In such cases, the most effective way of controlling Carpobrotus is by rolling up the Carpobrotus mat from one side while severing the roots underneath with shovels. Alternatively, removal with a brush rake can also be effective (Albert Citation1995b).
Once hand pulled, the plant material should be moved to a “secure” place to dry and, if possible, it should be covered with a black plastic to accelerate the drying process and to kill roots and fragments that could otherwise regenerate. However, the transport and disposal of plant material may offer serious logistic problems (Fraga et al. Citation2005). As of 18 July 2017, “Plantas Invasoras em Portugal” listed on its website that, in these cases, the plant material can be left on site, avoiding the direct contact of the roots with the substrate. Moreover, controlled areas should be monitored for at least a decade to remove seedlings emerging from the seedbank and plants growing from fragments left during the initial clearing (Ruffino et al. Citation2015).
Prescribed fire. Fire apparently kills Carpobrotus seeds stored in the topsoil and this was confirmed by laboratory experiments with exposition at temperatures over 90°C for 5 min (D’Antonio, Odion, and Tyler Citation1993). However, fire with mild temperatures was found to possibly stimulate the germination of seeds and increase C. edulis and C. modestus S.T.Blake abundance in California and Australia, respectively (D’Antonio, Odion, and Tyler Citation1993; Parsons Citation1997). Moreover, due to an improvement in soil conditions after fire, Carpobrotus seedlings establishment and growth may be enhanced (D’Antonio, Odion, and Tyler Citation1993). Therefore, the use of control burning in areas where Carpobrotus is present must be carefully considered.
Solarization. Covering the soil with plastic sheets may also be used to control small Carpobrotus infestations. However, it is not a recommended method to control big infestations, since it may cause significant physical, chemical and biological changes in the soil that can last for several years (Tu, Hurd, and Randall Citation2001). Moreover, Theiss (Citation1994) did not found this method to be particularly effective to control Carpobrotus invasions.
Biological control
Biological control may be the best managing option for inaccessible areas and dense, monospecific mats where chemical application or mechanical/manual removal is extremely difficult (see ). However, as for many biological invasions, biological control involving Carpobrotus remains unexplored and underutilized and little information is available on the issue.
In California, several pathogens have been isolated from the roots and stems of symptomatic C. edulis plants (MacDonald, Hartman, and Shapiro Citation1984). Pathogenicity tests demonstrated that Pythium aphanidermatum ((Edson) Fitzp., 1923), Phytophthora cryptogea (Pethybr. & Lafferty, 1931), Phomopsis sp. and Verticillium dahliae (Klebahn, 1913) were the causal agent of diseased individual or patches of plants (wilted, chlorotic or dead) (see plant parasites and diseases section). In the Global Invasive Species Database (GISD Citation2017), it was reported that Verticillium dahliae can cause considerable damage to C. edulis; however its utilization must be carefully considered because it can also infect some important crop species (McCain, Raabe, and Wilhelm Citation1981). In Japan, Botrytis cinerea Pers. caused damage on the co-occurring Aizoaceae species Mesembryanthemum crystallinum (Kuzniak et al. Citation2010), but again it is not considered appropriate due to its broad host range.
Insects. In the 1970s, two accidentally introduced soft-scale insects (Pulvinariella mesembryanthemi and Pulvinaria delottoi) caused severe damage to C. edulis in California (Washburn and Frankie Citation1981; Schmalzer and Hinkle Citation1987), where it had been used in roadside planting to avoid erosion. However, a successful scale management program was developed (Washburn and Frankie Citation1981, Citation1985; Tassan, Kenneth, and Cassidy Citation1982), by introducing several natural enemies (predators and parasitoids) to control the mentioned scale insects because C. edulis was considered to be a desirable plant (Schmalzer and Hinkle Citation1987). These predators and parasitoids were so effective that currently soft scale insects cannot be used as control agent in the invaded Point Reyes National Seashore and other protected areas of California.
In South Africa, two diaspine scale species, Aonidiella mesembryanthemae and Entaspidiotus lounsburyi, attack the leaves of Carpobrotus, although they are usually rare and intensely parasitized in their natural environment in the Cape Provinces. Nevertheless, E. lounsburyi can be very destructive on Carpobrotus spp. populations grown in Pretoria, even when these insects are severely parasitized (S. Neser pers. comm.).
Since 2015, a biological control program to assess the impact of individual and combined effects of two possible control agents is being developped by the Functional Plant Ecology Group of the University of Santiago de Compostela, Spain. Under restricted greenhouse conditions, the generalist pathogenic fungus Sclerotinia sclerotiorum Lib. ((De Bary), 1884) and the soft scale insect P. mesembryanthemi are being tested. Although both agents seem to be able to affect negatively C. edulis, only P. mesembryanthemi decreased significantly the plant survival over a 6-month period (Vieites-Blanco, Retuerto, and Lema Citation2017). Also, periodical surveys are being carried out to monitor the effects of P. mesembryanthemi on the density, growth and fertility of naturally infested Carpobrotus populations occupying Atlantic coastal areas and to determine demographic changes of naturally infested Carpobrotus in the Northwest of the Iberian Peninsula. The aim of this biocontrol program is also to define the life cycle of the soft scale insect in the environmental conditions studied and to evaluate the extent of damage caused by natural parasitism in order to assess their use as control agent in this area. The direct or indirect effects on native species and the conservation benefits of biological control programs must be studied in depth.
Since control of many plant invasions has been achieved by introducing natural enemies, new surveys in the native range of Carpobrotus might help to find host specific or host restricted biological control candidates (eg Mesocelis monticola, see biotic factors section).
Grazing. Grazing by generalist species such as deers or rabbits can reduce the establishment and growth of Carpobrotus (D’Antonio, Odion, and Tyler Citation1993; D’Antonio Citation1993; Vilà and D’Antonio Citation1998b), although hybrids of C. edulis and C. chilensis are much less sensitive to grazing than C. chilensis (Vilà and D’Antonio Citation1998c). Despite the potential of grazing to control Carpobrotus, if fruits are also grazed, Carpobrotus seeds might be dispersed (see fruit/seed dispersal section). It is therefore not clear whether grazing can be used for management (D’Antonio and Thomsen Citation2004) knowing that generalist herbivores may have an overall effect that is facilitative rather than negative on invasive plant abundance (Maron and Vilà Citation2001).
Control costs
Control and eradication campaigns of Carpobrotus have been accomplished in the last two decades in different European countries (see section on eradication and control plans for more information) but, in most cases, control costs were not quantified.
In Spain, it has been reported that the total economic costs of Carpobrotus control between 2002 and 2007 was € 2,886,683 (0.58 million € per year). These funds were mostly spent in reducing populations through mechanical methods (Andreu, Vilà, and Hulme Citation2009). According to Andreu and Vilà (Citation2007), in Spain, Carpobrotus has been controlled in eight Autonomous Communities. In Menorca, between 2002 and 2005, Carpobrotus was eradicated in a total coastal area of 233,785 m2 formerly colonized, for a total amount of 9,041 working hours. As a result of this campaign, 24 out of 27.8 ha of Carpobrotus (832,148 kg) were removed (Fraga I Arguimbau Citation2007).
In Italy, the removal of Carpobrotus in several Islands was accomplished under several LIFE funded projects and the costs of its eradication were valued. For example, in Pianosa Island, where 25 ha of land were cleaned, the removal of Carpobrotus spp., among other invasive species, was estimated to 9.4 € per m2; in Linosa Island (Strait of Sicily) eradication of alien plants including C. cf. acinaciformis was around € 120,000; in Tavolara Island, the elimination of Carpobrotus was completed for a total cost of € 50,000; in the Sardinian coast, the removal of 606 ha of Carpobrotus spp. charged € 24,362 and in three islands in the Ponziane Archipelago, € 25,000 for cleaning 340 ha, while the expenses in Trapani province (western Sicily) were € 28,664 (Scalera et al. Citation2017).
In France, control costs for C. edulis and C. acinaciformis in the Mainland between 2009 and 2013 was € 167,000, with an annual expenditure of € 33,000 (EPPO Citation2018a, Reporting Service no. 11 – 2015 Num. article: 2015/215). Also, in 2003 a collaboration between a nursery and landscaping industry and “Conservatoire Botanique National Méditerranéen” of Porquerolles was initiated. In this context, local initiatives for C. acinaciformis eradication were carried out (Wittmann and Flores-Ferrer Citation2015).
In Ireland, the Heritage Council provided € 15,000 to control C. edulis during 2011 (Kelly et al. Citation2013). Also, it has been reported that control of C. edulis was conducted in Dublin (EPPO Citation2018b, Reporting Service no. 09 – 2013 Num. article: 2013/208).
Restoration
After the application of any of the above control methods, a specific restoration plan must be implemented. The remaining living parts and litter of Carpobrotus should be removed; the controlled areas should be over-seeded with the appropriate native species, and the establishment of opportunistic ruderal species should be avoided (Novoa et al. Citation2013; Fried et al. Citation2014).
Legacy effects, including changes in soil characteristics, and accumulation of allelochemicals and Carpobrotus seeds on the litter, may persist after the removal (for more information see negative impacts section). Ruderal opportunistic species may also benefit from these legacy effects (Novoa et al. Citation2013; Fried et al. Citation2014) due to their greater plasticity and opportunistic strategy or high abundance in the seed bank (Maurel et al. Citation2010). Therefore, a combination of legacy effects and competition with ruderal species and Carpobrotus may prevent the establishment of native dune species in the eradicated or controlled areas (Novoa et al. Citation2013; Novoa and González Citation2014).
To successfully restore dune ecosystems invaded by Carpobrotus, plants must be removed in the first stages of the invasion, to avoid the strong effects on soil conditions. This should be followed by the removal of necromass to avoid legacy effects (Vieites-Blanco and González-Prieto Citation2017), and by the removal of opportunistic ruderal species and Carpobrotus seedlings that are likely to constrain the establishment of the native dune species (Novoa et al. Citation2013). However, removing the litter may lead to soil erosion. To reduce such erosion, the bare ground can be covered with geotextile (Bhattacharyya et al. Citation2010). A different option is to leave a 50 cm strip of Carpobrotus plants and only remove it once native species have been established (Chenot et al. Citation2018). However, this strip needs to be monitored to prevent Carpobrotus recolonization.
Acknowledgments
The authors wish to thank two anonymous reviewers for useful suggestions and comments on a previous version of this manuscript.
Disclosure statement
No potential conflict of interest was reported by the authors.
Additional information
Funding
Notes on contributors
Josefina G. Campoy
Josefina G. Campoy is a plant ecologist with interests in plant invasions who is currently working in her PhD of Carpobrotus at the University of Santiago de Compostela. Contribution: She drafted some of the sections, contributing particularly to the description of the distribution in the invaded area. She also coordinated the team during the writing of the manuscript, provided additions and comments, and reviewed the final draft.
Alicia T. R. Acosta
Alicia T. R. Acosta is a plant ecologist at the Università degli Studi Roma TREE with a range of interests in community ecology but particularly concerned in understanding how sandy coastal ecosystems function, and how they will respond to ongoing environmental changes. Contribution: She contributed to the section of Negative impacts and reviewed the final draft.
Laurence Affre
Laurence Affre is a plant ecologist associate professor at the Aix Marseille University and IMBE (France). She conducts researches on population dynamics and structure of rare, common and invasive plants. Contribution: She contributed to the section Reproductive biology, and reviewed the final draft.
Rodolfo Barreiro
Rodolfo Barreiro is an ecologist at the University of A Coruña, with an interest in the use of molecular techniques to address issues in ecology. Contribution: He contributed to the section of the history of the introduction and spread and reviewed the final draft.
Giuseppe Brundu
Giuseppe Brundu is agronomist and botanist at the University Sassari, with interests in prevention, management and control of plant invasions. Contribution: He contributed to the section on Taxonomy and Legislation and reviewed the final draft.
Elise Buisson
Elise Buisson is an associate professor at Université d’Avignon et des Pays de Vaucluse and IMBE. She conducts research on plant communities, their functioning and vulnerability, and is interested in their organization, their resilience and their restoration. Contribution: She contributed to the sections of Reproductive Biology and Management and reviewed the final draft.
Luís González
Luís González is a plant physiology professor at the Faculty of Biology of the University of Vigo, Spain. He is interested in invasive plants ecophysiology, native/invasive relationship, mainly in protected areas. Contribution: He contributed to the sections Ecological constrains, climate and substratum and Responses to abiotic factors (salinity and nutrients availability) and reviewed the final draft.
Margarita Lema
Margarita Lema is a plant breeder and geneticist involved in biological control studies at the University of Santiago de Compostela. Contribution: She contributed to the sections of biotic factors, control cost and biological control and reviewed the final draft.
Ana Novoa
Ana Novoa is a Scientist at the Department of Invasion Ecology of the Institute of Botany of the Czech Academy of Sciences, with interests in the ecology and management of invasive plants globally and in the engagement of stakeholders in the management of invasive species. Contribution: She contributed to the sections of habitats and syntaxonomy in the native range, the economic importance and human uses, and the chemical and physical control, and reviewed the final draft.
Rubén Retuerto
Rubén Retuerto is a full Professor of Ecology in the Department of Functional Biology at the University of Santiago de Compostela, with interests in understanding how plants interact with their physical, chemical and biotic environments to determine plant performance. Contribution: He contributed to the section of abiotic factors (light, water and temperature), and reviewed the final draft.
Sergio R. Roiloa
Sergio R. Roiloa is an Assistant Professor of Ecology at the University of A Coruña, with interests in clonal plant ecology and biological invasions. Contribution: He contributed to the section of phenology and vegetative growth and reviewed the final draft.
Jaime Fagúndez
Jaime Fagúndez is a botanist and plant ecologist at the University of A Coruña, with interests in semi-natural habitats and species’ management. Contribution: He conceived the study, contributed to the sections of identification conflicts and habitats and syntaxonomy in the invaded range, and reviewed the final draft. He also coordinated the team during the writing of the manuscript.
Notes
1. Convention on the Conservation of European Wildlife and Natural Habitats, Bern, 19.IX.1979Revathy.
2. Regulation (EU) No 1143/2014 of the European Parliament and of the Council of 22 October 2014 on the prevention and management of the introduction and spread of invasive alien species.
4. HD = Council Directive 92/43/EEC of 21 May 1992 on the conservation of natural habitats and of wild fauna and flora.
References
- Acosta, A., C. F. Izzi, and A. Stanisci. 2006. “Comparison of Native and Alien Plant Traits in Mediterranean Coastal Dunes.” Community Ecology 7 (1): 35–41. doi:10.1556/ComEc.7.2006.1.4.
- Affre, L., C. M. Suehs, S. Charpentier, M. Vilà, G. Brundu, P. Lambdon, A. Traveset, and P. E. Hulme. 2010. “Consistency on the Habitat Degree of Invasion for Three Invasive Plant Species across Mediterranean Islands.” Biological Invasions 12 (8): 2537–2548. doi:10.1007/s10530-009-9662-6.
- Aigner, P. A. 2004. “Ecological and Genetic Effects on Demographic Processes: Pollination, Clonality and Seed Production in Dithyrea maritima.” Biological Conservation 116 (1): 27–34. doi:10.1016/S0006-3207(03)00170-8.
- Akeroyd, J. R., and C. D. Preston. 1990. “Notes on Some Aizoaceae Naturalized in Europe.” Botanical Journal of the Linnean Society 103: 197–200.
- Albert, M. E. 1995a. Morphological Variation and Habitat Associations within the Carpobrotus Species Complex in Coastal California. Berkeley: University of California.
- Albert, M. E. 1995b. “Portrait of an Invader II: The Ecology and Management of Carpobrotus edulis.” CalEPPC News 3: 4–6.
- Albert, M. E., C. M. D’Antonio, and K. A. Schierenbeck. 1997. “Hybridization and Introgression in Carpobrotus spp. (Aizoaceae) in California. I. Morphological Evidence.” American Journal of Botany 84 (7): 896–904. doi:10.2307/2446279.
- Alpert, P., and J. F. Stuefer. 1997. “Division of Labour in Clonal Plants.” In The Ecology and Evolution of Clonal Plants, edited by H. De Kroon and J. Van Groenendael, 137–154. Leiden: Backhuys Publishers.
- Andreu, J., E. Manzano-Piedras, I. Bartomeus, E. D. Dana, and M. Vilà. 2010. “Vegetation Response after Removal of the Invasive Carpobrotus Hybrid Complex in Andalucía, Spain.” Ecological Restoration 28 (4): 440–448.
- Andreu, J., and M. Vilà. 2007. “Análisis de la gestión de las plantas exóticas en los espacios naturales españoles. [Management of Invasive Alien Plant Species in Spanish Protected Areas].” Ecosistemas 16 (3): 109–124.
- Andreu, J., M. Vilà, and P. E. Hulme. 2009. “An Assessment of Stakeholder Perceptions and Management of Noxious Alien Plants in Spain.” Environmental Management 43 (6): 1244–1255. doi:10.1007/s00267-009-9280-1.
- Arianoutsou, M., I. Bazos, P. Delipetrou, and Y. Kokkoris. 2010. “The Alien Flora of Greece: Taxonomy, Life Traits and Habitat Preferences.” Biological Invasions 12 (10): 3525–3549. doi:10.1007/s10530-010-9749-0.
- Arslan, Z. F., A. Uludag, and I. Uremis. 2016. “Current Status of Invasive Alien Plants in EPPO Lists in Turkey.” In Turkey 6th Plant Protection Congress, produced by the International Association for the Plant Protection Sciences (IAPPS), Konya, Turkey, September 5–8.
- Ashmole, P., and M. Ashmole. 2000. “St. Helena and Ascension Island: A Natural History.” In Plants, 403–466. Oswestry, Shropshire: Anthony Nelson.
- Atlas of Living Australia Website. 2017. “Carpobrotus edulis.” Accessed July 15 2017. http://www.ala.org.au/.
- Badalamenti, E., L. Gristina, V. A. Laudicina, A. Novara, S. Pasta, and T. La Mantia. 2016. “The Impact of Carpobrotus cf. acinaciformis (L.) L.Bolus on Soil Nutrients, Microbial Communities Structure and Native Plant Communities in Mediterranean Ecosystems.” Plant and Soil 409 (1–2): 19–34. doi:10.1007/s11104-016-3076-x.
- Baker, J. G. 1871. “On the Botany of the Lizard Peninsula.” Journal of Botany 9: 353–358.
- Bardsley, D. K., and G. Edwards-Jones. 2007. “Invasive Species Policy and Climate Change: Social Perceptions of Environmental Change in the Mediterranean.” Environmental Science & Policy 10 (3): 230–242. doi:10.1016/j.envsci.2006.12.002.
- Barina, Z., M. Rakaj, G. Somogyi, Z. Erős‐Honti, and D. Pifkó. 2014. “The Alien Flora of Albania: History, Current Status and Future Trends.” Weed Research 54 (2): 196–215. doi:10.1111/wre.12061.
- Bartomeus, I., J. Bosch, and M. Vilà. 2008. “High Invasive Pollen Transfer, yet Low Deposition on Native Stigmas in a Carpobrotus-Invaded Community.” Annals of Botany 102 (3): 417–424. doi:10.1093/aob/mcn109.
- Bartomeus, I., and M. Vilà. 2009. “Breeding System and Pollen Limitation of Two Supergeneralist Alien Plants Invading Mediterranean Shrublands.” Australian Journal of Ecology 57: 109–115. doi:10.1071/BT08169.
- Bartomeus, I., M. Vilà, and L. Santamaría. 2008. “Contrasting Effects of Invasive Plants in Plant–Pollinator Networks.” Oecologia 155 (4): 761–770. doi:10.1007/s00442-007-0946-1.
- Bazzichetto, M., M. Malavasi, A. T. R. Acosta, and M. L. Carranza. 2016. “How Does Dune Morphology Shape Coastal EC Habitats Occurrence? A Remote Sensing Approach Using Airborne LiDAR on the Mediterranean Coast.” Ecological Indicators 71: 618–626. doi:10.1016/j.ecolind.2016.07.044.
- Bech, J., and A. M. Hernández. 1976. “Estudios sobre suelos y vegetación del delta del Llobregat. [A Study on Soils and Vegetation of the Delta of the Llobregat Area].” Collectanea Botanica 10: 31–105.
- Berti, M., R. Wilckens, S. Fischer, A. Solis, and B. Johnson. 2011. “Seeding Date Influence on Camelina Seed Yield, Yield Components, and Oil Content in Chile.” Industrial Crops and Products 34 (2): 1358–1365.
- Bhattacharyya, R., T. Smets, M. A. Fullen, J. Poesen, and C. A. Booth. 2010. “Effectiveness of Geotextiles in Reducing Runoff and Soil Loss: A Synthesis.” Catena 81 (3): 184–195. doi:10.1016/j.catena.2010.03.003.
- Biffin, E., H. R. Toelken, K. Van Dijk, J. Kellermann, and M. Waycott. 2016. “An Assessment of Native and Introduced Carpobrotus spp. In the AMLR Region.” Final report for Natural Resources Adelaide and Mt Lofty Ranges. Adelaide: State Herbarium of South Australia, Department of Environment, Water and Natural Resources. 21pp.
- Blake, S. T. 1969. “A Revision of Carpobrotus and Sarcozona in Australia, Genera Allied to Mesembryanthemum (Aizoaceae).” Contributions to the Queensland Herbarium 7: 1–65.
- Boršić, I., M. Milović, I. Dujmović, S. Bogdanović, P. Cigić, I. Rešetnik, T. Nikolić, and B. Mitić. 2008. “Preliminary Check-List of Invasive Alien Plant Species (IAS) in Croatia.” Natura Croatica Periodicum Musei Historiae Naturalis Croatici 17 (2): 55–71.
- Bourgeois, K., C. M. Suehs, E. Vidal, and F. Médail. 2005. “Invasional Meltdown Potential: Facilitation between Introduced Plants and Mammals on French Mediterranean Islands.” Ecoscience 12 (2): 248–256. doi:10.2980/i1195-6860-12-2-248.1.
- Brandes, D. 2001. Urban Flora of Sousse (Tunisia). Brunswick: Publications of the Working Group on Vegetation Ecology and Experimental Plant Sociology Botanical Institute and Botanical Garden of the TU Braunschweig. Accessed May 7 2018. http://www. digibib.tu-bs.de.
- Brown, A. H. D., and J. J. Burdon. 1987. “Mating Systems and Colonizing Success in Plants.” In The 26th symposium of the British Ecological Society held jointly with the Linnean Society of London, edited by A. J. Gray, M. J. Crawley, and P. J. Edwards, 115–131. Oxford: Blackwell Scientific Publications.
- Cabello, J. 2009. “Aizoaceae.” In Flora Vascular de Andalucía Oriental [Vascular Flora of Eastern Andalusia], edited by G. Blanca, B. Cabezudo, M. Cueto, C. Fernández López, and C. Morales Torres, 180–185. II vols. Sevilla: Consejería de Medio ambiente, Junta de Andalucía.
- CABI. 2017. “‘Carpobrotus edulis.’ [Original Text by C. Parker].” In Invasive Species Compendium (ISC). Wallingford, UK: CAB International. Accessed July 15 2017. www.cabi.org/isc.
- Cal-IPC. 2006. California Invasive Plant Inventory. “Carpobrotus edulis”. (California Invasive Plant Council). Accessed July 15 2017. http://www.cal-ipc.org/
- Callaway, R. M., S. Jones Jr., W. R. Ferren, and A. Parikh. 1990. “Ecology of a Mediterranean-Climate Estuarine Wetland at Carpinteria, California: Plant Distributions and Soil Salinity in the Upper Marsh.” Canadian Journal of Botany 68 (5): 1139–1146.
- Calviño-Cancela, M., R. Méndez-Rial, J. Reguera-Salgado, and J. Martín-Herrero. 2014. “Alien Plant Monitoring with Ultralight Airborne Imaging Spectroscopy.” PLoS ONE 9 (7): e102381.
- Camarda, I., T. A. Cossu, L. Carta, A. Brunu, and G. Brundu. 2016. “An Updated Inventory of the Non-Native Flora of Sardinia (Italy).” Plant Biosystems 150 (5): 1106–1118. doi:10.1080/11263504.2015.1115438.
- Campos, J. A., and M. Herrera. 2009. “Análisis de la Flora Alóctona de Bizkaia (País Vasco, España). [Analysis of the Alien Flora in Bizkaia (Basque Country, Spain)].” Lazaroa 30: 7–33.
- Campos, J. A., M. Herrera, I. Biurrun, and J. Loidi. 2004. “The Role of Alien Plants in the Natural Coastal Vegetation in Central-Northern Spain.” Biodiversity and Conservation 13 (12): 2275–2293. doi:10.1023/b:bioc.0000047902.27442.92.
- Campoy, J. G., R. Retuerto, and S. R. Roiloa. 2017. “Resource Sharing Strategies in Ecotypes of the Invasive Clonal Plant Carpobrotus edulis: Specialization for Abundance or Scarcity of Resources.” Journal of Plant Ecology 10 (4): 681–691. doi:10.1093/jpe/rtw073.
- Carranza, M. L., C. Ricotta, M. Carboni, and A. T. R. Acosta. 2011. “Habitat Selection by Invasive Alien Plants: A Bootstrap Approach.” Preslia 83 (4): 529–536.
- Carranza, M. L., M. Carboni, S. Feola, and A. T. R. Acosta. 2010. “Landscape-Scale Patterns of Alien Plant Species on Coastal Dunes: The Case of Iceplant in Central Italy.” Applied Vegetation Science 13 (2): 135–145. doi:10.1111/j.1654-109X.2009.01065.x.
- CEEEI. 2013. “Catálogo Español de Especies Exóticas Invasoras Ficha CAREDU/EEI/FL020 [Spanish Catalog of Invasive Alien Species Sheet CAREDU/EEI/FL020].” Accessed April 25 2017. http://www.mapama.gob.es/es/biodiversidad/temas/conservacion-de-especies/especies-exoticas-invasoras/ce_eei_flora.aspx.
- Chenot, J., L. Affre, A. Passetti, and E. Buisson. 2014. “Consequences of Iceplant (Carpobrotus) Invasion on the Vegetation and Seed Bank Structure on a Mediterranean Island: Response Elements for Their Local Eradication.” Acta Botanica Gallica 161 (3): 301–308. doi:10.1080/12538078.2014.910473.
- Chenot, J., L. Affre, R. Gros, L. Dubois, S. Malecki, A. Passetti, A. Aboucaya, and E. Buisson. 2018. “Eradication of Invasive Carpobrotus sp.: Effects on Soil and Vegetation.” Restoration Ecology. Restoration Ecology 26 (1): 106–113. doi:10.1111/rec.12538.
- Chinnock, R. J. 1972. “Natural Hybrids between Disphyma and Carpobrotus (Aizoaceae) in New Zealand.” New Zealand Journal of Botany 10 (4): 615–625.
- Clavreul, D. 2008. Histoire Illustrée du Jardin Exotique de Batz Créé par Georges Delaselle. [Illustrated History of Batz Exotic Garden Created by Georges Delaselle]. Paris: Gallimard.
- Clement, E. J., and M. C. Foster. 1994. Alien Plants of the British Isles: A Provisional Catalogue of Vascular Plants (Excluding Grasses), Handbooks for Field Identification Series. London: Botanical Society of the British Isles.
- Codd, L. E., and M. Gunn. 1985. “Additional Biographical Notes on Plant Collectors in Southern Africa.” Bothalia 15 (3/4): 631–654.
- Collins, L., and J. K. Scott. 1982. “Interaction of Ants, Predators, and the Scale Insect, Pulvinariella mesembryanthemi, on Carpobrotus edulis, an Exotic Plant Naturalized in Western Australia.” Australian Entomological Magazine 8 (5): 73–78.
- Conser, C., and E. F. Connor. 2009. “Assessing the Residual Effects of Carpobrotus edulis Invasion, Implications for Restoration.” Biological Invasions 11 (2): 349–358. doi:10.1007/s10530-008-9252-z.
- Costa, J. C., J. Capelo, C. Neto, M. D. Espírito-Santo, and M. Lousa. 1997. “Notas fitosociológicas sobre os tojais do Centro e Sul de Portugal. [Phytosociological Notes on Gorse Communities from Center and South Portugal].” Silva Lusitana 5 (2): 275–282.
- Cowling, R. M., I. A. W. Macdonald, and M. T. Simmons. 1996. “The Cape Peninsula, South Africa: Physiographical, Biological and Historical Background to an Extraordinary Hot-Spot of Biodiversity.” Biodiversity and Conservation 5 (5): 527–550.
- Cullen, J. 2011. “Carpobrotus.” In The European Garden Flora: Flowering Plants, edited by J. Cullen, S. G. Kness, and H. S. Cubey, 71. III vols, 640. Cambridge: Cambridge University Press.
- D’Antonio, C. M. 1990a. “Invasion of coastal plant communities by the introduced succulent, Carpobrotus edulis (Aizoaceae).” PhD diss., University of California, Santa Barbara.
- D’Antonio, C. M. 1990b. “Seed Production and Dispersal in the Non-Native, Invasive Succulent Carpobrotus edulis (Aizoaceae) in Coastal Strand Communities of Central California.” Journal of Applied Ecology 27 (2): 693–702. doi:10.2307/2404312.
- D’Antonio, C. M. 1993. “Mechanisms Controlling Invasion of Coastal Plant-Communities by the Alien Succulent Carpobrotus edulis.” Ecology 74 (1): 83–95. doi:10.2307/1939503.
- D’Antonio, C. M., and B. E. Mahall. 1991. “Root Profiles and Competition between the Invasive, Exotic Perennial, Carpobrotus edulis, and Two Native Shrub Species in California Coastal Scrub.” American Journal of Botany 78 (7): 885–894. doi:10.2307/2445167.
- D’Antonio, C. M., D. C. Odion, and C. M. Tyler. 1993. “Invasion of Maritime Chaparral by the Introduced Succulent Carpobrotus edulis.” Oecologia 95 (1): 14–21. doi:10.1007/BF00649501.
- D’Antonio, C. M., J. Levine, and M. Thomsen. 2001. “Ecosystem Resistance to Invasion and the Role of Propagule Supply: A California Perspective.” Journal of Mediterranean Ecology 2: 233–245.
- D’Antonio, C. M., and L. A. Meyerson. 2002. “Exotic Plant Species as Problems and Solutions in Ecological Restoration: A Synthesis.” Restoration Ecology 10 (4): 703–713.
- D’Antonio, C. M., and M. Thomsen. 2004. “Ecological Resistance in Theory and Practice.” Weed Technology 18: 1572–1577. doi:10.1614/0890-037x(2004)018[1572:eritap]2.0.co;2.
- Dall’Osto, L., C. Lico, J. Alric, G. Giuliano, M. Havaux, and R. Bassi. 2006. “Lutein Is Needed for Efficient Chlorophyll Triplet Quenching in the Major LHCII Antenna Complex of Higher Plants and Effective Photoprotection in Vivo under Strong Light.” BMC Plant Biology 6 (1): 32. doi:10.1186/1471-2229-6-32.
- Dana, E. D., M. Sanz-Elorza, S. Vivas, and E. Sobrino. 2005. Especies Vegetales invasoras en Andalucía. [Invasive Plant Species in Andalusia]. Sevilla: Dirección General de la Red de Espacios Naturales Protegidos y Servicios Ambientales. Consejería de Medio Ambiente, Junta de Andalucía.
- Davey, F. H. 1909. Flora of Cornwall. Penryn: Chegwidden.
- Dehnen-Schmutz, K., M. L. Chas-Amil, and J. Touza. 2010. “Stakeholders’ Perceptions of Plant Invasions in Galicia, Spain.” Aspects of Applied Biology 104: 13–18.
- Delipetrou, P. 2009. “Carpobrotus edulis (L.) N.E.Br., Freeway Iceplant (Aizoaceae Magnoliophyta).” In The Handbook of European Alien Species, ed. DAISIE, 399, 345. Dordrecht: Springer.
- Delotto, G. 1979. “Sost Scales (Homoptera, Coccidae) of South-Africa. 4.” Journal of the Entomological Society of Southern Africa 42 (2): 245–256.
- Demmig-Adams, B., and W. W. Adams. 1992. “Photoprotection and Other Responses of Plants to High Light Stress.” Annual Review of Plant Physiology 43 (1): 599–626.
- Demmig-Adams, B., and W. W. Adams. 1996. “The Role of Xanthophyll Cycle Carotenoids in the Protection of Photosynthesis.” Trends in Plant Science 1 (1): 21–26.
- Dong, B. C., P. Alpert, W. Guo, and F. H. Yu. 2012. “Effects of Fragmentation on the Survival and Growth of the Invasive, Clonal Plant Alternanthera philoxeroides.” Biological Invasions 14 (6): 1101–1110. doi:10.1007/s10530-011-0141-5.
- Dufour-Dror, J. M., ed. 2013. Israel’s Least Wanted Alien Ornamental Plant Species. Potentially Invasive in Israel’s Natural Ecosystems.Technical Report. Israel: Israel Ministry of Environmental Protection, Ministry of Agriculture, Nature & Parks Authority, and Hebrew University Botanical Gardens. 19 pp.
- Earnshaw, M. J., K. A. Carver, and W. A. Charlton. 1987. “Leaf Anatomy, Water Relations and Crassulacean Acid Metabolism in the Chlorenchyma and Colourless Internal Water-Storage Tissue of Carpobrotus edulis and Senecio mandraliscae.” Planta 170 (3): 421–432.
- Ellstrand, N. C., and K. A. Schierenbeck. 2000. “Hybridization as a Stimulus for the Evolution of Invasiveness in Plants?” Proceedings of the National Academy of Sciences 97 (3): 7043–7050. doi:10.1073/pnas.97.13.7043.
- Elton, C. S. 1958. The Ecology of Invasions by Animals and Plants. Chicago: University of Chicago Press.
- EPPO (European and Mediterranean Plant Protection Organization). 2006. EPPO Lists of Invasive Alien Plants. Accessed May 7 2018. https://gd.eppo.int.
- EPPO Global Database. 2017. Reporting Service no. 08-2013 Num. article: 2013/178. Accessed May 7 2018. https://gd.eppo.int.
- EPPO Global Database. 2018a. Reporting Service no. 11-2015 Num. article: 2015/215. Accessed May 7 2018. https://gd.eppo.int.
- EPPO Global Database. 2018b. Reporting Service no. 09-2013 Num. article: 2013/208. Accessed May 7 2018. https://gd.eppo.int.
- Eswaran, H., and P. Reich. 2005. Global Soil Regions. 1: 130,000,000. Global Soil Suborder Map, 1. 5,000,000. United States Department of Agriculture. Accessed May 8 2018. https://www.nrcs.usda.gov/wps/portal/nrcs/site/national/home/
- Fagúndez, J., and M. Barrada. 2007. Plantas Invasoras de Galicia. [Invasive Alien Plant Species of Galicia], 209. Santiago de Compostela: Consellería de Medio Ambiente, Xunta de Galicia.
- Falleh, H., R. Ksouri, M. Boulaaba, S. Guyot, C. Abdelly, and C. Magné. 2012. “Phenolic Nature, Occurrence and Polymerization Degree as Marker of Environmental Adaptation in the Edible Halophyte Mesembryanthemum edule.” South African Journal of Botany 79: 117–124. doi:10.1016/j.sajb.2011.10.001.
- FAO-Unesco. 1971. Soil Map of the World, 1:5,000,000. IV vols. South America. Paris: UNES.
- FAO-Unesco. 1977. Soil Map of the World, 1:5,000,000. VI vols. Africa. Paris: UNES.
- FAO-Unesco. 1978. Soil Map of the World, 1:5,000,000. X vols. Australasia.Paris: UNES.
- FAO-Unesco. 1981. Soil Map of the World, 1:5,000,000. V vols. Europe. Paris: UNES.
- Farrell, C., R. E. Mitchell, C. Szota, J. P. Rayner, and N. S. G. Williams. 2012. “Green Roofs for Hot and Dry Climates: Interacting Effects of Plant Water Use, Succulence and Substrate.” Ecological Engineering 49: 270–276. doi:10.1016/j.ecoleng.2012.08.036.
- Faulkner, K. T., B. P. Hurley, M. P. Robertson, M. Rouget, and J. R. U. Wilson. 2017. “The Balance of Trade in Alien Species between South Africa and the Rest of Africa.” Bothalia-African Biodiversity & Conservation 47 (2): 1–16.
- Fédération des Conservatoires botaniques nationaux. 2013. Accessed February 2 2018. http://www.fcbn.fr/si-flore/.
- Fenollosa, E., D. A. Roach, and S. Munné-Bosch. 2016. “Death and Plasticity in Clones Influence Invasion Success.” Trends in Plant Science 21 (7): 551–553. doi:10.1111/ppl.12542.
- Fenollosa, E., S. Munné-Bosch, and M. Pintó-Marijuan. 2017. “Contrasting Phenotypic Plasticity in the Photoprotective Strategies of the Invasive Species Carpobrotus edulis and the Coexisting Native Species Crithmum maritimum.” Physiologia Plantarum 160 (2): 185–200. doi:10.1111/ppl.12542.
- Fernández Prieto, J. A., and J. Loidi. 1984. “Estudio de las Comunidades Vegetales de los Acantilados Costeros de la Cornisa Cantábrica. [Studies on the Plant Communities in Maritime Cantabrian Cliffs].” Documents Phytosociologiques 8: 185–218.
- Ferragut, F., F. Garcia-Marí, J. Costa-Comelles, and R. Laborda. 1987. “Influence of Food and Temperature on Development and Oviposition of Euseius stipulatus and Typhlodromus phialatus (Acari: Phytoseiidae).” Experimental and Applied Acarology 3 (4): 317–329.
- Fielding, J., and N. Turland. 2005. Flowers of Crete. edited by B. Mathew. Kew: Royal Botanic Garden.
- Fitter, A. H., and R. K. M. Hay. 2002. Environmental Physiology of Plants. San Diego: Academic press.
- Florence, J. 2004. Flore de la Polynésie Française. [Flora of French Polynesia] . Vol. II. Collection Faune Et Flore Tropicales 41, 42. Paris: IRD éditions et Muséum national d’histoire naturelle. 518 pp.
- Foxcroft, L. C., P. Pyšek, D. M. Richardson, and P. Genovesi, eds. 2013. Plant Invasions in Protected Areas: Patterns, Problems and Challenges. Invading Nature – Springer Series in Invasion Ecology. 7 vols. Dordrecht: Springer. doi:10.1007/978-94-007-7750-7
- Fraga I Arguimbau, P. 2007. “Conservación de flora amenazada y plantas invasoras en la isla de Menorca. [Conservation of the Endangered Flora and Invasive Plants in Menorca Island].” Conservación Vegetal 11: 30–32.
- Fraga, P., I. Estaún, J. Olives, G. Da Cunha, A. Alarcón, R. Cots, J. Juaneda, and X. Riudavets. 2005. “Eradication of Carpobrotus (L.) N.E.Br. In Minorca.” In Invasive plants in Mediterranean Type Regions of the World. Proceedings of the International Workshop, edited by S. Brunel, 203–208. Strasbourg: Council of Europe Publishing.
- Fried, G., B. Laitung, C. Pierre, N. Chagué, and F. D. Panetta. 2014. “Impact of Invasive Plants in Mediterranean Habitats: Disentangling the Effects of Characteristics of Invaders and Recipient Communities.” Biological Invasions 16 (8): 1639–1658. doi:10.1007/s10530-013-0597-6.
- Galán, P. 2008. “Efecto de la planta invasora Carpobrotus edulis sobre la densidad del eslizón tridáctilo (Chalcides striatus) en una localidad costera de Galicia. [Effects of the invasive plant Carpobrotus edulis over the density of Chalcides striatus in a coastal site of Galicia].” Boletín de la Asociación Herpetológica Española 19: 117–121.
- Gallagher, K. G., K. A. Schierenbeck, and C. M. D’Antonio. 1997. “Hybridization and Introgression in Carpobrotus spp. (Aizoaceae) in California. II. Allozyme Evidence.” American Journal of Botany 84: 905–911.
- Gallo, A. G., W. W. de la Torre, and V. M. Rodríguez. 2008. “Especies vegetales consideradas invasoras de hábitats, en la Historia Natural de Canarias. [Alien Plants Considered Invasive in the Natural History of the Canary Islands].” Lazaroa 29: 49–67.
- García-Llorente, M., B. Martín-López, J. A. González, P. Alcorlo, and C. Montes. 2008. “Social Perceptions of the Impacts and Benefits of Invasive Alien Species: Implications for Management.” Biological Conservation 141 (12): 2969–2983.
- Garzón, O., J. M. Castillo, and M. E. Figueroa. 2005. “Competition between the Invasive Species Carpobrotus edulis and the Endemic Species Limonium emarginatum in Gibraltar Straight.” In Invasive plants in Mediterranean Type Regions of the World. Proceedings of the International Workshop, edited by S. Brunel, 239. Strasbourg: Council of Europe publishing.
- Gawronska, K., E. Romanowska, Z. Miszalski, and E. Niewiadomska. 2013. “Limitation of C3–CAM Shift in the Common Ice Plant under High Irradiance.” Journal of Plant Physiology 170 (2): 129–135. doi:10.1016/j.jplph.2012.09.019.
- GBIF (Global Biodiversity Information Facility) 2017. Accessed May 1 2017. http://www.gbif.org.
- GBIF.org. 2017a. “Carpobrotus acinaciformis (L.) L.Bolus.” Occurrence Download 539 records. Accessed May 1 2017. doi: 10.15468/dl.hzsn8l.
- GBIF.org. 2017b. “Carpobrotus edulis (L.) N.E.Br.” Occurrence Download 3987 records. Accessed May 1 2017. doi: 10.15468/dl.lkoncy.
- Giardina, G., F. M. Raimondo, and V. Spadaro. 2007. “A Catalogue of Plants Growing in Sicily.” Bocconea 20: 5–582.
- Gillam, C. 2017. Whitewash: The Story of a Weed Killer, Cancer, and the Corruption of Science. Washington, DC: Island Press.
- Gioria, M., P. Pyšek, and L. Moravcová. 2012. “Soil Seed Banks in Plant Invasions: Promoting Species Invasiveness and Long-Term Impact on Plant Community Dynamics.” Preslia 84 (2): 327–350.
- GISD (Global Invasive Species Database) 2017. “Carpobrotus edulis.” Accessed July 15 2017. http://www.iucngisd.org/gisd/.
- Goldblatt, P. 1997. “Floristic Diversity in the Cape Flora of South Africa.” Biodiversity and Conservation 6 (3): 359–377.
- Goldblatt, P., and J. C. Manning. 2002. “Plant Diversity of the Cape Region of Southern Africa.” Annals of the Missouri Botanical Garden 89 (2): 281–302.
- Gonçalves, M. L. 1990. “Carpobrotus N. E. Br.” In Flora Iberica, edited by S. Castroviejo, M. Laínz, G. López González, P. Montserrat, F. Muñoz Garmendia, J. Paiva, and L. Villar, 82–85. II vols. Madrid: C.S.I.C.
- Greuter, W., and G. Domina. 2015. “Checklist of the Vascular Plants Collected during the 12th Iter Mediterraneum in Tunisia, 24 March – 4 April 2014.” Bocconea 27 (1): 21–61.
- Guerreiro, A. R. 1977. “Evaluation Trials for Herbicides to Control Hottentot-Fig (Carpobrotus edulis (L.) N.E.Br.).” In Proceedings II Simposio Nacional de Herbologia, 125–134. Oeiras: CABI Publishing.
- Gunn, M., and L. E. W. Codd. 1981. Botanical Exploration of Southern Africa: An Illustrated History of Early Botanical Literature on the Cape Flora: Biographical Accounts of the Leading Plant Collectors and Their Activities in Southern Africa from the Days of the East India Company until Modern Times. Cape Town: Published for the Botanical Research Institute by A.A. Balkema.
- Hartmann, H. E. K. 1993. “Aizoaceae.” In The Families and Genera of Vascular Plants. Flowering Plants - Dicotyledons. Magnoliid, Hamamelid and Caryophyllid Families, edited by K. Kubitzki, J. G. Rohwer, and V. Bittrich, 37–69. Berlin: Springer-Verlag.
- Hartmann, H. E. K. 2001. Illustrated Handbook of Succulent Plants: Aizoaceae. Berlin: Springer-Verlag.
- Hartmann, H. E. K., ed. 2012. Illustrated Handbook of Succulent Plants: Aizoaceae A-E. Berlin: Springer Science & Business Media.
- Howell, C. 2008. Consolidated List of Environmental Weeds in New Zealand. Technical Report. Wellington, New Zealand: Science & Technical Publishing, Department of Conservation. Accessed May 9 2018 http://www.doc.govt.nz/documents/science-and-technical/drds292.pdf.
- Hueso Alcaide, M. C. 2017. “Evolución del glifosato en suelo y plantas de la devesa de L’Albufera de Valencia tras su aplicación para el control de Carpobrotus edulis. [Evolution of glyphosate in soil and plants of the devesa of L’Albufera (Valencia) after its application for the control of Carpobrotus edulis].” Parc Natural de L’Albufera. Accessed July 18 2017. http://www.albufera.com/parque/sites/default/files/descargas/carpobrotus.pdf.
- Hutchings, M. J., and D. K. Wijesinghe. 1997. “Patchy Habitats, Division of Labour and Growth Dividends in Clonal Plants.” Trends in Ecology and Evolution 12 (10): 390–394.
- Invasive Alien Species in Belgium Website. 2013. Carpobrotus spp. Accessed July 15 2017. http://ias.biodiversity.be/.
- Isbell, R. and the National Committee on Soil and Terrain. 2016. The Australian Soil Classification. Clayton, South VIC: CSIRO publishing.
- Jakobsson, A., B. Padrón, and A. Traveset. 2008. “Pollen Transfer from Invasive Carpobrotus spp. To Natives - A Study of Pollinator Behaviour and Reproduction Success.” Biological Conservation 141 (1): 136–145. doi:10.1016/j.biocon.2007.09.005.
- Jardim, R., M. Sequeira, J. Capelo, C. Aguiar, J. C. Costa, D. Espírito-Santo, and M. Lousã. 2003. “XXXVI: The Vegetation of Madeira: IV-Coastal Vegetation of Porto Santo Island (Archipelag of Madeira).” Silva Lusitana 11 (1): 116–120.
- Jørgensen, P. M., M. H. Nee., and S. G. Beck. eds. 2014. “Catálogo de las Plantas Vasculares de Bolivia. [List of the Vascular Plants of Bolivia].” Monographs in Systematic Botany from the Missouri Botanical Garden. 127 (1–2): I–Viii, 1–1744. St. Louis: Missouri Botanical Garden. In Missouri Botanical Garden Website 2008. Tropicos database. Accessed May 7 2018 http://www.tropicos.org/.
- Jucker, T., M. Carboni, and A. T. R. Acosta. 2013. “Going beyond Taxonomic Diversity: Deconstructing Biodiversity Patterns Reveals the True Cost of Iceplant Invasion.” Diversity and Distributions 19 (12): 1566–1577. doi:10.1111/ddi.12124.
- Kelly, J., and C. M. Maguire. 2009. “Hottentot Fig (Carpobrotus edulis) Invasive Species Action Plan.” Invasive Species Ireland. A report prepared for the Northern Ireland Environment Agency and the National Parks and Wildlife Service: Invasive Species Ireland.
- Kelly, J., D. Tosh, K. Dale, and A. Jackson. 2013. “The Economic Cost of Invasive and Non-Native Species in Ireland and Northern Ireland.” A report prepared for the Northern Ireland Environment Agency and the National Parks and Wildlife Service: Invasive Species Ireland.
- Klak, C., A. Khunou, G. Reeves, and T. Hedderson. 2003. “A Phylogenetic Hypothesis for the Aizoaceae (Caryophyllales) Based on Four Plastid DNA Regions.” American Journal of Botany 90 (10): 1433–1445. doi:10.3732/ajb.90.10.1433.
- Knapp, D. A. 2014. “Effects of an Exotic Plant Invasion on Arthropod Assemblages.” PhD diss. Santa Barbara: University of California.
- Konlechner, T. M., D. A. Orlovich, and M. J. Hilton. 2016. “Restrictions in the Sprouting Ability of an Invasive Coastal Plant, Ammophila arenaria, from Fragmented Rhizomes.” Plant Ecology 217 (5): 521–532. doi:10.1007/s11258-016-0597-6.
- Kottek, M., J. Grieser, C. Beck, B. Rudolf, and F. Rubel. 2006. “World Map of the Köppen-Geiger Climate Classification Updated.” Meteorologische Zeitschrift 15 (3): 259–263. doi:10.1127/0941-2948/2006/0130.
- Krebs, E., L. Affre, A. Aboucaya, A. Allègre, J. Chenot, C. Montegu, A. Passetti, D. Pavon, and E. Buisson. 2015. “Résilience de la Flore Indigène Après Éradication des Griffes de Sorcière (Carpobrotus sp.) Sur Une Île Méditerrranéenne. [Resilience of Native Plant Communities after Eradication of Ice Plant (Carpobrotus sp.) On a Mediterranean Island].” Revue d’Ecologie 70: 80–90.
- Kuzniak, E., A. Kornas, B. Gabara, C. Ullrich, M. Sklodowska, and Z. Miszalski. 2010. “Interaction of Botrytis cinerea with the Intermediate C3-CAM Plant Mesembryanthemum crystallinum.” Environmental and Experimental Botany 69 (2): 137–147. doi:10.1016/j.envexpbot.2010.03.010.
- Larcher, W. 1995. Physiological Plant Ecology. Berlin: Springer-Verlag.
- Le Sueur, F. 1984. Flora of Jersey. Jersey: Societe Jersiaise.
- Lechuga-Lago, Y., M. Sixto-Ruiz, S. R. Roiloa, and L. González. 2016. “Clonal Integration Facilitates the Colonization of Drought Environments by Plant Invaders.” AoB Plants 8: plw023. doi:10.1093/aobpla/plw023.
- Lin, H. F., P. Alpert, and F. H. Yu. 2012. “Effects of Fragment Size and Water Depth on Performance of Stem Fragments of the Invasive, Amphibious, Clonal Plant Ipomoea aquatica.” Aquatic Botany 99: 34–40. doi:10.1016/j.aquabot.2012.01.004.
- Liu, W. Y., H. J. Ridgway, T. K. James, E. K. James, W. M. Chen, J. I. Sprent, J. P. W. Young, and M. Andrews. 2014. “Burkholderia sp. Induces Functional Nodules on the South African Invasive Legume Dipogon lignosus (Phaseoleae) in New Zealand Soils.” Microbial Ecology 68 (3): 542–555. doi:10.1007/s00248-014-0427-0.
- López González, G. 1995. “Carpobrotus aequilaterus (Haw.) NE Br. [syn.: C. chilensis (Molina) NE Br.] (Aizoaceae), una especie que se debe excluir de la flora de la Península Ibérica [C. aequilaterus, a species to be excluded from the Iberian Peninsula flora].” Anales Del Jardín Botánico De Madrid 53 (2): 251–252.
- Lousley, J. E. 1971. Flora of the Isles of Scilly. Newton Abbot: David and Charles.
- MacDonald, J. D., J. R. Hartman, and J. D. Shapiro. 1984. “Pathogens of Ice Plant in California.” Plant Disease 68 (11): 965–967.
- MacDonald, J. D., J. R. Hartman, J. D. Shapiro, and K. Reinke. 1983. Diseases of Ice Plant along California Roadsides. Report FHWA/CA/UCD-83–1, 35. Sacramento, CA: California State Department of Transportation.
- Madawala, S., S. Hartley, and K. S. Gould. 2014. “Comparative Growth and Photosynthetic Responses of Native and Adventive Iceplant Taxa to Salinity Stress.” New Zealand Journal of Botany 52 (3): 352–364. doi:10.1080/0028825X.2014.925478.
- Majer, J. D. 1982. “Ant-Plant Interactions in the Darling Botanical District of Western Australia.” In Ant-Plant Interactions in Australia, edited by R. C. Buckley, 45–61. Dordrecht: Springer.
- Malan, C., and A. Notten. 2006. Kirstenbosch National Botanical Garden. Carpobrotus edulis (L.) L.Bolus. Accessed July 15 2017. http://www.plantzafrica.com/plantcd/carpobed.htm.
- Malavasi, M., R. Santoro, M. Cutini, A. T. R. Acosta, and M. L. Carranza. 2016. “The Impact of Human Pressure on Landscape Patterns and Plant Species Richness in Mediterranean Coastal Dunes.” Plant Biosystems - an International Journal Dealing with All Aspects of Plant Biology 150 (1): 73–82. doi:10.1080/11263504.2014.913730.
- Malta Environment and Planning Authority (MEPA). 2013. “Major Plant Invaders and Possible Management Options.” In Guidelines on Managing Non-Native Plant Invaders and Restoring Native Plant Communities in Terrestrial Settings in the Maltese Islands. State of the Environment Reports. Malta: Biodiversity Publications-ERA. 88 pp.
- Maltez-Mouro, S., F. T. Maestre, and H. Freitas. 2010. “Weak Effects of the Exotic Invasive Carpobrotus edulis on the Structure and Composition of Portuguese Sand-Dune Communities.” Biological Invasions 12 (7): 2117–2130. doi:10.1007/s10530-009-9613-2.
- Manning, J., and C. Paterson-Jones. 2007. Field Guide to Fynbos. Cape Town: Struik Publishers.
- Marchante, E., and H. Marchante. 2016. “Engaging Society to Fight Invasive Alien Plants in Portugal - One of the Main Threats to Biodiversity.” In Biodiversity and Education for Sustainable Development, edited by P. Castro, U. Azeiteiro, P. Bacelar-Nicolau, W. Leal Filho, and A. Azul, 107–122. Cham: Springer. World Sustainability Series. doi:10.1007/978-3-319-32318-3_8.
- Marchante, H., M. Morais, H. Freitas, and E. Marchante. 2014. Guia Prático Para a Identificação de Plantas Invasoras Em Portugal. [A Practical Guide for the Identification of Invasive Alien Plants in Portugal]. Coimbra: Coimbra University Press. 207 pp.
- Marloth, R. 1913. The Flora of South Africa. I vols. Capetown: Darter Bros.
- Maron, J. L., and M. Vilà. 2001. “When Do Herbivores Affect Plant Invasion? Evidence for the Natural Enemies and Biotic Resistance Hypotheses.” Oikos 95 (3): 361–373. doi:10.1034/j.1600-0706.2001.950301.x.
- Masciadri, S., E. Brugnoli, and P. Muniz. 2010. “InBUy Database of Invasive and Alien Species (IAS) in Uruguay: A Useful Tool to Confront This Threat to Biodiversity.” Biota Neotropica 10 (4): 205–213.
- Matsiliza, B., and N. P. Barker. 2001. “A Preliminary Survey of Plants Used in Traditional Medicine in the Grahamstown Area.” South African Journal of Botany 67 (2): 177–182.
- Maurel, N., S. Salmon, J. F. Ponge, N. Machon, J. Moret, and A. Muratet. 2010. “Does the Invasive Species Reynoutria japonica Have an Impact on Soil and Flora in Urban Wastelands?” Biological Invasions 12 (6): 1709–1719. doi:10.1007/s10530-009-9583-4.
- Mazzeo, G., P. Suma, and A. Russo. 2008. “Scale Insects on Succulent Plants in Southern Italy.” In Procedings of the 11th International Symposium on Scale Insect Studies, edited by M. Branco, J. C. Franco, and C. Hodgson, 149–152. Oeiras, Portugal. September 24–27. Lisbon: ISA Press.
- Mazzeo, G., S. Longo, G. Pellizzari, F. Porcelli, P. Suma, and A. Russo. 2014. “Exotic Scale Insects (Coccoidea) on Ornamental Plants in Italy: A Never-Ending Story.” Acta Zoologica Bulgarica S6: 55–61.
- McCain, A. H., R. D. Raabe, and S. Wilhelm. 1981. “Plants Resistant or Susceptible to Verticillium Wilt.” In Leaflet 2703, edited by Division of Agricultural Sciences, Berkeley: University of California. Cooperative Extn. (USA).
- McClintock, D. 1975. The Wild Flowers of Guernsey: With Notes of the Frequencies of All Species Recorded for the Channel Islands. London: Collins.
- Meyer, J.-Y. 2000. “Preliminary Review of the Invasive Plants in the Pacific Islands (SPREP Member Countries).” In Invasive Species in the Pacific: A Technical Review and Draft Regional Strategy, edited by G. Sherley, tech. ed. 190, 104. Apia, Samoa: South Pacific Regional Environment Programme (SPREP).
- Ministerio de Medio Ambiente, y Medio Rural y Marino. 2011. Plan de control y eliminación de especies vegetales invasoras de sistemas dunares. [Control and Eradication Plan of Alien Invasive Plant Species in Dune Systems]. Madrid: Ministerio de Agricultura y Pesca, Alimentación y Medio Ambiente. Accessed 18 July 2017. http://www.mapama.gob.es/es/costas/temas/proteccion-costa/TODO_tcm7-338467.pdf
- Molinari, N., C. D’Antonio, and G. Thomson. 2007. “Carpobrotus as a Case Study of the Complexities of Species Impacts.” Chapter 7. In Ecosystem Engineers: Plants to Protists, Theoretical Ecology Series, edited by K. Cuddington, J. E. Byers, W. G. Wilson, and A. Hastings, 139–162. IV vols. London: Academic Press.
- Moragues, E., and A. Traveset. 2005. “Effect of Carpobrotus spp. On the Pollination Success of Native Plant Species of the Balearic Islands.” Biological Conservation 122 (4): 611–619. doi:10.1016/j.biocon.2004.09.015.
- Moragues, E., A. Traveset, and F. Valladares. 2005. “Good Performance in Both Sun and Shade Habitats Leads to the Spreading of Invasive Carpobrotus spp. In Mediterranean Ecosystems.” In Invasive Plants in Mediterranean Type Regions of the World. Proceedings of the International Workshop, edited by S. Brunel, 251. Strasbourg: Council of Europe publishing.
- Moragues, E., and J. Rita. 2005. Els vegetals introduïts a les Illes Balears. [Introduced Plants in the Balearic Islands]. Technical Report. Palma de Mallorca, Spain: Conselleria de Medi Ambient, Govern de les Illes Balears.
- Morales, C. L., and A. Traveset. 2009. “A Meta-Analysis of Impacts of Alien vs. Native Plants on Pollinator Visitation and Reproductive Success of Co-Flowering Native Plants.” Ecology Letters 12 (7): 716–728. doi:10.1111/j.1461-0248.2009.01319.x.
- Morzaria-Luna, H. N., and J. B. Zedler. 2007. “Does Seed Availability Limit Plant Establishment during Salt Marsh Restoration?” Estuaries and Coasts 30 (1): 12–25.
- Moss, D. 2008. EUNIS Habitat Classification–A Guide for Users, 1–27. European Topic Centre on Biological Diversity.
- Mostert, E., M. Gaertner, P. M. Holmes, A. G. Rebelo, and D. M. Richardson. 2017. “Impacts of Invasive Alien Trees on Threatened Lowland Vegetation Types in the Cape Floristic Region, South Africa.” South African Journal of Botany 108: 209–222. doi:10.1016/j.sajb.2016.10.014.
- Motti, R., V. Antignani, and M. Idolo. 2009. “Traditional Plant Use in the Phlegraean Fields Regional Park (Campania, Southern Italy).” Human Ecology 37 (6): 775–782.
- Mucina, L., H. Bültmann, K. Dierßen, J. P. Theurillat, T. Raus, A. Čarni, K. Šumberová et al. 2016. “Vegetation of Europe: Hierarchical Floristic Classification System of Vascular Plant, Bryophyte, Lichen, and Algal Communities.” Applied Vegetation Science 19 (1): 3–264.
- Mucina, L., J. B. Adams, I. C. Knevel, M. C. Rutherford, L. W. Powrie, J. J. Bolton, J. H. van der Merwe, et al. 2006. “Coastal Vegetation of South Africa.” Strelitzia 19:658 –583.
- Museum National d’Histoire Naturelle. 2017. The Vascular Plants Collection (P) at the Herbarium of the Muséum National d’Histoire Naturelle. Paris: MNHN.
- Neto, C., J. Capela, and J. C. Costa. 2004. “De Vegetatio Lusitana Notae-III.: 8. “Comunidades vegetais dos solos arenosos podzolizados do Sado e Costa da Galé. Uma interpretação fitossocio-lógica dos dados paleoecológicos e geomorfológicos” “[Plant communities in sandy soils from Sado and Galé coasts. A phytosociological interpretation of the paleoecologic and geomorphologic data].” Silva Lusitana 12 (2): 256–262.
- Neto, C., P. Arsénio, and J. C. Costa. 2009. “Flora e Vegetação do sudoeste de Portugal continental. [Flora and Vegetation from South-East Continental Portugal].” Quercetea 9: 43–144.
- Neto, C. S. 2002. “A flora e a vegetação do Superdistrito Sadense (Portugal). [Flora and vegetation of the Sadense super-district (Portugal)].” Guineana 8: 1–269.
- Nikolić, T., ed. 2017. Flora Croatica Database (FCD). Accessed July 30 2017. http://hirc.botanic.hr/fcd.
- Novoa, A., and L. González. 2014. “Impacts of Carpobrotus edulis (L.) NE Br. On the Germination, Establishment and Survival of Native Plants: A Clue for Assessing Its Competitive Strength.” PLoS ONE 9 (9): e107557. doi:10.1371/journal.pone.0107557.
- Novoa, A., L. González, L. Moravcová, and P. Pyšek. 2012. “Effects of Soil Characteristics, Allelopathy and Frugivory on Establishment of the Invasive Plant Carpobrotus edulis and a Co-Occuring Native, Malcolmia littorea.” PLoS ONE 7 (12): e53166. doi:10.1371/journal.pone.0053166.
- Novoa, A., L. González, L. Moravcová, and P. Pyšek. 2013. “Constraints to Native Plant Species Establishment in Coastal Dune Communities Invaded by Carpobrotus edulis: Implications for Restoration.” Biological Conservation 164: 1–9. doi:10.1016/j.biocon.2013.04.008.
- Novoa, A., R. Rodríguez, D. M. Richardson, and L. González. 2014. “Soil Quality: A Key Factor in Understanding Plant Invasion? The Case of Carpobrotus edulis (L.) N.E.Br.” Biological Invasions 16 (2): 429–443.
- Omoruyi, B. E., A. J. Afolayan, and G. Bradley. 2014. “Chemical Composition Profiling and Antifungal Activity of the Essential Oil and Plant Extracts of Mesembryanthemum edule (L.) Bolus Leaves.” African Journal of Traditional Complementary and Alternative Medicines 11 (4): 19–30. doi:10.4314/ajtcam.v11i4.4.
- Omoruyi, B. E., G. Bradley, and A. J. Afolayan. 2012. “Antioxidant and Phytochemical Properties of Carpobrotus edulis (L.) Bolus Leaf Used for the Management of Common Infections in HIV/AIDS Patients in Eastern Cape Province.” BMC Complementary and Alternative Medicine 12. doi:10.1186/1472-6882-12-215.
- Ordway, D., J. Hohmann, M. Viveiros, A. Viveiros, J. Molnar, C. Leandro, M. J. Arroz, M. A. Gracio, and L. Amaral. 2003. “Carpobrotus edulis Methanol Extract Inhibits the MDR Efflux Pumps, Enhances Killing of Phagocytosed S-Aureus and Promotes Immune Modulation.” Phytotherapy Research 17 (5): 512–519. doi:10.1002/ptr.1314.
- Ortiz, D. G., E. L. Lumbreras, and J. A. Rosselló. 2008. “Flora alóctona suculenta valenciana: Aizoaceae y Portulacaceae [Alochtonous succulent Valencian flora: Aizoaceae and Portulacaceae].” Monografías De Bouteloa 7: 1–68.
- Palmer, W. A., T. A. Heard, and A. W. Sheppard. 2010. “A Review of Australian Classical Biological Control of Weeds Programs and Research Activities over the past 12 Years.” Biological Control 52 (3): 271–287.
- Parnell, J., and T. Curtis. 2012. Webb´S an Irish Flora. Ireland: Corks University Press.
- Parsons, R. F. 1997. “Carpobrotus modestus (Aizoaceae), a Post-Fire Pioneer in Semi-Arid Southern Australia.” Journal of Arid Environments 37 (3): 453–459. doi:10.1006/jare.1997.0288.
- Pastor, J. 1987. “Aizoaceae.” In Flora de Andalucía Occidental [Flora of Western Andalusia], edited by B. Valdés, S. Talavera, and F. Galiano, 167–168. I vols. Barcelona: Ketres.
- Pirie, A., D. Parsons, J. Rengglia, C. Narkowicza, G. A. Jacobson, and S. Shabalab. 2013. “Modulation of Flavonoid and Tannin Production of Carpobrotus rossii By. Environmental Conditions.” Environmental and Experimental Botany 87: 19–31. doi:10.1016/j.envexpbot.2012.10.001.
- Pitzalis, M., L. Luiselli, and M. A. Bologna. 2010. “Co-Occurrence Analyses Show that Non-Random Community Structure Is Disrupted by Fire in Two Groups of Soil Arthropods (Isopoda Oniscidea and Collembola).” Acta Oecologica 36 (1): 100–106. doi:10.1016/j.actao.2009.10.009.
- Portela, R., and S. R. Roiloa. 2017. “Effects of Clonal Integration in the Expansion of Two Alien Carpobrotus Species into a Coastal Dune System – A Field Experiment.” Folia Geobotanica 1–9. doi:10.1007/s12224-016-9278-4.
- Preston, C. D., and P. D. Sell. 1988. “The Aizoaceae Naturalized in the British Isles.” Watsonia 17 (3): 217–245.
- Prinsloo, G. L., and V. M. Uys, eds. 2015. Insects of Cultivated Plants and Natural Pastures in Southern Africa. Pretoria, South Africa: Entomological Society of Southern Africa.
- Pyšek, P. 1997. “Clonality and Plant Invasions: Can a Trait Make a Difference?” In The Ecology and Evolution of Clonal Plants, edited by H. De Kroon and J. Van Groenendael, xii + 453. 405–427. Leiden: Netherlands, Backhuys Publishers.
- Ragusa, S., and E. Swirski. 1975. “Feeding Habits, Development and Oviposition of the Predacious Mite Amblyseius swirskii Athias-Henriot (Acarina: Phytoseiidae) on Pollen of Various Weeds.” Israel Journal of Entomology 10: 93–103.
- Ragusa, S., M. A. Zedan, and M. A. Sciacchitano. 1986. “The Effects of Food from Plant and Animal Sources on the Development and Egg Production of the Predaceous Mite Hypoaspis aculeifer (Canestrini) (Parasitiformes, Dermanyssidae).” Redia 69: 481–488.
- Raimondo, D., L. Von Staden, W. Foden, J. E. Victor, N. A. Helme, R. C. Turner, D. A. Kamundi, and P. A. Manyama. 2009. Red List of South African Plants: Strelitzia 25. Pretoria: South African National Biodiversity Institute.
- Razzaghmanesh, M., S. Beecham, and F. Kazemi. 2014. “The Growth and Survival of Plants in Urban Green Roofs in a Dry Climate.” Science of the Total Environment 476: 288–297. doi:10.1016/j.scitotenv.2014.01.014.
- Reuveny, H., E. Palevsky, and U. Gerson. 1996. “Laboratory Life History Studies of the Predaceous Mite Typhlodromus athiasae (Acari: Phytoseiidae).” Systematic and Applied Acarology 1 (1): 45–53.
- Reynolds, S. C. 2002. A Catalogue of Alien Plants in Ireland. Glasnevin, Dublin, Ireland: National Botanic Gardens.
- Richardson, D. M., P. Pyšek, M. Rejmanek, M. G. Barbour, F. D. Panetta, and C. J. West. 2000. “Naturalization and Invasion of Alien Plants: Concepts and Definitions.” Diversity and Distributions 6 (2): 93–107.
- Ríos, M., L. Bartesaghi, V. Piñeiro, A. Garay, P. Mai, L. Delfino, S. Masciadri, E. Alonso-Paz, M. J. Bassagoda and A. Soutullo. 2010. “Caracterización y distribución espacial del bosque y matorral psamófilo. [Characterization and spatial distribution of the forest and shrubland in sandy soils].” In Series de Informes delSistema Nacional De Áreas Protegidas-Gestión Integrada de la Zona Costera, edited by EcoPlata and SNAP, 23. Montevideo, Uruguay: Ministerio de Vivienda Ordenamiento Territorial y Medio Ambiente (MVOTMA).
- Rioux, J.-A., J. Roux, and S. Pignatti. 1955. “Les Associations Littorales des Albères (Étude Critique) [Coastal Plant Communities from Albères].” Vie Et Milieu 6 (1): 1–37.
- Rodrigues, A. S. B., S. E. Silva, E. Marabuto, D. N. Silva, M. R. Wilson, V. Thompson, S. Yurtsever, et al. 2014b. “New Mitochondrial and Nuclear Evidences Support Recent Demographic Expansion and an Atypical Phylogeographic Pattern in the Spittlebug Philaenus spumarius (Hemiptera, Aphrophoridae).” PLoS ONE 9 (6). doi:10.1371/journal.pone.0098375.
- Rodrigues, M. J., K. N. Gangadhar, C. Vizetto-Duarte, S. G. Wubshet, N. T. Nyberg, L. Barreira, J. Varela, and L. Custódio. 2014a. “Maritime Halophyte Species from Southern Portugal as Sources of Bioactive Molecules.” Marine Drugs 12 (4): 2228–2244.
- Rodríguez, J., A. Novoa, V. Thompson, A. Cordero-Rivera, D. M. Richardson, and L. González. 2017. “Novel Interactions between Carpobrotus edulis and Invertebrate Species in the Native and Non-Native Range.” In EMAPI International Conference on Ecology and Management of Alien Plant Invasions.- Syntheses, Challenges and New Opportunities, edited by C. Máguas, C. Crous, and C. Costa, 195, Lisbon, Portugal, September 4–8.
- Rodríguez, J., M. Calbi, S. R. Roiloa, and L. González. 2018. “Herbivory Induced Non-Local Responses of the Clonal Invader Carpobrotus edulis are Not Mediated by Clonal Integration.” Science of the Total Environment 633: 1041–1050. doi:10.1016/j.scitotenv.2018.03.264.
- Rodríguez, J., V. Thompson, M. Rubido-Bará, and L. González. 2015. “Is the Meadow Spittlebug philaenus Spumarius (Hemiptera: Aphrophoridae) Changing Their Ecological Behavior in the Presence of the Invasive Plant Carpobrotus edulis?” In 4º Congreso Ibérico de Ecología, edited by Asociación Española de Ecología Terrestre (AEET), 300. Coimbra, Portugal. June 16–19.
- Roiloa, S. R., J. G. Campoy, and R. Retuerto. 2015. “Importancia de la Integración Clonal En Las Invasiones Biológicas. [The Importance of Clonal Integration in Biological Invasions].” Ecosistemas 24 (1): 76–83. doi:10.7818/ECOS.2015.24-1.12.
- Roiloa, S. R., and R. Retuerto. 2016. “Effects of Fragmentation and Seawater Submergence on Photochemical Efficiency and Growth in the Clonal Invader Carpobrotus edulis.” Flora - Morphology, Distribution, Functional Ecology of Plants 225: 45–51. doi:10.1016/j.flora.2016.10.002.
- Roiloa, S. R., R. Retuerto, J. G. Campoy, A. Novoa, and R. Barreiro. 2016. “Division of Labour Brings Greater Benefits to Clones of Carpobrotus edulis in the Non-Native Range: Evidence for Rapid Adaptive Evolution.” Frontiers in Plant Science (Section Functional Plant Ecology) 7: 349. doi:10.3389/fpls.2016.00349.
- Roiloa, S. R., S. Abalde, C. Y. Xu, and L. López. 2017. “The Effect of Stolon Fragmentation on the Colonization of Clonal Invasive Carpobrotus edulis in a Coastal Dune System: A Field Test.” Plant Species Biology 32: 460–465. doi:10.1111/1442-1984.12157.
- Roiloa, S. R., S. Rodríguez-Echeverría, A. López-Otero, R. Retuerto, and H. Freitas. 2014. “Adaptive Plasticity to Heterogeneous Environments Increases Capacity for Division of Labor in the Clonal Invader Carpobrotus edulis (Aizoaceae).” American Journal of Botany 101 (8): 1301–1308. doi:10.3732/ajb.1400173.
- Roiloa, S. R., S. Rodríguez-Echeverría, E. de la Peña, and H. Freitas. 2010. “Physiological Integration Increases the Survival and Growth of the Clonal Invader Carpobrotus edulis.” Biological Invasions 12 (6): 1815–1823. doi:10.1007/s10530-009-9592-3.
- Roiloa, S. R., S. Rodriguez-Echeverría, and H. Freitas. 2014. “Effect of Physiological Integration in Self/Non-Self Genotype Recognition on the Clonal Invader Carpobrotus edulis.” Journal of Plant Ecology 7 (4): 413–418. doi:10.1093/jpe/rtt045.
- Roiloa, S. R., S. Rodríguez-Echeverría, H. Freitas, and R. Retuerto. 2013. “Developmentally-Programmed Division of Labour in the Clonal Invader Carpobrotus edulis.” Biological Invasions 15 (9): 1895–1905. doi:10.1007/s10530-013-0417-z.
- Ruffino, L., E. Krebs, A. Passetti, A. Aboucaya, L. Affre, D. Fourcy, O. Lorvelec, et al. 2015. “Eradications as Scientific Experiments: Progress in Simultaneous Eradications of Two Major Invasive Taxa from a Mediterranean Island.” Pest Management Science 71 (2): 189–198. doi:10.1002/ps.3786.
- Rumlerová, Z., M. Vilà, J. Pergl, W. Nentwig, and P. Pyšek. 2016. “Scoring Environmental and Socioeconomic Impacts of Alien Plants Invasive in Europe.” Biological Invasions 18 (12): 3697–3711. doi:10.1007/s10530-016-1259-2.
- Russo, A., G. Mazzeo, and P. Suma. 1999. “Sulla presenza di Entaspidiotus lounsburyi (Marlatt, 1908) su Mesembryanthemaceae in Italia (Homoptera Coccoidea). [On the presence of Entaspidiotus lounsburyi (Marlatt, 1908) on Mesembryanthemaceae in Italy (Homoptera, Coccoidea)].” Redia 82: 83–87.
- Santoro, R., M. Carboni, M. L. Carranza, and A. T. R. Acosta. 2012a. “Focal Species Diversity Patterns Can Provide Diagnostic Information on Plant Invasions.” Journal for Nature Conservation 20 (2): 85–91. doi:10.1016/j.jnc.2011.08.003.
- Santoro, R., T. Jucker, M. Carboni, and A. T. R. Acosta. 2012b. “Patterns of Plant Community Assembly in Invaded and Non-Invaded Communities along a Natural Environmental Gradient.” Journal of Vegetation Science 23 (3): 483–494. doi:10.1111/j.1654-1103.2011.01372.x.
- Santoro, R., T. Jucker, M. Carranza, and A. T. R. Acosta. 2011. “Assessing the Effects of Carpobrotus Invasion on Coastal Dune Soils. Does the Nature of the Invaded Habitat Matter?” Community Ecology 12 (2): 234–240. doi:10.1016/j.jnc.2011.08.003.
- Sanz-Elorza, M., E. D. Dana, and E. Sobrino. 2004. Atlas de las Plantas Alóctonas Invasoras en España. [Atlas of the Invasive Alien Plant Species in Spain]. Madrid: Dirección General para la Biodiversidad, Ministerio de Medio Ambiente.
- Sanz-Elorza, M., E. D. Dana, and E. Sobrino. 2005. “Aproximación al listado de plantas vasculares alóctonas invasoras reales y potenciales en las islas Canarias. [An Approach to a List of Actual and Potential Invasive Species in the Canary Islands].” Lazaroa 26: 55–66.
- Sayed, O. H. 2001. “Crassulacean Acid Metabolism 1975–2000, a Check List.” Photosynthetica 39 (3): 339–352.
- Scalera, R., A. Cozzi, C. Caccamo, and I. Rossi. 2017. “A Catalogue of LIFE Projects Contributing to the Management of Alien Species in the European Union.” In Platform Meeting on Invasive Alien Species (IAS), edited by R. Scalera, A. Cozzi, C. Caccamo, and I. Rossi, 140. Milan (Italy), November 29–30. LIFE14 IP/IT/000018 Nature Integrated Management to 2020 (GESTIRE 2020).
- Schaminée, J. H. J., M. Chytrý, S. M. Hennekens, L. Mucina, J. S. Rodwell, and L. Tichý. 2012. Development of Vegetation Syntaxa Crosswalks to EUNIS Habitat Classification and Related Data Sets. Final report EEA/NSV/12/001. Alterra, Wageningen, NL.
- Schierenbeck, K. A., V. V. Symonds, K. G. Gallagher, and J. Bell. 2005. “Genetic Variation and Phylogeographic Analyses of Two Species of Carpobrotus and Their Hybrids in California.” Molecular Ecology 14 (2): 539–547. doi:10.1111/j.1365-294X.2005.02417.x.
- Schmalzer, P. A., and C. R. Hinkle. 1987. “Species Biology and Potential for Controlling Four Exotic Plants (Ammophila arenaria, Carpobrotus edulis, Cortaderia jubata, and Gasoul crystallinum) on Vandenberg Air Force Base, California.” In NASA Technical Memorandum 100980, edited by National Aeronautics and Space Administration (NASA), 103. Florida: Kennedy Space Center.
- Silva, L., and C. W. Smith. 2006. “A Quantitative Approach to the Study of Non-Indigenous Plants: An Example from the Azores Archipelago.” Biodiversity and Conservation 15 (5): 1661–1679.
- Silva, S. E., A. S. Rodrigues, E. Marabuto, S. Yurtsever, P. A. Borges, J. A. Quartau, O. S. Paulo, and S. G. Seabra. 2015. “Differential Survival and Reproduction in Colour Forms of Philaenus spumarius Give New Insights to the Study of Its Balanced Polymorphism.” Ecological Entomology 40 (6): 759–766.
- Sintes, T., E. Moragues, A. Traveset, and J. Rita. 2007. “Clonal Growth Dynamics of the Invasive Carpobrotus affine acinaciformis in Mediterranean Coastal Systems: A Non-Linear Model.” Ecological Modelling 206 (1–2): 110–118. doi:10.1016/j.ecolmodel.2007.03.027.
- Slade, A. J., and M. J. Hutchings. 1987. “An Analysis of the Costs and Benefits of Physiological Integration between Ramets in the Clonal Perennial Herb Glechoma hederacea.” Oecologia 73: 425–431.
- Smyth, N., M. Jebb, and A. Booth. 2011. “Conserving Farmland Biodiversity.” In Invasive Species Control Case Study: Hottentot Fig (Carpobrotus edulis) Control in Ireland, edited by D. Ó hUallacháin and J. Finn, 17–18. Carlow: Teagasc.
- Sotes, G. J., L. A. Cavieres, and R. Rodríguez. 2015. “Carpobrotus edulis (L.) NE Br. (Aizoaceae) and Its Presence in the Chilean Flora.” Gayana Botanica 72 (1): 149–151. doi:10.4067/S0717-66432015000100018.
- Stock, W. D., and G. A. Verboom. 2012. “Phylogenetic Ecology of Foliar N and P Concentrations and N: P Ratios across Mediterranean‐Type Ecosystems.” Global Ecology and Biogeography 21 (12): 1147–1156.
- Strid, A., and K. Tan. 1997. “Carpobrotus.” In Flora Hellenica I, edited by A. Strid and K. Tan. Königsten: Koeltz Scientific Books.
- Suehs, C. M., F. Médail, and L. Affre. 2001. “Ecological and Genetic Features of the Invasion by the Alien Carpobrotus Plants in Mediterranean Island Habitats.” In Plant Invasions: Species Ecology and Ecosystem Management, edited by G. Brundu, J. Brock, L. Camarda, L. Child, and M. Wade, 145–158. Leiden, Netherlands: Backhuys Publishers.
- Suehs, C. M., F. Médail, and L. Affre. 2003. “Invasion by South African Carpobrotus (Aizoaceae) Taxa in the Mediterranean Basin: The Effects of Insularity on Plant Reproductive Systems.” In Plant Invasions: Ecological Threats and Management Solutions, edited by L. Child, 247–263. Leiden, Netherlands: Backhuys Publishers.
- Suehs, C. M., L. Affre, and F. Médail. 1999. “Ecological and Genetic Features of the Invasion by the Alien Carpobrotus (Aizoaceae) Plants in Mediterranean Island Habitats.” In Proceedings of the 5th International Conference on the Ecology of Invasive Alien Plants, edited by I. Camarda and G. Brundu, 107. La Maddalena, Sardinia, Italy: Dipartimento di Botanica ed Ecologia vegetale Università degli Studi di Sassari Italy. October 13 –16.
- Suehs, C. M., L. Affre, and F. Médail. 2004a. “Invasion Dynamics of Two Alien Carpobrotus (Aizoaceae) Taxa on a Mediterranean Island: I. Genetic Diversity and Introgression.” Heredity 92 (1): 31–40. doi:10.1038/sj.hdy.6800374.
- Suehs, C. M., L. Affre, and F. Médail. 2004b. “Invasion Dynamics of Two Alien Carpobrotus (Aizoaceae) Taxa on a Mediterranean Island: II. Reproductive Strategies.” Heredity 92 (6): 550–556. doi:10.1038/sj.hdy.6800454.
- Suehs, C. M., L. Affre, and F. Médail. 2005. “Unexpected Insularity Effects in Invasive Plant Mating Systems: The Case of Carpobrotus (Aizoaceae) Taxa in the Mediterranean Basin.” Biological Journal of the Linnean Society 85 (1): 65–79. doi:10.1111/j.1095-8312.2005.00473.x.
- Suehs, C. M., S. Charpentier, L. Affre, and F. Médail. 2006. “The Evolutionary Potential of Invasive Carpobrotus (Aizoaceae) Taxa: Are Pollen-Mediated Gene Flow Potential and Hybrid Vigor Levels Connected?” Evolutionary Ecology 20 (5): 447–463. doi:10.1007/s10682-006-0013-0.
- Swirski, E., A. Amitai, and N. Dorzia. 1970. “Laboratory Studies on the Feeding Habits, Post-Embryonic Survival and Oviposition of the Predaceous Mites Amblyseius chilenensis Dosse and Amblyseius Hibisci Chant (Acarina: Phytoseiidae) on Various Kinds of Food Substances.” Entomophaga 15 (1): 93–106.
- Swirski, E., and N. Dorzia. 1969. “Laboratory Studies on the Feeding, Development and Fecundity of the Predaceous Mite Typhlodromus occidentalis Nesbitt (Acarina: Phytoseiidae) on Various Kinds of Food Substances.” Israel Journal of Agricultural Research 19 (3): 143–145.
- Tassan, R. L., S. H. Kenneth, and D. V. Cassidy. 1982. “Imported Natural Enemies Established against Ice Plant Scales in California.” California Agriculture 36 (9): 16–17.
- Theiss, K. Associates. 1994. Methods for Removal of Carpobrotus edulis from Coastal Dunes, Humboldt County. Arcata: Nature Conservancy.
- Thuiller, W., D. M. Richardson, P. Pyšek, G. F. Midgley, G. O. Hugues, and M. Rouget. 2005. “Niche-Based Modelling as a Tool for Predicting the Risk of Alien Plant Invasions at a Global Scale.” Global Change Biology 11 (12): 2234–2250. doi:10.1111/j.1365-2486.2005.01018.x.
- Thurston, E., and C. C. Vigurs. 1922. A Supplement to F. Hamilton Davey’s Flora of Cornwall, Journal of the Royal Institution of Cornwall. Truro: Oscar Blackford, Royal Printeries.
- Torre Fernández, F. D. L. 2003. “Las Plantas Invasoras en Asturias. [Invasive Plant Species of Asturias].” Naturalia Cantabricae 2: 33–43.
- Torre Fernández, F. D. L., and R. Alvarez-Arbesú. 1999. “Control of Noxious Weeds in Sensitive Areas.” In 1º Encontro Invasoras Lenhosas [Conference on woody invading plants], edited by SPCF/ADERE Peneda-Gerês, 203–208. Gerês, Portugal: Sociedade portuguesa de Ciências Florestais (SPCF), November 16–18.
- Traveset, A., E. Moragues, and F. Valladares. 2008. “Spreading of the Invasive Carpobrotus aff. acinaciformis in Mediterranean Ecosystems: The Advantage of Performing in Different Light Environments.” Applied Vegetation Science 11 (1): 45–54. doi:10.3170/2007-7-18303.
- Traveset, A., G. Brundu, M. Carta, I. Mprezetou, P. Lambdon, M. Manca, F. Médail, et al. 2008. “Consistent Performance of Invasive Plant Species within and among Islands of the Mediterranean Basin.” Biological Invasions 10 (6): 847–858. doi:10.1007/s10530-008-9245-y.
- Treichel, S., and P. Bauer. 1974. “Different NaCl-dependence of Circadian CO2-gas Exchange of Some Halophil Growing Coastal Plants.” Oecologia 17 (1): 87–95.
- Tu, M., C. Hurd, and J. M. Randall. 2001. Weed Control Methods Handbook: Tools & Techniques for Use in Natural Areas. Utah: U.S. Government Documents. Accessed May 15 2017. http://digitalcommons.usu.edu/govdocs/533
- Tutin, T. G. 1993. “Carpobrotus” In Flora Europaea Vol I. Lycopodiaceae to Platanaceae, 2nd Ed, edited by T. G. Tutin, V. H. Heywood, N. A. Burges, D. H. Valentine, S. M. Walters, and D. A. Webb, 112–113. Cambridge: Cambridge University Press.
- Ungar, I. A. 1978. “Halophyte Seed Germination.” The Botanical Review 44 (2): 233–264.
- USDA National Genetic Resources Program. 2012. Germplasm Resources Information Network - (GRIN). Beltsville: National Germplasm Resources Laboratory.
- USDA. Soil Conservation Service. 1999. Soil Taxonomy: A Basic System of Soil Classification for Making and Interpreting Soil Surveys. USDA National Genetic Resources Program, Agriculture Handbook number 436. Washington, DC: United States Department of Agriculture, Natural Resources Conservation Service.
- Vahdati, N., A. Tehranifar, and F. Kazemi. 2017. “Assessing Chilling and Drought Tolerance of Different Plant Genera on Extensive Green Roofs in an Arid Climate Region in Iran.” Journal of Environmental Management 192: 215–223. doi:10.1016/j.jenvman.2017.01.027.
- van den Berg, L. J. L., H. B. M. Tomassen, J. G. M. Roelofs, and R. Bobbink. 2005. “Effects of Nitrogen Enrichment on Coastal Dune Grassland: A Mesocosm Study.” Environmental Pollution 138 (1): 77–85. doi:10.1016/j.envpol.2005.02.024.
- van der Heijden, M. G. A., R. D. Bardgett, and N. M. van Straalen. 2008. “The Unseen Majority: Soil Microbes as Drivers of Plant Diversity and Productivity in Terrestrial Ecosystems.” Ecology Letters 11 (3): 296–310. doi:10.1111/j.1461-0248.2007.01139.x.
- van der Watt, E., and J. C. Pretorius. 2001. “Purification and Identification of Active Antibacterial Components in Carpobrotus edulis L.” Journal of Ethnopharmacology 76 (1): 87–91. doi:10.1016/s0378-8741(01)00197-0.
- Van Grunsven, R. H. A., F. Bos, B. S. Ripley, C. M. Suehs, and E. M. Veenendaal. 2009. “Release from Soil Pathogens Plays an Important Role in the Success of Invasive Carpobrotus in the Mediterranean.” South African Journal of Botany 75 (1): 172–175. doi:10.1016/j.sajb.2008.09.003.
- Van Wyk, B. E., H. de Wet, and F. R. Van Heerden. 2008. “An Ethnobotanical Survey of Medicinal Plants in the Southeastern Karoo, South Africa.” South African Journal of Botany 74 (4): 696–704. doi:10.1016/j.sajb.2008.05.001.
- Varnham, K. 2006. “Non-Native Species in UK Overseas Territories: A Review.” JNCC Report No. 372. Peterborough, United Kingdom. Accessed May 15 2017. http://jncc.defra.gov.uk/page-3660.
- Varone, L., R. Catoni, A. Bonito, E. Gini, and L. Gratani. 2017. “Photochemical Performance of Carpobrotus edulis in Response to Various Substrate Salt Concentrations.” South African Journal of Botany 111: 258–266.
- Verlaque, R., L. Affre, K. Diadema, C. M. Suehs, and F. Médail. 2011. “Unexpected Morphological and Karyological Changes in Invasive Carpobrotus (Aizoaceae) in Provence (S-E France) Compared to Native South African Species.” Comptes Rendus De Biologies 334 (4): 311–319.
- Vieites-Blanco, C., R. Retuerto, and M. Lema. 2017. “Testing the Effectiveness of Two Biological Control Agents on the Invasive Plant Carpobrotus edulis.” In EMAPI International Conference on Ecology and Management of Alien Plant Invasions. - Syntheses, Challenges and New Opportunities, edited by C. Máguas, C. Crous, and C. Costa, 97. Lisbon, Portugal. September 4–8 .
- Vieites-Blanco, C., and S. J. González-Prieto. 2017. “Effects of Carpobrotus edulis Invasion on Main Litter and Soil Characteristics in Backdune and Rocky Coastal Habitats with Oceanic Climate.” Plant and Soil 1–12. doi:10.1007/s11104-018-3598-5.
- Vieites-Blanco, C., and S. J. González-Prieto. 2018. “Effects of Carpobrotus edulis Invasion on Soil Gross N Fluxes in Rocky Coastal Habitats.” Science of the Total Environment 619: 966–976. doi:10.1016/j.scitotenv.2017.11.154.
- Vilà, M., A. D. Siamantziouras, G. Brundu, I. Camarda, P. Lambdon, F. Médail, E. Moragues, et al. 2008. “Widespread Resistance of Mediterranean Island Ecosystems to the Establishment of Three Alien Species.” Diversity and Distributions 14 (5): 839–851. doi:10.1111/j.1472-4642.2008.00503.x.
- Vilà, M., and C. M. D’Antonio. 1998a. “Fitness of Invasive Carpobrotus (Aizoaceae) Hybrids in Coastal California.” Ecoscience 5 (2): 191–199. doi:10.1080/11956860.1998.11682460.
- Vilà, M., and C. M. D’Antonio. 1998b. “Fruit Choice and Seed Dispersal of Invasive Vs. Noninvasive Carpobrotus (Aizoaceae) in Coastal California.” Ecology 79 (3): 1053–1060. doi:10.1890/0012-9658(1998)079[1053:fcasdo]2.0.co;2.
- Vilà, M., and C. M. D’Antonio. 1998c. “Hybrid Vigor for Clonal Growth in Carpobrotus (Aizoaceae) in Coastal California.” Ecological Applications 8 (4): 1196–1205. doi:10.1890/1051-0761(1998)008[1196:hvfcgi]2.0.co;2.
- Vilà, M., E. Weber, and C. M. D’Antonio. 1998. “Flowering and Mating System in Hybridizing Carpobrotus (Aizoaceae) in Coastal California.” Canadian Journal of Botany 76 (7): 1165–1169. doi:10.1139/b98-130.
- Vilà, M., I. Bartomeus, A. C. Dietzsch, T. Petanidou, I. Steffan-Dewenter, J. C. Stout, and T. Tscheulin. 2009. “Invasive Plant Integration into Native Plant–Pollinator Networks across Europe.” Proceedings of the Royal Society of London 276(1674):3887–3893. The Royal Society. doi:10.1098/rspb.2009.1076.
- Vilà, M., M. Tessier, C. M. Suehs, G. Brundu, L. Carta, A. Galanidis, P. Lambdon, et al. 2006. “Local and Regional Assessments of the Impacts of Plant Invaders on Vegetation Structure and Soil Properties of Mediterranean Islands.” Journal of Biogeography 33 (5): 853–861. doi:10.1111/j.1365-2699.2005.01430.x.
- von Holle, B., and D. Simberloff. 2005. “Ecological Resistance to Biological Invasion Overwhelmed by Propagule Pressure.” Ecology 86: 3212–3218.
- von Willert, D. J. 1992. Life Strategies of Succulents in Deserts: With Special Reference to the Namib Desert. Cambridge: Cambridge University Press.
- von Willert, D. J., D. A. Thomas, W. Lobin, and E. Curdts. 1977. “Ecophysiologic Investigations in the Family Mesembryanthemaceae: Occurrence of CAM and Ion Content.” Oecologia 29: 67–76.
- Washburn, J. O., and G. W. Frankie. 1981. “Dispersal of a Scale Insect, Pulvinariella mesembryanthemi (Homoptera, Coccoidea) on Iceplant in California.” Environmental Entomology 10 (5): 724–727.
- Washburn, J. O., and G. W. Frankie. 1985. “Biological Studies of Iceplant Scales, Pulvinariella mesembryanthemi and Pulvinaria Delottoi (Homoptera, Coccidae), in California.” Hilgardia 53 (2): 1–27.
- Washburn, J. O., G. W. Frankie, and J. K. Grace. 1985. “Effects of Density on Survival, Development, and Fecundity of the Soft Scale, Pulvinariella mesembryanthemi (Homoptera: Coccidae), and Its Host Plant.” Environmental Entomology 14 (6): 755–761.
- Washburn, J. O., J. K. Grace, and G. W. Frankie. 1987. “Population Responses of Pulvinariella mesembryanthemi and Pulvinaria delotti (Homoptera, Coccidae) to Nitrogen and Water Conditions of Their Host Plant.” Environmental Entomology 16 (1): 286–295.
- Webb, C. J., W. R. Sykes, and P. J. Garnock-Jones. 1988. Flora of New Zealand. Vol. IV. Naturalised Pteridophytes, Gymnosperms, Dicotyledons, 95. Christchurch: Botany Division, D.S.I.R.
- Weber, E. 2003. Invasive Plant Species of the World: A Reference Guide to Environmental Weeds. Oxon: CABI Publishing.
- Weber, E., and C. M. D’Antonio. 1999. “Germination and Growth Responses of Hybridizing Carpobrotus Species (Aizoaceae) from Coastal California to Soil Salinity.” American Journal of Botany 86 (9): 1257–1263.
- Weber, E., and C. M. D’Antonio. 2000. “Phenotypic Plasticity in Hybridizing Carpobrotus spp. (Aizoaceae) from Coastal California and Its Role in Plant Invasion.” Canadian Journal of Botany 77 (10): 1411–1418.
- Winter, K. 1973. “NaCl-induzierter Crassulacee nsäurestoffwechsel Bei Einer Weiteren Aizoacee: Carpobrotus edulis [Discovery of NaCl induced crassulacean acid metabolism in a second member of the Aizoaceaefamily: Carpobrotus edulis].” Planta 115 (2): 187–188.
- Winter, K., and J. A. M. Holtum. 2014. “Facultative Crassulacean Acid Metabolism (CAM) Plants: Powerful Tools for Unravelling the Functional Elements of CAM Photosynthesis.” Journal of Experimental Botany 65 (13): 3425–3441.
- Wisura, W., and H. F. Glen. 1993. “The South African Species of Carpobrotus (Mesembryanthema-Aizoaceae).” Contributions from the Bolus Herbarium 15: 76–107.
- Witkowski, E. T. F., and D. T. Mitchell. 1987. “Variations in Soil Phosphorus in the Fynbos Biome, South Africa.” Journal of Ecology 75 (4): 1159–1171.
- Wittmann, A., and A. Flores-Ferrer. 2015. “Analyse économique des espèces exotiques envahissantes en France. [Economic Analysis of Exotic Invasive Species in France].” In Études et documents/Commissariat Général au Développement Durable, edited by X. Bonnet, 2102–4723; No 130. Paris: Commissariat général au développement durable, Service de l’économie, de l’évaluation et de l’intégration du développement durable.
- Wunderlin, R. P., B. F. Hansen, A. R. Franck, and F. B. Essig. 2018. Atlas of Florida Plants Website. Tampa: Institute for Systematic Botany, University of South Florida. Accessed May 15 2017. http://florida.plantatlas.usf.edu/.
- Yelenik, S. G., W. D. Stock, and D. M. Richardson. 2004. “Ecosystem Level Impacts of Invasive Acacia saligna in the South African Fynbos.” Restoration Ecology 12 (1): 44–51.
- Zedler, P. H., and G. A. Scheid. 1988. “Invasion of Carpobrotus edulis and Salix lasiolepis after Fire in a Coastal Chaparral Site in Santa Barbara County, California.” Madroño 35 (3): 196–201.
- Zhang, C., P. W. G. Sale, G. J. Clark, W. Liu, A. I. Doronila, S. D. Kolev, and C. Tang. 2015. “Succulent Species Differ Substantially in Their Tolerance and Phytoextraction Potential When Grown in the Presence of Cd, Cr, Cu, Mn, Ni, Pb, and Zn.” Environmental Science and Pollution Research 22 (23): 18824–18838.
- Zia, S., and M. A. Khan. 2008. “Seed Germination of Limonium stocksii under Saline Conditions.” Pakistan Journal of Botany 40: 683–695.