ABSTRACT
The genus Lavandula L. (Lamiaceae) comprises 40 species, of which only a few are used to extract essential oils for perfume and detergent industries. To date, the compound diversity of Lavandula essential oils, and most notably terpene variation amongst species, is poorly documented. In this work, we report the diversity of terpene content in 33 accessions from the subgenera Lavandula and Fabricia. We used these terpenes to distinguish subgenera and sections and to find autapomorphic and synapomorphic traits as taxonomic markers. We demonstrate that terpene composition is not appropriate for phylogenetic reconstruction and chemotaxonomy in Lavandula, but we show nevertheless that terpene diversity allow for distinguishing the two subgenera, Lavandula and Fabricia and for separating the sections, respectively i/Dentatae, Stoechas and Lavandula, and ii/Pterostoechas, Chaetostachys, Subnudae and Hasikenses. Furthermore, amongst terpenes with putative ecological roles, we found that camphor, 1,8-cineole and carvacrol are synapomorphic traits, or at least markers respective of each subgenus, that trans-α-necrodol is an autapomorphic trait of L. stoechas subsp. luisieri and that linalool is either a symplesiomorphic trait or a homoplasic trait. Based on these results, we propose evolutionary hypotheses and future work directions relative to the putative ecological role of these terpenes in other species and to terpene biosynthesis studies in Lavandula.
Introduction
Lavandula L. (Lamiaceae) is an Old World genus that comprises 40 species and 78 wild infra-specific taxa and hybrids (Upson et al. Citation2004; Passalacqua, Tundis, and Upson Citation2017). More than 400 cultivars have also been identified and selected for ornamental traits or essential oil (EO) quality and yield. The selection of lavenders meets the growing demand for standardized and high-quality EO in perfumery, cosmetic and aromatherapy (Lis-Balchin Citation2002; Cavanagh and Wilkinson Citation2002; Hajhashemi, Ghannadi, and Sharif Citation2003).
Two species and their hybrid are widely cultivated for the quality of their EOs and their interesting properties for human usage. Lavandula angustifolia subsp angustifolia Mill. (fine lavender) and L. latifolia Medik. (spike lavender) are both native to southern Europe. Their hybrid L. × intermedia Emeric ex Loisel. (lavandin) appears naturally in the overlapping geographical areas. Up to 85 volatile organic compounds (VOCs) were found in L. angustifolia EO using two-dimensional GC-MS analysis (Shellie et al. Citation2002). Major compounds are linalool and linalyl acetate, and only traces of camphor are present. EO of fine lavender is therefore particularly appreciated for perfumes and cosmetic products. Lavandin and spike lavender are enriched in linalool, 1,8-cineole and camphor (Guitton et al. Citation2010a, Citation2010b; Herraiz-Peñalver et al. Citation2013). These two latter monoterpenes impact negatively the fragrance and the economic value of lavandin EO. As a consequence, it is mostly used for low-value products like soap and detergent. Including these emblematic species, only 14 of the 40 Lavandula species have been chemically described so far (Table S1; Palá-Paúl et al. Citation2004; Chograni, Messaoud, and Boussaid Citation2008; Chograni et al. Citation2010; Hanamanthagouda et al. Citation2010; Messaoud, Chograni, and Boussaid Citation2012; Ait Said et al. Citation2015; Al-Badani et al. Citation2017). A better knowledge of the chemical diversity found in Lavandula genus could be very helpful for industrial and agronomical purposes.
EOs of lavenders are mainly composed of monoterpenes and sesquiterpenes (Upson et al. Citation2000). The terpene bouquets of Lavandula species have often been used in studies to infer relationships at low taxonomical level (species, subspecies and populations). Chemical content was studied in wild species of the Lavandula subgenus in order to discriminate between taxa and to detect hybrid origins (Garcia-Vallejo Citation1992). For example, several EO chemotypes were identified in L. stoechas populations, distinguished by the ratio of their main terpenes (fenchone, camphor and 1,8-cineole; Kokkalou Citation1988; Skoula, Abidi, and Kokkalou Citation1996; González-Coloma et al. Citation2006, Citation2011; Zuzarte et al. Citation2009; Benabdelkader et al. Citation2011). However, terpenes play an important role in plant chemical communication (Gershenzon and Dudareva Citation2007). They ensure various ecological functions such as defence against enemies (eg phytophagous insects and pathogen microorganisms), attraction of pollinators or parasitoids, or inhibition of competing plants by allelopathy (reviewed in Baldwin Citation2010; Muhlemann, Klempien, and Dudareva Citation2014). Experiments performed in various biotic and abiotic conditions showed alteration of terpene biosynthesis (Gouinguene Citation2002; Wang et al. Citation2010). This variability in terpene content is then the result of gene expression, biochemical backgrounds and influence of the environment. Therefore, chemotaxonomic approaches should take into account such biotic and abiotic bias.
Examining the variation of traits (morphological, chemical, etc.) on a phylogeny is a powerful method to understand evolutionary processes at work shaping fundamental aspects of living organisms. This method was used for example to understand diversification of floral traits during the Caribbean plant radiation (Martén‐Rodríguez et al. Citation2010) or the evolution of defence molecules and floral scent (Wink Citation2003; Raguso et al. Citation2006; Schiestl Citation2010). For example, phylogenetic constraints on floral scent variation were analysed in oil secreting orchids (tribe Diseae), demonstrating a greater effect of phylogeny versus pollinator-mediated selection (Steiner, Kaiser, and Dotterl Citation2011). In Myrtaceae, α-pinene was shown to be the most likely ancestral foliar terpene (Padovan et al. Citation2014). In Araceae, sequential evolution of VOCs was argued against coevolution of floral scent and olfactory preferences of pollinators (Schiestl and Dötterl Citation2012). In Lavandula, this approach has never been tested, despite the availability of several phylogenetic studies for the genus. The genus Lavandula is monophyletic and composed of three subgenera (Kaufmann and Wink Citation1994; Paton et al. Citation2004; Upson et al. Citation2004; Moja et al. Citation2015), each divided into different sections (Table S1). Today, 40 species are acknowledged, but based on phenotypic traits and terpene content, some isolated populations have recently been proposed as new species. For example, populations of L. angustifolia from Calabria were renamed as L. austroapennina (Passalacqua, Tundis, and Upson Citation2017). These authors also proposed to raise L. angustifolia subsp. pyrenaica to species level according to spatial distribution, morphological and molecular data. Furthermore, geographic chemotype variation has been described, although these chemotypes were not raised as new species (Jianu et al. Citation2013). Lavandula occupies a large diversity of ecological niches: high mountains, Mediterranean climate regions, rocky slopes in deserts of North Africa and Arabia, and disturbed habitats in India (Upson et al. Citation2004). This chemodiversity has never been studied from a phylogenetic point of view.
To increase botanical knowledge on the genus Lavandula, we analysed in the present work the chemical composition of 33 taxa of Lavandula including for the first time 16 species of the subgenus Fabricia. Moreover, we compared terpene composition of inflorescences and leaves in 26 species. The main purpose of this work was to use terpene profiles to separate taxa in the genus Lavandula. As such, we address the following questions: can terpene diversity be used to distinguish subgenera and sections? Can terpenes be used as chemotaxonomical markers at the species level? Is it possible to find autapomorphic and synapomorphic traits amongst terpenes as taxonomic markers?
Material and methods
Plant material and sample collection
Thirty-three Lavandula taxa accessions from two subgenera and seven botanical sections were used. Seeds of wild plants were collected in the natural distribution area of each species by an experimented botanist of the Conservatoire National des Plantes Médicinales Aromatiques et Industrielles (CNPMAI) (Table S2). After sowing, plants were cultivated in the same field at the CNPMAI (Milly-la-Forêt, France) and collected during the first year for VOC analysis.
Terpenes extraction and analysis
Leaves and full bloom inflorescences were collected separately for each species and three samples were collected per species and organ. Inflorescence or leaf extracts were missing for L. stoechas subsp. luiseiri, L. aristibracteata, L. nimmoi, L. hasikensis and L. samhanensis. For each sample, 100 mg of fresh weight was added to 2 mL of hexane. Extraction was performed at 4°C overnight. The organic phase was then collected and stored at −20°C until gas chromatography and mass spectrometry (GC-MS) analysis.
Compounds were separated by GC (Agilent 6850 equipment) on a 30-m-long capillary HP-Innowax column. To help improve compound identification using the Adams database (Adams Citation2007), a sub-sample was analysed with a DB5 column. Compounds identification was performed by MS (Agilent 5973). Chromatographic conditions were as follows: helium was used as a carrier gas at a flow rate of 1.2 mL min−1; the oven was set at 40 °C for 6 min after injection followed by a 1°C min−1 temperature ramp to 60°C, a 2°C min−1 temperature ramp to 140°C and a 12°C min−1 temperature ramp to 240°C. Temperature was then kept on hold at 240°C for 5 min; the injection volume was 2 μL in split mode with a 2:1 split ratio; temperatures of the ion source and of the GC-MS interface were set at 230°C. Ionizing voltage (ei-mode) was set at 70 eV, and the mass scan rate and range were 2.45 scans s−1 and 35–350 m.z−1.
Compound identification and metabolite profiling
The R package MSeasy was used to generate a final matrix with each sample in line and relative area of compounds in column. MSeasy facilitates the profiling of numerous GC-MS metabolites by clustering mass spectra (Nicolè et al. Citation2012). It is particularly well suited when several species are compared and when shifts in retention time are observed between taxa. Furthermore, the unbiased and unsupervised method of analysis does not require knowledge about compounds and works well on non-model species where compounds are usually unknown. Clustering of mass spectra was achieved with the MS.clust function, with parameters set as follows: mz = 30:600, clV = TRUE, ncmin = 2, ncmax = 250, varRT = 0.2, disMeth = “Euclidean”, linkMeth = “ward”, clustMeth = “hierarchical”. The output matrix was corrected manually to obtain the final matrix for post-processing. Cluster identification was obtained by comparing mass spectra with those of commercial standards and with NIST and laboratory databases. It was refined with Kovats indexes using an n-alkanes C8–C20 solution (Fluka, Lyon, France). Tables S3 and S4 list the chemical composition of the species expressed as relative percentages of the total. Table S5 presents the retention times from HP-Innowax and DB5 columns, and the estimated retention index of each molecule compared with those presented in mass spectra database (Adams Citation2007) and from webbook.nist.gov. Tables S3, S4 and S5 can be downloaded respectively from the following hyperlinks: https://mycore.core-cloud.net/index.php/s/YYUQHYdMwCS4B0J/download; https://mycore.core-cloud.net/index.php/s/lOPo8OVvkRSa1Zy/download; https://mycore.core-cloud.net/index.php/s/xfXVdphCaNvzBuO/download.
Statistical analyses
We performed statistical analyses according to Hervé, Nicolè, and Lê Cao (Citation2018). Chemical compounds could not be considered as independent variables because some compounds participate in the same biosynthetic pathway. Furthermore, the number of variables is higher than the number of samples, and these two characteristics prevented us from using classical analytical methods. Therefore, partial least squares discriminant analyses (PLS-DA) were more suited to discriminate terpene content at different phylogenetic levels. A centred log ratio transformation appropriate for compositional data was applied on our matrix. PLS-DA were performed using R v3.2.3 (CRAN R project) and the package mixOmics. The package RVAideMemoire was used to validate the PLS-DA with cross-validations (MVA.cv function) and to calculate misclassification rate. To test which factors significantly influenced the observed chemical variation, we performed a distance-based redundancy analysis (dbRDA). RDA is a method by which to extract and summarize the variation of a set of response variables (taxonomic levels and organs) that can be explained by a set of explanatory variables (chemical composition). It is an extension of multiple linear regression allowing regression of multiple response variables on multiple explanatory variables. Because our number of explanatory variables is higher than the number of objects (samples or observations) in our data matrix, we used dbRDA extended for a response dataset transformed as a distance matrix. The significance of the response variables was obtained with the function MVA.anova from RVAideMemoire package.
Terpene assignment on phylogeny
A maximum likelihood phylogenetic tree based on cpDNA markers published in Moja et al. (Citation2015) was used as a basis to explore phylogenetic hypotheses about compound evolution. The MacClade software (© 2011 by David R. Maddison and Wayne P. Maddison) was used to depict compound distribution on the phylogenetic tree using a binary matrix of terpene presence for each organ, leaves and inflorescences. Trees were redrawn using Inkscape v0.92.
Results
Mass spectra obtained from 148 samples from 33 Lavandula taxa were aligned to construct a profiling matrix. A total of 107 VOCs with a relative peak area of ≥ 0.5 % of the integrated peaks were selected (Tables S3 and S4). Eighty-seven VOCs were identified with RI and NIST database, nine terpenes were identified with NIST database, and 11 molecules were not identified that may correspond to co-elution or to compounds not present in the NIST database (Table S5).
Chemical diversity at different taxonomical levels
The bouquet of Lavandula species, including the 16 newly analysed species in this study, was dominated by monoterpenes and sesquiterpenes. No compound was detected in L. bipinnata and L. buchii leaves, as well as in L. dhofarensis inflorescences. The mean absolute quantity of terpenes was about 50–100 times higher in samples of the subgenus Lavandula than those of the subgenus Fabricia.
Compound diversity per sample was significantly higher in the subgenus Lavandula with 26.8 ± 7.3 compounds versus 7.8 ± 4.3 compounds for the subgenus Fabricia (Wilcoxon rank test W = 129.5, P < 10−6). The number of compounds differed greatly between species for both subgenera, from 1.3 (L. nimmoi) to 14.6 (L. minutolii) in the sub-genus Fabricia, and from 22.8 (L. angustifolia pyrenaica) to 36.5 (L. × heterophylla) in the sub-genus Lavandula. In the 16 newly analysed Fabricia species, the mean number of compounds was 7.7 ± 4.3 with terpinolene, myrcene and carvacrol as main leaf compounds and β-caryophyllene, terpinolene, α-pinene and myrcene as main inflorescence compounds. We performed a PLS-DA in order to identify differences in chemical composition at the subgenus level (). The first discriminant axis represented 100% of the intergroup variance with a clear distinct chemical composition between the two subgenera. The cross-validation test gave only 1.9% of misclassifications with a standard error of 0.26%. The most discriminant compounds were camphor, camphene, 1,8-cineole, borneol, linalool, β-pinene and cis-sabinene hydarte, which are more specific to the subgenus Lavandula, whereas α-campholenal, carvacrol and an unknown VOC U12 were specific to the subgenus Fabricia. The chemical composition of the 22 species within the subgenus Fabricia was much more homogeneous than for the 11 species of the subgenus Lavandula as indicated by the size of the ellipse on . A PLS-DA performed separately for each subgenus revealed differences in chemical composition at the section level (). The three sections Lavandula, Stoechas and Dentatae of the subgenus Lavandula () were well separated with a misclassification rate of 3.49 ± 0.55% using cross-validation tests. The section Stoechas showed the highest dispersion of individuals, confirming the high terpene diversity amongst the four species of this section. Selina-3,7(11)-diene, γ-elemene, trans-carveol and fenchone were discriminant compounds associated with the section Stoechas, whereas β-caryophyllene was associated with the Lavandula section. Lavandula and Stoechas sections differed from the section Dentatae on the second axis by the amount of p-cymene-8-ol, α-chamigrene, myrtenal, myrtenol and β-eudesmol. Within the subgenus Fabricia ()), the section Pterostoechas was characterized by a high amount of carvacrol. Section Hasikensis presented an unknown VOC U10 and δ-3-carene. Section Subnudae was differentiated by α-humulene, germacrene D, dihydrocarveol, piperitone, borneol, eucarvone and β-caryophyllene. The mean mis-classification rate was low (4.65 ± 0.43%), indicating clear differences between sections of the subgenus Fabricia summarized by the two first axes.
Figure 1. PLS-DA on the compositional matrix of VOCs. For each graph, the 10 most discriminant compounds (with the highest VIP) were represented in grey font. (a) PLS-DA at the subgenus level. (b) PLS-DA within the Lavandula subgenus at the section level. (c) PLS-DA within the Fabricia subgenus at the section level. Ellipse includes 95% of the samples.
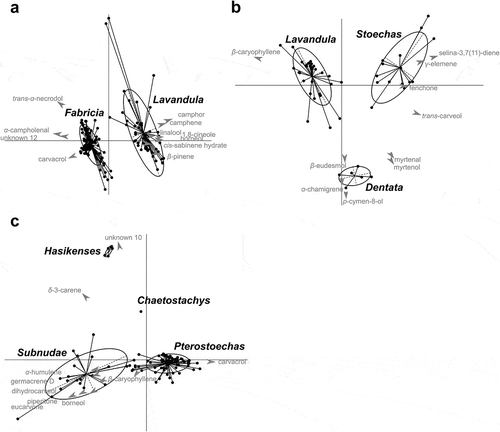
Differences in terpene composition between leaf and inflorescence prevent a chemotaxonomical approach
We performed a distance-based redundancy analysis (dbRDA) to test which factors and interactions of factors significantly influenced the observed chemical variation within each subgenus (). We excluded Hasikenses and Chaetostachys from the analysis sections because of the lack of samples for both organs.
Figure 2. dbRDA plots of the interaction between sections and organs within the Lavandula subgenus (a) and within the Fabricia subgenus (b). Ellipses were added manually to depict groups of samples. L, leaves; I, inflorescence; Den, Dentatae section; Lav, Lavandula section; Stoe, Stoechas section; Sub, Subdunae section; Ptero, Pterostoechas section.
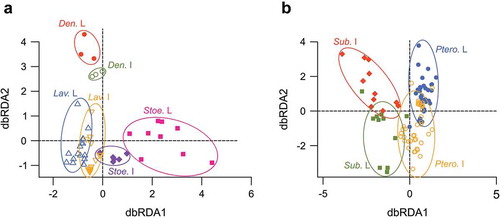
Within the subgenus Lavandula (), inflorescences of the section Stoechas had similar chemical profiles, whereas leaves showed a larger variability. In the section Lavandula, the level of variability was similar between leaves and inflorescences. Within the subgenus Fabricia (), the chemical profiles of inflorescences and leaves in section Pterostoechas overlapped partially. Differences were more noticeable between organs in the section Subnudae. For both subgenera Lavandula and Fabricia, there was a significant interaction between organ and section indicating that the chemical composition varied differently within a section between leaf and inflorescence (Lavandula: df = 2, F = 3.32, P < 0.001; Fabricia: df = 1, F = 2.3, P < 0.001). This result shows that using chemical composition is not appropriate to perform phylogenetic reconstructions because resulting phylogenies will have topologies depending on the organ analysed. It is therefore preferable to use a molecular phylogeny to examine the evolution of chemical characters in order to explore the evolution of scent within the genus Lavandula.
Some terpenes are restricted to subgenera, clades or organs
We showed that terpene composition was highly diverse within the genus Lavandula, both between the two subgenera and between each section within each subgenus. To explore evolutionary hypotheses about terpene biosynthesis pathways in lavender, we correlated terpene presence to species relationships in Lavandula using a cpDNA phylogenetic tree (; Moja et al. Citation2015). Sections Hasikenses and Chaetostachys, absent from the cpDNA tree, were excluded from this analysis. We focused our attention on five terpenes because of their putative ecological functions and their distribution in the genus Lavandula (): (1) linalool as a potent and ubiquitous VOC with antagonistic functions as both pollinator attractor and insect repellent (Raguso and Pichersky Citation1999; Knudsen et al. Citation2006; Junker, Gershenzon, and Unsicker Citation2011; Raguso Citation2016); (2) camphor, carvacrol and 1,8-cineole as defence molecules and because of their role in biotic and abiotic stresses in some plant species (Muñoz-Bertomeu, Arrillaga, and Segura Citation2007; Zuzarte et al. Citation2009; Herraiz-Penalver Citation2013; Thompson et al. Citation2013; Friedman Citation2014; Jeon, Yang, and Lee Citation2014; Al-Badani et al. Citation2017); (3) necrodol, an anti-insectan terpene from defensive secretion of carrion beetle (Eisner et al. Citation1986) only found so far in two plant species, L. stoechas subsp. luisieri (García-Vallejo et al. Citation1994; González-Coloma et al. Citation2006, Citation2011) and Evolvulus alsinoides L., a species belonging to Convolvulaceae (Kashima and Miyazawa Citation2014).
Figure 3. Mapping of presence or absence of linalool (a), camphor (b), 1,8-cineole (c) and carvacrol (d) along a maximum likelihood tree based on cpDNA markers (Moja et al. Citation2015). Mapping was done with the help of MacClade, and resulting trees were then redrawn. Bold branches denote the presence of a molecule, thin branches absence and dotted branches ambiguous status. Den, Dentatae section; Lav, Lavandula section; Stoe, Stoechas section; Sub, Subdunae section; Ptero, Pterostoechas section; Chae, Chaetostachys section.
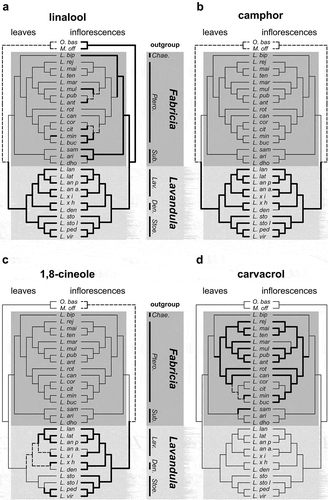
Linalool was found in inflorescences of at least one species of each botanical section of Lavandula and was not detected only in nine species (), Tables S3 and S4). In leaves, linalool was detected in all species of the subgenus Lavandula, except L. latifolia. By contrast, we only detected linalool in L. minutolii leaves within the subgenus Fabricia. Thus, it is likely that linalool biosynthesis was present in the common ancestor of all Lavandula and actively synthesized in inflorescences. Co-option of linalool synthesis in leaves of the Lavandula subgenus ancestor could have served an important ecological role, yet to be discovered, inherited in extant plants. However, our data cannot rule out a shutdown of the biosynthesis in the leaves of the ancestor of subgenus Fabricia.
Camphor was present in variable proportions in the Lavandula subgenus, both in inflorescences and in leaves (), Tables S3 and S4), whereas it was totally absent in the Fabricia dataset. The camphor biosynthetic pathway also produces limonene, α-pinene, α-terpinolene, β-myrcene, borneol and camphene (Wise et al. Citation1998). Proportions of these chemicals varied in each species examined (Tables S3 and S4). Lavandula angustifolia and L. latifolia inflorescences produced a small percentage of camphor (< 1–5%), whereas inflorescences of the species found in the section Stoechas produce 5–48% of camphor. Camphor was only absent from L. dentata inflorescences. These observations could pinpoint a crucial ecological role of camphor in the subgenus Lavandula, likely as a resistance factor against biotic or abiotic stresses. Like camphor, 1,8-cineole was only detected in the subgenus Lavandula, but with more secondary losses, notably in leaves ()).
Contrary to camphor and 1,8-cineole, carvacrol was detected only in the Fabricia subgenus ()). It was present in inflorescences and leaves of 10 of the 13 species making the section Pterostoechas, in relative quantity ranging from 0 to 75% (Tables S3 and S4). It was not detected in two species: L. coronopifolia and L. citriodora. In the section Subnudae, it was only detected in leaves of L. samhanensis. Taken together, these results show that camphor and 1,8-cineole are likely synapo-morphies of the Lavandula subgenus, whereas carvacrol presence is a synapomorphy of the subgenus Fabricia with some secondary losses.
Trans-α-necrodol, which was found to be discriminant at the subgenus level, was detected specifically in L. stoechas subsp. luisieri (10% of the chemical profile). Trans-α-necrodyl acetate was also specific to L. stoechas subsp. luisieri (34% of the chemical profile; Tables S3 and S4).
Discussion
In this study, we analysed terpenes in inflorescences and leaves in 33 lavender taxa in order to assess hypotheses about putative molecular markers of the clades composing Lavandula. As a general observation, the subgenus Lavandula showed a higher chemodiversity than the subgenus Fabricia. Indeed, we found a heterogeneous distribution of terpenes amongst species of the subgenus Lavandula while terpene content was relatively homogeneous within the subgenus Fabricia. We also demonstrated that the chemodiversity was not appropriate for phylogenetic reconstruction in Lavandula because the resulting tree depends on the organ analysed. We thus favoured the use of a molecular phylogeny to examine the evolution of chemical characters to find terpenes that could be used as taxonomic markers or to support evolutionary hypotheses. Such chemicals need to be non-neutral traits. Consequently, we focused our attention on terpenes with putative ecological roles demonstrated in other species because their independent evolutionary selection and retention in Lavandula are likely a consequence of environmental pressure and the hallmark of adaptation.
Terpenes cannot be used for chemotaxonomy in the Lavandula genus
Our PLS-DAs separated with great confidence the two subgenera (98%) and the different sections (96.5% for subgenus Lavandula; 95.4% for subgenus Fabricia). Performing a dbRDA on our dataset showed that an interaction effect exists between organs (leaves and inflorescences) and subgenera sections. As such, building a classification on chemical dataset will produce results depending on organs that have been sampled, thus leading to mis-classifications. This was firstly stated by Knudsen et al. (Citation2006). At the infra-specific level, chemical composition may still serve to establish chemotypes amongst populations (Jiang et al. Citation2006; Waterman Citation2007; Rustaiee et al. Citation2013). However, relating chemical composition to established phylogeny is a more powerful approach and demonstrates that not all molecules (and more accurately their biosynthetic pathways) have the same evolutionary trajectory. It is particularly true concerning terpenes because terpene synthases can easily neofunctionalize (Chen et al. Citation2011). Notably, it has been shown that only a few mutations in the catalytic site are sufficient to modify end products of these enzymes (Kampranis et al. Citation2007). The similarity between terpene synthases sequences is rather dependent on the species and less linked to the function. For example, the fenchol synthase of L. pedunculata has a very similar sequence to other terpene synthases of lavenders but fewer similarities with the fenchol synthase of Ocimum basilicum (data not shown and Chen et al. Citation2011). This high evolutionary plasticity is likely the consequence of a strong selection exerted by the environment towards plant terpene diversification allowing for better adaptation of the species.
Camphor, 1,8-cineole and carvacrol are synapomorphic traits, or at least markers, of each subgenus, Lavandula and Fabricia
Amongst discriminating compounds between subgenera, three oxygenated monoterpenes, camphor, 1,8-cineole and carvacrol, are highly produced in many species. It is striking that camphor and 1,8-cineole are only present in the subgenus Lavandula, and at the opposite, carvacrol only in the subgenus Fabricia. As such, camphor and 1,8-cineole biosynthesis are synapomorphies of the subgenus Lavandula whereas carvacrol biosynthesis might be a syn-apomorphies of the subgenus Fabricia. However, we cannot rule out an independent evolution of carvacrol biosynthesis in L. samhanensis, thus restricting carvacrol as a synapomorphy of the section Pterostoechas only.
The role of camphor in many plants is unknown. However, its repellent function and toxicity have been established against red fire ants (Zhang et al. Citation2014), and camphor is used as acaricidal agent against house dust mites (Jeon, Yang, and Lee Citation2014). Carvacrol, like camphor, is known for its broad range of insecticidal and antibacterial activities (reviewed in Friedman Citation2014; Nabavi et al. Citation2015). Interestingly, a similar role in plant adaptation to altitude has been reported for camphor, 1,8-cineole and carvacrol: at low altitudes, Lavandula latifolia, which belongs to the subgenus Lavandula, produces more camphor and more 1,8-cineole than at high altitudes (Muñoz-Bertomeu, Arrillaga, and Segura Citation2007; Herraiz-Peñalver et al. Citation2013); similarly, populations of Thymus vulgaris cultivated at low altitude were shown to accumulate carvacrol (Thompson et al. Citation2013). Thus, our results suggest that camphor and 1,8-cineole on the one hand and carvacrol on the other hand might fulfil the same role against environmental stresses (eg insects, climate) for subgenera Lavandula and Fabricia.
While they might have similar functions, camphor, 1,8-cineole and carvacrol are biosynthesized by different pathways (Degenhardt, Köllner, and Gershenzon Citation2009; Crocoll Citation2011; Demissie et al. Citation2012; Sarker et al. Citation2012). Camphor biosynthesis is mediated by three enzymes (Wise et al. Citation1998), not all characterized in lavenders. In lavender, 1,8-cineole is synthesized by a terpene synthase. This 1,8-cineole synthase produces 1,8-cineole as its main product (80%) and a few by-products including sabinene (7.9%), α-phellandrene (6.6%), limonene (2.3%) and α-terpineol (1.7%) (Demissie et al. Citation2012). Carvacrol biosynthetic pathway is not entirely identified but is known to contain an oxidation step of γ-terpinene by a cytochrome P450 mono-oxygenase (Crocoll Citation2011). In lavender, carvacrol biosynthetic pathway is yet to be discovered. A likely scenario is the neofunctionalization of a cytochrome P450 monooxygenase in the ancestor of the subgenus Fabricia. Camphor and by-products of the camphor pathway, 1,8-cineole and carvacrol, content varied in the lavender species we examined, suggesting a species-specific regulation of the three pathways. A more thorough study is now needed to unveil putative co-regulations of camphor and 1,8-cineole biosynthetic pathways in species belonging to the subgenus Lavandula. Moreover, it will be important to determine if correlations can be clearly established between environmental factors, most likely insect interactions and climatic conditions related to altitude, and camphor, 1,8-cineole and carvacrol biosynthetic pathway activity in lavender.
Linalool distribution is complex and could be considered as a symplesiomorphy or as a homoplasy
Linalool is a widespread monoterpene and is found in 70% of all angiosperms (Knudsen et al. Citation2006). Several linalool synthases from different classes of terpene synthases have been described so far (reviewed in Chen et al. Citation2011). Linalool and its acetate derivative are emblematic major compounds of the inflorescences EO of the fine lavender. Linalool is synthesized in inflorescences with a maximum rate at full bloom stage (Landmann et al. Citation2007; Guitton et al. Citation2010a). We found that 72% of the species synthesized linalool in at least one organ examined. Overall, these results suggest a single ancestral biosynthetic pathway (or perhaps a single linalool synthase) that has evolved through modulation of its activity. Thus, linalool could be regarded as a symplesiomorphy. However, in the subgenus Fabricia, no linalool synthase gene sequence is known yet. We cannot entirely exclude the independent evolution of a linalool synthase in Fabricia, as compared with Lavandula and linalool distribution in lavender would reflect then a homoplasy of the character. Linalool and its acetate derivative content vary greatly between species and organs. The exceptional level of linalool in L. angustifolia might suggest an important role of this molecule for adaptation of the species to its original environment. For example, linalool was found to be the most toxic terpene over 21 tested during experimental fumigations on larvae of Plutella xylostella, a parasite damaging economically important cruciferous crops (Yi et al. Citation2016). A recent review (Raguso Citation2016) presents the complex interplay between pollinator attraction and plant defence mediated by linalool and its derivatives. Thus, linalool could have multiple and context-dependent functions in lavender.
Trans-α-necrodol and its acetate derivative as an autapomorphic trait of L. stoechas subsp. luisieri
By contrast to linalool, necrodol is almost absent from the plant kingdom. This type of terpene has only been found in a lavender species L. stoechas subsp. luisieri (García-Vallejo et al. Citation1994) and in a Convolvulaceae species, Evolvulus alsinoides L. (Kashima and Miyazawa Citation2014). We confirmed the presence of trans-α-necrodol in L. stoechas subsp. luisieri and could not detect this molecule in any other Lavandula species. Thus, acquisition of the capacity to synthesize trans-α-necrodol is likely an autapomorphy of L. stoechas subsp. luisieri. Trans-α-necrodol, was firstly discovered in the rectal secretions of a North American beetle, Necrodes surinamensis (Eisner et al. Citation1986). A closely related molecule, β-necrodol, has repellent properties to 31 species of insects including Coleoptera, Hymenoptera and Hemiptera species (Eisner et al. Citation1986). Acquisition of trans-α-necrodol synthesis by a lavender species raises the question of the origin of the gene coding for the enzyme. Given that terpene synthases can easily neofunctionalize (Chen et al. Citation2011), it is likely that a mutation after a possible gene duplication occurred during speciation of L. stoechas subsp. luisieri. An alternative hypothesis could be a gene transfer that occurred from an insect to the ancestor of L. stoechas subsp. luisieri, either directly or not (Gao et al. Citation2014). In any case, a large quantity of trans-α-necrodol and of its acetate derivative in the leaves of L. stoechas subsp. luisieri could be a key feature in adaptation and speciation of this endemic lavender of the South-West and West Iberian peninsula. A chemical ecology study of this species would certainly shed light on the potential ecological role in plants of this class of terpenes.
Conclusion
In this study, we reported for the first time the chemical composition of 33 over 40 taxa composing the genus Lavandula. We included 16 species of subgenus Fabricia not previously examined for their terpene content before. Furthermore, we compared terpenes of inflorescences and leaves in 26 lavender species. We have obtained 107 molecules including 88 terpenes. We used these data to test their abilities to discriminate between taxa of the genus Lavandula and showed that terpene diversity could be used to distinguish the two subgenera, Lavandula and Fabricia. In both subgenera, PLS-DA allowed for separating the sections, respectively i/Dentatae, Stoechas and Lavandula, and ii/Pterostoechas, Chaetostachys, Subnudae and Hasikenses.
As the opposite, we showed that terpenes cannot be used as chemotaxonomical markers at the species level. Indeed, dbRDA showed an interaction between leaves, inflorescences and sections. Thus chemotaxonomical classification on leaves and inflorescences would be incongruent. Finally, we superimposed some terpenes to a cpDNA phylogenetic tree and found interesting autapomorphic and synapomorphic traits. We concluded that the distributions of camphor and carvacrol are synapomorphies of respectively Lavandula and Fabricia subgenera, that trans-α-necrodol is an autapomorphy of L. stoechas subsp. luisieri and that linalool is either a symplesiomorphic trait or a homoplasic trait. We proposed evolutionary hypotheses for linalool, 1,8-cineole, camphor, carvacrol and trans-α-necrodol related to their ecological roles in other species and to the current knowledge about terpene synthases in Lavandula. These hypotheses could be the basis of a future work on the evolution of the genes involved in the biosynthesis of terpenes in Lavandula.
Supplemental Material
Download MS Excel (15.3 KB)Supplemental Material
Download MS Excel (44.1 KB)Supplemental Material
Download MS Excel (92.8 KB)Table_S2.docx
Download MS Word (22.2 KB)Table_S1.docx
Download MS Word (19.2 KB)figure_S1.png
Download PNG Image (127.8 KB)Acknowledgements
We thank Nicolas Boyer for lavender cultivation. We are also grateful to the CNPMAI for providing plants. We thank Sylvie Baudino for scientific discussions, Frédéric Hache and Neil Finn (Department of Foreign Languages, UJM) for text revision.
Disclosure statement
No potential conflict of interest was reported by the authors.
SUPPLEMENTAL DATA
Supplemental data for this article can be accessed here.
Additional information
Funding
Notes on contributors
Yann Guitton
Yann Guitton was the PhD student involved in the present project. He is now manager of a metabolomic core facility (Laberca, Nantes, France) and bio-informatician for workflow4metabolomics.org project dedicated to MS and NMR based metabolomics studies processing.Contribution: He contributed to data collection and interpretation, and performed critical reading of the manuscript
Florence Nicolè
Florence Nicolè is junior lecturer at University Jean Monnet. Her field of expertise is chemical ecology and lavender volatiles. She develops biostatistical tools to explore metabolomic data. Contribution: She analysed data and collaborated to the manuscript preparation.
Frédéric Jullien
Frédéric Jullien is reader at University Jean Monnet. He is a plant molecular biologist working on TPSs of lavenders and other species. Contribution: He analysed a part of the sampling and collaborated to the manuscript writing.
Jean-Claude Caissard
Jean-Claude Caissard is senior lecturer at University Jean Monnet. His field of expertise is chemical ecology and Rosaceae and Lamiaceae volatiles. Contribution: He contributed to scientific discussions and manuscript correction.
Denis Saint-Marcoux
Denis Saint-Marcoux is junior lecturer at University Jean Monnet. He is expert in plant evolution and bioinformatics analyses. Contribution: He contributed to scientific discussions and manuscript preparation.
Laurent Legendre
Laurent Legendre is full professor at University Jean Monnet. He is a plant biochemist. Contribution: He collected samples and supervised the experimental design.
Bernard Pasquier
Bernard Pasquier is a botanist, head of the CNPMAI and expert of Lamiaceae. Contribution: He collected and cultivated all the lavenders analysed.
Sandrine Moja
Sandrine Moja is senior lecturer at University Jean Monnet. Her field of expertise is volatiles diversity and species evolution. Contribution: She managed the compilation of the data, made analyses and wrote the manuscript.
References
- Adams, R. P. 2007. Identification of Essential Oil Components by Gas Chromatography/Mass Spectroscopy. 4th ed. Carol Stream, IL: Allured Pub. Corp.
- Ait Said, L., K. Zahlane, I. Ghalbane, S. El Messoussi, A. Romane, C. Cavaleiro, and L. Salgueiro. 2015. “Chemical Composition and Antibacterial Activity of Lavandula coronopifolia Essential Oil against Antibiotic-Resistant Bacteria.” Natural Product Research 29: 582–585. doi:10.1080/14786419.2014.954246.
- Al-Badani, R. N., J. K. R. Da Silva, W. N. Setzer, N. A. Awadh Ali, B. A. Muharam, and A. A. J. A. Fahad. 2017. “Variations in Essential Oil Compositions of Lavandula pubescens (Lamiaceae) Aerial Parts Growing Wild in Yemen.” Chemistry & Biodiversity 14: 1–8. doi:10.1002/cbdv.201600286.
- Baldwin, I. T. 2010. “Plant Volatiles.” Current Biology 20: R392–R397. doi:10.1016/j.cub.2010.02.052.
- Benabdelkader, T., A. Zitouni, Y. Guitton, F. Jullien, D. Maitre, H. Casabianca, L. Legendre, and A. Kameli. 2011. “Essential Oils from Wild Populations of Algerian Lavandula stoechas L.: Composition, Chemical Variability, and in Vitro Biological Properties.” Chemistry & Biodiversity 8: 937–953. doi:10.1002/cbdv.201000301.
- Cavanagh, H. M. A., and J. M. Wilkinson. 2002. “Biological Activities of Lavender Essential Oil.” Phytotherapy Research 16: 301–308. doi:10.1002/ptr.1103.
- Chen, F., D. Tholl, J. Bohlmann, and E. Pichersky. 2011. “The Family of Terpene Synthases in Plants: A Mid-Size Family of Genes for Specialized Metabolism that Is Highly Diversified Throughout the Kingdom: Terpene Synthase Family.” The Plant Journal 66: 212–229. doi:10.1111/j.1365-313X.2011.04520.x.
- Chograni, H., C. Messaoud, and M. Boussaid. 2008. “Genetic Diversity and Population Structure in Tunisian Lavandula stoechas L. And Lavandula multifida L. (Lamiaceae).” Biochemical Systematics and Ecology 36: 349–359. doi:10.1016/j.bse.2007.11.005.
- Chograni, H., Y. Zaouali, C. Rajeb, and M. Boussaid. 2010. “Essential Oil Variation among Natural Populations of Lavandula multifida L. (Lamiaceae).” Chemistry & Biodiversity 7: 933–942. doi:10.1002/cbdv.200900201.
- Crocoll, C. 2011. “Biosynthesis of the Phenolic Monoterpenes, Thymol and Carvacrol, by Terpene Synthases and Cytochrome P450s in Oregano and Thyme.” Academic Dissertation. Der Biologisch-Pharmazeutischen Fakultät der Friedrich-Schiller-Universität Jena
- Degenhardt, J., T. G. Köllner, and J. Gershenzon. 2009. “Monoterpene and Sesquiterpene Synthases and the Origin of Terpene Skeletal Diversity in Plants.” Phytochemistry 70: 1621–1637. doi:10.1016/j.phytochem.2009.07.030.
- Demissie, Z. A., M. A. Cella, L. S. Sarker, T. J. Thompson, M. R. Rheault, and S. S. Mahmoud. 2012. “Cloning, Functional Characterization and Genomic Organization of 1,8-Cineole Synthases from Lavandula.” Plant Molecular Biology 79: 393–411. doi:10.1007/s11103-012-9920-3.
- Eisner, T., M. Deyrup, R. Jacobs, and J. Meinwald. 1986. “Necrodols: Anti-Insectan Terpenes from Defensive Secretion of Carrion Beetle (Necrodes surinamensis).” Journal of Chemical Ecology 12: 1407–1415. doi:10.1007/BF01012360.
- Friedman, M. 2014. “Chemistry and Multibeneficial Bioactivities of Carvacrol (4-Isopropyl-2-Methylphenol), a Component of Essential Oils Produced by Aromatic Plants and Spices.” Journal of Agricultural and Food Chemistry 62: 7652–7670. doi:10.1021/jf5023862.
- Gao, C., X. Ren, A. S. Mason, H. Liu, M. Xiao, J. Li, and D. Fu. 2014. “Horizontal Gene Transfer in Plants.” Functional & Integrative Genomics 14: 23–29. doi:10.1007/s10142-013-0345-0.
- Garcia-Vallejo, M. I. 1992. “Aceites Esenciales De Las Lavandulas Ibericas. Ensayo De La Quimiotaxonomıa.” Ph.D. Thesis. Universidad Complutense de Madrid, Facultad de Biologıa.
- García-Vallejo, M. I., M. C. García-Vallejo, J. Sanz, M. Bernabe, and A. Velasco-Negueruela. 1994. “Necrodane (1,2,2,3,4-Pentamethylcyclopentane) Derivatives in Lavandula luisieri, New Compounds to the Plant Kingdom.” Phytochemistry 36: 43–45. doi:10.1016/S0031-9422(00)97009-2.
- Gershenzon, J., and N. Dudareva. 2007. “The Function of Terpene Natural Products in the Natural World.” Nature Chemical Biology 3: 408–414. doi:10.1038/nchembio.2007.5.
- González-Coloma, A., D. Martín-Benito, N. Mohamed, M. C. García-Vallejo, and A. C. Soria. 2006. “Antifeedant Effects and Chemical Composition of Essential Oils from Different Populations of Lavandula luisieri L.” Biochemical Systematics and Ecology 34: 609–616. doi:10.1016/j.bse.2006.02.006.
- González-Coloma, A., F. Delgado, J. M. Rodilla, L. Silva, J. Sanz, and J. Burillo. 2011. “Chemical and Biological Profiles of Lavandula luisieri Essential Oils from Western Iberia Peninsula Populations.” Biochemical Systematics and Ecology 39: 1–8. doi:10.1016/j.bse.2010.08.010.
- Gouinguene, S. P. 2002. “The Effects of Abiotic Factors on Induced Volatile Emissions in Corn Plants.” Plant Physiology 129: 1296–1307. doi:10.1104/pp.001941.
- Guitton, Y., F. Nicolè, S. Moja, N. Valot, S. Legrand, F. Jullien, and L. Legendre. 2010a. “Differential Accumulation of Volatile Terpene and Terpene Synthase mRNAs during Lavender (Lavandula angustifolia and L. x Intermedia) Inflorescence Development.” Physiologia Plantarum 138: 150–163. doi:10.1111/j.1399-3054.2009.01315.x.
- Guitton, Y., F. Nicolè, S. Moja, T. Benabdelkader, N. Valot, S. Legrand, F. Jullien, and L. Legendre. 2010b. “Lavender Inflorescence: A Model to Study Regulation of Terpenes Synthesis.” Plant Signaling & Behavior 5: 749–751. doi:10.4161/psb.5.6.11704.
- Hajhashemi, V., A. Ghannadi, and B. Sharif. 2003. “Anti-Inflammatory and Analgesic Properties of the Leaf Extracts and Essential Oil of Lavandula angustifolia Mill.” Journal of Ethnopharmacology 89: 67–71.
- Hanamanthagouda, M. S., S. B. Kakkalameli, P. M. Naik, P. Nagella, H. R. Seetharamareddy, and H. N. Murthy. 2010. “Essential Oils of Lavandula bipinnata and Their Antimicrobial Activities.” Food Chemistry 118: 836–839. doi:10.1016/j.foodchem.2009.05.032.
- Herraiz-Peñalver, D., M. Á. Cases, F. Varela, P. Navarrete, R. Sánchez-Vioque, and J. Usano-Alemany. 2013. “Chemical Characterization of Lavandula latifolia Medik. Essential Oil from Spanish Wild Populations.” Biochemical Systematics and Ecology 46: 59–68. doi:10.1016/j.bse.2012.09.018.
- Hervé, M. R., F. Nicolè, and K.-A. Lê Cao. 2018. “Multivariate Analysis of Multiple Datasets: A Practical Guide for Chemical Ecology.” Journal of Chemical Ecology 44: 215–234. doi:10.1007/s10886-018-0932-6.
- Jeon, J.-H., J.-Y. Yang, and H.-S. Lee. 2014. “Evaluation of the Acaricidal Toxicities of Camphor and Its Structural Analogues against House Dust Mites by the Impregnated Fabric Disc Method: House Dust Mites and Camphor Structural Analogues.” Pest Management Science 70: 1030–1032. doi:10.1002/ps.3769.
- Jiang, H., Z. Xie, H. J. Koo, S. P. Mclaughlin, B. N. Timmermann, and D. R. Gang. 2006. “Metabolic Profiling and Phylogenetic Analysis of Medicinal Zingiber Species: Tools for Authentication of Ginger (Zingiber officinale Rosc.).” Phytochemistry 67: 1673–1685. doi:10.1016/j.phytochem.2005.08.001.
- Jianu, C., G. Pop, A. T. Gruia, and F. G. Horhat. 2013. “Chemical Composition and Antimicrobial Activity of Essential Oils of Lavender (Lavandula angustifolia) and Lavandin (Lavandula x intermedia) Grown in Western Romania”.” International Journal of Agriculture and Biology 15: 772–776.
- Junker, R. R., J. Gershenzon, and S. B. Unsicker. 2011. “Floral Odor Bouquet Loses Its Ant Repellent Properties after Inhibition of Terpene Biosynthesis.” Journal of Chemical Ecology 37: 1323–1331. doi:10.1007/s10886-011-0043-0.
- Kampranis, S. C., D. Ioannidis, A. Purvis, W. Mahrez, E. Ninga, N. A. Katerelos, S. Anssour, et al. 2007. “Rational Conversion of Substrate and Product Specificity in a Salvia Monoterpene Synthase: Structural Insights into the Evolution of Terpene Synthase Function.” The Plant Cell 19: 1994–2005. doi:10.1105/tpc.106.047779.
- Kashima, Y., and M. Miyazawa. 2014. “Chemical Composition and Aroma Evaluation of Essential Oils from Evolvulus alsinoides L.” Chemistry & Biodiversity 11: 396–407. doi:10.1002/cbdv.201300234.
- Kaufmann, M., and M. Wink. 1994. “Molecular Systematics of the Nepetoideae (Family Labiatae): Phylogenetic Implications from rbcL Gene Sequences”.” Zeitschrift Für Naturforschung C 49: 635–645.
- Knudsen, J. T., R. Eriksson, J. Gershenzon, and B. Ståhl. 2006. “Diversity and Distribution of Floral Scent.” The Botanical Review 72: 1–120. doi:10.1663/0006-8101(2006)72[1:DADOFS]2.0.CO;2.
- Kokkalou, E. 1988. “The Constituents of the Essential Oil from Lavandula stoechas Growing Wild in Greece.” Planta Medica 54: 58–59. doi:10.1055/s-2006-962337.
- Landmann, C., B. Fink, M. Festner, M. Dregus, K.-H. Engel, and W. Schwab. 2007. “Cloning and Functional Characterization of Three Terpene Synthases from Lavender (Lavandula angustifolia).” Archives of Biochemistry and Biophysics 465: 417–429. doi:10.1016/j.abb.2007.06.011.
- Lis-Balchin, M., ed. 2002. Lavender: The Genus Lavandula. London: Taylor & Francis.
- Martén‐Rodríguez, S., C. B. Fenster, I. Agnarsson, L. E. Skog, and E. A. Zimmer. 2010. “Evolutionary Breakdown of Pollination Specialization in a Caribbean Plant Radiation.” New Phytologist 188: 403–417. doi:10.1111/j.1469-8137.2010.03330.x.
- Messaoud, C., H. Chograni, and M. Boussaid. 2012. “Chemical Composition and Antioxidant Activities of Essential Oils and Methanol Extracts of Three Wild Lavandula L. Species.” Natural Product Research 26: 1976–1984. doi:10.1080/14786419.2011.635343.
- Moja, S., Y. Guitton, F. Nicolè, L. Legendre, B. Pasquier, T. Upson, and F. Jullien. 2015. “Genome Size and Plastid trnK-matK Markers Give New Insights into the Evolutionary History of the Genus Lavandula L.” Plant Biosystems 150: 1–9.
- Muhlemann, J. K., A. Klempien, and N. Dudareva. 2014. “Floral Volatiles: From Biosynthesis to Function.” Plant, Cell & Environment 37: 1936–1949. doi:10.1111/pce.12314.
- Muñoz-Bertomeu, J., I. Arrillaga, and J. Segura. 2007. “Essential Oil Variation within and among Natural Populations of Lavandula latifolia and Its Relation to Their Ecological Areas.” Biochemical Systematics and Ecology 35: 479–488. doi:10.1016/j.bse.2007.03.006.
- Nabavi, S. M., A. Marchese, M. Izadi, V. Curti, M. Daglia, and S. F. Nabavi. 2015. “Plants Belonging to the Genus Thymus as Antibacterial Agents: From Farm to Pharmacy.” Food Chemistry 173: 339–347. doi:10.1016/j.foodchem.2014.10.042.
- Nicolè, F., Y. Guitton, E. Courtois, S. Moja, L. Legendre, and M. Hossaert-Mckey. 2012. “MSeasy: Unsupervised and Untargeted GC-MS Data Processing.” Bioinformatics 28: 2278–2280. doi:10.1093/bioinformatics/bts427.
- Padovan, A., A. Keszei, C. Külheim, and W. J. Foley. 2014. “The Evolution of Foliar Terpene Diversity in Myrtaceae.” Phytochemistry Reviews 13: 695–716. doi:10.1007/s11101-013-9331-3.
- Palá-Paúl, J., J. J. Brophy, R. J. Goldsack, and B. Fontaniella. 2004. “Analysis of the Volatile Components of Lavandula canariensis (L.) Mill., A Canary Islands Endemic Species, Growing in Australia.” Biochemical Systematics and Ecology 32: 55–62. doi:10.1016/S0305-1978(03)00177-7.
- Passalacqua, N. G., R. Tundis, and T. M. Upson. 2017. “A New Species of Lavandula Sect. Lavandula (Lamiaceae) and Review of Species Boundaries in Lavandula angustifolia.” Phytotaxa 292: 161–170. doi:10.11646/phytotaxa.292.2.
- Paton, A. J., D. Springate, S. Suddee, D. Otieno, R. J. Grayer, M. M. Harley, F. Willis, M. S. J. Simmonds, M. P. Powell, and V. Savolainen. 2004. “Phylogeny and Evolution of Basils and Allies (Ocimeae, Labiatae) Based on Three Plastid DNA Regions.” Molecular Phylogenetics and Evolution 31: 277–299. doi:10.1016/j.ympev.2003.08.002.
- Raguso, R. A. 2016. “More Lessons from Linalool: Insights Gained from a Ubiquitous Floral Volatile.” Current Opinion In Plant Biology 32: 31–36. doi:10.1016/j.pbi.2016.05.007.
- Raguso, R. A., B. O. Schlumpberger, R. L. Kaczorowski, and T. P. Holtsford. 2006. “Phylogenetic Fragrance Patterns in Nicotiana Sections Alatae and Suaveolentes.” Phytochemistry 67: 1931–1942. doi:10.1016/j.phytochem.2006.05.038.
- Raguso, R. A., and E. Pichersky. 1999. “A Day in the Life of A Linalool Molecule: Chemical Communication in A Plant-Pollinator System. Part 1: Linalool Biosynthesis in Flowering Plants.” Plant Species Biology 14: 95–120. doi:10.1046/j.1442-1984.1999.00014.x.
- Rustaiee, A. R., A. Yavari, V. Nazeri, M. Shokrpour, F. Sefidkon, and M. Rasouli. 2013. “Genetic Diversity and Chemical Polymorphism of Some Thymus Species.” Chemistry & Biodiversity 10: 1088–1098. doi:10.1002/cbdv.201200020.
- Sarker, L. S., M. Galata, Z. A. Demissie, and S. S. Mahmoud. 2012. “Molecular Cloning and Functional Characterization of Borneol Dehydrogenase from the Glandular Trichomes of Lavandula X Intermedia.” Archives of Biochemistry and Biophysics 528: 163–170. doi:10.1016/j.abb.2012.09.013.
- Schiestl, F. P. 2010. “The Evolution of Floral Scent and Insect Chemical Communication: Evolution of Floral Scent.” Ecology Letters 13: 643–656. doi:10.1111/j.1461-0248.2010.01451.x.
- Schiestl, F. P., and S. Dötterl. 2012. “The Evolution of Floral Scent and Olfactory Preferences in Pollinators: Coevolution or Pre‐Existing Bias?” Evolution 66: 2042–2055. doi:10.1111/j.1558-5646.2012.01593.x.
- Shellie, R., L. Mondello, P. Marriott, and G. Dugo. 2002. “Characterisation of Lavender Essential Oils by Using Gas Chromatography–Mass Spectrometry with Correlation of Linear Retention Indices and Comparison with Comprehensive Two-Dimensional Gas Chromatography.” Journal of Chromatography A 970: 225–234.
- Skoula, M., C. Abidi, and E. Kokkalou. 1996. “Essential Oil Variation of Lavandula stoechas L. ssp. stoechas Growing Wild in Crete (Greece).” Biochemical Systematics and Ecology 24: 255–260. doi:10.1016/0305-1978(96)00023-3.
- Steiner, K. E., R. Kaiser, and S. Dotterl. 2011. “Strong Phylogenetic Effects on Floral Scent Variation of Oil-Secreting Orchids in South Africa.” American Journal of Botany 98: 1663–1679. doi:10.3732/ajb.1100141.
- Thompson, J., A. Charpentier, G. Bouguet, F. Charmasson, S. Roset, B. Buatois, P. Vernet, and P.-H. Gouyon. 2013. “Evolution of a Genetic Polymorphism with Climate Change in a Mediterranean Landscape.” Proceedings of the National Academy of Sciences USA 110: 2893–2897. doi:10.1073/pnas.1215833110.
- Upson, T., S. Andrews, G. Harriott, C. King, and J. Langhorne. 2004. The Genus Lavandula. Portland, Oregon: Timber Press.
- Upson, T. M., J. R. Grayer, J. R. A. Greenham, C. Williams, F. Al-Ghamdi, and F.-H. Chen. 2000. “Leaf Flavonoids as Systematic Characters in the Genera Lavandula and Sabaudia.” Biochemical Systematics and Ecology 28: 991–1007.
- Wang, R.-L., C. Staehelin, S.-L. Peng, W.-T. Wang, X.-M. Xie, and H.-N. Lu. 2010. “Responses of Mikania micrantha, an Invasive Weed to Elevated CO2: Induction of β-Caryophyllene Synthase, Changes in Emission Capability and Allelopathic Potential of β-Caryophyllene.” Journal of Chemical Ecology 36: 1076–1082. doi:10.1007/s10886-010-9843-x.
- Waterman, P. G. 2007. “The Current Status of Chemical Systematics.” Phytochemistry 68: 2896–2903. doi:10.1016/j.phytochem.2007.06.029.
- Wink, M. 2003. “Evolution of Secondary Metabolites from an Ecological and Molecular Phylogenetic Perspective.” Phytochemistry 64: 3–19.
- Wise, M. L., T. J. Savage, E. Katahira, and R. Croteau. 1998. “Monoterpene Synthases from Common Sage (Salvia officinalis): CDNA Isolation, Characterization, and Functional Expression of (+)-Sabinene Synthase, 1,8-Cineole Synthase, and (+)-Bornyl Diphosphate Synthase.” Journal of Biological Chemistry 273: 14891–14899.
- Yi, C. G., T. T. Hieu, S. H. Lee, B.-R. Choi, M. Kwon, and Y.-J. Ahn. 2016. “Toxicity of Lavandula angustifolia Oil Constituents and Spray Formulations to Insecticide-Susceptible and Pyrethroid-Resistant Plutella xylostella and Its Endoparasitoid Cotesia glomerata: Lavender Oil Constituent Toxicity to P. xylostella and C. glomerata.” Pest Management Science 72: 1202–1210. doi:10.1002/ps.4098.
- Zhang, N., L. Tang, W. Hu, K. Wang, Y. Zhou, H. Li, C. Huang, C. Huang, J. Chun, and Z. Zhang. 2014. “Insecticidal, Fumigant, and Repellent Activities of Sweet Wormwood Oil and Its Individual Components against Red Imported Fire Ant Workers (Hymenoptera: Formicidae).” Journal of Insect Science 14: 241. doi:10.1093/jisesa/ieu122.
- Zuzarte, M., M. J. Gonçalves, C. Cavaleiro, A. M. Dinis, J. M. Canhoto, and L. R. Salgueiro. 2009. “Chemical Composition and Antifungal Activity of the Essential Oils of Lavandula pedunculata (Miller) Cav.” Chemistry & Biodiversity 6: 1283–1292. doi:10.1002/cbdv.v6:8.