ABSTRACT
This paper discusses the main steps leading to the acquisition, during the Palaeozoic, of the seed habit. The earliest spermatophytes originated in the Late Devonian and produced small cupulate ovules characterized by an elaborated sporangial apex involved in pollination. The diversification of ovules during the Carboniferous led to a wider range of sizes and shapes, and an increasing importance of the integument. The earliest seeds containing embryos are reported in Carboniferous coniferophytes.
Characterizing the seed habit of the spermatophytes
The seed habit is commonly viewed as combining four processes: heterospory (production of micro- and megaspores), monomegaspory (reduction to one functional megaspore per megasporangium), endospory (gametophyte development within the spore wall), and megasporangium integumentation. These processes evolved in two distinct lineages in the Palaeozoic, the isoetalean lycopsids and the spermatophytes. The former inhabited the equatorial late Carboniferous coal marshes. They were not seed plants and their seed-like type of habit did not free them from their aquatic needs for reproduction (Phillips Citation1979). Rather, they got extinct when the climate became dryer at the end of the late Carboniferous, about 300 million years ago. Actually, in addition to heterospory, monomegaspory, endospory and integumentation, the seed habit of the spermatophytes is characterized by a fifth process, pollination, which consists of delivering the pollen grain (i.e. the male gametophyte enclosed in the spore wall) to the female gametophyte retained on the parent plant (Haig and Westoby Citation1989).
Origin of the seed habit
The seed habit evolved in the Late Devonian, between −382 and −359 million years ago, about 100 million years after the conquest of the land by plants at the bryophytic grade. Ovules of the earliest seed plants are uncommon in fossil localities of early Late Devonian age (Frasnian, −382 to −372 Ma) but become numerous and diverse in late Late Devonian outcrops (Famennian, −372 to −359 Ma) (Prestianni and Gerrienne Citation2010).
Phylogenies, whether molecular or based on morphological characters and including both extant and extinct plants, resolve the spermatophytes as monophyletic (Gerrienne, Servais, and Vecoli Citation2016) (). The spermatophytes belong to the lignophytes, the group of plants characterized by a bifacial cambium. They evolved from a progymnosperm ancestor and most current phylogenies resolve them as closely related to the Archaeopteridales, a group of free-sporing (i.e. releasing spores from dehiscent sporangia) heterosporous trees that originated in the Middle Devonian and was widespread in the Late Devonian (). The earliest spermatophytes had small (i.e. generally less than 5 mm long), radially symmetrical, ovules produced terminally or subterminally on large fronds (). All Late Devonian ovules were borne in a cupule (). All had an hydrasperman morphology characterized by the development, at the distal end of the indehiscent megasporangium, of a hollow pollen chamber with a parenchymatous central column, and an elongated funnel-like structure, the salpinx (Taylor, Taylor, and Krings Citation2009) ( and ). This modification of the megasporangium (called nucellus in the spermatophytes) contributed to directing the pollen to the protected environment of the pollen chamber, next to the female gametophyte and its archegonia. Most Late Devonian ovules had an integument dissected into numerous, free or partially fused, segments (). Cupules were either uni- () or multi-ovulate (). Ovules detached from the parent plants and found isolated in the fossil deposits never contain embryos. This observation suggests that pollination and fertilization were separated in time, fertilization occurring after dispersal and embryos growing immediately after fertilization.
Figure 1. Simplified phylogenetic tree of Palaeozoic lignophytes (progymnosperms and spermatophytes). Stratigraphic ranges of extant taxa are in green. Hatched parts of the hydrasperman spermatophytes stratigraphic range are debatable.
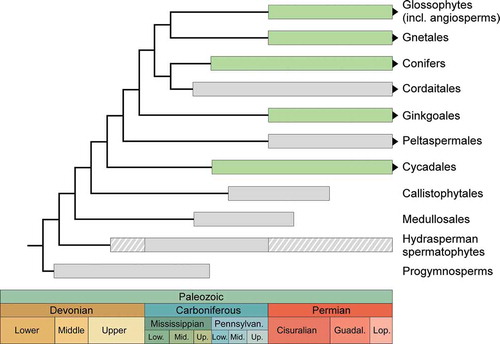
Figure 2. (a): Origin of the seed habit; hypothetical acquisition of characters indicated in white. (b): Hypothetical relationships between Runcaria, Coumiasperma and the spermatophytes; unknown characters in dotted lines.
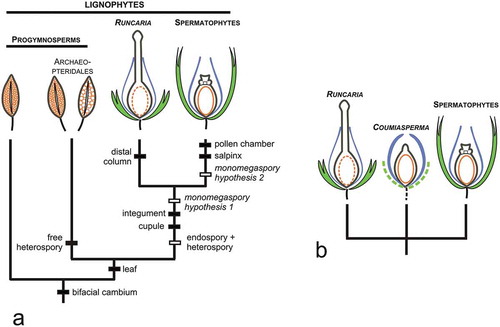
Figure 3. Diversity of ovules in the earliest spermatophytes from the Devonian of Belgium. (a): Runcaria heinzelinii Stockmans, isolated proto-ovule; (b-d), Moresnetia zalesskyi Stockmans; (b): distal part of the dichotomous seed-bearing system showing several cupules; (c,d): cupules with preserved ovules; (e): Pseudosporogonites bertrandii (Stockmans) Prestianni et al. showing its collaret cupule; (f): Dorinnotheca streelii Fairon-Demaret showing a putative collaret cupule; (g): two Condrusia rumex Stockmans specimens showing their cupule consisting of two leaf-like segments closely adpressed against each other. C = cupule; I = integument; N = nucellus. Scale bars in a, c-f: 1 mm; in b: 5 mm; in g: 2 mm.
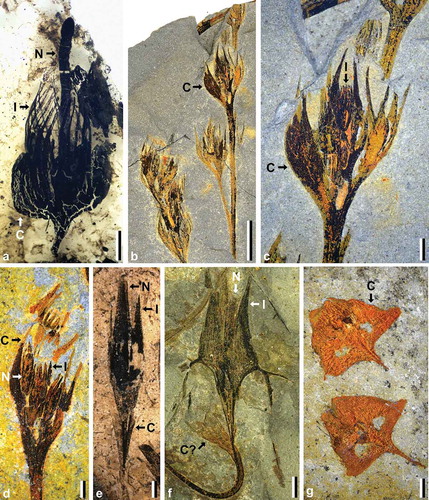
There are marked morphological differences between the naked sporangium of a seed-free lignophyte releasing spores at maturity like that of the progymnosperms and the cupulate ovule of a hydrasperman spermatophyte with its enclosed megagametophyte (). With few known morphological intermediates in the fossil record, the origin of the seed habit remains speculative (Taylor, Taylor, and Krings Citation2009).
Regarding spores, the earliest isolated palynomorphs interpreted as megaspores are specimens exceeding 200 µm in diameter found in sediments of late Early Devonian age (Emsian, about 400 Ma; Chaloner Citation1967). Free megaspores were numerous and diverse in the Middle Devonian; their abundance and diversity decreased in the Late Devonian, in parallel with the advent of the earliest seed plants. This observation led to the long-held hypothesis of a progressive evolution from homospory to heterospory, followed by endospory, and then the seed habit in the Famennian. This hypothesis was strongly supported by the Middle Devonian Spermasporites regarded by Marshall and Hemsley (Citation2003) as a heterosporous reproductive structure at an intermediate stage between heterospory and endospory in this sequence. Spermasporites is a megaspore tetrad consisting of a large megaspore and three smaller, presumably abortive spores, topped by a mass of microspores, and attached to fragments of a broken sporangial envelope. The wall ultrastructure of the functional megaspore was interpreted as being of the progymnosperm-spermatophyte type (Marshall and Hemsley Citation2003). On the other hand, because heterospory is associated with endospory in most extant spore-producing plants, DiMichele, Davis, and Olmstead (Citation1989) proposed an alternative scenario whereby heterospory resulted from endospory in the seed plant lineage. In a process leading to the precocious development of the gametophytes inside the sporangia, their metabolic environment within the sporophyte determined their sexual fate. According to this scenario, heterosporous plants with endosporic gametophytes in the seed-plant lineage would have evolved directly from homosporous plants; free heterospory in the Archaeopteridales would have evolved independently ().
Unravelling the sequence of acquisition of the cupule, the integument and the elaborated nucellar apex (i.e. comprising a pollen chamber, a central column and a salpinx) of the hydrasperman ovules is also far from clear. However, the discovery of Runcaria, a radially symmetrical proto-ovule of late Middle Devonian age (Givetian, about 385 Ma), brings some light on possible transitional stages (Gerrienne et al. Citation2004). Runcaria is borne in a cupule and its size is in the range of the earliest ovules ( and ). Like the latter, it has a dissected integument consisting of separate segments surrounding an indehiscent sporangium. The sporangium content of Runcaria is unknown but its indehiscent character, indicating endospory, makes megaspory very likely. Monomegaspory, however, is uncertain and may have evolved either in the common ancestor of Runcaria and the spermatophytes, or only in the latter (). The morphology of Runcaria indicates that, together with endospory and heterospory, the evolution of the integument and the cupule was plesiomorphic (i.e. ancestral) for the Runcaria-seed plant complex. Several hypotheses have been proposed for the origin of the integument, including sporangial sterilization or modification of an axial branching system subtending the sporangium (Haig and Westoby Citation1989; Taylor, Taylor, and Krings Citation2009). On the other hand, and despite its widespread occurrence in hydrasperman spermatophytes, the origin of the cupule has not retained much attention. Actually, how the integument and the cupule originated and whether they preceded or followed the evolution of endospory and heterospory is currently unresolved.
Morphologically, the distal extension of the sporangium in Runcaria ( and (a)) differs markedly from the nucellar apex of the hydrasperman ovules. It consists of an unopened column with an enlarged apex rising well above the cupule and the integumentary segments. This shape suggests that it contributed to the capture of anemophilous spores (Gerrienne et al. Citation2004). How pollination and fertilization were actually achieved in Runcaria is highly conjectural. The same uncertainties about pollination and fertilization exist about Coumiasperma, an early Carboniferous ovule which may show another step in the evolution of the nucellar apex (Galtier and Rowe Citation1989). The distal extension of the sporangium in Coumiasperma consists of a reduced column of parenchyma overarched by the separate segments of the dissected integument (). This apex lacks all the attributes of a hydrasperman nucellar apex. It is unknown whether Coumiasperma was subtended by a cupule at a pre-dispersal stage.
Morphological and functional diversification of ovules/seeds in the Palaeozoic
In addition to the hydrasperman seed plants, the best studied and major groups of early spermatophytes in terms of abundance and taxonomic diversity include the Medullosales, the Callistophytales and the coniferophytes, a derived group comprising the Palaeozoic Cordaitales and the conifers (). The former bear their reproductive organs on large fronds while the coniferophytes bear cones. Early Cycadales have been reported in the late Carboniferous and early Ginkgoales in the Permian but they are poorly known.
Early seed plants showed a stepwise diversification of their female reproductive organs that first involved the structures surrounding the nucellus. At least 20 species of hydrasperman ovules of Famennian age are now recognized, mainly from northeastern USA, Belgium and China (Prestianni and Gerrienne Citation2010; Xue et al. Citation2018). They correspond to more than five morphotypes based on cupule morphology and dissection of the integument. Despite this diversity, Famennian spermatophytes are interpreted as rather uniform in growth habit and ecological preferences, i.e. as pioneer plants of modest stature colonizing shorelines and disturbed habitats (Rothwell and Scheckler Citation1988). However, reconsiderations of the phylogenetic affinities of woody axes of Famennian age suggest that early spermatophytes may also have included large trees (Decombeix, Meyer-Berthaud, and Galtier Citation2011).
Further steps in the diversification of the female reproductive organs and evolution of the seed habit include an increased range of ovule sizes and shapes, the loss of the cupule, an increased importance of the integument which became more complex, and a simplification of the nucellar apex (). Medullosalean ovules reached up to 10 cm in length, those in the Callistophytales and the coniferophytes acquired a bilateral symmetry.
Ovules in the Medullosales, and all other more derived groups of spermatophytes, were acupulate. Their integument, which was no longer dissected, completely enclosed the nucellus. It showed a wide range of features probably related to pollination, dispersal and antiherbivore defense. Whether these integuments were all homologous, and whether the cupule was actually lost or fused with the integument in some of these spermatophytes is unresolved (Haig and Westoby Citation1989; Endress Citation2011). A micropyle differentiated at the distal end of the integument became the structure that canalized the pollen grains to the pollen chamber (). Post-pollination sealing mechanisms that prevented extended exposure of the female gametophyte to pathogens or desiccation involved the closure of the nucellar apex by different means in the hydrasperman spermatophytes and the Medullosales (). It involved the closure of both the nucellar apex and the micropyle in the Callistophytales and the Cordaitales (), and the closure of the micropyle only in the conifers (Serbet and Rothwell Citation1995). The last step in the evolution of the seed habit in the early spermatophytes was achieved in the coniferophytes where the earliest seeds containing embryos have been recorded in Cordaitales and conifers of late Carboniferous-early Permian age (Stidd and Cosentino Citation1976; Mapes, Rothwell, and Haworth Citation1989) (). The ability of these plants to delay germination is viewed as an adaptation to the climatic changes and increase in dryness that occurred at the end of the Palaeozoic.
Pollen grains in early spermatophytes
If this paper is primarily devoted to the evolution of ovules and seeds in the early spermatophytes, a brief statement about the evolution of their pollen grains appears necessary (). Those found in the pollen chamber of hydrasperman ovules do not differ morphologically from the trilete microspores of the progymnosperms. The pollen grains of the Medullosales were very large (100–600 µm wide) and bilateral. Like the hydrasperman pollens, however, they retained a proximal suture through which they may have released flagellated gametes in the pollen chamber. A significant change occurred in the Callistophytales which produced small saccate pollen grains resembling those of the coniferophytes. They germinated through a distal furrow and formed branched pollen tubes similar to those of living cycads and Ginkgo (Rothwell Citation1972).
Perspectives
The current, yet incomplete and not fully consistent, understanding of the patterns and processes involved in the origin and early diversification of the seed habit emerged from three lines of evidence: palaeobotanical, developmental, and functional. Because the following decisive steps were acquired in groups that have no extant representatives, progresses in unravelling how the cupule, integument and elaborated nucellar apex of the basalmost spermatophytes were acquired will necessitate the discovery of additional fossils showing intermediate morphologies between progymnosperm sporangia and hydrasperman ovules. Such fossils should ideally be at a pre-dispersal state and still attached to the parent plant for a better inference of the structures homologous to the cupule and integument. Even though younger fossils should not be discarded, plants that will most probably provide information on the progymnosperm-basalmost spermatophyte transition should be preferably searched for in the Middle Devonian-Frasnian time interval.
Acknowledgments
This paper is a contribution to the “MARCON” project supported by the LabEx CEMEB.
Disclosure statement
No potential conflict of interest was reported by the authors.
Additional information
Funding
Notes on contributors
Brigitte Meyer-Berthaud
Brigitte Meyer-Berthaud is Research Director at the Centre National de la Recherche Scientifique (CNRS), in a laboratory dedicated to the modeling of plant architecture and vegetation in Montpellier, southern France. She is a palaeobotanist with a main interest on Devonian and Early Carboniferous tracheophytes. She is specialized in anatomically preserved plants and is currently involved in the study of floras from Australia and Morocco.Contribution: conceived and wrote the paper.
Philippe Gerrienne
Philippe Gerrienne holds a F.R.S.-FNRS Senior Research Associate position at the University of Liège. His main research interests focus on the evolution, the palaeobiology and the diversification of the earliest land plants.Contribution: provided critical feedback and made Figures 1 and 3.
Cyrille Prestianni
Cyrille Prestianni is research assistant at the Royal Belgian Institute of Natural Sciences. His research is supported by the BR/143/A3/COLDCASE Belspo grant. He is palaeobotanist. His main research interests focus on the Devonian/Carboniferous transition. He is specialized in compression plants and is currently involved in the study of floras from South Africa and Argentina.Contribution: provided critical feedback and photos for Figure 3.
References
- Chaloner, W. G. 1967. “Spores and Land-Plant Evolution.” Review of Palaeobotany and Palynology 1: 83–93. doi:10.1016/0034-6667(67)90112-1.
- Decombeix, A. L., B. Meyer-Berthaud, and J. Galtier. 2011. “Transitional Changes in Arborescent Lignophytes at the Devonian-Carboniferous Boundary.” Journal of the Geological Society 168: 547–557. doi:10.1144/0016-76492010-074.
- DiMichele, W. A., J. I. Davis, and R. G. Olmstead. 1989. “Origins of Heterospory and the Seed Habit: The Role of Heterochrony.” Taxon 38: 1–11. doi:10.2307/1220881.
- Endress, P. K. 2011. “Angiosperm Ovules: Diversity, Development, Evolution.” American Journal of Botany 107: 1465–1489.
- Galtier, J., and N. P. Rowe. 1989. “A Primitive Seed-Like Structure and Its Implications for Early Gymnosperm Evolution.” Nature 340: 225–227. doi:10.1038/340225a0.
- Gerrienne, P., B. Meyer-Berthaud, M. Fairon-Demaret, M. Streel, and P. Steemans. 2004. “Runcaria, a Middle Devonian Seed Plant Precursor.” Science 306: 856–858. doi:10.1126/science.1102491.
- Gerrienne, P., T. Servais, and M. Vecoli. 2016. “Plant Evolution and Terrestrialization during Palaeozoic Times—The Phylogenetic Context.” Review of Palaeobotany and Palynology 227: 4–18. doi:10.1016/j.revpalbo.2016.01.004.
- Haig, D., and M. Westoby. 1989. “Selective Forces in the Emergence of the Seed Habit.” Biological Journal of the Linnean Society 38: 215–238. doi:10.1111/bij.1989.38.issue-3.
- Mapes, G., G. W. Rothwell, and M. T. Haworth. 1989. “Evolution of Seed Dormancy.” Nature 337: 645–646. doi:10.1038/337645a0.
- Marshall, J. E. A., and A. R. Hemsley. 2003. “A Mid Devonian Seed-Megaspore from East Greenland and the Origin of the Seed Plants.” Palaeontology 46: 647–670. doi:10.1111/1475-4983.00314.
- Phillips, T. L. 1979. “Reproduction of Heterosporous Arborescent Lycopods in the Mississippian–Pennsylvanian of Euramerica.” Review of Palaeobotany and Palynology 27: 239–289. doi:10.1016/0034-6667(79)90014-9.
- Prestianni, C., and P. Gerrienne. 2010. “Early Seed Plant Radiation: An Ecological Hypothesis.” In The Terrestrialization Process: Modelling Complex Interactions at the Biosphere–Geosphere Interface, edited by M. Vecoli, G. Clément, and B. Meyer-Berthaud, 71–80. London: Geological Society.
- Rothwell, G. W. 1972. “Evidence of Pollen Tubes in Paleozoic Pteridosperms.” Science 175: 772–774. doi:10.1126/science.175.4023.772.
- Rothwell, G. W., and S. E. Scheckler. 1988. “Biology of Ancestral Gymnosperms.” In Origin and Evolution of Gymnosperms, edited by C. B. Beck, 85–134. New York: Columbia University Press.
- Serbet, R., and G. W. Rothwell. 1995. “Functional Morphology and Homologies of Gymnospermous Ovules: Evidence from a New Species of Stephanospermum (Medullosales).” Canadian Journal of Botany 73: 650–661. doi:10.1139/b95-069.
- Stidd, B. M., and K. Cosentino. 1976. “Nucellangium: Gametophytic Structure and Relationship to Cordaites.” Botanical Gazette 137: 242–249. doi:10.1086/336865.
- Taylor, T. N., E. L. Taylor, and M. Krings. 2009. Paleobotany. The Biology and Evolution of Fossil Plants, 1230. Burlington, MA: Elsevier/Academic Press.
- Xue, J., P. Huang, D. Wang, C. Xiong, L. Liu, and J. F. Basinger. 2018. “Silurian-Devonian Terrestrial Revolution in South China: Taxonomy, Diversity, and Character Evolution of Vascular Plants in a Paleogeographically Isolated, Low-Latitude Region.” Earth-Science Reviews 180: 92–125. doi:10.1016/j.earscirev.2018.03.004.