Abstract
Purpose: This article proposed and investigated the application of bonebed-malleus short process registration in minimally invasive cochlear implantation, and assessed the value of the proposed strategy for image-guided otologic surgery.
Materials and methods: Ten temporal bone specimens were marked with both shallow and deep targets in order to measure registration resolutions. Two registration methods were applied. Method A (a new registration method) consisted of bonebed-malleus short process registration, while method B (traditional registration method) comprised registration using four titanium screws attached at the mastoid surface. The target registration errors (TRE) were measured at both the shallow and deep fiducial targets. After registration achieved by method A, percutaneous cochlear drilling was conducted on the other eight temporal bone specimens in order to observe the deviation from the target point and entry point.
Results: Using method A, the error observed at the shallow fiducial markers of specimens was less than 1 mm, while the error observed at the deep fiducial markers was approximately equal to 1 mm. No significant difference was observed when results were compared with the application of method B. In eight studied cases using method A, drilling operations were successfully conducted. The deviations from the target point and the entry point were 0.84 ± 0.30 and 0.66 ± 0.51 mm, respectively.
Conclusions: Results of the present study indicated that the new registration method demonstrated identical accuracy when compared to traditional registration method, achieved less invasiveness. Thus, the proposed method might be a feasible registration method for image-guided otologic surgery due to its mild invasiveness and high accuracy.
Introduction
Cochlear implantation is currently the only effective means to treat severe to extremely severe deafness. In order to ensure patient safety during the implantation procedure, the routine operation includes mastoidectomy, which provides successive exposure of the facial nerve recess and the cochlear basal turn. The size of the open cavity in traditional cochlear implant surgery is significantly larger than that necessary for the passing of the electrode. This will result in significant patient trauma, particularly for children and the elderly. Additionally, traditional cochlear implant surgery requires enhanced surgical skills, and requires longer operation time and recovery time,[Citation1] which makes this technology difficult to popularize. Following the development of medical navigation and the concept of minimally invasive operations, minimally invasive cochlear implantation will reduce the necessary dependence on the experience level of the surgeon, decrease the operation and healing time, reduce the economic burden to patients, and achieve rapid, precise, minimally invasive results while providing enhanced fatigue resistance.
The key to minimally invasive image-guided cochlear implantation is to access the cochlea via a single drill-pass through the facial recess; many institutions have conducted related research to improve the safety and precision of this system. One proposed method, which was still undergoing clinical trials, required a locked drill trajectory via a reserved channel in a patient-customized microstereotactic frame.[Citation2–6] Another proposed method utilized a self-developed and constructed robot to drill along a specified path, by which high resolution surgery could be achieved.[Citation7,Citation8] Registration is an important aspect of surgical navigation resolution. However, due to the registration of the navigation system, the infliction of additional trauma cannot be ignored in the above two approaches.
In image-guided surgery, there are two common registration methods: one method is based on invasive fiducial markers, while the other one is based on non-invasive fiducial markers. In the invasive fiducial marker method, the fiducial markers are mounted to the bone mass using a bone anchor,[Citation9] typically comprised of titanium screws.[Citation10] Alternatively, the non-invasive fiducial marker method generally achieves lower accuracy, as it typically adopts the use of patches or surface profile sketching.
Surgical navigation is most commonly used in sinus and skull-based surgeries. Non-invasive registration is often applied to sinus surgery, due to its advantageous characteristics including small invasiveness and acceptable accuracy of 3–4 mm.[Citation11] Alternatively, invasive registration, the use of which may be life-threatening, is often adopted in skull-based surgical cases so as to achieve higher accuracy of 1-2 mm.[Citation12,Citation13] In otologic surgery, the invasive registration method is typically employed in order to meet the accuracy requirement of less than 1 mm. However, this approach requires the operation of a drill, necessitating the use of local anesthesia and increasing pains and infections, in addition to delayed wound healing. Otologic conditions are usually not life threatening; hence, invasive registration is not easily accepted by patients. Additionally, the typical patient undergoing percutaneous cochlear implantation is usually very young or elderly, both of which represent populations that are particularly susceptible to the aforementioned risks. Alternatively, the resolution of noninvasive registrations such as dental reference frameworks, laser profilometers and anatomical orientation [Citation9,Citation14] are typically unable to meet the operational requirements.
Thus, it is important to develop a method of registration with higher accuracy and minimal invasiveness. This study proposes a joint registration approach based on the bonebed-malleus short process. The proposed method requires the drilling of a bone hole only at the site of implantation; subsequent registration (with sub-millimeter resolution, which is comparable to the resolution achieved via the titanium screw method) can be achieved using the characteristics of the surrounding bone surface and malleus short process.
The second portion of the paper details the preparation of the cadaveric specimens as well as the planning, registration and measurements of accuracy. The third portion shows the study results. Finally, discussion and conclusions are provided.
Materials and methods
Cadaveric specimen preparation
The Institutional Review Board (IRB) approval was obtained from the Peking University Third Hospital Medicinal Ethics Committee. A total of 10 formalin-fixed adult cadaveric specimens were selected; two of which demonstrated temporal bone in combination with otitis media were excluded, resulting in the selection of 18 temporal bone cases for the experimental trials. Selection criteria for the specimens include the presence of a complete external auditory canal, tympanic membrane and ossicle structure. The bone surface was exposed prior to surgery. Next, a vertical hole with a depth of 1 mm was made in the conventional cochlear implant transplant bed area in the temporal region with a diamond drill 1 mm in diameter (NSK Volvere GX surgical drill, Japan, with a speed of 35,000 rpm).
The shallow and deep targets were identified in order to measure the registration resolution in the shallow and deep mastoid. Serving as the shallow fiducial markers, four titanium screws were anchored nonlinearly and irregularly on the mastoid surface (diameter 2.0 mm, length 6.0 mm, Xi’an China). Mastoidectomy was then performed in order to release the facial recess and expose the round window niche. Then, one titanium screw was anchored at the retrofacialis air cell and another at the bony canal of the lateral semicircular canal, followed by the fixation of a stainless prill in the round window niche. The three locations were used as the deep fiducial markers. The shallow and deep fiducial markers were used to make resolution measurements as shown in .
Bonebed-malleus short process registration
After establishment of the fiducial markers, high-resolution CT scans were performed on the temporal bone of the cadaveric specimens by a 64-slice spiral CT scan machine (SIEMENS/SOMATOM Definition Flash, Forchheim Germany), with a thickness of 0.6 mm, a pitch of 0.3 mm, and pixels of 0.412 mm. Mimics 10.01 image processing software (Materialise, Leuven, Belgium) was used to extract the three-dimensional data as an stereolithography (STL) file in Digital Imaging and Communications in Medicine (DICOM) data format, which was then input into the independent-development ENT navigation software system (copyright registration number: 2014SR050996) based on Visualization tool kit (VTK).
In the reconstructed three-dimensional CT image, the point clouds of the bone surface were collected on the sphere centered at the temporal bone hole with a certain radius (usually 10 mm) using VTK. The sphere should then wrap around the short process of the malleus, enabling all point clouds inside the sphere to be collected. The point clouds in the virtual registration space can be consequently generated via all incorporated point clouds.
In the cadaveric specimens, a sphere with identical diameter and center was also selected to collect point clouds with the probe of a three-dimensional photo-electric navigation positioning system (NDI, Ontario, Canada). While collecting the data, the probe tip remained in contact with the bone surface, so as to evenly and completely cover the round surface as much as possible. Therefore, the point clouds collected by the probe during the sliding process can be regarded as equivalent to the point clouds of the bone surface. The otoendoscope tip was then probed into the external auditory canal and placed in contact with the malleus short process, and the point clouds were gently collected. Care must be exercised during the procedure to avoid any damage to the tympanic membrane or disturbance to the ossicular chain. The point clouds described above were combined to generate the point clouds in the real space as shown in .
Figure 2. (a, b) Point clouds collected at the bone-bed in real space; (c) Point clouds collected at the transplant bone-bed in the virtual space; (d, e) Point clouds collected at the malleus short process using an otoendoscope. (f) Point clouds collected at the malleus short process of the ossicles using the collecting sphere in the virtual space.
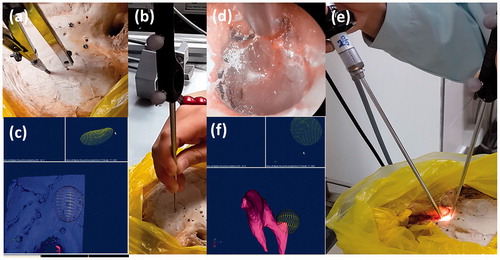
Principal component analysis (PCA) was first applied to the point clouds of both the virtual and real spaces to obtain the eigenvectors for primary registration. The iterative closest point (ICP) algorithm was then applied to perform the fine registration.[Citation15,Citation16] Eventually, the registration matrix can be derived. The method described previously was denoted as method A; method B achieves registration via four titanium screws attached at the mastoid surface. Both registration methods were applied to the same temporal bones.
Surgical simulation and target resolution measurement
Accuracy verifications of registration achieved by the two methods were conducted on the four shallow targets and three deep targets of each specimen, respectively. When in contact with the targets, the position coordinates of the probe tip were acquired via an NDI system, which was then converted to the virtual space by the registration algorithm. The error between the tip position and the target position in the virtual space can then be measured. The average value obtained from 50 continuous measurements was considered to represent the target registration error (TRE).
The drilling operation for the PCI surgery was performed at the temporal bone in other eight temporal bone cases, after registration of the navigation system was completed with the use of the joint registration of the bone bed-malleus short process (method A). The planned path was a line segment, which passed through the facial recess and was tangent to the basal turn of the cochlear. The target point of the line segment represented the most reasonable position for the cochleostomy, while the entry point intersected the surface of the temporal bone. The NDI system was used as a visual navigation system. The markers which can be identified by the NDI system were fixed onto the hand drill. The position coordinates of the drill bit were then converted to the virtual space. The motion direction of the drill bit was coincided with the line segments as far as possible. Deviation of the actual drilling path from the preoperative planned path was measured, as well as the deviation from the drill entry point to the target point. The distances between the drilling path and the facial nerve, tympanic cord nerve, posterior wall of the external meatus and ossicles were measured, respectively.
Statistical analysis was conducted using SPSS 18.0 software (SPSS Inc., Chicago, IL). Descriptive analysis was adopted to analyze the target registration errors (TREs) of two different registration methods. Quantitative results were expressed as the mean ± standard deviation, and the variance of repeated measurements was used to compare accuracy among the studied methods. A general linear regression model was applied to the shallow and deep targets, including the two registration methods as the repeated measurement factors and the targets as the fixed factors. Finally, results were adjusted according to the Bonferroni method, so as to explore the variability of the measured accuracy.
Results
shows the TREs of all targets. The TREs of the shallow targets were less than 1 mm, and no significant differences were observed between methods A and B. Deeper targets resulted in slightly lower registration resolutions for both methods A and B, while the resolution of method B demonstrated the greatest stability and accuracy in maintaining a registration of less than 1 mm. However, no significant difference was observed.
Table 1. TRE of the various registration methods.
Among the eight percutaneous temporal bone drilling operations conducted with navigation guidance, the drilling points at the cochleostomy demonstrated a deviation of 0.84 ± 0.30 mm, while the deviation of the entry points was equal to 0.66 ± 0.51 mm. shows all the comparative results before and after drilling, and shows the distances from the actual trajectory to the important structural elements.
Table 2. Comparison of results before and after drilling (n = 8).
Table 3. Distance from the actual trajectory to important structural elements (n = 8).
Discussion
The accuracy of the entire system is an important parameter in navigation surgery, which determines whether the system can be applied to the required surgery.[Citation17,Citation18] Alternatively, registration is the factor which most greatly affects the resolution of a system. With the development of computer-aided surgery and minimal invasion concepts, both accuracy and minimal invasion are taken into account. For example, the accuracy of registration using a combination of fiducial and anatomical markers is greater than that achieved by the use of fiducial markers alone. Staecker et al. [Citation19] proposed a registration method with a combination of lateral and malleus short process, which can improve the accuracy of temporal bone surgery to 0.9–1.5 mm. Surface template-assisted marker positioning (STAMP) was also proposed by Japanese researchers,[Citation20,Citation21] which could achieve smaller TREs (<1 mm near the cochlear implant point) in both simulations and actual surgeries, though this method required relatively greater exposure of the bone surface during the operation. Considering these aspects, a combination of an anatomical marker malleus short process and the morphological characteristics of the temporal bone surface were proposed by the current study, in order to obtain high registration accuracy and minimal trauma. The operations were illustrated as follows:
The bone hole at the center of the bone bed, which could be easily identified in both thin-section CT images of the temporal bone and also on the skull surface, was regarded as a paired fiducial marker.
Point collection in bone bed area was conducted after setting the bone bed center. Unique circles of a certain radius (10 mm in the current study) could be identified in both CT images and the bone bed, respectively. As the probe traveled along the circle, the probe trajectory was equivalent to the point clouds collected by surface plethysmography. Greater point cloud area indicated more collected information, and was often regarded as a paired reference surface. Since the probe was located on the bone surface, error induced by the deformation of skin and soft tissue was also avoided.
Point collection at malleus short process was conducted to define the direction of the point clouds. The malleus short process was a small area, which protrudes above the malleus handle; it was easily identified, as it was covered only by a thin layer of tympanic membrane approximately 0.1 mm thick. However, it was relatively difficult to confirm the exact location of the malleus short process according to two-dimensional CT scans. Therefore, in this study, ossicle STL data were utilized to identify the malleus short process and its point clouds were collected in the reconstructed three-dimensional image of the ossicles.
The proposed method represented a combination of three different registration methods: registration of bone-anchored paired fiducial markers, registration using surface profiles, and registration via anatomical landmarks. Comparatively, the proposed method demonstrates several advantages, including high precision and minimal trauma.
The bone bed was used in order to reduce the impact of physical trauma. Compared to other registration methods, which required at least three bone-anchored implants, the proposed method required the drilling of a single bone hole, which significantly reduced the associated trauma.[Citation4,Citation22,Citation23] The circular area required can be in the temporal bone or mastoid areas, which would be exposed during the surgery. Thus, selection of the bone surface for registration would not induce additional trauma.
The bone bed area utilized by the proposed method was large, which helped to accentuate its registration resolution as compared to the widespread titanium screw method. Subsequently, the deeper location of the malleus short process helped to improve the registration resolution due to decreased error in navigation in the deep temporal bone.
The current study utilized TRE to represent the resolution of the navigation system.[Citation24] Shallow targets were located at the body surface, while deep targets were located more closely to the critical area in otologic surgery. For instance, retrofacilis titanium screws and titanium screws at the lateral semicircular canal were adjacent to the facial nerve; therefore, they could measure accuracy around the facial nerve recess, while the stainless prill in the round window niche could measure accuracy around the cochlea and the drilling hole.
As shown in , the TRE at the mastoid surface can reach 0.5–0.6 mm using joint registration of the bonebed-malleus short process. As compared to the error obtained using the traditional titanium screws registration method, no statistical difference was observed. However, registration via titanium screws demonstrated higher stability in deep target measurement, the resolutions of which at the round window niche and facial recess were within 1 mm. While the TRE achieved by method A was approximately equal to 1 mm at the deeper targets. There was no significant difference between these two methods.
Comparison of TREs at different target locations demonstrated that the registration resolution declined with increasing depth of the target. The resolution achieved using method A on the surface is less than 1 mm, while the resolution near the round window niche reached 1.16 ± 0.46 mm. Two primary causes of this phenomenon might be explained as follows: (1) the small error generated by registration at the body surface might be amplified by increasing depth and rotational effects; and (2) the use of the prill (a spherical surface which may induce greater human error when defining the target position) as the fiducial marker to obtain TRE near the round window. Therefore, the results in this location were considered only as reference values.
The proposed method retained certain limitations. Currently, point clouds could only be obtained by invasive drilling in the bone bed center, which requires subsequent CT scans. Additionally, experienced surgeons were required to collect the point clouds at the malleus short process, so as to avoid influencing the ossicular chain. Another limitation of the current study was its small sample size. Target measurements were only obtained from 10 temporal bone specimens, and drilling measurements were only obtained from eight specimens. Hence, to remedy such limitations in future research, a proper radiocontrast agent must be determined for both CT scans and for liquid stain marks during surgery, in order to replace drill operation. More samples should be collected to further verify the validity of the proposed approach.
Conclusion
The joint registration of the bone bed-malleus short process method requires the drilling of a bone hole only in the temporal bone surface, which enables complete use of the bone surface of the bone bed regions for otological surgery and the malleus short process. This method can achieve higher registration accuracy with minimal invasiveness, and demonstrates promising application prospects for navigational surgery.
Acknowledgments
The authors thank Ying Liu with the Radiology team at Peking University Third Hospital. The authors also thank Nan Li for the statistical representation of the data.
Disclosure statement
The authors report no conflict of interest for this work.
Funding
This work was supported by the grant from The China Capital Health Development Project [Nos. 2011-4023-03 and 2016-2-4094].
References
- Semaan MT, Fredman ET, Shah JR, et al. Surgical duration of cochlear implantation in an academic university-based practice. Am J Otolaryngol 2013;34:382–387.
- Labadie RF, Chodhury P, Cetinkaya E, et al. Minimally invasive, image-guided, facial-recess approach to the middle ear: demonstration of the concept of percutaneous cochlear access in vitro. Otol Neurotol 2005;26:557–562.
- Labadie RFMD, PhD, Noble JHBS, et al. Clinical validation of percutaneous cochlear implant surgery: initial report. Laryngoscope 2008;118:1031–1039.
- Labadie RF, Balachandran R, Mitchell JE, et al. Clinical validation study of percutaneous cochlear access using patient-customized microstereotactic frames. Otol Neurotol 2010;31:94–99.
- Labadie RF, Balachandran R, Noble JH, et al. Minimally invasive image-guided cochlear implantation surgery: first report of clinical implementation. Laryngoscope 2014;124:1915–1922.
- Balachandran R, Reda FA, Noble JH, et al. Minimally invasive image-guided cochlear implantation for pediatric patients: clinical feasibility study. Otolaryngol Head Neck Surg 2014;150:631–637.
- Bell B, Stieger C, Gerber N, et al. A self-developed and constructed robot for minimally invasive cochlear implantation. Acta Otolaryngol 2012;132:355–360.
- Bell B, Gerber N, Williamson T, et al. In vitro accuracy evaluation of image-guided robot system for direct cochlear access. Otol Neurotol 2013;34:1284–1290.
- Metzger MC, Rafii A, Holhweg-Majert B, et al. Comparison of 4 registration strategies for computer-aided maxillofacial surgery. Otolaryngol Head Neck Surg 2007;137:93–99.
- Maurer Jr CR, Fitzpatrick JM, Wang MY, et al. Registration of head volume images using implantable fiducial markers. IEEE Trans Med Imaging 1997;16:447–462.
- Citardi MJ, Batra PS. Image-guided sinus surgery: current concepts and technology. Otolaryngol Clin North Am 2005;38:439–452.
- Brinker T, Arango G, Kaminsky J, et al. An experimental approach to image guided skull base surgery employing a microscope-based neuronavigation system. Acta Neurochir (Wien) 1998;140:883–889.
- Grayeli AB, Esquia-Medina G, Nguyen Y, et al. Use of anatomic or invasive markers in association with skin surface registration in image-guided surgery of the temporal bone. Acta Otolaryngol 2009;129:405–410.
- Luebbers HT, Messmer P, Obwegeser JA, et al. Comparison of different registration methods for surgical navigation in cranio-maxillofacial surgery. J Craniomaxillofac Surg 2008;36:109–116.
- Wang JC, Wang TM, Xu Y, et al. Registration method based on ICP algorithm for 3D surgical navigation. J Beijing Univ Aeronaut 2009;35:434–438.
- Xiang H, Wang CW, Wang GZ. Methods of three dimensional registration in the image guided surgery. Med J Air Force 2011;27:149–152.
- Kobler JP, Schoppe MG, Lexow J, et al. Temporal bone borehole accuracy for cochlear implantation influenced by drilling strategy: an in vitro study. Int J Comput Assist Radiol Surg 2014;9:1033–1043.
- Dillon NP, Balachandran R, Labadie RF. Accuracy of linear drilling in temporal bone using drill press system for minimally invasive cochlear implantation. Int J Comput Assist Radiol Surg 2016;11:483–493.
- Staecker H, O'malley BW, Eisenberg H, et al. Use of the LandmarXtrade mark surgical navigation system in lateral skull base and temporal bone surgery. Skull Base 2001;11:245–255.
- Matsumoto N, Hong J, Hashizume M, et al. A minimally invasive registration method using surface template-assisted marker positioning (STAMP) for image-guided otologic surgery. Otolaryngol Head Neck Surg 2009;140:96–102.
- Matsumoto N, Oka M, Cho B, et al. Cochlear implantation assisted by noninvasive image guidance. Otol Neurotol 2012;33:1333–1338.
- Warren FM, Balachandran R, Fitzpatrick JM, et al. Percutaneous cochlear access using bone-mounted, customized drill guides: demonstration of concept in vitro. Otol Neurotol 2007;28:325–329.
- Labadie RF, Mitchell J, Balachandran R, et al. Customized, rapid-production microstereotactic table for surgical targeting: description of concept and in vitro validation. Int J Comput Assist Radiol Surg 2009;4:273–280.
- Fitzpatrick JM, West JB, Maurer Jr CR. Predicting error in rigid-body point-based registration. IEEE Trans Med Imaging 1998;17:694–702.