Abstract
Background: The minimally invasive technique of closed intramedullary (IM) nailing fixation is currently considered the standard of treatment for the operative management of displaced traumatic fractures of the femur, with fracture reduction and repositioning being the first and most important step of the procedure. Skeletal-muscle traction and alignment of the fracture fragments are always performed as individual components of the reduction and repositioning phase of the procedure.
Methods: As use of high traction force and repositioning forces and torques can cause additional soft tissue injury, we developed a sensor-based system to monitor these forces and torques during the treatment of diaphyseal fractures of the femur, including the monitoring of traction forces during the entire procedure, from reduction to IM nail implantation and fixation.
Results: Based on a local coordinate system localized at the center of the fracture, maximum forces of 203 N along the medial-lateral (X) axis, 517 N along the anterior–posterior (Y) axis and 505 N along the shaft of the femur (Z-axis) were identified, with maximum torques of 16.4 Nm calculated around Y-axis and 38.3 Nm around X-axis. The pressure between the counteraction post and the perineum was also recorded, with magnitudes as high as 523 N being recorded. Excessive forces were identified and the difference in force–torque magnitudes during different stages of the reduction and fixation procedure were calculated.
Conclusion: The measurement system provides surgeons with real-time information which can assist them in performing effective repositioning of fracture fragments within safe margins of applied forces and torques.
1. Introduction
The femur, which is wrapped by powerful muscles and other soft tissues, is a tremendously strong bone. Therefore, fractures of the femoral shaft typically result from high-energy trauma, such as motor vehicle accidents or falls from a height. Low bone density and osteoporosis, which weaken bone, can also result in femoral fractures from lower energy trauma. Fractures of the femoral shaft are usually serious injuries, with the thigh musculature acting as a deforming force of the fracture. Currently, closed intramedullary (IM) nailing is the standard treatment for displaced fractures of the femur due to its advantage in preserving the anatomical structure of fracture sites and providing a mechanical environment which facilitates fracture healing.[Citation1] Reduction and repositioning of the fracture fragments is the most important component of the IM procedure.
Complete reduction performed by qualified and experienced surgeons reduces the need for repeated and forceful manipulations or distractions which may result in additional soft-tissue injury and associated difficulty in maintaining alignment at the fracture site.[Citation2] In addition, the use of a counteraction post against the perineum to provide a fulcrum to resist prolonged longitudinal traction applied to the thigh may result in nerve entrapment syndromes, presenting as numbness in the genital region.[Citation3,Citation4] Moreover, reduction, repositioning, and IM fixation is performed under radiographic monitoring resulting in high radiation exposure to both patients and medical staff. The need for surgical assistance to precisely maintain the alignment of fracture fragments by traction over a prolonged period of time must also be considered as an important risk factor for occupational injuries, including neck and low-back pain.
In view of the drawbacks associated with traditional reduction, repositioning and IM techniques, robot-assisted femoral shaft fracture reduction systems have been designed and their application in the operative theatre evaluated. These systems are currently limited by the absence of a generic set-up and application of robot-assisted systems due to a lack of understanding of reduction forces. Knowledge of minimal reduction forces and torques and of the counteracting force on the perineal post needed for different fracture types would be important in assisting surgeons in completing the reduction, repositioning and IM fixation efficiently, while reducing the risk of additional soft tissue injury.
The magnitude of fracture reduction forces used in practice have been evaluated in two studies. One of these studies [Citation5] evaluated reduction forces for femoral shaft fractures in seven polytraumatized patients. They reported that additional injury is likely to be sustained with implantation of the Schanz screws in the distal fracture fragment for reduction. Maeda et al. [Citation6] reported the maximum traction and repositioning force tolerated by healthy volunteers without a history of lower limb impairment or injury. While providing us with a general reference of maximal forces tolerated, the significant of these values to practice is limited as volunteers were not under anesthesia and the fracture repositioning forces were applied to the foot, which is not equivalent to real situations. Moreover, due to the strength of the thigh musculature, that contributes to significant displacement of the fracture fragments, skeletal-muscle traction forces that exceed the threshold of conscious tolerance will need to be applied to achieve a satisfactory reduction and repositioning of the fracture fragments.[Citation7]
In this paper, we describe a measurement system which we developed to measure the fracture reduction and repositioning forces and torques, as well as skeletal-muscle traction forces, to assist orthopedic surgeons in performing safe and effective reductions of displaced, traumatic, femoral shaft fractures. We provide a complete description of our system and of preliminary evaluation of the magnitudes of forces and torques measured intraoperatively for six tests. Our system was designed to adhere to the guiding rule of avoiding additional trauma to patients. We also discuss the integration of our measurement system into a robot-assisted system designed to perform typical repositioning procedures for displaced femoral shaft fractures.
2. Materials and methods
2.1. Apparatus for intraoperative measurement
Closed reduction of femoral shaft fractures may be complicated by misalignment, where the fracture fragments lie along non-uniplanar lines. A six-degree-of-freedom (6 DOF) measurement system is, therefore, needed to achieve anatomical alignment of the fracture fragments, including reduction of both linear and rotational displacements around a defined coordinate system local to the fracture. Application of skeletal traction is critical to counteract the muscle contraction forces which tend to pull fracture fragments apart and displace the reduction. The displacement of the fracture fragment can be quantified from lateral and anterior/posterior (AP) radiographic views (). Anatomical and cross-sectional imaging, which provides information on changes in cortical width resulting from circumferential rotation of the fracture fragments, is an effective method to assess and correct rotational component of the displacement during fracture reduction.[Citation8,Citation9]
The essential steps in performing closed reduction and fixation with IM nailing include: positioning the patient in a supine position on a traction table; connecting the patient’s foot to the limb repositioning device; obtaining computed tomography and radiographic images of the fracture site; and performing the fracture reduction, followed by implantation of screws, or nails, and fixation of the distal/proximal interlocking system. Therefore, skeletal-muscle traction and alignment of bone fragments are carried out as individual components in clinical practice.[Citation10]
We developed a device, consisting of three separate but inter-related components, to provide intraoperative measurement of applied three-dimensional forces and torques applied during fracture reduction and repositioning.[Citation11] The coordinate system for reduction was localized at the center of the fracture, with the Z-axis oriented along the femoral shaft, the X-axis along the medial-lateral direction, and the Y-axis upwards ().
Figure 2. Definition of the local coordinate system of the fracture and the experimental setup for our measurement device.
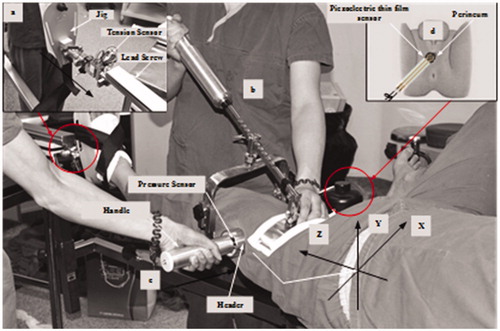
The measurement device was implemented on a traction table, with the capacity for skeletal traction against a perineal counteraction post, which is the common technique used for repositioning of femoral shaft fractures. The traction table was retrofitted to include a tension sensor between the jig and the lead screw to monitor skeletal-muscle traction forces over the entire closed reduction, repositioning and IM nailing fixation phases of the procedure (). Typically, as traction is performed, muscle contraction may partly reduce fracture fragments along directions other than the direction of traction. However, in many tests, displacement of the fracture fragments persists along the Y-axis, due to the tension of the gastrocnemius muscle and the weight of the lower limb, causing the distal fragment to fall in a position posterior to the proximal fragment.
Specific instruments and measurement systems, using sensor technology, have been developed to measure forces required to align the fracture fragments along the X- and Y-axes. Sterile tourniquet and either a commercially or home-made ‘F-tool’ are examples of two closed reduction techniques which apply the mechanical principle of levers to align the fragments of the femoral fracture.[Citation12] Accordingly, our measurement system includes two independent components, the ‘F-arm’ () for reduction of the fracture fragments along the Y-axis and ‘handgrip’ () for reduction of the fracture along the X-axis. The integrated system ‘F-arm’ includes the following components: a lever providing repositioning forces; two tension/pressure sensors transmitting voltage analog signals of the applied forces; and a flexible bracket to hold the distal fragment of the fracture fragment. The flexible bracket component can be assembled to simultaneously provide anterior/posterior alignment of the distal fragment relative to the proximal fragment. Eleven arc-shaped grooves were distributed equidistantly along the lever to adjust the fit of the jig for individual patients (). The adjustable frame is connected to the tension sensor by a bolt. The handgrip, which consists of a header, a pressure sensor and a handle, is positioned with one of its limb on either side of the fracture, with repositioning of the fragments completed by moving the handle to reduce the displacement.[Citation12] The F-arm measures the force applied along the Y-axis and the torque around the X-axis, Mx. Use of the two ‘handgrips’ allows the surgeon to apply force along the X-axis and the torque around the Y-axis, My, to correct the rotational alignment of the fracture. The torque about the X- and Y-axes (Mx and My) were computed by multiplying the force applied along the Y- and X-axis, respectively, by the corresponding distance between the point of force application and the center of the fracture site. Positioning of steel (radio-opaque) balls, the marrow cavity of the femur and localization points on the system itself were used as reference points to determine the distance between the contact point and fracture site. Moments around the Z-axis could not be calculated. Data were analyzed using Microsoft Visio 2013 (Microsoft, Redmond, WA).
Although complications associated with the use of a perineal counteraction post for reduction, such as pudendal and peroneal nerve injuries, have been well described, to our knowledge, the pressure between the counteraction post and the perineum has not been evaluated in previous research. Therefore, we included a thin and flexible piezoelectric film sensor in our device to measure these pressure values during the entire fracture reduction and repositioning procedure (). Pressure values were measured from the change in the sensor’s resistance with an applied force on the sensitive area of the sensor, with the measured resistance converted into a voltage signal for transmission.
2.2. Design of data acquisition system
For the acquisition of signals from the sensor array, which is the core module of our repositioning system, we used a distributed architecture which is widely adopted in target surveillance systems to ensure data reliability and quality.[Citation13] A distributed architecture provides several advantages including real-time monitoring and ease of maintenance through the integration of small subsystems, which lowers the overall cost of component replacement. The data acquisition module of our system used a series of C8051F Micro Controller Units interfaced with a personal computer. The proprietary hardware design, data information acquisition circuit, driver, and application software are described below.
The measurement system consisted of the following components: tension/pressure sensors; signal conditioning module; analog-to-digital (A/D) signal converter; a wireless RF module; and a personal computer (). Reduction forces were detected by sensors and the conditioning module converted the signal to appropriate voltage. Analog voltages were converted to digital signals by the A/D module and sampled (RF WLAN module, 10 Hz), with normalized signals sent to computer via serial communication for real-time curve creation of force information and storage for further off-line analysis. Data acquisition and management were controlled using custom-designed programs (Borland C++ builder), with data saved in text files for post-processing analysis. The data were analysed with the aim of specifying a surgeon’s behaviour.
2.3. Experimental methods
As previously stated, the impetus for our research was to develop a non-invasive system, using an array of sensors to measure the forces and torques applied during closed reduction of femoral fractures to assist surgeons in obtaining a clinically acceptable reduction without additional soft tissue injury. The Institutional Review Board of China approved the measurements in vivo of participants with femur shaft fractures in the research.
For the measurement of reduction and repositioning forces, the surgeon were asked to follow a standardized procedure, which included: (1) positioning the patient in a supine position on the traction table; (2) connecting the foot of the involved limb to the limb repositioning device; (3) performing skeletal traction to achieve reduction of the fracture fragments along the Z-axis; (4) lifting the lever of the ‘F-arm’ to obtain reduction of the fragment components along the Y-axis; and (5) moving the ‘handgrips’ to complete reduction of the fragment components along the X-axis. The surgeon were asked to only use sufficient force needed. All reductions were performed under radiographic monitoring ().[Citation5]
Femoral shaft fractures were classified using the AO/ASIF system, with typical diaphyseal femoral fractures (type A1-spiral, A2-oblique and A3-transverse) included in our study. Standard aseptic procedures were performed prior to using the measurement instrument. All intraoperative measurements were completed under general anesthesia, including injection of skeletal muscle relaxants prior to fracture reduction.
3. Results
No adverse events, associated with intraoperative measurement of reduction forces and torques, were identified. With assistance of the measurement system, six selected patients with traumatic fractures of femur shaft were involved from May 2014 to November 2015. Pressure between the counteraction post and the perineum were obtained in only two tests as this component was integrated into the device after the start of the study. Due to software issues, forces along the Z-axis were not recorded in one test. Magnitudes of fracture reduction forces and torques are reported for the six tests in . In all tests, the highest force values were recorded along the Z-axis due to the applied skeletal-muscle traction. The maximum alignment forces along the X-, Y- and Z-axes were 203 N, 517 N and 505 N, respectively. The maximum peak torques around the Y- and X-axes were 16.4 Nm and 38.3 Nm, respectively. The average force along the Y- and Z-axes was approximately 2.5 times greater than along the X-axes, due to influence of the weight of the lower limb segment (Y-axis) and tension of the thigh musculature (Z-axis), with the majority of the operative time dedicated to completing the fracture reduction along these two axes. A maximum pressure of 523 N was recorded between the counteraction post and the perineum.
Table 1. Maximal forces and torques measured during fracture reduction.
The traction force, which was recorded during the entire fracture reduction and closed IM nailing fixation procedure, was approximately divided into five stages, based on the procedure (): fracture repositioning; reaming of marrow cavity; implantation of Schanz screws; distal/proximal interlocking of the fixation screw; and so on.
A complete profile of the traction force measured during each of the five phases of the procedure is shown for three representative tests in . The forces are expressed as box-plot, where the central line represents the median force, the box represents the range between the 25th and the 75th percentiles of the force, and the interval lines represent the maximum and minimum values. Outlier (°) and extreme (*) traction force values were defined as values 1.5- and 3-fold greater than the range between the 25th and the 75th percentiles, respectively. The percentage of outliers for each stage of the reduction, repositioning and fixation procedure is reported in . Overall, 5.7% of values were deemed to be outliers, with the highest proportion being in the implantation of Schanz screws phase, indicative of the relative difficulty of this phase of the procedure. The patient in test 2 was treated with open reduction and plate fixation, with the percentage of outlier values being significantly lower than for the other two tests treated by closed IM nailing (p < .05, one-way ANOVA). The change in traction force between subsequent phases of the procedure is reported in , showing an average reduction in force of 22.4% from Phase 1 to Phase 5.
Figure 7. Traction force expressed as a function of the five stages of fracture reduction and fixation for three representative tests.
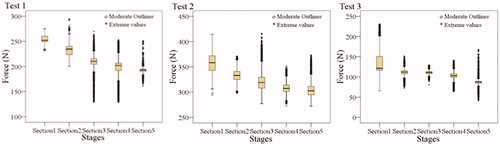
Table 2. Percentage of outlier values for each phase of the reduction and fixation procedure.
4. Discussion and future work
The counteracting force of skeletal muscle is the most important problem in obtaining and maintaining alignment of bone fragments of femoral shaft fractures. Avoiding undesirable manipulation forces, while obtaining an acceptable alignment, is important to prevent additional injury to soft tissues.[Citation14] In this study, we describe the design and preliminary clinical evaluation of an automated system developed to provide detailed information about the three-dimensional forces and moments applied by a surgeon during the reduction of diaphyseal fractures of the femur. Our system also monitors traction forces applied over the entire procedure, as well as pressure force between the counteraction post and the perineal region. Our methods are clinically meaningful in providing meta-cognitive information to surgeons regarding the forces and torques that are applied during closed reduction of the femoral shaft fractures to assist them in optimizing their technique.
Table 3. Percentage of force reduction for each phase of the reduction and fixation procedure.
Previous studies have reported on applied fracture reduction forces. Gösling et al. [Citation5] used a six-DOF sensor, rigidly fixed to an external fixator tube connected to the distal fragment through two Schanz screws, to collect and transmit reduction forces and torques. The opposite surface of the sensor was attached to a bar with two handles, used for alignment of the fracture fragments. Gösling et al.’s device was successfully used for intraoperative measurement of forces and torque for eight femoral fracture tests, with a maximum force of 396 N measured along the femoral shaft (Z-axis) and peak torque of 74 Nm around the related X-axis. The measured forces directly reflect the counteracting forces directly applied to align the distal fragment of the fracture fragment through two Schanz screws. Maeda et al. [Citation6] evaluated maximum tolerable forces applied to the lower limb of healthy volunteers without lower limbs injuries. Typical repositioning forces and torques were applied using a KSR-II system with forces and torques recorded during the mock manipulation procedure until volunteers self-reported that they could not tolerate a higher magnitude. The average maximum force attained were 232.9 N of skeletal traction, 7.7 Nm of internal rotation torque and 6.3 Nm of external rotation torque. Our data varied from the maximum values reported by Maeda et al. due to differences between the mock situation of Maeda et al. and our intraoperative methods.
The limitations of our own study are also important to acknowledge. Foremost, stability was not consistently provided as a manual repositioning technique was used. Rotation around the Z-axis (i.e. torsion) could not be measured during the reduction and fixation procedure in practice. Although we measured the force between the counteraction post and the perineum, this is a problem which requires further measurement and development of solutions. As well, we only measured reduction forces and torques with patients in a supine position on a traction table. Moreover, all patients received muscle relaxants prior to manipulation for fracture reduction. Patient positioning and the status of muscle relaxation will certainly influence magnitudes of applied reduction forces and torques. Although maximum magnitudes of forces and torques might vary, our device would be used in similar intraoperative circumstances and, therefore, our values provide a reference. An important feature of our device is the independent measurement of skeletal traction force and fracture fragment repositioning forces and torques, with both sets of data required to control a robot-assisted femoral fracture reduction system.
Currently in clinical practice, minimally invasive surgery for closed IM nailing is considered to be the standard technique for treatment of femoral shaft fractures. However, the technique is associated with several drawbacks, as previously stated, which include: high exposure to radiation during radiographic monitoring for both patient and medical staff, as well as exposure to repetitive and uncontrolled movements during manual reduction which increase the risk for additional soft tissue trauma.[Citation2,Citation15] Robot-assisted fracture reduction systems provide a viable alternative, providing sufficient and continuous repositioning forces. These systems are being developed on an ongoing basis, with a need to develop a unified standard for fracture repositioning. Extensive research and development in the area of robot-assisted systems for fracture reduction has been carried out by Westphal et al. [Citation16–18] These researchers developed a surgical manipulator for femoral fractures based on an industrial robot Stäubli (model RX 90). However, the end-effector of their robot-assisted system is rigidly fixed to the distal fracture fragment via Schanz screws, which causes additional injury to patients and increases the difficulty of IM nail implantation. Wang et al. [Citation19–21] developed a parallel robot for closed diaphyseal shaft fracture reduction based on the Stewart platform. The robot includes two ring plates, a mobile and a ground plate, connected by six linear actuators, with each actuator controlled via independent motors. The robotic system works as an external fixator. However, this system was perceived by patients as being intrusive, with patients reporting difficulty in performing simple activities of daily living when wearing the fixation platform.
In summary, we presented the design and application of a novel measurement system to monitor three-dimensional components of applied forces and torques during reduction, repositioning and fixation of femoral shaft fractures. Monitored forces and torques provide meta-cognitive feedback to surgeons to assist them in performing these procedures within safe limits to lower the risk for additional soft tissue injury. Future development will focus on enhancing monitoring of the force between the counteraction post and the perineum, including design innovation to lower the risk of injury to the perineal region. Future research is also needed to develop a robot-assisted fracture reduction systems based on these values.
Disclosure statement
The authors report that they have no conflicts of interest.
Funding
This research was supported by the Fundamental Research Funds for National Health and Family Planning Commission of PR China (W201307), and the Fundamental Research Funds for the Central Universities, the Graduate Student Scientific Research Innovation projects of General University of Jiangsu Province (No. KYLX_0101).
References
- Cleather D, Goodwin J, Bull A. Hip and knee joint loading during vertical jumping and push jerking. Clin Biomech (Bristol, Avon). 2013;28:98–103.
- Oszwald M, Ruan Z, Westphal R, et al. A rat model for evaluating physiological responses to femoral shaft fracture reduction using a surgical robot. J Orthop Res. 2008;26:1656–1659.
- Bressel E, Reeve T, Parker D, et al. Influence of bicycle seat pressure on compression of the perineum: a MRI analysis. J Biomech. 2007;40:198–202.
- Slikker W III, Van Thiel GS, Chahal J, et al. The use of double-loaded suture anchors for labral repair and capsular repair during hip arthroscopy. Arthrosc Tech. 2012;1:e213–e217.
- Gösling T, Westphal R, Faülstich J, et al. Forces and torques during fracture reduction: intraoperative measurements in the femur. J Orthop Res. 2006;24:333–338.
- Maeda Y, Tamura Y, Saito M, et al. Measurement of traction load and torque transferred to the lower extremity during simulated fracture reduction. In: Lemke HU, Inamura K, Doi K, et al., editors. 2005 International Congress and Exhibition on Computer Assisted Radiology and Surgery (CARS). Berlin, Germany: Elsevier Science BV; 2005. p. 674–679.
- Füchtmeier B, Egersdoerfer S, Mai R, et al. Reduction of femoral shaft fractures in vitro by a new developed reduction robot system ‘RepoRobo’. Injury. 2004;35:113–119.
- Langer JS, Gardner MJ, Ricci WM. The cortical step sign as a tool for assessing and correcting rotational deformity in femoral shaft fractures. J Orthop Trauma. 2010;24:82–88.
- Fang C, Gibson W, Lau T, et al. Important tips and numbers on using the cortical step and diameter difference sign in assessing femoral rotation – should we abandon the technique? Injury. 2015;46:1393–1399.
- Ye R, Chen Y, Yau W. A simple and novel hybrid robotic system for robot-assisted femur fracture reduction. Adv Robotics. 2012;26:83–104.
- Zhu Q, Sun X, Wang X, et al. Development of intraoperative noninvasive force measuring system during femoral fracture reduction. In: Proceedings of the 2015 IEEE International Symposium on Medical Measurements and Applications (MeMeA). Italy Turin: IEEE; 2015. p. 335–339.
- Riehl J, Widmaier J. Techniques of obtaining and maintaining reduction during nailing of femur fractures. Orthopedics. 2009;32:581–588.
- Rashid S, Qaisar S, Saeed H, et al. A method for distributed pipeline burst and leakage detection in wireless sensor networks using transform analysis. Int J Distrib Sens N. 2014;2014:1–14.
- Levy RM, Prince JM, Yang R, et al. Systemic inflammation and remote organ damage following bilateral femur fracture requires Toll-like receptor 4. Am J Physiol-Reg I. 2006;291:970–976.
- Dewey P, George S, Gray A. (i) Ionising radiation and orthopaedics. Curr Orthopaed. 2005;19:1–12.
- Westphal R, Winkelbach S, Gösling T, et al. A surgical telemanipulator for femur shaft fracture reduction. Int J Med Robot Comp Assist Surg. 2006;2:238–250.
- Westphal R, Winkelbach S, Wahl F, et al. Robot assisted long bone fracture reduction. Int J Robotics Res. 2009;28:1259–1278.
- Oszwald M, Westphal R, Bredow J, et al. Robot-assisted fracture reduction using three-dimensional intraoperative fracture visualization: an experimental study on human cadaver femora. J Orthop Res. 2010;28:1240–1244.
- Wang T, Li C, Hu L, et al. A removable hybrid robot system for long bone fracture reduction. Bio-Med Mater Eng. 2014;24:501–509.
- Du H, Hu L, Li C, et al. Advancing computer‐assisted orthopaedic surgery using a hexapod device for closed diaphyseal fracture reduction. Int J Med Robotics Comput Assist Surg. 2015;11:348–359.
- Li C, Wang T, Hu L, et al. A visual servo-based teleoperation robot system for closed diaphyseal fracture reduction. J Eng Med. 2015;229:629–637.