Abstract
Purpose: Detection of optic canal fractures is often difficult because of the subtleness of the fracture. If we could clarify impact on which region around the orbit is likely to accompany the fracture of the optic canal, the knowledge should be useful to make early diagnosis of optic canal fractures. The present study was conducted to elucidate this issue.
Methods: Ten finite element models were produced simulating the skulls of ten humans (8 males and 2 females; 43.8 ± 10.2 y/o). The peri-orbital area of each of the ten models was divided into eight regions in a clockwise fashion per 45 degrees. These regions were defined as Superior-Medial (0–45 degrees), Medial-Superior (45–90 degrees), Medial-Inferior (90 to 135 degrees), Inferior-Medial (135 to 180 degrees), Inferior-Lateral (180–225 degrees), Lateral-Inferior (225 to 270 degrees), Lateral-Superior (270–315 degrees), and Superior-Lateral regions (315–360 degrees), respectively. Dynamic simulation of applying traumatic energy on each of these regions was conducted. Resultant fracture patterns were evaluated using finite element analyses. Thereafter, frequencies of fracture involvement of the optic canal were evaluated for each of the eight regions.
Results: The involvement of the optic canal was most frequent for the Superior-Medial region (7/10), followed by the Medial-Superior region (5/10).
Conclusion: Optic canal fracture is likely to occur when the area between the supra-orbital notch and the medial canthus are strongly impacted. When evident fracture or serious damage of soft tissue is observed in this area, occurrence of optic canal fracture should be suspected.
Introduction
Fractures of the orbital region sometimes include the optic canal [Citation1,Citation2]. When fracture occurs on the optic canal, the optic nerve can develop edema, compressing itself against the canal, and degenerate, potentially leading to blindness. To prevent this serious complication, it is necessary to decompress the nerve by administering steroids or by surgical decompression of the optic canal [Citation3–6]. Early detection of the fracture is required to perform preventive measures effectively.
On the other hand, detection of an optic canal fracture is often challenging, since it usually appears as a minor slit, which is too subtle to identify with computer tomography.
Some might argue that damage to the optic canal and optic nerve can be diagnosed by examining visual function. It is surely true that presence/absence of optic nerve edema can be diagnosed by asking whether or not the patient can see―when the patient is conscious. However, patients with orbital fractures often present impaired thinking, or are unconscious due to brain injury, making queries about optic function of little value [Citation7].
Then, how can the fracture of the optic canal be detected under such conditions? We hypothesized that if we could clarify the regions that are likely to result in optic canal fracture when struck, this would be helpful for diagnosis. When peri-orbital regions are struck, the impact is transmitted through the orbital wall and reaches the optic canal, potentially causing fracture there [Citation8,Citation9]. The severity of impact transmission should differ depending on the transmission route, because each wall of the orbit presents specific anatomical structures. Some parts of the orbital walls include fissures or foramens, while others don’t [Citation10]; some walls neighbor the paranasal sinuses, while others don’t. Furthermore, the bone presents different thicknesses between walls [Citation11]. Therefore, the portion of an impact transmitted from the peri-orbital region to the optic canal should differ. Accordingly, the likelihood of optic canal fracture should differ, depending on which peri-orbital region is struck.
The present study clarifies the peri-orbital regions that are likely to result in optic canal fracture when struck.
Materials and methods
1. Model production
Ten finite element models of the orbit were produced in reference to DICOM (Digital Image and Communication in Medicine) data obtained by computed tomography of ten persons (8 males and 2 females; 43.8 ± 10.2 y/o). None of the ten persons had congenital or acquired deformity of the skull. The computer tomography was performed to screen intra-cranial damages after traumatic incidents. Informed consent was obtained with each person for his skull data to be used in this study, and the study was conducted in keeping with the Helsinki Declaration. The DICOM data was processed into finite element models by Simpleware (Simpleware Inc, California, USA) ―preprocessor software developed for graphic imaging and transformation of DICOM data into mathematical models. The left orbit part of the skull was taken and transformed into finite element models. The orbital part was cut out at 2.5 centimeters above the superior rim of the orbit, 4 centimeters lower than the inferior rim of the orbit, and 1 centimeter over the midline. Young’s modulus of the bone was calculated based on Kopperdahl’s methods [Citation12], referring to computer tomographic densities. Soft tissues―the skin, muscle, fat tissue, and components of the orbit―were simulated with a rubber material with a density of 10-9 ton/mm3, shear modulus of 0.7 MPa, and Young’s modulus of 2.05 MPa. Each model consisted of from 195000 to 220000 elements ().
2. Dynamic simulation
Dynamic simulation of applying impact to the peri-orbital region was conducted with the three finite element models. A circular-coordinates system was defined in the coronal plane, centering on the optic canal. The peri-orbital area was divided into eight regions of 45 degrees, clockwise (). The angles in the parentheses are defined with the perpendicular axis at 0 degrees. The eight regions were named as follows.
Figure 2. Each of the eight regions was assumed to be impacted with a 400-gram brass weight dropped from a height of 1.2 meters.
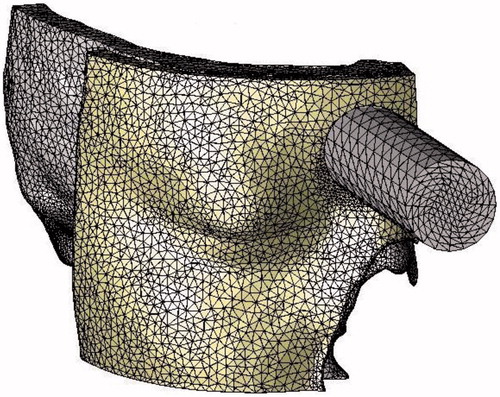
Superior-Medial region (0–45 degrees)Medial-Superior region (45–90 degrees)Medial-Inferior region (90–135 degrees)Inferior-Medial region (135–180 degrees)Inferior-Lateral region (180–225 degrees)Lateral-Inferior region (225–270 degrees)
Lateral-Superior region (270–315 degrees)Superior-Lateral region (315–360 degrees)
Ahmad states that orbital fracture occurs when a 400-gram weight is dropped on the orbit from a height of 1.2 meters (Ahmad et al., 2003). Traumatic energy was applied to each of the eight regions, simulating this condition (). The impact time was set to be 0.0002 seconds. The bone breakdown threshold was set as 0.00015.
Figure 3. For each of the eight regions, the impactor was applied to that the edge of the impactor comes at the orbital rim of each region. The round regions indicate the regions where the impactor was applied for the S-M (Superior-Medial), M-S (Medial-Superior), M-I (Medial-Inferior), I-M (Inferior-Medial), I-L (Inferior-Lateral), L-I (Lateral-Inferior), L-S (Lateral-Superior), S-L (Superior-Lateral) regions.
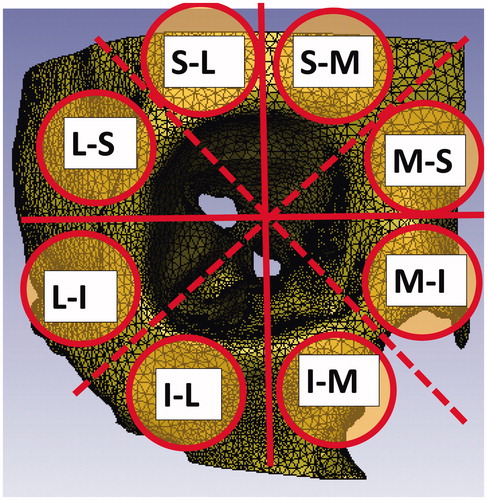
Dynamic calculation was performed under these conditions with LS-DYNA (Livermore Software Technology Corporation, California, USA), and fracture patterns resulting from the impact were calculated. Transient analyses were used in the calculation.
3. Evaluation
The ratio of presence of optic canal fracture was calculated for each of the eight regions for the results of the ten models. Thereby, peri-orbital regions likely to result in optic canal fracture when struck were identified.
Results
Calculations were possible for all of the 80 sets of analysis (10 analysis sets for the eight regions of each of the ten models). Examples of analysis results are demonstrated in (Superior-Medial, Medial-Superior, Medial-Inferior, Inferior-Medial regions) and (Inferior-Lateral, Lateral-Inferior, Lateral-Superior, Superior-Lateral regions).
Figure 4. Examples of fracture patterns resulting from impact on the S-M (Superior-Medial), M-S (Medial-Superior), M-I (Medial-Inferior), and I-M (Inferior-Medial) regions.
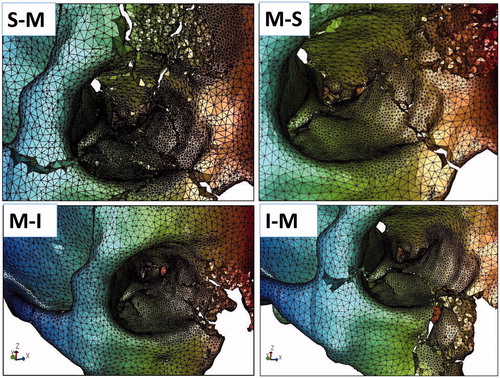
Figure 5. Examples of fracture patterns resulting from impact on the I-L (Inferior-Lateral), L-I (Lateral-Inferior), L-S (Lateral-Superior), and S-L (Superior-Lateral) regions.
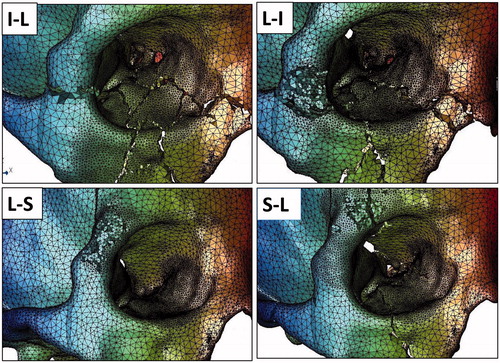
The ratios of optic canal fracture for the eight regions are shown in . Optic canal fracture occurred most often in impacts on the Superior-Medial region, followed by impacts on the Medial-Superior region. These regions are located between the supra-orbital notch and medial canthus.
Table 1. Frequency of optic canal fracture for each peri-orbital region.
Discussion
Background
Optic canal fractures need to be detected as early as possible, because they can cause a serious complication―blindness. On the other hand, optic canal fractures often present themselves as subtle slits of the bone, which are difficult to identify with graphic screening. To overcome the difficulty in making diagnosis of optic canal fractures, we developed an idea of evaluating the optic canal damage by observing the orbit, rather than by directly observing the optic canal itself. If we could find a law that connects fracture patterns of peri-orbital regions and the involvement of the optic canal, the law should be useful in making diagnosis of optic canal fractures. Driven by this idea, we conducted the present study.
Methodology
The present study employed dynamic calculation as its study method. As a study method for facial bone fracture, actual application of impacts on cadavers’ orbits might be more convincing than dynamic calculation because reality is superior to simulation. Indeed, the mechanisms of orbital fracture used to be studied on cadavers in the past [Citation13,Citation14]. However, destruction of cadavers is ethically unacceptable nowadays.
On the other hand, computer simulation with the finite element method allows us to evaluate how facial bones break without actually breaking them. Finite element analyses are often used to clarify the mechanisms of facial bone fracture [Citation15–20]. A great advantage of computer simulation is that it allows us to arrange exact experimental conditions―including the direction and intensity of impact and the regions to be struck. For these reasons, we employed finite element analyses in the present study.
Clinical meanings of findings
The results demonstrate a tendency that the optic canal is likely to develop fracture when impact works on the upper medial periorbital regions (the regions defined as S-M and M-S regions in this study).
These findings are useful in making diagnosis of optic canal fractures by referring to the conditions of facial injury in emergency situations. For instance, laceration of the skin is observed on the medial part of the eyebrow in the clinical case given in the left picture of (dotted area). Besides the laceration, subdermal hemorrhage and severe edema are observed in the same region. Judging from these symptoms, it is surmised that this patient received serious impact on the upper-medial peri-orbital regions. So, based on the above-stated likelihood, it is surmised that the patient’s optic canal might have damage.
Figure 6. (Left) Judging from the injury pattern of the soft tissue, it is surmised that the upper-medial peri-orbital region was strongly impacted (dotted area). Hence, injury of the optic canal should be suspected. (Right) Destruction of the upper-medial peri-orbital region implies that strong impact worked on this region (triangular arrows). So, optic canal fracture should be suspected.
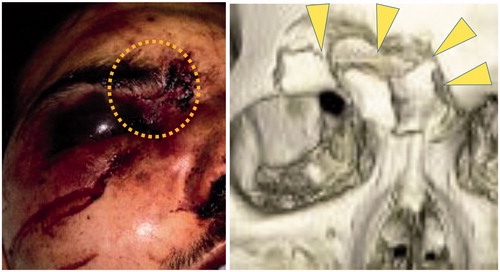
The above-stated rule is also useful in detecting the presence/absence of optic canal fracture when only low-resolution graphic images are available. For instance, the right picture of presents a computer-tomography image of a facial trauma case. Since the graphic data were obtained in an emergency situation, resolution is insufficient to identify subtle fractures. So, even if fracture exists on the optic canal, it might be overlooked. On the other hand, evident destruction of the bone is observed in the superior-medial regions of the peri-orbit (triangular arrows, implying strong impact was present on these regions. Hence, based on our results, it is speculated that the fracture might have involved the optic canal in this case. Therefore, re-screening of the fracture should be done to observe the optic canal more minutely, by such means as performing computer-tomography at a higher resolution level [Citation21]. At the same time, prophylactic administration of steroids should be done to prevent potential damage to the optic nerve.
Thus, the findings of the present study enable us to make early-stage diagnosis and prevent blindness.
Future studies
By using dynamic calculation, the present study theoretically elucidates the relationship between the risk of optic canal fracture and the location of traumatic impact on the peri-orbital region
In order to secure the reliability of the finding of this study―that impact on the upper-medial peri-orbital regions is likely to cause optic canal fracture―it is desirable to verify the theoretical conclusion in clinical studies. That is, if the conclusion is supported by statistical analyses of actual orbital fracture cases, its reliability would be more certain. As an advanced study, we’re planning to perform such clinical studies in a near future. However, since optic canal fracture is a relatively rare trauma, it is difficult to collect statistically significant numbers of clinical cases solely at our institute. It would be more desirable to conduct the study as a multi-center project. We solicit readers of this article to take part in this project.
Disclosure statement
No potential conflict of interest was reported by the author.
Additional information
Funding
References
- Choi KE, Lee J, Lee H, et al. The paradoxical predominance of medial wall injuries in blowout fracture. J Craniofac Surg. 2015;26:e752–e755.
- Almousa R, Amrith S, Mani AH, et al. Radiological signs of periorbital trauma - the Singapore experience. Orbit. 2010;29:307–312.
- Otani N, Wada K, Fujii K, et al. Usefulness of extradural optic nerve decompression via trans-superior orbital fissure approach for treatment of traumatic optic nerve injury: surgical procedures and techniques from experience with 8 consecutive patients. World Neurosurg. 2016;90:357–363.
- Vaitheeswaran K, Kaur P, Garg S. Minimal invasive transcaruncular optic canal decompression for traumatic optic neuropathy. Orbit. 2014;33:456–458.
- Yang QT, Zhang GH, Liu X, et al. The therapeutic efficacy of endoscopic optic nerve decompression and its effects on the prognoses of 96 cases of traumatic optic neuropathy. J Trauma Acute Care Surg. 2012;72:1350–1355.
- Zhilin G, Huoniu O, Zhihua C, et al. Wide optic nerve canal decompression for the treatment of blindness resulting from an indirect optic nerve injury. J Craniofac Surg. 2011;22:1463–1465.
- Yu-Wai-Man P. Traumatic optic neuropathy-clinical features and management issues. Taiwan J Ophthalmol. 2015;5:3–8.
- Matsuda Y, Sakaida H, Kobayashi M, et al. Successful application of endoscopic modified medial maxillectomy to orbital floor trapdoor fracture in a pediatric patient. Auris Nasus Larynx. 2016;43:575–578.
- Akiyama K, Karaki M, Hoshikawaa H, et al. Retrieval of ruptured medial rectus muscle with an endoscopic endonasal orbital approach. A case report and indication for surgical technique. Auris Nasus Larynx. 2015;42:241–244.
- Güler C, Uysal IÖ, Polat K, et al. Analysis of ethmoid roof and skull base with coronal section paranasal sinus computed tomography. J Craniofac Surg. 2012;23:1460–1464.
- Lethaus B, Weigl S, Kloss-Brandstätter A, et al. Looking for landmarks in medial orbital trauma surgery. Int J Oral Maxillofac Surg. 2013;42:209–213.
- Kopperdahl DL, Morgan EF, Keaveny TM. Quantitative computed tomography estimates of the mechanical properties of human vertebral trabecular bone. J Orthop Res. 2002;20:801–805.
- Ahmad F, Kirkpatrick WN, Lyne J, et al. Strain gauge biomechanical evaluation of forces in orbital floor fractures. Br J Plast Surg. 2003;56:3–9.
- Ahmad F, Kirkpatrick NA, Lyne J, et al. Buckling and hydraulic mechanisms in orbital blowout fractures: fact or fiction? J Craniofac Surg. 2006;17:438–441.
- Huempfner-Hierl H, Schaller A, Hierl T. Biomechanical investigation of the supraorbital arch - a transient FEA study on the impact of physical blows. Head Face Med. 2014;10:13.
- Huempfner-Hierl H, Bohne A, Wollny G, et al. Blunt forehead trauma and optic canal involvement: finite element analysis of anterior skull base and orbit on causes of vision impairment. Br J Ophthalmol. 2015;99:1430–1434.
- Nagasao T, Miyamoto J, Nagasao M, et al. The effect of striking angle on the buckling mechanism in blowout fracture. Plast Reconstr Surg. 2006;117:2373–2380.
- Nagasao T, Miyamoto J, Shimizu Y, et al. What happens between pure hydraulic and buckling mechanisms of blowout fractures? J Craniomaxillofac Surg. 2010;38:306–313.
- Nagasao T, Miyamoto J, Jiang H, et al. Interaction of hydraulic and buckling mechanisms in blowout fractures. Ann Plast Surg. 2010;64:471–476.
- Nagasao T, Miyamoto J, Tamaki T, et al. Inferior meatal antrostomy impairs dynamic stability of the orbital walls. Auris Nasus Larynx. 2009;36:431–437.
- Yan C, He B, Wang X, et al. Accuracy of high-resolution computed tomography in revealing fallopian canal fracture of patients with traumatic facial paralysis. Auris Nasus Larynx. 2015;42:374–376.