Abstract
Robotics in spinal surgery has significant potential benefits for both surgeons and patients, including reduced surgeon fatigue, improved screw accuracy, decreased radiation exposure, greater options for minimally invasive surgery, and less time required to train residents on techniques that can have steep learning curves. However, previous robotic systems have several drawbacks, which are addressed by the innovative ExcelsiusGPSTM robotic system. The robot is secured to the operating room floor, not the patient. It has a rigid external arm that facilitates direct transpedicular drilling and screw placement, without requiring K-wires. In addition, the ExcelsisuGPSTM has integrated neuronavigation, not present in other systems. It also has surveillance marker that immediately alerts the surgeon in the event of loss of registration, and a lateral force meter to alert the surgeon in the event of skiving. Here, we present the first spinal surgery performed with the assistance of this newly approved robot. The surgery was performed with excellent screw placement, minimal radiation exposure to the patient and surgeon, and the patient had a favorable outcome. We report the first operative case with the ExcelsisuGPSTM, and the first spine surgery utilizing real-time image-guided robotic assistance.
Introduction
Technological improvements, including real-time computer-assisted navigation, intraoperative 3D imaging, and surgical robotics have significantly advanced the field of spinal surgery [Citation1–3]. Such advancements benefit both surgeons and patients, including reduced surgeon fatigue, improved screw accuracy, decreased radiation exposure, greater options for minimally invasive surgery, and less time required to train residents on techniques that can have steep learning curves.
Several studies have demonstrated improved screw placement accuracy with the use of robotics in spinal surgery, compared to both conventional fluoroscopy-guided and navigation-guided screw placement [Citation4–6]. However, previous robotic systems have several drawbacks, including the requirement for the placement of K-wires, the potential for malregistration due to patient or interspinous clamp movement, or skiving of screw hole preparation tools [Citation2,Citation7]. Additional drawbacks are seen in systems that attach to the operative table or patient, which can be cumbersome, especially in obese patients.
FDA-approved for use in 2017, the ExcelsiusGPSTM (Globus Medical Inc. Audubon, PA, USA) robotic system addresses many of the drawbacks of previous robotic systems, incorporating imaging-agnostic registration, two-source tracking, real-time monitoring of navigation integrity, and a rigid end effector through which the surgeon can both drill and place screws. We performed the first spine surgery with the ExcelsiusGPSTM and describe the first operative case utilizing this novel system. In addition, this is the first reported spine surgery utilizing real-time image-guided robotic assistance.
Case illustration
Pre-operative presentation
A 69-year-old female presented to the neurosurgical spine service with 10 years of intractable lower back pain, as well as left lower buttock pain that radiated down the left leg into the dorsal surface of her left foot. Her past medical history was notable for a disk herniation 10 years prior, resulting in a foot drop that has since improved. Upon initial presentation, she noted one year of bladder incontinence, with inadequate urinary sphincter control, but no bowel symptoms. She also complained of gait unsteadiness and frequent falls. The patient failed multiple conservative therapies, including pain medications, injections, and physical therapy.
Imaging
MR imaging demonstrated severe lumbar stenosis at L4/L5, with an unstable Grade 2 spondylolisthesis at this level. Given her presentation and imaging findings, she was a good candidate for an L4-5 decompression with pedicle screw fixation, utilizing the robotic-guided system.
Surgical technique
After prepping and draping the patient in the usual sterile fashion, the patient reference tracking array (called the dynamic reference base, or DRB) was placed in the right posterior superior iliac spine (PSIS) via a small stab incision. A single “surveillance” marker on the end of a short pin was additionally placed in the left PSIS with another stab incision. The intraoperative CT reference frame (ICT) was attached to the DRB in a plane parallel to the floor and just above the patient’s skin. The camera stand, which tracks optical markers on the patient and compatible instruments, was detached and docked at the foot of the table. The DRB is securely placed in a rigid anatomic structure (i.e. PSIS) separate from the operative field, but in view of the robotic system’s detachable camera. Once registered, the DRB serves as an optical reference that may be readily identified by the camera—representing a standard anatomic landmark influenced by patient position. The location of this external landmark is utilized to triangulate instrument position relative to surgical anatomy, in real-time, relying on identifiable optical markers and registered patient imaging. Optical tracking is continuously updated with the location of the robotic arm, operative field, and compatible instruments. Robotic guidance and navigation with this system may be used in combination with preoperative CT, intraoperative CT, and intraoperative fluoroscopy. The O-arm (Medtronic Navigation, Inc., Louisville, CO) was then brought into the field, and a 3D cone beam CT scan was obtained including the surgical field of interest and the ICT. This image volume was transferred to the robotic guidance system for screw planning. On the workstation, the placement of each pedicle screw was planned, including trajectory, screw length, and diameter.
The reference frame (ICT) was then removed from the field. The navigated instruments, including a probe, drill, and navigated screw driver were verified for use with the system by checking tip accuracy. A typical open spine exposure was then performed, including subperiosteal dissection of the spinous processes, lamina, and exposure of facet joints at the appropriate levels. Pedicle screws were then placed prior to decompression. For screw placement, the robotic arm was draped sterilely, wheeled into the surgical field, and locked securely to the floor by deploying motorized pistons adjacent to the casters. The surgeon was able to directly manipulate the end effector, which is the rigid guide tube at the end of the robotic arm (), as well as the screen (which is draped sterilely) during the case.
Figure 1 Rigid arm with end effector and guide tube for direct transpedicular drilling, and screw insertion. Yellow star: End-effector; Red star: Instrument guide tube; Green star: Intraoperative CT (ICT) reference frame.
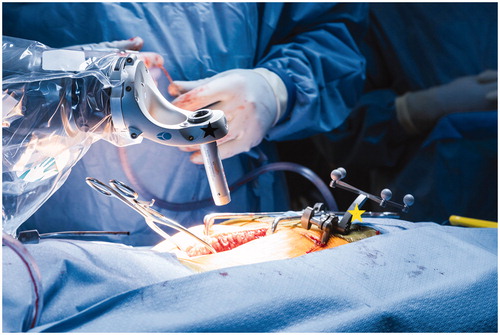
The surgeon utilized a foot pedal to bring the robotic arm (with the end effector and guide tube) to the desired position, corresponding to the screw trajectory planned. With the end effector in position, the surgeon first drilled and then placed the screw through the stable, rigid end effector, while maintaining tactile feedback. While placing the screw, the surgeon watched the robotic system monitor for real-time visualization of their trajectory and indication of excessive skive force (), which is manifested as increased lateral force sensed by the load cell located on the robotic arm. A color-coded information ring at the base of the station (which can blink green, yellow, or red) provided an additional safety measure.
After screw placement, the robot was removed from the surgical field. A second O-arm spin showed excellent screw placement. A standard lumbar decompression was then performed. Rods were placed, transverse processes and lamina were decorticated, and demineralized bone matrix and cancellous chips were placed to promote fusion. A routine, multi-layered spine closure was performed. The patient was exposed to 4.2 seconds of fluoroscopy throughout the case. Utilizing the fluoroscopy exposure per unit time, from the findings of Bindal et al. [Citation8], and the O-arm radiation exposure reported by Costa et al. [Citation9], the patient here was exposed to 8 mSv of radiation (2 O-arm spins +4.2 seconds of fluoroscopy) and the surgeon was exposed to 11 μSv—significantly less than the ICRP (International Commission on Radiological Protection) guidelines which limit clinician yearly radiation exposure to 20 mSv [Citation10].
Post-operative outcome
At the 2-week post-operative visit, the patient’s back pain and left lower extremity pain had completely resolved, with only mild residual numbness in her left leg. On physical exam, she was full strength in all muscle groups and was able to ambulate independently without any assistive devices. At her 6-week follow-up visit, she had no pain or numbness. She was walking daily and had discontinued all pain medication ().
Discussion
Robotic assistance has been successfully implemented in many surgical specialties, and has significant potential benefit in spinal surgery. Previous robotic systems have shown improved screw accuracy [Citation4–6,Citation11,Citation12], but have significant limitations that have hindered acceptance and widespread use [Citation7,Citation11,Citation13]. A recent systematic review of robotics in spine surgery summarized the most common causes of robotic failure from 12 studies—including registration failure, soft-tissue hindrance, and lateral drill guide skiving [Citation12].
In a prospective, randomized control trial comparing conventional free-hand lumbosacral screws to robot-assisted placement with the SpineAssist (MAZOR Robotics Inc®, Orlando, Florida), Ringel et al. [Citation7] actually found reduced screw accuracy in the robot-assistance group. The authors attributed this inaccuracy to instability with K-wire placement, bone mount movement, lateral docking of the drill sleeve, and skiving of the drill on bony surfaces with the SpineAssist robot. ExcelsiusGPSTM robotic system avoids drawbacks inherent to mounting references frames in the operative field and registration relative to the operating table.
A prospective case-matched study by Lonjon et al. [Citation11] showed greater accuracy in screw placement utilizing the ROSA robot (Medtech S.A., Montpellier, France): 97.3% with the robot, compared to 92% screw accuracy with the free-hand technique. The ROSA robotic arm is registered to the patient and moves independently: it is not rigid and still requires K-wires to be placed prior to pedicle screws.
Intraoperative imaging is certainly a useful tool to ensure safe instrumentation. However, its use in conjunction with a guidance arm can substantially reduce surgeon physical fatigue, and tremor. Notably, this technology may inform optimal surgical trajectory, from the skin surface, and allow for a less invasive operative field [Citation1,Citation2,Citation4–6]. This can be particularly helpful in the placement of technically challenging screws or those requiring a steep surgical trajectory; such as C1-C2 transarticular screws, C2 translaminar screws, L5-S1 transdiscal screws, iliac screws, anterior odontoid screws, or in those with anomalous anatomic structures (i.e. Klippel-Feil syndrome, axially rotated vertebrae, kyphosis, scoliosis) [Citation2,Citation4,Citation13–16]. Reduced tremor and a guidance arm may also be beneficial to surgical trainees for greater tactile appreciation of optimally placed screws [Citation2]. The rigid arm reduces the need for K-wires, susceptible to migration or fracture, and may cause devastating neurovascular injury if inappropriately advanced. Examples include great vessel injury when advanced beyond the anterior cortex of transpedicular instrumentation, or brainstem injury in the case of anterior odontoid screws [Citation17–20].
The ExcelsiusGPSTM robotic system addresses several drawbacks of prior systems. The robot is secured to the operating room floor, not the patient. It has a rigid external arm that facilitates direct transpedicular drilling and screw placement, without requiring K-wires. In addition, the ExcelsisuGPSTM has integrated neuronavigation, which is not included in the Mazor SpineAssist. Finally, the ExcelsiusGPSTM has a surveillance marker that immediately alerts the surgeon in the event of loss of registration, and a lateral force meter to alert the surgeon in the event of skiving.
Limitations of this study are inherent to the small sample. As such, it was not possible to prospectively collect patient reported outcomes or compile surgical details (i.e. device set-up time).
In summary, the ExcelsiusGPSTM robot has several advantages over prior spinal robots. Here, we present the first spinal surgery performed with the assistance of this newly approved robot, and the first spine surgery utilizing real-time image-guided robotic assistance. The surgery was performed with excellent screw placement, minimal radiation exposure to the patient and surgeon, and the patient had a favorable outcome.
Disclosure statement
Dr. Theodore is an inventor of a device manufactured by Globus Medical, Inc., which was used in the study discussed in this publication. He is entitled to royalty payments on future sales of the device and he is a paid consultant to Globus Medical, Inc. This arrangement has been reviewed and approved by the Johns Hopkins University in accordance with its conflict of interest policies. Neil Crawford currently owns stock, receives royalties and is employed by Globus Medical, Inc. Corinna C. Zygourakis, MD: received travel grants to attend resident education course from Globus, Nuvasive, Depuy, and Medtronic. She is supported by an AO Spine fellowship grant. Camilo A. Molina, MD: Consultant for Augmedics.
References
- Amiot LP, Lang K, Putzier M, et al. Comparative results between conventional and computer-assisted pedicle screw installation in the thoracic, lumbar, and sacral spine. Spine. 2000;25:606–614.
- Overley SC, Cho SK, Mehta AI, et al. Navigation and robotics in spinal surgery: where are we now? Neurosurgery. 2017;80:S86–S99.
- Schwarzenbach O, Berlemann U, Jost B, et al. Accuracy of computer-assisted pedicle screw placement. An in vivo computed tomography analysis. Spine. 1997;22:452–458.
- Kantelhardt SR, Martinez R, Baerwinkel S, Burger R, Giese A, Rohde V. Perioperative course and accuracy of screw positioning in conventional, open robotic-guided and percutaneous robotic-guided, pedicle screw placement. Eur Spine J. 2011;20(6):860–868.
- Roser F, Tatagiba M, Maier G. Spinal robotics: current applications and future perspectives. Neurosurgery. 2013;72(suppl 1):12–18.
- Schizas C, Thein E, Kwiatkowski B, et al. Pedicle screw insertion: robotic assistance versus conventional C-arm fluoroscopy. Acta Orthop Belg. 2012;78(2):240–245.
- Ringel F, Stuer C, Reinke A, et al. Accuracy of robot-assisted placement of lumbar and sacral pedicle screws: a prospective randomized comparison to conventional freehand screw implantation. Spine. 2012;37(8):E496–E501.
- Bindal RK, Glaze S, Ognoskie M, et al. Surgeon and patient radiation exposure in minimally invasive transforaminal lumbar interbody fusion. J Neurosurg Spine. 2008;9:570–576.
- Costa F, Tosi G, Attuati L, et al. Radiation exposure in spine surgery using an image-guided system based on intraoperative cone-beam computed tomography: analysis of 107 consecutive cases. J Neurosurg Spine. 2016;25:654–659.
- The 2007 recommendations of the International Commission on Radiologic protection. ICRP Publication 103. Ann ICRP. 2007;37:1–332.
- Lonjon N, Chan-Seng E, Costalat V, et al. Robot-assisted spine surgery: feasibility study through a prospective case-matched analysis. Eur Spine J. 2016;25(3):947–955.
- Joseph JR, Smith BW, Liu X, et al. Current applications of robotics in spine surgery: a systematic review of the literature. Neurosurg Focus. 2017;42:E2.
- Urakov TM, Chang KH, Burks SS, et al. Initial academic experience and learning curve with robotic spine instrumentation. Neurosurg Focus. 2017;42:E4.
- Collados-Maestre I, Lizaur-Utrilla A, Bas-Hermida T, et al. Transdiscal screw versus pedicle screw fixation for high-grade L5-S1 isthmic spondylolisthesis in patients younger than 60 years: a case-control study. Eur Spine J. 2016;25:1806–1812.
- Stokes IA. Axial rotation component of thoracic scoliosis. J Orthop Res. 1989;7:702–708.
- Wray S, Mimran R, Vadapalli S, et al. Pedicle screw placement in the lumbar spine: effect of trajectory and screw design on acute biomechanical purchase. J Neurosurg Spine. 2015;22:503–510.
- Scheer JK, Harvey MJ, Dahdaleh NS, et al. K-Wire fracture during minimally invasive transforaminal lumbar interbody fusion: Report of six cases and recommendations for avoidance and management. Surg Neurol Int. 2017;5:S520–S522.
- Furuhata R, Nishida M, Morishita M, et al. Migration of a Kirschner wire into the spinal cord: a case report and literature review. J Spinal Cord Med. 2018;15:1–4.
- Hussain NS, Perez-Cruet MJ. Complication management with minimally invasive spine procedures. Neurosurg Focus. 2011;31:E2.
- Mobbs RJ, Sivabalan P, Li J. Technique, challenges and indications for percutaneous pedicle screw fixation. J Clin Neurosci. 2011;18:741–749.