Abstract
IntroductionIn craniofacial surgery, magnetic navigation systems can effectively extend the doctor’s limited visual range, improve their surgical precision, shorten the operation time, and reduce the incidence of surgical complications. Owing to the ease of magnetic navigation, the accuracy of the magnetic navigation system is affected by various equipment in the operating room. Therefore, its large-scale application is lacking because the navigation accuracy requirement can be extremely high during craniofacial surgery. Therefore, the accuracy of magnetic navigation systems is crucial. Various surgical instruments have been evaluated to effectively reduce the interference of magnetic navigation systems with surgical instruments. In craniofacial surgery, magnetic navigation systems can effectively extend the doctor’s limited visual range, improve their surgical precision, shorten the operation time, and reduce the incidence of surgical complications. Owing to the ease of magnetic navigation, the accuracy of the magnetic navigation system is affected by various equipment in the operating room. Therefore, its large-scale application is lacking because the navigation accuracy requirement can be extremely high during craniofacial surgery. Therefore, the accuracy of magnetic navigation systems is crucial. Various surgical instruments have been evaluated to effectively reduce the interference of magnetic navigation systems with surgical instruments. In the surgical environment, the use of surgical instruments during mandibular surgery was simulated by selecting several conventional surgical instruments to record errors in the magnetic navigation system. The fluctuation values of the magnetic navigation errors were subsequently estimated and changes in its accuracy measured. MATLAB was used to calculate and analyze the fluctuations of the magnetic navigation errors. As results, the high-frequency electrosurgical system caused the greatest interference with the magnetic navigation system during surgery while powered on, with a maximum fluctuation error value of 1.8120 mm, and the maximum fluctuation error values of the stitch scissors, teeth forceps, and a needle holder were 1.3662, 1.3781, and 0.3912 mm, respectively. The closer the instrument is to the magnetic field generator or navigation target, the greater its impact. In conclusion, stitch scissors, teeth forceps, a needle holder, and the high-frequency electrosurgical system all affect magnetic navigation system accuracy. Therefore, it is necessary to avoid magnetic navigation system use and surgical instrument disturbances during surgery or select surgical instruments that do not interfere with the system. Surgical instruments must be evaluated for electromagnetic interference before they can be used in surgery with a magnetic navigation system.
Introduction
With the development of medical surgical robot technology [Citation1–3], a variety of navigation systems have been used for preoperative navigation and positioning in robotic surgery [Citation4]. The magnetic navigation system is the most widely used [Citation5]. Magnetic navigation positioning systems can replace traditional infrared navigation technology. Compared to traditional infrared navigation technology, the magnetic navigation system’s sensor size is reduced [Citation6], which saves unnecessary labor during its transport. Simultaneously, it is portable but has the disadvantage of being susceptible to stray electromagnetic field interference. In an operating room, a surgical device positioned near a magnetic navigation generator or stylus may cause inaccurate navigation and large measurement errors [Citation7]. The magnitude of the error observed by the doctor in the operating environment is highly variable and depends on the quality and elemental composition of the metallic objects in the surgical field and the frequency of the electromagnetic field [Citation8–10]. Different surgical instruments affect the magnetic navigation system differently. Although interference from stainless steel objects has been observed in laboratory environments [Citation11,Citation12], the relative error magnitudes caused among magnetic navigation systems by various metals that may be present in surgical instruments are unknown. In addition, no possible solution has been proposed or evaluated to resist the interference caused by foreign metal objects (such as various surgical instruments) in the clinical environment.
The interference with a magnetic navigation system involves two aspects: ferromagnetism and conductivity. Ferromagnetic interference from surgical instruments with a high iron content is directly proportional to the object’s mass [Citation7,Citation12]. Conductivity includes materials that conduct currents and generate magnetic fields. However, these materials can cause interference when non-conductive [Citation10]. The electromagnetic field emitted by the navigation system induces eddy currents in the conductive material and generates a secondary magnetic field [Citation12].
Commercial magnetic navigation systems aim to eliminate external interference by using a dedicated computer program that analyses the output of magnetic sensors and detects false signals. Once interference is detected, it tells the software not to use erroneous data. In fact, a computer program that controls the magnetic navigation system must be set to prevent the display of data only when electromagnetic interference exceeds a threshold. Below this threshold, the system ignores any interference; however, it can lead to measurement errors that cannot be detected by medical practitioners. Although the accuracy of the current magnetic navigation systems has been evaluated in several settings [Citation13], a few studies deliberately introduced sources of error and none directly evaluated the risk of incorrect measurements in the clinic. The present study evaluated the ability of four surgical instruments (needle holder, teeth forceps, stitch scissors, high-frequency electric system) to induce error and affect the accuracy of the magnetic navigation system. A surgeon who is aware of the impact of common surgical instruments on magnetic navigation system error and accuracy can avoid their simultaneous use during surgery, thereby improving magnetic navigation system accuracy.
The present study aimed to determine: (1) the error caused by a magnetic navigation system during surgery versus that caused by surgical instrument placement; and (2) the magnitudes of the error fluctuation and accuracy changed of the magnetic navigation system following the placement of such surgical instruments.
In 2010, Stevens et al. selected nine metals (6061 aluminum, 260 brass, 110 copper, cast cobalt chromium, 304 and 316 stainless steel, titanium, 1018 cold rolled steel, and A-36 hot-rolled steel) to reflect the interference of common metals with magnetic navigation systems [Citation14].
This study focused on the degree of interference of four common surgical instruments on a magnetic navigation system.
Clinical computed tomography data of the mandible were collected from a clinical patient, and the mandibular model was printed using a three-dimensional printer.
We used a LEGO-spliced 64 mm × 64 mm base plate to illustrate the positions of the surgical instruments relative to the magnetic navigation system generator. A frame was used to fix the mandibular position.
The experiment was conducted in a Stryker operating room (). The operating room layout was consistent with that of an actual operating environment. It was designed to simulate the effects of surgical instruments on the error value and accuracy of the magnetic navigation system of a surgical robot in a real surgical environment. The position of the navigation generator was (0, 0). The experimental equipment included a magnetic navigation system, mandible model, stitch scissors, teeth forceps, a needle holder, and a high-frequency electrosurgical system (VIO 300S; Erbe, Germany). An Aurora V3 System magnetic navigation system was used, and the surgical instruments (Qiangkang, China) were composed of ordinary 316 stainless steel.
Figure 1. Experimental platform: A: operating room; B: evaluating systematic error; C: evaluating fluctuations in error influenced by surgical instruments; D: real surgical environment.

The system was calibrated before the experiment to ensure the accuracy and authenticity of the experimental data. The model data were imported into the magnetic navigation system to evaluate systematic errors in the system.
Materials and methods
Five sets of experiments were conducted to explore the potential impact of the surgical instruments on the magnetic navigation system’s error and accuracy. In the first four sets of experiments, we placed stitch scissors, teeth forceps, a needle holder, and a high-frequency electric system in the range of 64 mm × 64 mm in front of the magnetic navigation system’s generator. The magnitude of the systematic error was estimated and eliminated. In the fifth set of experiments, we determined the magnitude of errors in the magnetic navigation system due to the simultaneous placement of stitch scissors, teeth forceps, and needle holders in the range of 64 mm × 64 mm near the magnetic navigation system’s generator. By recording the maximum and minimum error fluctuations at different positions, we aimed to determine the size and range of fluctuations in the error value of each surgical instrument. The systematic error values before and after the placement of each surgical instrument are listed in .
Table 1. System error values prior to placement of each surgical instrument.
All measurements were performed in an isolated, metal-free environment to minimize electromagnetic interference from uncontrolled sources. The model was installed on a fixed frame assembled using LEGO (mainly made of plastic particles of acrylonitrile butadiene styrene copolymer) without interfering with the magnetic navigation system. The average system error at the model installation position was 0.3827 mm.
General surgical instruments (stitch scissors, teeth forceps, a needle holder, and a powered high-frequency electric system) were introduced into a 64 mm × 64 mm surgical area to evaluate the influence of electromagnetic interference on navigation system accuracy. Surgical instruments were placed in 81 standard positions at 8-cm intervals. The coordinates of each position from the magnetic navigation generator are listed in , where 37 (0, 0) indicates the position of the magnetic navigation generator. For each surgical instrument, the maximum and minimum error values of fluctuations were recorded at 81 locations.
Table 2. The 81 standard positions and coordinate values.
The maximum and minimum error values of these measured values were compared to obtain the fluctuation of the error value at each position, and the measured values were compared with the systematic error determined in the initial experiment to determine magnetic navigation system accuracy changes. We determined the deviations in the magnetic navigation system error values caused by the presence or absence of surgical instruments at various locations and used MATLAB software to fit and plot the fluctuation values of the systematic error deviations caused by placing different surgical instruments at each location.
In the present study, MATLAB and DATAGRAPH software were used to process and analyze the measured data and construct a graph that reflects the fluctuations in the error values and accuracy changes of the magnetic navigation system.
Evaluation procedure: We used a LEGO substrate as the distance between each surgical instrument and the magnetic navigation generator to determine the specific position of the surgical instrument in the experimental plane. The dimensional accuracy of the experimental LEGO substrate was measured using a coordinate measuring machine. Measurements were performed to ensure the accuracy of the surgical instrument’s position relative to that of the magnetic navigation generator ().
Results
Interference of various surgical instruments on magnetic navigation system
Stitch scissors
In the first group of experiments, the stitch scissors were placed in a 64 mm × 64 mm experimental area and the error value of the system was measured at positions 1–81. Maximum and minimum error values were recorded for each position. The fluctuation value of the error at each position point was calculated, and the MATLAB software was used to program the interference map of the stitch scissors in the magnetic navigation system. As shown in , the stitch scissors were at the position 39 (0, 16). This position was disturbed by the magnetic navigation system and the maximum fluctuation value of the error was 1.3662 mm. Under these conditions, the positions of the stitch scissors from the magnetic navigation generator were as follows: x = 0 cm and y = 16 cm. From the above error value fluctuation chart, we deduced that the scissors exhibited significant interference with the magnetic navigation system within 20 cm from the magnetic navigation generator and that the 20–60 cm range exhibited little interference with the magnetic navigation system. Beyond the 60-cm range, the magnetic navigation system interference was negligible.
Teeth forceps
In the second set of experiments, the teeth forceps were placed into a 64 mm × 64 mm experimental area and the error values tested at the 1–81 positions. Maximum and minimum error values were recorded at each position. The error fluctuation at each position was estimated. Finally, MATLAB software was used to assess the interference map of the teeth forceps on the magnetic navigation system. As shown in , when the tooth forceps moved to 37 position (0, 0), the system exhibited the largest interference, and the maximum fluctuation value of the error was 1.3781 mm. The error value fluctuation chart demonstrated that the teeth forceps significantly interfered with the magnetic navigation system within 20 cm of the magnetic navigation generator. Thus, the system interference can be ignored.
Needle holder
In the third group of experiments, the needle holder was placed in a 64 mm × 64 mm experimental area, and the effect of the original error value of the system was assessed at positions 1–81. Maximum and minimum error values were recorded for each position. The fluctuation value of the error at each position point was calculated, and MATLAB software was used to program the interference map of the needle holder to the magnetic navigation system. As shown in , the needle holder was at position 38 (0, 8). The interference with the magnetic navigation system was the largest, and the maximum error fluctuation was 0.3912 mm. The needle holder of the magnetic navigation generator was positioned at x = 0 and y = 8 cm. From the error value fluctuation chart, it can be deduced that the needle holder exhibited a large interference with the magnetic navigation system within 20 cm from the magnetic navigation generator, and that a 20–60 cm range exhibited a small interference with the magnetic navigation system. Beyond 60 cm, the needle holder exhibited magnetic interference. The interference from the navigation system was negligible, which can be explained by the maximum range of magnetic navigation exceeding 60 cm from the magnetic navigation generator.
High-frequency electrosurgical system
In the fourth set of experiments, the high-frequency electrosurgical system was placed in a 64 × 64 mm experimental area, and the impact of the original error value was assessed at positions 1–81. The maximum and minimum error values at each position were calculated using the error fluctuation value at each position. MATLAB software was used to program the interference map of the stitch scissors in the magnetic navigation system. As shown in , when the stitch scissors were at position 28 (−8, 0), the maximum fluctuation of error was 1.8120 mm. The error value fluctuation chart showed that when the high-frequency electrosurgical system was powered on, the magnetic navigation system exhibited high interference in the range of 60 cm from its generator. As the y-axis value increases, the magnetic navigation system interference tends to decrease, showing symmetrical interference. This interference increases significantly when the high-frequency electrosurgical system is switched on. The interference is relatively small when no power is applied.
Simultaneous use of multiple surgical instruments
In the fifth group of experiments, multiple surgical instruments, including stitch scissors, teeth forceps, and a needle holder, were placed in a 64 mm × 64 mm experimental area, and the impact of the original error values on the system was tested by their placement at positions 1–81. The maximum and minimum error values were recorded at each position, and the fluctuation value of the error was calculated for each. Finally, MATLAB software was used to program the interference map of multiple surgical instruments on the magnetic navigation system (). The concomitant introduction of multiple surgical instruments into the experimental area caused high interference in the magnetic navigation system, and the maximum fluctuation of the error was estimated at 1.1 mm. As shown in the fluctuation chart, each surgical instrument exhibited high interference with the magnetic navigation system within 20 cm of its generator. A 20–60-cm range exhibited minimal interference with the magnetic navigation system. The interference from the magnetic navigation system was negligible since the navigation range was exceeded at a distance of >60 cm from the magnetic navigation generator.
In summary, here we drew a polyline wave chart of the degree of interference of four types of surgical instruments, namely stitch scissors, teeth forceps, needle holders, and a high-frequency electrosurgical system, at positions 1–81 (). The energized high-frequency electrosurgical system exhibited the highest interference with the magnetic navigation system. The stitch scissors, teeth forceps, needle holders, and high-frequency electrosurgical system interference peak points are represented as A, B, C, and D, respectively.
Figure 8. Comparison of interference degree of common surgical instruments on magnetic navigation system. A: Coordinates of peak point position of the stitch scissors (0, 16); B: Coordinates of peak point position of teeth forceps (0, 0); C: Coordinates of peak point position of the needle holder wave (0, 0); and D: Coordinates of the peak point position of the high-frequency electrosurgical system wave (16, 40).
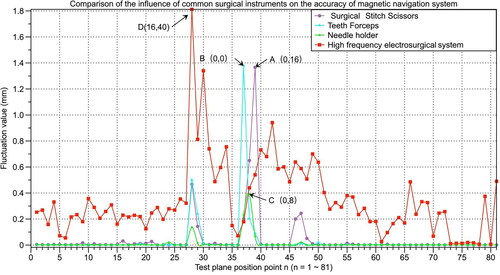
Discussion
The accuracy of magnetic navigation systems may significantly affect the outcomes of clinical surgery. For example, in cochlear implants, sub-millimeter-level navigation errors can lead to the incorrect placement of implanted electrodes [Citation15] or permanent damage to tissue structures such as the facial nerves [Citation16]; in craniofacial orthopedic surgery, the clinically required surgical error was 2 mm. Therefore, an error at the millimeter level could easily lead to surgical failure. For example, in mandibular angle osteotomy, the mandibular angles can be asymmetric, which may damage the facial nerve and cause facial paralysis. However, the navigation system provides clinical certification for robotic surgical navigation. The magnetic guidance system can reduce the inherent positioning uncertainty in the clinic and the error and uncertainty of the doctor’s visual judgment during surgery. Although the magnetic navigation system is calibrated and compensated prior to the operation, a craniomaxillofacial surgical navigation system cannot always meet the requirements for clinical navigation during surgery. In clinical surgery, it is impossible to estimate the interference of various surgical instruments using magnetic navigation systems. Thus, evaluating the interference of various surgical instruments on magnetic navigation systems, while studying and reducing the impact of commonly used surgical instruments during navigation surgery, can aid in the clinical application of robotic surgical navigation systems.
Conclusion
This study evaluated the influence of four commonly used surgical instruments on the accuracy of the magnetic navigation system (). Using five groups of experiments, specific values were obtained for the influence of the four surgical instruments on the accuracy of the magnetic navigation system. The initial accuracy of the magnetic navigation system was 0.48 mm. The maximum deviation in the magnetic navigation system error caused by the stitch scissors was 1.3662 mm. The average accuracy was 0.5107 mm, showing a decrease of 0.0307 mm. The largest deviation caused by the tooth forceps was 1.3781 mm, its average accuracy was 0.5001 mm, and its average decreased by 0.0201 mm. The maximum deviation of the magnetic navigation system error caused by the needle holder was 0.3912 mm, and the average accuracy was 0.4874 mm, which was reduced by 0.0074 mm. The maximum deviation in the magnetic navigation system error caused by the high-frequency electrosurgical system, which was the largest among the four instruments, was 1.8120 mm. The average accuracy was 0.6623 mm, a decrease of 0.1823 mm.
Figure 9. Effect of stitch scissors, teeth forceps, needle holder, and high-frequency electrosurgical system (HFES) on magnetic navigation system accuracy.
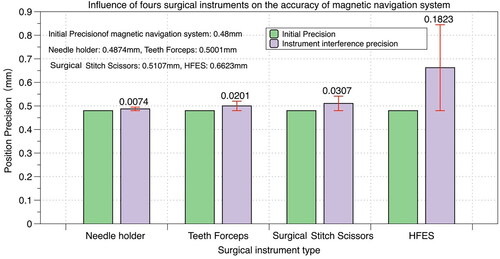
The current experiments demonstrate that the placement of these metal instruments in a magnetic field environment affects navigation system accuracy. However, different positions in the magnetic field affect accuracy to varying degrees. The general conditions were as follows: 1. The closer the instrument is to the magnetic field generator, the greater the impact; 2. The closer the instrument is to the navigation target (the mandible model), the greater its influence; 3. The influence was greatest when the device was positioned between the generator and the navigation target al.though a navigation deviation of <2 mm may seem acceptable, the deviation increases with the number of instruments and time. As the surgery progresses, the deviation sources move in and out of the navigation area, the offset of which gradually accumulates. These conditions guide us to place the metal instrument as far away as possible when using magnetic navigation technology in clinical practice and when it is inevitable due to surgery needs, we should ensure that it is not directly between the magnetic field generator and the navigation target. Surgical instruments with currents that cause magnetic field interference, such as high-frequency electrosurgical instruments, should be temporarily disabled during navigation. After use of the instrument, which may affect magnetic navigation accuracy during surgery, it should be recalibrated in a timely manner to eliminate interference.
Research involving human participants and/or animals
This article does not describe studies of human participants or animals performed by any of the authors.
Disclosure statement
No potential conflict of interest was reported by the author(s).
Additional information
Funding
References
- Schleer P, Drobinsky S, DE LA Fuente M, et al. Toward versatile cooperative surgical robotics: a review and future challenges. Int J Comput Assist Radiol Surg. 2019;14(10):1673–1686.
- Jiang T, Zhu M, Chai G, et al. Precision of a novel craniofacial surgical navigation system based on augmented reality using an occlusal splint as a registration strategy. Sci Rep. 2019;9(1):501.
- Sukegawa S, Kanno T, Furuki Y. Application of computer-assisted navigation systems in oral and maxillofacial surgery. JPN Dent Sci Rev. 2018;54(3):139–149.
- Kuang S, Tang Y, Lin A, et al. Intelligent control for human-robot cooperation in orthopedics surgery. Adv Exp Med Biol. 2018;1093:245–262.
- Kügler D, Krumb H, Bredemann J, et al. High-precision evaluation of electromagnetic tracking. Int J Comput Assist Radiol Surg. 2019;14(7):1127–1135.
- Song S, Ren H. Magnetic tracking in medical robotics [M]. Electromagnet Actuat Sensing Med Robot. 2018;7:141–162.
- Wagner A, Schicho K, Birkfellner W, et al. Quantitative analysis of factors affecting intraoperative precision and stability of optoelectronic and electromagnetic tracking systems. Med Phys. 2002;29(5):905–912.
- Schicho K, Figl M, Donat M, et al. Stability of miniature electromagnetic tracking systems. Phys Med Biol. 2005;50(9):2089–2098.
- Lascalza S, Arico J, Hughes R. Effect of metal and sampling rate on accuracy of flock of birds electromagnetic tracking system. J Biomech. 2003;36(1):141–144.
- Fang DM, Ritter MA, Davis KE. Coronal alignment in total knee arthroplasty: just how important is it?. J Arthroplasty. 2009;24(6 Suppl):39–43.
- Milne A, Chess D, Johnson J, et al. Accuracy of an electromagnetic tracking device: a study of the optimal operating range and metal interference. J Biomech. 1996;29(6):791–793.
- Poulin F, Amiot L-P. Interference during the use of an electromagnetic tracking system under or conditions. J Biomech. 2002;35(6):733–737.
- Sadjadi H, Hashtrudi-Zaad K, Fichtinger G. Simultaneous localization and calibration for electromagnetic tracking systems. Int J Med Robot. 2016;12(2):189–198.
- Stevens F, Conditt MA, Kulkarni N, et al. Minimizing electromagnetic interference from surgical instruments on electromagnetic surgical navigation. Clin Orthop Relat Res. 2010;468(8):2244–2250.
- Wimmer W, Venail F, Williamson T, et al. Semiautomatic cochleostomy target and insertion trajectory planning for minimally invasive cochlear implantation. Biomed Res Int. 2014;2014(3):596498.
- Labadie RF, Balachandran R, Mitchell J, et al. Clinical validation study of percutaneous cochlear access using patient customized micro-stereotactic frames. Otol Neurotol. 2010;31(1):94–99.