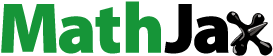
ABSTRACT
An investigation was carried out to examine the potential to utilise densified wood dowels as a reinforcement for timber subjected to compression loading perpendicular to the grain. While timber has a high strength-to-weight ratio parallel to the grain, it demonstrates poor strength perpendicular to the grain and in recent years there has been a significant number of studies examining the use of steel screws and bonded-in rods as reinforcement in this area. This is becoming more and more important with the increased use of timber in medium-to-high rise structures. In this study, thermo-mechanical densified wood in the form of dowels are utilised as compression reinforcement perpendicular to the grain and tested to failure. Thermo-mechanically densified dowel reinforcement arrangements of 2, 4, and 6 dowels are examined experimentally under a compressive load and compared to timber samples similarly reinforced but with steel self-tapping screws. The results have demonstrated the potential to utilised densified wood to create an all-wood solution to reinforce against compressive stresses perpendicular to the grain. Additionally, modifications to recently proposed Eurocode 5 recommendations for the design of compression reinforcement using self-tapping steel screws are presented, which are suitable for the design of compression reinforcement using densified wood dowels.
Introduction
With the increased focus in recent years on global warming and the impact of human activities on the environment, the construction industry is striving to reduce its carbon footprint. This has led to a considerable body of research into the development of timber structures as a sustainable alternative to steel and concrete. There has been a significant amount of innovation in different technologies and materials to develop highly engineered wood products for the construction industry which has resulted in the increased use of timber in medium- to high-rise construction. Advances in engineered wood products and connection systems have allowed timber to rival more commonly used construction materials and to meet the structural demands of modern construction. While timber has good strength parallel to the grain it demonstrates poorer strength perpendicular to the grain. As a result, stress perpendicular to the grain is an important factor in the design of engineered wood products used in timber structures and these stresses need to be taken into careful consideration in the design process, especially in areas of support (Hassan et al., Citation2014).
The use of reinforcement in the form of glued-in rods or screws in timber structures has also helped advance the possibilities when it comes to building with timber. Currently, the use of glued-in rods or steel screws as reinforcement is not prescribed in Eurocode 5 (CEN, Citation2005). Recent developments, which are well described in the literature (Bejtka & Blass, Citation2006; Dietsch & Brandner, Citation2015; Harte & Dietsch, Citation2015; Harte et al., Citation2015), allow for the reinforcement of timber perpendicular to the grain using self-tapping screws. These methods can also be used to reinforce notches, holes in beams, or to reinforce against tension stresses perpendicular to the grain in curved or pitched cambered beams, for example, (Dietsch & Brandner, Citation2015; Harte & Dietsch, Citation2015; Harte et al., Citation2015). For practical applications, the reinforcement of timber perpendicular to the grain is currently governed by European Technical Approvals (ETAs), which are supplier-specific (O’Ceallaigh & Harte, Citation2019); however, the use of reinforcement is due to be prescribed in the next generation of Eurocode 5, which is currently under development (Dietsch, Citation2019).
While self-tapping screws are a simple economic method of reinforcing timber, this approach relies on the use of carbon-intensive steel, which may be ultimately underutilised in terms of stress. Previous studies examining the use of hardwood dowels as reinforcement perpendicular to the grain have shown significant potential to improve the load-carrying capacity of timber perpendicular to the grain (Crocetti et al., Citation2012; Ed & Hasselqvist, Citation2011) but ultimately, they were outperformed by steel screw alternatives. In this study, timber elements are reinforced perpendicular to the grain with densified wood dowels and are compared with similar timber specimens reinforced with steel self-tapping screws. Densified wood dowels are made from softwood timber that has been radially compressed under heat and pressure, which increases its density and enhances its structural properties. In this study, tests were conducted on unreinforced timber specimens which form the basis for comparison. Test configurations with 2, 4 and 6 screws/dowels are examined and compared to unreinforced timber specimens. The experimental results are also compared to the analytical design predictions.
Compression reinforcement perpendicular to the grain
Load carrying capacity
When a load above the elastic limit is applied to a timber element perpendicular to the grain the stresses cause the longitudinally orientated fibers of the timber to collapse. This can cause densification and increased compressive strength but also causes permanent deformation. The compressive strength of a timber element typically depends on its density and the denser the timber the higher the compressive strength. The characteristic compressive strength perpendicular to the grain can be estimated at 10% to 20% of the parallel to the grain compressive strength or at 0.007 times the density of the timber for softwoods (Harte, Citation2009).
Experimentally, the compressive strength perpendicular to the grain of a timber element, fc,90, can be determined from EquationEquation (1)(1)
(1) in accordance with the European standard EN 408 (CEN, Citation2012).
where b is the width and l is the length of the specimen and the force Fc,90,max is determined from the load-deformation curve, constructed as shown in .
Figure 1. Load-deformation curve in accordance with EN 408 (CEN, Citation2012)
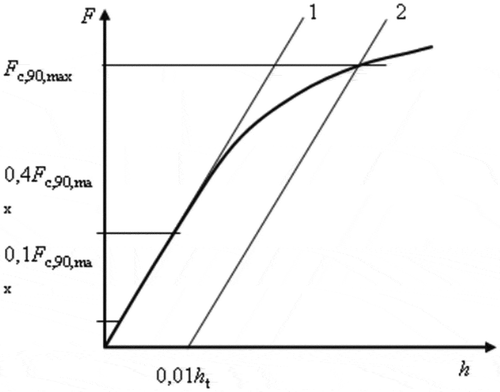
To distribute the perpendicular to the grain stresses throughout the section, a timber element can be reinforced by using screws or dowels inserted into the section perpendicular to the grain to improve stress dispersion into the timber (Dietsch, Citation2019). This reinforcement prevents early cracking, increases the load-bearing capacity perpendicular to the grain, decreases deformation and increases stiffness. Reinforcement against perpendicular to the grain stresses can be an important design requirement at end-bearing supports, internal supports, at notches and holes within structural timber elements. Buckling and pushing-in failure are the two types of failure modes often associated with screw reinforcement subjected to compressive stresses perpendicular to the grain (Dietsch, Citation2019; ETA-11/Citation0030, 2019; Hassan et al., Citation2014).
Design of compression reinforcement
For the purpose of developing a modern design standard, the CEN standardisation committee TC 250 has established a Working Group 7 “Reinforcement” to investigate current technologies for the new generation of Eurocode 5. Dietsch (Citation2019) provides a description of the work items, work plan, structure, design approaches and background information with regard to the new section of the proposed Eurocode 5. The committee is examining the use of reinforcement of timber in curved beams, notches and holes, connections and support sections subjected to compression perpendicular to the grain. EquationEquation (2)(2)
(2) is the method proposed for the new section of Eurocode 5 to specify the design of reinforcement of members under compression perpendicular to the grain (Dietsch, Citation2019) (also see ETA-11/Citation0030 (2019) for information related to the VGS screws produced by Rothoblaas used in this study).
where:
Fc,90,Rk = Load carrying capacity perpendicular to the grain according to ETA-11/Citation0030 (2019)
kc,90 = Compression factor according to 6.1.5.1 in CEN (Citation2005)
bc = Contact width
= Effective length parallel according to 6.1.5 in CEN (Citation2005)
n = Number of screws
= Pull through capacity according to CEN (Citation2005) or ETA-11/Citation0030 (2019)
= Buckling capacity according to CEN (Citation2005) or ETA-11/Citation0030 (2019)
b = Width of the beams
= Effective distribution length CEN (Citation2005)
Densified wood dowels
This study focuses on determining the suitability of densified wood as a potential reinforcement option against perpendicular to the grain stresses. The primary aim of densification is to increase the mechanical properties (e.g., strength, elastic modulus and hardness) by increasing the density. In this study, densification is carried out using a process known as thermo-mechanical densification by heating the timber and subjecting it to a compressive force. Additionally, timber can be densified by impregnating the voids between the cell walls with different materials such as molten metals/sulphur and polymers (Bodig & Jayne, Citation1993; Kollmann et al., Citation1975; Schwarzkopf, Citation2020) however, this is not considered in this study. The degree to which the timber has been densified is often termed the compression ratio. The compression ratio (or densification ratio) refers to the difference between the initial and final thickness of the wood as a percentage of the initial thickness. EquationEquation (3)(3)
(3) gives the formula for the compression ratio (CR), where t0 and tc are the thicknesses (in the compression direction) before and after compression, respectively.
Densification of wood is typically carried out in the radial direction (Beaud et al., Citation2008a; Kutnar et al., Citation2015). Typically, densification in the tangential direction is avoided as tangential compression can lead to damage and buckling of the latewood annual rings resulting in a zig-zag pattern forming through the cross-section of the densified wood material (Kutnar et al., Citation2015; Sandberg et al., Citation2013; Sotayo et al., Citation2020). The temperature required for the densification of wood typically ranges between 120–160°C (Kutnar et al., Citation2015). Heating the timber results in softening of the lignin which results in the dissipation of internal stresses in the wood which enables the free folding of the cell walls under the compressive action and the lumens of the cells can be almost completely closed. With this method, compression ratios of up to 68% can be achieved in the radial direction for spruce. Factors such as species, pre/post-treatment conditions, compression ratio, pressure applied, and pressing time, temperature and speed can influence the process, quality, and mechanical properties of the densified material (Beaud et al., Citation2008b, Citation2008c; Sotayo et al., Citation2020).
Densified wood dowels have demonstrated the potential to be a sustainable and environmentally friendly alternative to hardwood and metallic fasteners such as self-tapping steel screws. When tested in shear, densified wood dowels showed good properties when compared with other standard hardwood dowels (Jung et al., Citation2008). It was also shown that as the density of the dowel is increased, the results show a proportional improvement in stiffness, yield load and maximum load (Jung et al., Citation2008). When used in post-and-beam connections, densified wood dowels showed very good properties in resisting pull-out and moment rotation forces and demonstrated the potential for this type of modified timber material to be used for structural purposes such as long-span frame structures and has the potential to be further optimised (Jung et al., Citation2009).
The densified wood used in this study was produced by thermo-mechanical compression of softwood timber (Scots pine – Pinus sylvestris) to increase its density, strength, stiffness and hardness (Mehra et al., Citation2019, Citation2018) making it highly suited to demanding applications.
Experimental procedure
Introduction
The test programme comprised 35 compression test specimens within 7 test series/configurations as seen in . There are 5 repetitions of each test configuration. This resulted in 5 unreinforced specimens, 15 reinforced with steel screws and 15 reinforced with densified wood dowels. For the reinforced specimens, five specimens were reinforced with six self-tapping screws/dowels, five specimens reinforced with four screws/dowels and five specimens reinforced with two screws/dowels. The reinforcement configurations can be seen in where the screw reinforced specimens are shown. The spacing requirements chosen for this study were in excess of the minimum spacing, end and edge distances specified in Eurocode 5 (CEN, Citation2005) and ETA-11/Citation0030 (2019). The same reinforcement orientation and spacing requirements were used for the densified wood dowel reinforced specimens.
Figure 2. Reinforcement configurations, (a) reinforced with six self-tapping screws, (b) reinforced with four self-tapping screws and (c) reinforced with two self-tapping screws
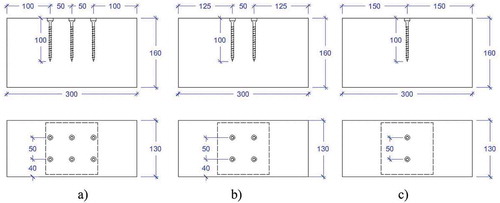
Table 1. Experimental test programme
Glued laminated timber
The timber material used in this study was Douglas fir (Pseudotsuga menziesii) grown in Ireland. The density and dynamic modulus of elasticity of the sawn boards were assessed, and glued laminated members were designed and manufactured to minimise the variation of elastic modulus and density between the specimens. The laminates were allowed to condition to approximately 12% moisture content before being planed to a finished laminate thickness of 40 mm. The laminates where then glued together using a one-component PUR adhesive and clamped in a rig at a minimum pressure of 0.6 MPa in accordance with EN 14080 (CEN, Citation2013). The boards remained under pressure until the adhesive cured. All the glued laminated specimens were then placed in a climate-controlled conditioning room at a temperature of 20 ± 2°C temperature and 65 ± 5 % relative humidity to ensure a mean moisture content of all specimens was approximately 12% prior to testing. The final test specimens measuring 300 mm in length, 130 mm wide and 160 mm in depth were cut from the manufactured glued laminated members and were accurately prepared to ensure that the loaded surfaces were plane and parallel to each other.
Screw reinforcement
The screws utilised in this study were 9 mm diameter VGS screws with a length of 100 mm supplied by Rothoblaas. These screws are specifically designed to reinforce timber elements subjected to compressive stresses perpendicular to the grain and their use in timber structures is governed by ETA-11/Citation0030 (2019). The preparation of the screw reinforced specimens can be seen in . Pre-drilling of the timber was required as the screw diameter is greater than 6 mm, but the diameter of the pre-drilling holes could not be greater than 5.6 mm in accordance with Eurocode 5 (CEN, Citation2005). The holes were drilled with a 5 mm diameter drill bit to a depth of 100 mm. The fully threaded self-tapping steel screws were screwed into the timber until the countersunk head was flush with the surface of the timber specimen.
Densified wood dowel reinforcement
The densified timber utilised in this study was manufactured from Scots Pine (Pinus Sylvestris) wood. The timber was thermo-mechanically compressed in the radial direction to a compression ratio of approximately 54% ± 1% at the University of Liverpool. The dowels were manufactured by heating un-densified timber specimens measuring 200 mm in length, 22 mm in depth (radial direction) and 10 mm in width (tangential direction) to a temperature of 130°C. The timber specimens were heated in a specially designed mould with curved ends with a radius of 5 mm. The mould was then subjected to a pressure compressing the radial dimension of the timber and forming a 10 mm diameter dowel when the mould is fully closed. The densified timber dowels were then cooled under pressure until the temperature was less than 66°C (Mehra et al., Citation2018). This resulted in a final mean density ranging between 1100–1500 kg/m3. The densified wood dowel diameter used was 10 ± 0.5 mm and the dowel length was 100 mm.
Preparation of the dowel reinforced specimens can be seen in . The specimens were predrilled with a 10.5 mm diameter drill bit to a depth of 100 mm and debris and dust were cleaned with the use of pressurised air. A one-component PUR adhesive was applied evenly on the surface of the dowels and in the predrilled holes at a rate of 160 g/m2. The densified wood dowels with a length of 200 mm were then inserted into the predrilled holes ejecting excess adhesive and ensuring complete coverage of the dowel/timber interface. The adhesive was then allowed to cure after which the dowels were then cut at the surface of the timber specimen to a finished length of 100 mm and sanded to ensure the remaining dowel length has a flush finish between the dowel and timber surface.
Density matching of test series
There is a strong relationship between the compressive load-bearing capacity of timber and the timber density. As a result, it was important to ensure matched groups, statistically equal in terms of mean density, were created. Statistical methods were implemented to ensure the distribution of the density is similar for each series prior to reinforcement. Therefore, the difference in results from a reinforced series should represent the effect of the reinforcement on the load-bearing capacity of the timber member (O’Ceallaigh et al., Citation2018).
There were three types of statistical tests performed on each test series. In this study, all statistical tests were carried out to a significance level of 0.95 (α = 0.5). Shapiro-Wilk tests were used to check for normality and have shown each series to be normally distributed. The Levene’s test was used to examine the homogeneity of each series or the variance within the series and showed no significant difference in the variance between each series. As a result, the Student’s t-test was carried out to examine the mean density of each series and it was shown that there was no statistically significant difference in the mean density values between each series. The formation of each series, statistically equal in terms of the mean density, formed a basis for the comparative study of the different reinforcement arrangements.
Test procedure
The test procedure used was based on an extensive review of the literature and the test procedure specified in EN 408 (CEN, Citation2012) related to the evaluation of compressive strength of glued laminated elements perpendicular to the grain. A graphical representation of the test set-up and six screw/dowel reinforcement configurations can be seen in . The timber cross-section remained constant for each test and a common bearing area (120 x 120 mm2) was used in all tests to focus on the influence of the reinforcement. Each specimen was centrally loaded with a constant crosshead movement to ensure failure was achieved in 300 ± 120s. Two linear variable displacement transformers (LVDTs) were located centrally on either side of the specimen over a gauge length of 0.6 x the height of the specimen to determine the stiffness of the reinforcing scheme using EquationEquation (4)(4)
(4) .
where l1 is the gauge length, A is the area and ΔF and Δw are the corresponding load and deflection increments, respectively on the straight line proportion of the load-deformation curve (See ).
Experimental results
Density and moisture content results
The density and moisture content were recorded for all 35 timber specimens. The density of the timber ranged from 485.8 kg/m3 to 653.1 kg/m3 with a mean density of 555.2 kg/m3 and a standard deviation of 35.6 kg/m3. Density tests were also conducted on the densified wood dowels. The density ranged from a minimum density of 1132.4 kg/m3 to a maximum density of 1437.9 kg/m3. The mean density of the dowels was 1253.8 kg/m3 with a standard deviation from the mean of 66.1 kg/m3. After the compression tests were completed, moisture content tests were conducted on all specimens using the over-dry method in accordance with EN 13183–1 (CEN, Citation2002). The results showed that the test specimens had a moisture content ranging between 11.3% and 13.2% with a mean moisture content of 12.3% for all specimens. The moisture content results had a standard deviation of 0.5% for the entire test programme.
Unreinforced test results
The unreinforced test series results are shown in and the corresponding load-deformation curves are shown in . The experimental results show a mean value for the maximum compressive force perpendicular to the grain (Fc,90,max) of 123.6 kN, for the compressive strength perpendicular to the grain (fc,90) of 8.6 N/mm2 and for the modulus of elasticity perpendicular to the grain (Ec,90) of 1841.3 N/mm2. These values have standard deviations of 28.2 kN, 2.0 N/mm2 and 598.9 N/mm2, respectively. The mean results for the unreinforced series form a basis for comparison for the reinforced test results using both self-tapping screw and densified wood dowel reinforcement.
Table 2. Unreinforced test series results
The range of values observed for the unreinforced series was found to be generally dependent on the density of the timber. For example, U3 had the highest capacity of 153.4 kN and also had the highest density of 576 kg/m3 while U4 had the lowest capacity of 94.1 kN and was found to have the lowest density with a value of 528 kg/m3.
Screw reinforcement results
In this section, the experimental results of screw reinforced timber specimens subjected to compressive loading perpendicular to the grain are presented. The load-deformation results for specimens reinforced with 6, 4 and 2 screws can be seen in . Generally, there is some variation in the results for each reinforcement configuration. To examine the behaviour further, the mean results from screw reinforced compression tests are presented in and the percentage increase in the mean results when compared to the unreinforced specimens is presented. The results of the experimental tests for specimens reinforced with 6 screws have shown a 64% increase in mean strength when compared to the unreinforced series and a mean stiffness increase of 84%. By comparison, the results for specimens reinforced with 4 screws showed an increase in mean strength of 41% and an increase in stiffness of 52%. Specimens reinforced with only 2 screws also demonstrated an increase in mean strength of 21% over the unreinforced series and an increase of 14% in mean stiffness.
Table 3. Test results for screw reinforced specimens
Densified wood dowel reinforcement results
The experimental results have shown that there is also an improvement in the compressive stiffness and strength of timber specimens reinforced with densified wood dowels. The load-deformation results for specimens reinforced with 6, 4 and 2 densified wood dowels can be seen in .
The load-deformation behaviour for all specimens was similar for all except two of the 4 dowel specimens. An investigation into the failure of these two specimens showed a lack of bond between the dowel and the inner surface of the timber as seen in ). As the cross-section was cut, the dowel separated from the timber with little effort. These specimens also showed large compression failure zones in the timber below the dowel indicating a failure of the bond and little composite action between the dowel and the timber. It is possible that there was excess dust remained from drilling or too smooth a surface post drilling, which adversely affected the adhesive bond. Further investigation is also required to examine the duration of time between drilling and adhesive application. It is reported that a shorter duration between timber preparation and adhesive application prevents unwanted oxidation and increases the infiltration of the adhesive into the exposed surface, which improves mechanical interlocking (Frihart, Citation2009). On the other hand, specimens with better bonds resulted in little to no compression damage at the bottom of the dowel as seen in ). ) shows a specimen with a good adhesive bond between the timber and the dowel which has resulted in compression failure or buckling of the longitudinal fibers occurring within the densified wood dowel.
Figure 9. Experimental investigation, (a) specimen with failure of the adhesive bond (4-dowel configuration), (b) specimen with good adhesive bond and compression wrinkle in the densified wood dowel (6-dowel configuration)
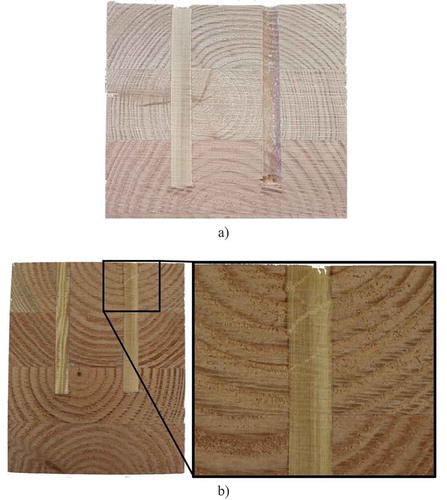
The mean results of the densified wood dowel reinforced compression tests are presented in . The results of the experimental tests showed an increase in mean strength of 30% for specimens reinforced with 6 densified wood dowels when compared to the unreinforced series and a mean stiffness increase of 37%. Specimens reinforced with 4 densified wood dowels had an increase in mean strength of 14% over the unreinforced series and an increase of 18% in mean stiffness but it should be noted that only three values were used to calculate the mean strength values due to the premature failure of two specimens. The stiffness values of the 4 dowel specimens were calculated using the complete data set as the elastic stiffness was unaffected by the premature failure observed in two specimens. Finally, specimens reinforced with 2 densified wood dowels showed an increase in mean strength of 16% over the unreinforced series and an increase of 20% in mean stiffness.
Table 4. Test results for densified wood dowel reinforced specimens
Comparison of results
To safely predict the behaviour of steel/dowel reinforcement perpendicular to the grain, it is important to compare the analytical predictions of current design equations to the experimental results in this study. As the reinforcement of timber perpendicular to the grain is proposed to be included in the next generation of Eurocode 5, the design approaches presented by Dietsch (Dietsch, Citation2019) were used to calculate design values for both steel screws and the densified wood dowel reinforcement.
EquationEquation (2)(2)
(2) previously presented was used to calculate the load-bearing capacity of the screw reinforced specimens. As seen in , the experimental results for the steel screw reinforced samples were greater than the characteristic design capacity results of all specimens demonstrating that the design equations are conservative and safe for use.
To estimate the load-bearing capacity of the test specimens reinforced with densified wood dowels, the equation used to estimate the capacity of steel screws was adapted. EquationEquation (2)(2)
(2) considers the minimum value of the strength of the timber and the strength of the screw/rod reinforcement (Dietsch, Citation2019; ETA-11/Citation0030, 2019). This equation has been adapted as shown in EquationEquation (4)
(4)
(4) . The pull-through capacity, Fax,α,Rk, of the steel screw has been removed from the formula as it is not applicable for the calculation for densified wood dowel reinforcement resulting in EquationEquation (4)
(4)
(4) .
where Fb,Rk is the buckling capacity of the densified wood dowel, n is the number of densified wood dowels and the other terms are as previously described. The densified wood dowel properties in the parallel to the grain direction used in this study are based on experimental results presented by Mehra et al. (Citation2018). shows a comparison between the characteristic design results and the recorded results from testing of the specimens reinforced with densified wood dowels. The graph shows that all specimens have a greater capacity than the design values, even the two specimens with 4 dowels for which there were manufacturing defects in the test specimens ()). It is important to note that the characteristic density used in the calculations is based on the density of all 35 specimens tested in this study in accordance with EN 14358 (CEN, Citation2006). The findings have shown that the design equations are conservative when compared to the experimental results, but further tests are required to establish characteristic values from experimental testing.
When examining the percentage increase in stiffness of the different reinforcement configurations, it was shown that the overall stiffness of the screw reinforced specimens was higher than that of the dowel reinforced specimens achieving a capacity but at lower load levels during the elastic phase of loading, the dowel reinforced specimens demonstrated better stiffness behaviour. This was also found by Crocetti et al. (Citation2012) who showed that hardwood dowel reinforced beams had a better stiffness during the initial elastic loading period when compared to steel dowel reinforced beams, however, steel-reinforced beams ultimately achieved higher loads and better stiffness. It was suggested that the variations in strength and stiffness at lower load levels were due to the difficulty in achieving a smooth finish between the steel dowel and the timber surface. Without a smooth flush finish between the steel and timber surfaces, the load will not be equally distributed between all dowels. A similar experiment conducted by Ed and Hasselqvist (Citation2011) confirms that the steel dowels were not perfectly flush with the timber surface after insertion.
Conclusions
An experimental investigation of timber specimens reinforced with self-tapping screws and densified wood dowels has been presented. A total of 7 series and 35 specimens were tested with varying reinforcement configurations.
The results of the compression tests have shown that improvements in compression strength and stiffness perpendicular to the grain can be achieved when utilising self-tapping screws. Specimens reinforced with just 2 self-tapping screws demonstrated an increase in mean strength and stiffness of 21% and 14%, respectively, when compared to unreinforced specimens. This increases to 64% and 84% for mean strength and stiffness, respectively, for specimens reinforced with 6 screws. When examining specimens reinforced with densified wood dowels, the results showed an increase in compressive strength of 16% for specimens reinforced with only 2 densified wood dowels, and a mean increase of 30% for specimens reinforced with 6 densified wood dowels. While the steel screw reinforcement demonstrates superior performance, there is an increase in strength perpendicular to the grain for the densified wood dowel option. Reinforcement with densified wood dowels also resulted in increased stiffness perpendicular to the grain with a mean increase of up 36% for specimens reinforced with 6 dowels.
Bond failure was observed in a limited number of specimens for the series reinforced with 4 densified wood dowels. The issue is likely due to excess sawdust from drilling and has demonstrated the importance of good quality control during the manufacturing process.
The design equations, which have been put forward for reinforcement of timber with steel screws in the new Eurocode 5, were used to predict the load-bearing capacity for the specimens tested in this study. The results were shown to give good predictions of the load-bearing capacity of steel self-tapping screw reinforced timber and an adapted formula to consider the buckling capacity of the densified wood dowels has also been shown to conservatively predict the failure behaviour of densified wood dowel reinforced timber. While the current findings show promising results, further tests are required to establish characteristic values from experimental testing to further evaluate the performance of densified wood dowels as a possible reinforcement against stresses perpendicular to the grain. The influence of dowel length and dowel diameter may also have a considerable effect on the compressive strength and stiffness of specimens reinforced perpendicular to the grain and this requires further attention. Also, an investigation is required to evaluate efficient and effective means of bonding the dowel into the timber element, which would compete with the ease of handling of the self-tapping steel screws.
Acknowledgments
The authors would also like to thank Murray Timber Group for supplying the Douglas fir, Rothoblaas for supplying the screws used in this study and A. Sotaya and Z. Guan of the University of Liverpool for supplying the densified wood dowels utilised in this study.
Disclosure statement
No potential conflict of interest was reported by the author(s).
References
- Beaud, F. J., Niemz, P., Blumer, H., Wüthrich, H.-P., & Wüthrich, S. (2008a). Herstellung und Eigenschaften von großformattgen Platten aus verdichtetem Frichten-und Tannenholz: Teil 1: Plattenherstellung. Holztechnologie, 49(2), 9–14.
- Beaud, F. J., Niemz, P., Blumer, H., Wüthrich, H.-P., & Wüthrich, S. (2008b). Herstellung und Eigenschaften von großformattgen Platten aus verdichtetem Frichten-und Tannenholz: Teil 2: Physikalische Eigenschaften. Holztechnologie, 49(3), 14–19.
- Beaud, F. J., Niemz, P., Blumer, H., Wüthrich, H.-P., & Wüthrich, S. (2008c). Herstellung und Eigenschaften von großformattgen Platten aus verdichtetem Frichten-und Tannenholz: Teil 3: Mechanische Eigenschaften. Holztechnologie, 49(4), 17–21.
- Bejtka, I., & Blass, H. J. (2006). Self-tapping screws as reinforcements in beam supports. International Council for Research and Innovation in Building and Construction - Working Commission W18 - Timber Structures (pp. 1–13). Florence.
- Bodig, J., & Jayne, B. A. (1993). Mechanics of wood composites. Reprinted ed. Von Nostrand Reinhold Company.
- CEN. (2002). EN 13183-1. Moisture content of a piece of sawn timber - Part 1: Determination of oven dry method. Comité Européen de Normalisation, Brussels. Comité Européen de Normalisation.
- CEN. (2005). EN 1995- 1-1.Eurocode 5: Design of timber structures - Part 1-1: General - Common rules and rules for buildings. Comité Européen de Normalisation.
- CEN. (2006). EN 14358. Timber structures - Calculation of characteristic 5-percentile values and acceptance criteria for a sample. Comité Européen de Normalisation.
- CEN. (2012). EN 408. Timber structures - Structural timber and glued laminated timber - Determination of some physical and mechanical properties. Comité Européen de Normalisation.
- CEN. (2013). EN 14080. Timber structures - Glued laminated timber and glued solid timber - Requirements. Comité Européen de Normalisation.
- Crocetti, R., Gustafsson, P. J., Ed, D., & Hasselqvist, F. (2012). Compression strength perpendicular to grain - full-scale testing of glulam beams with and without reinforcement. COST Action FP1004 Early Stage Researchers Conference (pp. 51–62). Zagreb.
- Dietsch, P. (2019). Reinforcement of timber structures - Standardization towards a new section for EC5. In Proceedings of the 5th International Conference on Structural Health Assessment of Timber Structures (SHATiS) 2019, 25-27th September, Guimarães.
- Dietsch, P., & Brandner, R. (2015). Self-tapping screws and threaded rods as reinforcement for structural timber elements-A state-of-the-art report. Construction and Building Materials, 97, 78–89. Reinforcement of Timber Structures. https://doi.org/10.1016/j.conbuildmat.2015.04.028
- Ed, D., & Hasselqvist, F. (2011). Timber compression strength perpendicular to the grain – Testing of glulam beams with and without reinforcement.
- ETA-11/0030. (2019). European technical assessment. Screws for use in timber construction. ETA-Denmark, Nordhavn.
- Frihart, C. R. (2009). Adhesive groups and how they relate to the durability of bonded wood. Journal of Adhesion Science and Technology, 23(4), 601–617. https://doi.org/10.1163/156856108X379137
- Harte, A. (2009). Introduction to timber as an engineering material. In M. Forde (Ed.), ICE manual of construction materials (Vol. 2, pp. 707–715). Thomas Telford. https://doi.org/10.1680/mocm.00000.0001
- Harte, A. M., & Dietsch, P. (2015). Reinforcement of timber structures: A state-of-the-art report ( A. M. Harte & P. Dietsch Eds.). Shaker Verlag GmbH.
- Harte, A. M., Jockwer, R., Stepinac, M., Descamps, T., & Dietsch, P. (2015). Reinforcement of timber structures - The route to standardisation. 3rd International Conference on Structural Health Assessment of Timber Structures, Wroclaw.
- Hassan, K. A., Hussain, T., & Arman, K. (2014). Compression perpendicular to grain in timber - Bearing strength for a sill plate [Master Thesis]. Linnaeus University.
- Jung, K., Kitamori, A., & Komatsu, K. (2008). Evaluation on structural performance of compressed wood as shear dowel. Holzforschung, 62(4), 461–467. https://doi.org/10.1515/HF.2008.073
- Jung, K., Kitamori, A., & Komatsu, K. (2009). Development of a joint system using a compressed wooden fastener I: Evaluation of pull-out and rotation performance for a column-sill joint. Journal of Wood Science, 55(4), 273–282. https://doi.org/10.1007/s10086-009-1027-3
- Kollmann, F., Kuenzi, E., & Stamm, A. (1975). Principles of wood science and technology: II wood based materials (1st ed.). Springer-Verlag Berlin Heidelberg New York.
- Kutnar, A., Sandberg, D., & Haller, P. (2015). Compressed and moulded wood from processing to products. Holzforschung, 69(7), 885–897. https://doi.org/10.1515/hf-2014-0187
- Mehra, S., Mohseni, I., O’Ceallaigh, C., Guan, Z., Sotayo, A., & Harte, A. M. (2019). Moment-rotation behaviour of beam-column connections fastened using compressed wood connectors. SWST 62 nd International Convention : Renewable Materials and the Wood-based Bioeconomy (pp. 2019). California, USA.
- Mehra, S., O’Ceallaigh, C., Hamid-Lakzaeian, F., Guan, Z., & Harte, A. M. (2018). Evaluation of the structural behaviour of beam-beam connection systems using compressed wood dowels and plates. WCTE 2018 - World Conference on Timber Engineering, August 20-23, 2018. Seoul. https://www.researchgate.net/publication/327186842
- O’Ceallaigh, C., & Harte, A. M. (2019). The elastic and ductile behaviour of CLT wall-floor connections and the influence of fastener length. Engineering Structures, 189(June2019), 319–331. https://doi.org/10.1016/j.engstruct.2019.03.100
- O’Ceallaigh, C., Sikora, K., McPolin, D., & Harte, A. M. (2018). An investigation of the viscoelastic creep behaviour of basalt fibre reinforced timber elements. Construction and Building Materials, 187(October2018), 220–230. https://doi.org/10.1016/j.conbuildmat.2018.07.193
- Sandberg, D., Haller, P., & Navi, P. (2013). Thermo-hydro and thermo-hydro-mechanical wood processing: An opportunity for future environmentally friendly wood products. Wood Material Science and Engineering, 8(1), 64–88. https://doi.org/10.1080/17480272.2012.751935
- Schwarzkopf, M. (2020). Densified wood impregnated with phenol resin for reduced set-recovery. Wood Material Science and Engineering, 16(1), 35–41. https://doi.org/10.1080/17480272.2020.1729236
- Sotayo, A., Bradley, D., Bather, M., Sareh, P., Oudjene, M., El-Houjeyri, I., … Guan, Z. (2020). Review of state of the art of dowel laminated timber members and densified wood materials as sustainable engineered wood products for construction and building applications. Developments in the Built Environment, 1, 100004. https://doi.org/10.1016/j.dibe.2019.100004