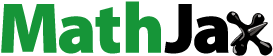
ABSTRACT
Purpose
To determine the changes in physical qualities of academy rugby union players over a 10-week unsupervised off-season period.
Methods
Body mass, jump height, sprint performance, and intermittent running (30:15 IFT) of 64 academy rugby union players (age = 17.2 ± 0.4 y) were recorded before and after the off-season.
Results
Changes in body mass (+1.4 ± 1.3 kg), countermovement jump (−2.2 ± 1.2 cm), squat jump (-1.5 ± 1.8 cm), 10 m sprint (+0.06 ± 0.05 s), 40 m sprint (+0.13 ± 0.11 s) and 30:15 IFT (-0.8 ± 0.8 kmŸh−1) were observed (P < 0.001, d = -1.77 to 0.47). Only changes in body mass were greater in forwards than backs (P = 0.036, d = 0.46). Players with higher end-of-season body mass, squat jump and 30:15 IFT had greater off-season changes (P = < 0.001 to 0.044; d = 0.63 to 0.94), whilst the pre-post difference in body mass influenced CMJ (P = 0.005, d = 0.75) and 10 m momentum change (P < 0.001, d = 1.61).
Conclusion
Understanding the individuality of the changes in physical qualities of academy rugby union players during the off-season is important to ensure players return safely to pre-season training loads.
Introduction
Rugby union players should possess well-developed strength, power, speed and intermittent running capacity as well as sport-specific attributes to compete at the highest standards (Argus et al. Citation2012; Smart and Gill Citation2013). Accordingly, a rugby union season is divided intro three main periods; namely, pre-season, in-season (comprising the main competition and play-offs), and off-season. Each of these periods has a specific focus where the volume, intensity and frequency of training are manipulated to promote appropriate physical and skill-related adaptations. The development and retention of physical qualities is typically dedicated to the pre- (4–10 weeks) and in-season (~32 weeks) periods, respectively, where practitioners implement a structured programme designed to maximise athletic performance, recovery and moderate injury risk. Given the loads imposed on young rugby union players throughout a season (Hartwig et al. Citation2009), the off-season offers a 6–12-week period that enables players to regenerate mentally and physically (Vassilis et al. Citation2019), comprising complete training cessation or incorporating individualised, unsupervised low-volume training to mitigate losses in physical qualties (Clemente et al. Citation2021) and at least maintain an athlete’s tolerance to pre-season training (Silva et al. Citation2016).
A reduced training load causes a partial or total physiological loss of training-induced adaptations due to a reduction in training volume (Melchiorri et al. Citation2014) or insufficient training stimuli (Mujika and Padilla Citation2000). A practically interesting contributor to detraining is the off-season, involving deliberate long term (>4 weeks) reductions in training volume, intensity and frequency. Detraining in team sport players after an off-season (≥4 weeks) causes reductions in maximal oxygen uptake (O2max; 0.8% to 21.2%; Caldwell and Peters Citation2009; Sotiropoulos et al. Citation2009; Koundourakis et al. Citation2014; Melchiorri et al. Citation2014), which are associated with reductions in blood and plasma volume (5–12%), increases in heart rate during submaximal and maximal training (5–10%), and lower stroke volume (10–17%) (Mujika and Padilla Citation2000; Silva et al. Citation2016). Reductions (14.8–22.6%) in intermittent running capacity have also been reported after short-term (~2 weeks) detraining periods (Thomassen et al. Citation2010; Christensen et al. Citation2011; Joo Citation2018), suggesting study of the effects of longer detraining periods is needed. Likewise, reductions in vertical jumping (2.1–6.3%) and increased sprint times (2.4–3.3%) (Caldwell and Peters Citation2009; Koundourakis et al. Citation2014; Requena et al. Citation2017) could be associated with reduced strength training and the associated neuromuscular and hormonal adaptations (Tsolakis et al. Citation2004). McMaster et al. (Citation2013) reported that maximum strength and power could be maintained for a period of 3 weeks without training, thereafter reductions in strength (2% per week) and power (0.1% to 0.6% per week) were observed after ~7 weeks and was related to neural mechanisms with atrophy evident if detraining is extended. While the addition of an off-season training programme might minimise any detraining effect (Clemente et al. Citation2021), changes to endurance, strength, power and speed seem a likely consequence of the off-season.
Several studies have studied off-season alterations in a range of physical qualities pertinent to rugby players. A 15-week off-season period caused increases in estimated one repetition maximum bench press, chin-up and box squat strength when adolescent players’ training was supervised by a coach, compared to a group who trained unsupervised and remained unchanged (Smart and Gill Citation2013). Conversley, Jensen et al. (Citation2018) reported improvements in O2max, squat strength and vertical jump, alongside increases in body fat percentage in collegiate rugby players after a 4-week unsupervised training break. These findings are in contrast to professional adult male rugby league players (Dobbin et al. Citation2020) and national league male adult rugby union players (Nirmalendran and Ingle Citation2010) who reported reductions in strength, power, speed and aerobic endurance after 8- and 6-week unsupervised off-seasons, respectively. It remains to be elucidated what effects longer periods of unsupervised training would have on the physical qualties of young rugby players. For example, organisational contraints for academies or where extended breaks in face-to-face training are forced (e.g. COVID-19; Girardi et al. Citation2020) mean young players could be exposed to long periods without access to structured coaching. Further, studies exploring the positional variations in physical qualties to an off-season period are required in rugby union given forwards and backs have different qualities (Wood et al. Citation2018). Dobbin et al. (Citation2020) reported a high variablity in the change in sprint mechanical properties in rugby league players, with backs reporting a larger decrease in force and power, and forwards reporting a larger decrease in velocity. Reductions in young rugby players’ physical qualities towards the end of the in-season period (Dobbin et al. Citation2019) implies the effect of baseline fitness (i.e. end of season) should be accounted for to extend our understanding of off-season detraining in rugby players.
To understand how players respond to a dedicated period without direct coaching intervention, the aim of this study was to examine the changes in specific physical qualities of academy rugby union backs and forwards over a 10-week off-season period. This study enables strength and conditioning coaches to observe how specific physical qualities of academy rugby union players change during the offseason period when training is unsupervised.
Methods
Participants and design
Sample size was calculated a priori using G*Power (Faul et al. Citation2007), with an alpha of 0.05, a power of 0.8 and effect sizes based on known changes in relevant physical qualities of rugby players over an off-season (standardised mean difference = 0.26–1.05; Nirmalendran and Ingle Citation2010). Using these values, estimated sample sizes of between 8 and 93 players, depending on the physical quality, were calculated. Given the number of players available was limited to those registered by the club that the researchers were working with, a total of 64 players were categorised as forwards (n = 35; age 17.1 ± 0.3 y; stature 180.6 ± 7.2 cm) and backs (n = 29; age 17.2 ± 0.4 y; stature 179.8 ± 4.5 cm) were recruited. All participants were currently part of a regional professional system, had been for a minimum of 2 years (range 2–4 years) and provided written informed consent and parental consent for their data to be used. Ethics approval was provided by the University of Chester’s Faculty of Medicine, Dentistry and Life Sciences’ Ethics Committee (Ref: 589/19/JW/SES).
Using a single group repeated measures design, players were tested before and after a 10-week off-season. During the 10-weeks, players were encouraged to stay active with guidance provided by the club’s strength and conditioning coach beforehand. In the final 3-weeks of the off-season (weeks 8–10), players were given an unsupervised conditioning programme and asked to complete this in their own time away from the training facility (). This programme was performed on Monday, Wednesday and Friday and comprised a mixture of upper- and lower-body resistance exercise and metabolic conditioning. This approach is typical of team sports (Silva et al. Citation2016; Clemente et al. Citation2021) and ensures players return to preseason capable of coping with an increase in training load. Players reported verbally to the strength and conditining coach that they had completed the 3-week programme, with training load not monitored to avoid the unintended negative consequences on players (Manley and Williams, Citation2019).
Table 1. Outline of the 3-week unsupervised off-season training programme.
Table 2. Changes in body mass for backs and forwards. Values are mean ± SD with ∆%.
All players had performed the selected tests of physical qualties before. Testing comprised a 10 min dynamic warm up, countermovement and squat jumps, a 40 m sprint with times taken at 10 and 40 m, and then the 30:15 Intermittent Fitness Test. A 10–15 min passive recovery period was allowed between each test. Tests were performed in the order described, all completed on the same day with repeated trials at a similar time of day (± 2 h), conducted by the same researcher, using the same facilities and equipment. Trials before and after off-season were performed at the start of the week with players having completed no strenuous exercise in the 48 h before.
Procedures
Dynamic warm-up
The first warm-up included a variety of dynamic movements focusing on lower-body mobilisation, the last 5 min contained a reminder on the technical elements of countermovement jump and squat jump protocols. The second warm up, which was completed before sprint testing, focused on hamstring mobility and activation as well as some brief explosive jumps and bounds to activate the ankle complex. Then followed a series of sprints at 50, 75, 90 and 100% maximal effort while reinforcing good start position mechanics.
Jump procedures
Participants started upright before squatting down to a self-selected depth and extending upwards for maximal height, keeping their hands on hips and legs straight in the air. Jumps that did not meet this criteria were repeated. Players also completed a squat jump, requiring them to keep their hands on hips and squat down to a 90 degree knee angle, confirmed using a goniometer. The researcher would then place a hand on the participant’s chest, giving a countdown before the participant was instructed to jump. A jump was repeated if the participant tried to dip to gain momentum, indicated by the researcher recognising prior movement from the chest. For both jump types, participants completed three repetitions on a jump mat (Just Jump System, Probotics, Huntsville, USA) with 3-minutes passive recovery between all jumps. Jump values were corrected using the equation: 0.8492 * jump height – 5.5131 (Dobbin et al. Citation2017), with the highest jump height used for analysis. The coefficient of variation for this test with rugby players is 5.9% (Dobbin et al. Citation2018).
Sprint test procedures
Sprint performance was measured indoors on artificial 4 G turf using single-beam electronic timing gates (Brower Speedtrap 2, Utah, USA) positioned at 0, 10 and 40 m. Participants wore studded footwear and started each sprint from a 2-point athletic stance positioned 0.3 m behind the start line (Dobbin et al. Citation2018). Three maximal sprints were recorded to the nearest 0.01 s and the lowest 40 m sprint time and corresponding 10 m split used for analysis. Participants had 3 minutes of passive recovery between each sprint. Momentum for 10 m sprint was calculated by multiplying body mass with mean speed (distance/time) divided by best recorded 10 m sprint time. The coefficient of variation for 10 and 40 m split times using timing gates with rugby players has been reported as 3.1% and 1.3%, respectively (Darrall-Jones et al. Citation2016).
30:15 intermittent fitness test
Intermittent running peak speed was assessed using the 30:15 Intermittent Fitness Test (30:15 IFT; Buchheit Citation2008) on artificial 4 G turf. The 30:15 IFT comprises 30 s runs interspersed with 15 s of recovery, with athletes required to run back and forward between two lines 40 m apart at the speed governed by an audio signal. The test began at 8 km·h−1 and increased 0.5 km·h−1 every 30 s, thereafter. The pacing strategy enabled players to run appropriate intervals and adjust their running speed to be within 3 m zones at each end and middle of the course when the audio signal sounded. Players then had 15 s to walk to the nearest line at either end or the middle before the next stage. The test was terminated when a player could not maintain the required running speed or was unable to meet the 3 m zone on three occasions. Athletes were instructed to run until volitional exhaustion, with the peak speed recorded (km·h−1). The coefficient of variation of 30:15 test in rugby players has been reported as 1.9% (Scott et al. Citation2015).
Statistical analysis
Data are reported as mean and standard deviation (SD) after meeting assumptions of normality as determined using the Shapiro–Wilk test. Separate paired samples t-tests were used to determine the differences in all measures between the end of season and preseason as this was the primary objective. To assess the between-group differences between playing position (forwards vs. backs) in the change across the off-season period, an analysis of covariance was used with the change variable as the dependent variable, group as the fixed factor and end-of-season scores and the change in body mass as the covariate to control for existing group differences. The influence of the covaraiate was also determined as was the standard deviation of change for each playing position. The change in body mass was included as a covariate given the reported association between body mass and all physical qualities herein (Darrall-Jones et al. Citation2016; Casserly et al. Citation2020). Inferential statistics were assessed with probability set at 0.05 and analysed using SPSS (version 26, Armonk, N.Y., USA). The standardised mean difference (Cohen’s d) between timepoints were determined with 95% confidence limits. Cohen’s d was also estimated from the partial eta squared of the ANCOVA based on the relationship between partial eta squared and Cohen’s d, allowing for consistency to aid in interpretation.
Results
Anthropometry
Body mass for the group was higher after the off-season period (t = 8.121, P < 0.001, d = 1.02 [0.17; 1.87]) (). The ANCOVA indicated a greater relative increase in body mass in forwards compared to backs (F = 4.594, P = 0.036, d = 0.55 [−0.10;1.20]) when controlling for end of season differences as well as a slightly larger SD of difference (1.8% cf. 1.4%). End of season body mass was associated with the change in body mass (F = 13.605, P < 0.001, d = 0.94 [0.20; 1.69]).
Physical qualities
Whole-group losses in CMJ (54.3 ± 6.4 cf. 52.1 ± 6.3 cm, t = −14.141, P < 0.001, d = −1.77 [−2.73; −0.81]) and SJ (45.3 ± 4.7 cf. 43.4 ± 4.6 cm, t = −7.330, P < 0.001, d = −0.91 [−1.73; −0.09]) height were observed across the off-season. Controlling for end of season values and the change in body mass, there was minimal positional difference in the loss of jump height from the CMJ (F = 1.230, P = 0.272, d = 0.28 [−0.29;0.86]) and SJ (F = 0.435, P = 0.513, d = 0.20 [−0.36;0.76]) over the off-season period, with the SD of the difference for forwards compared to backs calculated for CMJ = 3.1% cf. 2.2% and SJ = 5.7% cf. 4.3%, respectively. There was small-to-moderate influence of end of season values on the change in CMJ (F = 3.206, P = 0.078, d = 0.46 [−0.16;1.09]) and SJ (F = 4.307, P = 0.044, d = 0.63 [0.05;1.30]). There was a moderate-to-large effect of the change in body mass on the change in CMJ (F = 9.327, P = 0.005, d = 0.75 [0.04;1.45]) and SJ (F = 4.036, P = 0.051, d = 0.61 [−0.06;1.27]). Jump data are shown in .
Figure 1. Changes in (a) squat jump [SJ] and (b) countermovement jump [CMJ] before and after a 10-week off-season for backs (light grey bars) and forwards (dark grey bars). Bars show mean and line represents individual values. Relative changes in (c) squat jump [∆SJ] and d countermovement jump [∆CMJ] for backs (circles) and forwards (squares). Line represents mean % change alongside individual % change values. * main effect for time for both positional groups.
![Figure 1. Changes in (a) squat jump [SJ] and (b) countermovement jump [CMJ] before and after a 10-week off-season for backs (light grey bars) and forwards (dark grey bars). Bars show mean and line represents individual values. Relative changes in (c) squat jump [∆SJ] and d countermovement jump [∆CMJ] for backs (circles) and forwards (squares). Line represents mean % change alongside individual % change values. * main effect for time for both positional groups.](/cms/asset/75cdeb23-d104-4614-906e-5c7451748532/rsmf_a_1959944_f0001_b.gif)
Participants’ sprint times were higher (slower) when returning after the off-season over 10 m (1.71 ± 0.23 cf. 1.77 ± 0.25 s, t = 9.740, P < 0.001, d = 1.21 [0.33;2.10) and 40 m (5.44 ± 0.27 cf. 5.57 ± 0.28 s, t = 9.023, P < 0.001. d = 1.12 [0.26;1.99) (). Sprinting momentum was lower (worse) after the off-season over 10 m (493.6 ± 55.7 cf. 485.9 ± 52.9 kg.m.s−1, t = −3.736, P < 0.01, d = −0.47 [−0.79; −0.14]). The ANCOVA indicated trivial to small differences between forwards and backs for the change in 10 m sprint time (F = 0.248, P = 0.620, d = 0.13 [−0.41; 0.66]), 40 m sprint time (F = 3.264, P = 0.076, d = 0.47 [−0.16; 1.10]) and 10 m momentum (F = 0.398, P = 0.531, d = 0.17 [−0.38; 0.72]). End-of-season values and the change in body mass had a trivial to small influence on the change in 10 m (F = 2.435, P = 0.124, d = 0.41 [−0.21;1.02]; F = 2.320, P = 0.133, d = 0.40 [−0.21;1.01], respectively) and 40 m (F = 0.420, P = 0.519, d = 0.17 [−0.38;0.72]; F = 0.301, P = 0.585, d = 0.14 [−0.40;0.68], respectively) sprint times. There was no influence of end-of-season scores for 10 m momentum (F = 0.008, P = 0.928 d = 0.02 [−0.49;0.53]), though a large effect was observed for the change in body mass (F = 38.073, P < 0.001, d = 1.61 [0.73;2.48]). The SD of difference for forwards compared to backs was calculated for 10 m sprint time (2.8% cf. 2.3%, respectively), 10 m momentum (3.4% cf. 2.6%, respectively) and 40 m sprint time (2.2% cf. 2.8%, respectively). Data are shown in .
Figure 2. Changes in (a) 10 m sprint time, (b) 10 m momentum and (c) 40 m sprint time before and after a 10-week off-season for backs (light grey bars) and forwards (dark grey bars). Bars show mean and line represents individual values. Relative changes in (d) 10 m sprint time [∆time], (e) 10 m momentum [∆momentum] and (f) 40 m sprint time [∆time] for backs (circles) and forward (squares). Line represents mean % change alongside individual % change values. * main effect for time for both positional groups.
![Figure 2. Changes in (a) 10 m sprint time, (b) 10 m momentum and (c) 40 m sprint time before and after a 10-week off-season for backs (light grey bars) and forwards (dark grey bars). Bars show mean and line represents individual values. Relative changes in (d) 10 m sprint time [∆time], (e) 10 m momentum [∆momentum] and (f) 40 m sprint time [∆time] for backs (circles) and forward (squares). Line represents mean % change alongside individual % change values. * main effect for time for both positional groups.](/cms/asset/ec9acb8c-0421-4454-b179-f5434275431d/rsmf_a_1959944_f0002_b.gif)
Final velocity on the 30–15 IFT was lower after the off-season period (19.2 ± 1.2 cf. 18.4 ± 1.2 km.h−1, t = −7.834, P < 0.001, d = −0.97 [−0.14; −1.80]) (), with no positional difference observed when controlling for end-of-season values and the change in body mass (F = 0.346, P = 0.558, d = 0.16 [−0.39;0.70]) with a SD of difference in backs than forwards of 4.6% cf. 3.8%, respectively. The end-of-season 30–15 IFT performance influenced the change observed across the off-season when considering all participants (F = 9.055, P = 0.004, d = 0.71 [0.07; 1.49]) but the change in body mass has minimal effect (F = 0.104, P = 0.748, d = 0.09 [−0.44;0.61]).
Figure 3. Changes in VIFT before and after a 10-week off-season for backs (light grey bars) and forwards (dark grey bars). Bars show mean and line represents individual values. Relative changes in VIFT for backs (circles) and forward (squares). Line represents mean % change alongside individual % change values. * main effect for time for both positional groups.
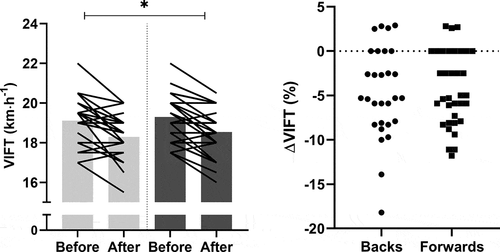
Discussion
A 10-week off-season, where players were not coached directly and training load not monitored, had a detrimental effect on body mass and all physical qualities. These reductions occurred despite players being encouraged to remain physically active and complete a 3-week training programme before the pre-season resumed. Changes were largely independent of playing position except for body mass, and the variability in the responses was greater in forwards for most physical qualities compared to backs. The variability, combined with the influence of end-of-season values on body mass, 10 m momentum and 30–15 IFT, suggests players with higher values can experience greater changes across the off-season.
Body mass over the 10-week off-season increased by an average of 1.7%, while individually there were larger increases of up to 5.5%. These data reaffirm ~1.7% increase in body mass across a 6-week off-season in national rugby union players (Nirmalendran and Ingle Citation2010) and 8-week period in professional rugby league players (Dobbin et al. Citation2020). Whilst changes in body composition remain unclear, it is possible that unsupervised training and a reduced training load accompanied a minimal adjustment of energy intake. Increases in body mass from greater fat mass negatively affect an athlete’s physical capacity and their ability to tolerate higher volumes of training during early preseason (Suarez-Arrones et al. Citation2019). A greater fat mass has also been associated with bone-related injuries in rugby players (Georgeson et al. Citation2012). Practitioners might implement strategies to influence lifestyle and dietary behaviours that help players avoid large increases in body fat mass to mitigate early season injuries and aid a return to training.
Decreases in countermovement and squat jump height of 4.2% and 4.9%, respectively, are indicative of losses in muscle strength and power after detraining (McMaster et al. Citation2013). These changes are larger than reductions reported in national rugby union players (0.8% to 1.6%; Nirmalendran and Ingle Citation2010). Slower detraining of neuromuscular performance in adults might explain the differences between our data and adult players. Neurological adaptations dominate losses in strength in youths where greater muscle cross-sectional area and hormonal adaptations combine with neurological changes to better preserve muscle function in adults (Tsolakis et al. Citation2004). However, values are similar to reductions in jump performance observed in professional soccer players (~6–7% in SJ and ~4–6% in CMJ) after a 6-week off-season comprising 2 weeks abstaining from training and 4 weeks of low-intensity running (Koundourakis et al. Citation2014). Our results indicate a high degree of variability in the response across the off-season. For example, based on a normal distribution, ~34% of forwards reported reductions in countermovement jump height of between 4.9 and 8.0%, whilst a further 13% reported a loss of between 8.1% and 11.1%. When considering the coefficient of variation, a large proportion of athletes in this study had changes greater than the typical error, though few exceeded both error and smallest worthwhile change (>9.1%; Dobbin et al. Citation2018). Nonetheless, this observation suggests a possible need for individualised lower-body resistance programmes in both positional groups, but particularly the forward group. While players verbally reported completing the 3-week off-season training this was not monitored by coaches and might have been subject to differences in adherence. It is also possible that the off-season training load was insufficient to mitigating any detraining effects in some players. These data indicate that an individualised and time-efficient maintenance resistance training programme using exercises with and without the stretch shortening cycle would be an effective strategy during the off-season.
There was a 3.3% and 2.4% increase in 10 m and 40 m sprint times, respectively, which is similar to that reported in other team-sport athletes across a 6–12-week off-season (Clemente et al. Citation2021; Dobbin et al. Citation2020; Jiménez-Reyes et al. Citation2020; Koundourakis et al. Citation2014). The magnitude of change also exceeded the coefficient of variation reported for 10 m (3.05%) and 40 m (1.33%) (Darrall-Jones et al. Citation2016). The change in 10 m sprint times was similar between positions, though the variability of this change was larger in forwards, suggesting a more individualised training approach to the off-season with this group. The change in 10 and 40 m sprint times when controlling for the change in body mass indicates poorer sprint performance was independent of an increase in body mass. A change in biomechanical (e.g., relative force; Jimenez-Reyes et al. Citation2020) or muscle properties (e.g., cross-sectional area and neural activity; McMaster et al. Citation2013) are potential candidates to explain these detraining effects. The associations between sprint ability and injury risk (Malone et al. Citation2018) suggest increases in sprint times might have important implications for injury risk given a higher incidence of hamstring injury in rugby union backs compared to forwards during pre-season training (Brooks et al. Citation2006). Programmes to maintain short- and long-distance sprint ability should be implemented as a potential preventative measure for soft tissue injuries in the return to pre-season training in academy rugby union players.
Large decreases (4.1%, mean reductions of ~1 km·h−1) in the 30–15 IFT peak running speed are greater than the coefficient of variation associated with this test (1.9%; Scott et al. Citation2015) and similar to reductions in intermittent running performance observed in soccer players after a short detraining period (Joo Citation2018). The magnitude of change was lower than the 10.5% reduction in the number of shuttles completed during a continuous 20 m incremental running test to exhaustion in national standard male adult rugby players after a 6-week off-season (Nirmalendran and Ingle Citation2010). While these tests might be influenced by different physical qualities, we speculate that a smaller reduction in shuttle running in our study could be offset by the 3-week off-season training programme included before the return to pre-season. Indeed, the inclusion of an off-season endurance training programme comprising short and long intervals can mitigate the detrimental effects of detraining on intermittent running performance in team sport athletes (Clemente et al. Citation2021). Reductions in intermittent endurance performance are likely explained by adaptations such as slower VO2 kinetics, alterations in oxidative enzymes (e.g. GLUT-4), reduced mitochondrial content and capillary density, higher sub-maximal heart rate, lower stroke volume and a higher respiratory exchange ratio (Mujika and Padilla Citation2000; Thomassen et al. Citation2010; Christensen et al. Citation2011). Increases in body mass (Darrall-Jones et al. Citation2016) and the impairment in sprint performance over the off-season might also have contributed to the reductions in 30–15 IFT peak speed. Regardless of the mechanism, this finding has important implications in moderating injury risk through better handling of high week-to-week training loads on return to pre-season training (Malone et al. Citation2017). Further, research should seek to determine the minimal dose of training required to minimise reductions in intermittent running ability during the off-season in forwards and backs. This training should target those players with better intermittent fitness at the end of the season and consider the mechanism given the changes in 30–15 IFT were independent of the increase in body mass.
The study is not without limitations. The study iused one regional academy, meaning the results are influenced by the playing group studied and to the planning adopted by the coaching staff. Secondly, a priori sample size estimations ranged from 8 to 93 participants for the variables of interest. The restrictions to a single academy meant that only 64 players were recruited, so it is likely that the analyses for selected measurements are underpowered.
Practical implications
Academy rugby union players are likely to detrain over an extended off-season period meaning they will return to a period of high weekly training loads (i.e. pre-season) physically impaired. The off-season for academy players should therefore adopt the minimal training dose possible that maintains or prevents, substantial declines in power, speed, and intermittent running fitness. Coaches might adopt appropriate, non-intrusive monitoring of players’ offseason training (e.g. session-RPE via a mobile application) combined with targeted nutritional and lifestyle education for players. Coaches must also be aware of the between-player variability in physical quality deterioration during the off-season, taking an individualised approach to programme design and monitoring.
Conclusion
Over the 10-week off-season period, body mass of academy rugby union players increased and performance across all measured physical qualities were impaired. There was a high degree of variability between individual players in the response to the off-season, with end of season fitness and playing position influencing physical qualities on the return to training in some players. Practitioners should consider innovative strategies or careful programming that ensure academy rugby union players return to pre-season training rested but ready to meet an increase in training load.
Supplemental Material
Download MS Word (23.5 KB)Disclosure statement
No potential conflict of interest was reported by the author(s).
Supplementary material
Supplemental data for this article can be accessed here.
Additional information
Funding
References
- Argus CK, Gill ND, Keogh JWL. 2012. Characterization of the differences in strength and power between different levels of competition in rugby union athletes. Journal of Strength and Conditioning Research. 26(10):2698–2704. doi:10.1519/JSC.0b013e318241382a.
- Brooks JHM, Fuller CW, Kemp SPT, Reddin DB. 2006. Incidence, risk, and prevention of hamstring muscle injuries in professional rugby union. American Journal of Sports Medicine. 34(8):1297–1306. doi:10.1177/0363546505286022.
- Buchheit M. 2008. The 30-15 intermittent fitness test: accuracy for individualizing interval training of young intermittent sport players. Journal of Strength and Conditioning Research. 22(2):365–374. doi:10.1519/JSC.0b013e3181635b2e.
- Caldwell BP, Peters DM. 2009. Seasonal variation in physiological fitness of a semiprofessional soccer team. Journal of Strength and Conditioning Research. 23(5):1370–1377. doi:10.1519/JSC.0b013e3181a4e82f.
- Casserly N, Neville R, Ditroilo M, Grainger A. 2020. Longitudinal changes in the physical development of elite adolescent rugby union players: effect of playing position and body mass change. Int J Sports Physiol Perform. 15(4):520–527. doi:10.1123/ijspp.2019-0154.
- Christensen PM, Krustrup P, Gunnarsson TP, Kiilerich K, Nybo L, Bangsbo J. 2011. VO2 kinetics and performance in soccer players after intense training and inactivity. Med Sci Sports Exerc. 43(9):1716–1724. doi:10.1249/mss.0b013e318211c01a.
- Clemente FM, Ramirez-Campillo R, Sarmento H. 2021. Detrimental effects of the off-season in soccer players: a systematic review and meta-analysis. Sports Medicine. 51(4):795–814. doi:10.1007/s40279-020-01407-4.
- Darrall-Jones J, Roe G, Carney S, Clayton R, Phibbs P, Read D, Weakley J, Till K, Jones B. 2016. The effect of body mass on the 30-15 intermittent fitness test in rugby union players. Int J Sports Physiol Perform. 11(3):400–403. doi:10.1123/ijspp.2015-0231.
- Dobbin N, Clarke J, Cushman S. 2020. The effects of an 8-week off-season period on the mechanical properties of sprinting in professional rugby league players: implications for training recommendations. Journal of Trainology. 9(1):15–19. doi:10.17338/trainology.9.1_15.
- Dobbin N, Highton J, Moss SL, Twist C. 2019. Factors affecting the anthropometric and physical characteristics of elite academy rugby league players: a multiclub study. Int J Sports Physiol Perform. 14(7):958–965. doi:10.1123/ijspp.2018-0631.
- Dobbin N, Hunwicks R, Highton J, Twist C. 2017. Validity of a jump mat for assessing countermovement jump performance in elite rugby players. Int J Sports Med. 38(2):99–104. doi:10.1055/s-0042-118313.
- Dobbin N, Hunwicks R, Highton J, Twist C. 2018. A reliable testing battery for assessing physical qualities of elite academy rugby league players. Journal of Strength and Conditioning Research. 32(11):3232–3238. doi:10.1519/JSC.0000000000002280.
- Faul F, Erdfelder E, Lang A-G, Buchner A. 2007. G*power 3: a flexible statistical power analysis program for the social, behavioral, and biomedical sciences. Behav. Res. Methods. 39(2):175–191.
- Georgeson EC, Weeks BK, McLellan C, Beck BR. 2012. Seasonal change in bone, muscle and fat in professional rugby league players and its relationship to injury: a cohort study. BMJ Open. 2(6):e001400. doi:10.1136/bmjopen-2012-001400.
- Girardi M, Casolo A, Nuccio S, Gattoni C, Capelli C. 2020. Detraining effects prevention: a new rising challenge for athletes. Front Physiol. 11. doi:10.3389/fphys.2020.588784.
- Hartwig TB, Naughton G, Searl J. 2009. Load, stress, and recovery in adolescent rugby union players during a competitive season. J Sports Sci. 27(10):1087–1094. doi:10.1080/02640410903096611.
- Jensen CD, Gleason D, Vanness M. 2018. Four-week unstructured break improved athletic performance in collegiate rugby players. Journal of Strength and Conditioning Research. 32(6):1671–1677. doi:10.1519/jsc.0000000000002417.
- Jiménez-Reyes P, Garcia-Ramos A, Párraga-Montilla JA, Morcillo-Losa JA, Cuadrado-Peñafiel V, Castaño-Zambudio A, Morin JB, et al. 2020. Seasonal changes in the sprint acceleration force-velocity profile of elite male soccer players. Journal of Strength and Conditioning Research. 10.
- Joo CH.2018. The effects of short term detraining and retraining on physical fitness in elite soccer players. PLoS ONE. 13(5):e0196212. doi:10.1371/journal.pone.0196212.
- Koundourakis NE, Androulakis NE, Malliaraki N, Tsatsanis C, Venihaki M, Margioris AN, Novelli G. 2014. Discrepancy between exercise performance, body composition, and sex steroid response after a six-week detraining period in professional soccer players. PLoS ONE. 9(2):2. doi:10.1371/journal.pone.0087803.
- Malone S, Owen A, Mendes B, Hughes B, Collins K, Gabbett TJ. 2018. High-speed running and sprinting as an injury risk factor in soccer: can well-developed physical qualities reduce the risk? Journal of Science and Medicine in Sport. 21(3):257–262. doi:10.1016/j.jsams.2017.05.016.
- Malone S, Roe M, Doran DA, Gabbett TJ, Collins K. 2017. High chronic training loads and exposure to bouts of maximal velocity running reduce injury risk in elite Gaelic football. Journal of Science and Medicine in Sport. 20(3):250–254. doi:10.1016/j.jsams.2016.08.005.
- Manley A, Williams S. 2019. ‘We’re not run on numbers, we’re people, we’re emotional people’: exploring the experiences and lived consequences of emerging technologies, organizational surveillance and control among elite professionals. Organization, 1350508419890078.
- McMaster DT, Gill N, Cronin J, McGuigan M. 2013. The development, retention and decay rates of strength and power in elite rugby union, rugby league and american football: a systematic review. Sports Medicine. 43(5):367–384. doi:10.1007/s40279-013-0031-3.
- Melchiorri G, Ronconi M, Triossi T, Viero V, De Sanctis D, Tancredi V, Salvati A, Padua E, Alvero Cruz JR. 2014. Detraining in young soccer players. Journal of Sports Medicine and Physical Fitness. 54(1):27–33.
- Mujika I, Padilla S. 2000. Detraining: loss of training-induced physiological and performance adaptations. Part I. Sports Medicine. 30(2):79–87. doi:10.2165/00007256-200030020-00002.
- Nirmalendran R, Ingle L. 2010. Detraining effect of the post-season on selected aerobic and anaerobic performance variables in national league rugby union players: a focus on positional status. Medicina Sportiva. 14(4):161–168. doi:10.2478/v10036-010-0026-1.
- Requena B, García I, Suárez-Arrones L, Sáez De Villarreal E, Naranjo Orellana J, Santalla A. 2017. Off-season effects on functional performance, body composition, and blood parameters in top-level professional soccer players. Journal of Strength and Conditioning Research. 31(4):939–946. doi:10.1519/JSC.0000000000001568.
- Scott TJ, Delaney JA, Duthie GM, Sanctuary CE, Ballard DA, Hickmans JA, Dascombe BJ. 2015. Reliability and usefulness of the 30–15 intermittent fitness test in rugby league. Journal of Strength & Conditioning Research. 29(7):1985–1990.
- Silva JR, Brito J, Akenhead R, Nassis GP. 2016. The transition period in soccer: a window of opportunity. Sports Medicine. 46(3):305–313. doi:10.1007/s40279-015-0419-3.
- Smart DJ, Gill ND. 2013. Effects of an off-season conditioning program on the physical characteristics of adolescent rugby union players. Journal of Strength and Conditioning Research. 27(3):708–717. doi:10.1519/JSC.0b013e31825d99b0.
- Sotiropoulos A, Travlos AK, Gissis I, Souglis AG, Grezios A. 2009. The effect of a 4-week training regimen on body fat and aerobic capacity of professional soccer players during the transition period. Journal of Strength and Conditioning Research. 23(6):1697–1703. doi:10.1519/JSC.0b013e3181b3df69.
- Suarez-Arrones L, Lara-Lopez P, Maldonado R, Torreno N, De Hoyo M, Nakamura FY, Di SV, Mendez-Villanueva A. 2019. The effects of detraining and retraining periods on fat-mass and fat-free mass in elite male soccer players. PeerJ. 2019(8). doi:10.7717/peerj.7466.
- Thomassen M, Christensen PM, Gunnarsson TP, Nybo L, Bangsbo J. 2010. Effect of 2-wk intensified training and inactivity on muscle Na +-K+ pump expression, phospholemman (FXYDI) phosphorylation, and performance in soccer players. J Appl Physiol. 108(4):898–905. doi:10.1152/japplphysiol.01015.2009.
- Tsolakis CK, Vagenas GK, Dessypris AG. 2004. Strength adaptations and hormonal responses to resistance training and detraining in preadolescent males. Journal of Strength and Conditioning Research. 18(3):625–629. doi:10.1519/1533-4287(2004)18<625:SAAHRT>2.0.CO;2.
- Vassilis S, Yiannis M, Athanasios M, Dimitrios M, Ioannis G, Thomas M. 2019. Effect of a 4-week detraining period followed by a 4-week strength program on isokinetic strength in elite youth soccer players. Journal of Exercise Rehabilitation. 15(1):67–73. doi:10.12965/jer.1836538.269.
- Wood DJ, Coughlan GF, Delahunt E. 2018. Fitness profiles of elite adolescent irish rugby union players. Journal of Strength and Conditioning Research. 32(1):105–112. doi:10.1519/JSC.0000000000001694.