Abstract
Successive Fenton oxidation (up to three times) was conducted to enhance the removal efficiency of nine USEPA priority PAHs in aged soil highly contaminated with PAHs. Results showed that PAHs concentrations decreased rapidly and the removal efficiency was relatively high during one-time Fenton oxidation. However, PAHs in soil exhibited a slow removal trend and decreased at an extremely slow rate during two- and three-times Fenton oxidation. Surfactant Tween 80 was used to increase the amount of PAHs available for hydroxyl radical (·OH) and enhance Fenton oxidation efficiency of PAHs in aged soil pre-treated with Fenton reagents in this study. Results showed that little effect of Tween 80 on desorption of nine USEPA priority PAHs was observed in treatment with low dose of Tween 80 (800 mg L−1). However, high dose of Tween 80 (6400 mg L−1) could improve desorption of 3- and 4-PAHs (10–18%) in soil pre-treated with Fenton reagents, therefore, Tween 80 could significantly improve degradation efficiency of 3- and 4-PAHs relative to control (13–22%). The results in this study offer a possible alternative to the removal of PAHs from soils.
1. Introduction
Polycyclic aromatic hydrocarbons (PAHs) are a class of organic compounds with two or more fused benzene rings in linear, angular or cluster structural arrangements (Antizar-Ladislao, Lopez-Real, & Beck, Citation2004; Rivas, Citation2006). They are wide-spread environmental contaminants that are mainly formed during the incomplete combustion of fossil fuels (Antizar-Ladislao et al., Citation2004; Jonsson et al., Citation2007; Rivas, Citation2006). Industrial sites associated with petroleum refining, manufactured gas plants, wood treatment facilities, steel-making factories, coking plants and thermal power plants are often highly contaminated with PAHs (Jonsson et al., Citation2007; Lemaire, Buès, Kabeche, Hanna, & Simonnot, Citation2013). PAHs are very persistent in soils due to their properties, such as low volatility, low water solubility and low biodegradability (López-Vizcaíno, Sáez, Cañizares, & Rodrigo, Citation2012). PAHs have been recognized as a potential health risk due to their bioaccumulation and potential toxicity to humans and wildlife (Lemaire et al., Citation2013; Rivas, Citation2006). Therefore, remediation of soil contaminated with PAHs has received great attention (Antizar-Ladislao et al., Citation2004; Gan, Yap, & Ng, Citation2013; Jonsson et al., Citation2007; Lemaire et al., Citation2013; López-Vizcaíno et al., Citation2012; Rivas, Citation2006). To remediate and restore functions of soil polluted by PAHs, effective technologies are necessary.
Over the last two decades, Fenton treatment has emerged as a promising remediation technology for PAHs-polluted soils (Gan, Lau, & Ng, Citation2009; Gan et al., Citation2013; Lemaire et al., Citation2013; Martens & Frankenberger, Citation1995; Rosas, Vicente, Santos, & Romero, Citation2013; Saxe, Allen, & Nicol, Citation2000; Venny, Gan, & Ng, Citation2012; Yap, Gan, & Ng, Citation2011). Fenton oxidation processes are environmentally clean technologies which depend on the formation of reactive and non-selective hydroxyl (·OH) radicals for oxidation (Diyáuddeen, Aziz, & Daud, Citation2012). The generated ·OH radicals can aggressively react with virtually all organic compounds (Munter, Citation2001). However, there are many limitations in applying sole Fenton oxidation to remediate soils polluted with PAHs (Gan et al., Citation2013; Martens & Frankenberger, Citation1995; Rosas et al., Citation2013; Saxe et al., Citation2000; Yap et al., Citation2011). A major challenge in Fenton treatment of PAHs-contaminated soils is the limited mass transfer of PAHs from the sorbed phase into the aqueous phase due to their strong sorption and low aqueous solubility (Yap et al., Citation2011). Limited mass transfer results in limited PAH available for the hydroxyl radical (·OH) which was generated in the aqueous phase (Venny et al., Citation2012). Therefore, integrating solubulizing agents (such as, co-solvent, surfactant, cyclodextrin and vegetable oil) with Fenton treatment is a possible alternative (Gan et al., Citation2009; Martens & Frankenberger, Citation1995; Rosas et al., Citation2013; Saxe et al., Citation2000; Venny et al., Citation2012; Yap et al., Citation2011).
Surfactants consist of organic molecules with hydrophobic and hydrophilic parts and can interact with polar and non-polar surfaces. At low concentrations in aqueous solution, single molecules (i.e., monomers) are present. However, the surfactant molecules will aggregate, form micelles and reduce the thermodynamic energy in the system at above a certain concentration (Ahn, Kim, Woo, & Park, Citation2008). These micelles lead to the increased pseudo-water-solubilities of hydrophobic organic compounds (HOCs), therebyincreasing the concentration gradient and mass transfer rates (Rosas et al., Citation2013). Many studies have observed that surfactants can facilitate the solubility and Fenton oxidation of HOCs (i.e., PAHs, TNT and p-Cresol) in soil (Li et al., Citation1997; Martens & Frankenberger, Citation1995; Rosas et al., Citation2013; Saxe et al., Citation2000; Wang, Hoag, Collins, & Naidu, Citation2013), and surfactants usually were introduced as a pre-treatment step before Fenton oxidation in these studies. However, there is a lack of knowledge about the potential of surfactant Tween 80 to enhance the Fenton oxidation of PAHs in aged soil pre-treated with Fenton reagents.
Apparent removal of PAHs had been observed in soil after Fenton oxidation in many studies (Martens & Frankenberger, Citation1995; Nam, Rodriguez, & Kukor, Citation2001; Saxe et al., Citation2000; Valderrama et al., Citation2009), but significant amount of PAHs in soil was also remained after applying sole Fenton oxidation in their studies. Therefore, it is necessary to further reduce concentration of PAHs after applying sole Fenton oxidation to remediate soils contaminated with PAHs. However, PAHs are low water solubility, which might impede Fenton oxidation of PAHs in soils (Yap et al., Citation2011). Surfactants can increase solubility of PAHs, thereby increasing the concentration gradient and mass transfer rates (Rosas et al., Citation2013). Therefore, it may be a possible alternative that applying surfactants to enhance desorption and Fenton oxidation of PAHs in soil pre-treated with Fenton reagents.
The objectives of this study were to: (1) investigate the efficiency of successive Fenton oxidation (up to three times) on the removal of PAHs; (2) investigate the effect of surfactant Tween 80 on desorption and Fenton oxidation of PAHs in an aged soil (from abandoned steel-making factories) pre-treated with Fenton reagents.
2. Materials and methods
2.1. Chemicals and reagents
All solvents used for sample processing and analysis (acetone, dichloromethane) were HPLC grade from J.T. Baker Chemical Company (USA). Anhydrous sodium sulphate (Na2SO4, AR, Beijing Chemical Factory, China) was oven-dried at 450 °C for 6 h to act as desiccant. Hydrogen peroxide (30%, w/w), FeSO4·7H2O and surfactant Tween 80 were obtained from Beijing Chemical Factory, China. Standard solutions of PAHs were purchased from Accustandard Inc, which, including naphthalene (NAP), acenaphthylene (ANY), acenaphthene (ACE), fluorine (FLU), phenanthrene (PHE), anthracene (ANT), fluoranthene (FLT), pyrene (PYR), benzo(a)anthracene (BaA), chrysene (CHR), benzo(b)fluoranthene (BbFA), benzo(k)fluoranthene (BkFA), benzo(a)pyrene (BaP), indeno(1,2,3,-cd)pyrene (IPY), dibenzo(a,h)anthracene (DBA) and benzo(ghi)perylene (BPE).
2.2. PAH contaminated soil
Sub-surface soil contaminated with PAHs at depths ranging from 3 to 4 m below ground surface was collected from abandoned steel-making factory in Beijing, China. The steel-making factory started in 1950s, and closed in 2007. Upon collection, the soil samples were homogenized and sieved (2 mm) to assure uniformity, and stored in a refrigerator (4 °C) until used. The soil was characterized as: sand soil (87.88% sand, 10.18% silt and 1.94% clay), pH 8.07, total organic carbon 16.33 g kg−1, total iron 3.05% and moisture 7.26%. The initial concentrations of PAHs are shown in Table . ACE, FLU, PHE, FLT, PRY, CHR, BbFA, BaP and DBA were the main components of PAHs in soil used in this study, which accounted for 98% of the total amount of PAHs.
Table 1. Some chemical properties of the 16 polycyclic aromatic hydrocarbons (PAHs) and concentration of PAHs in soil used in this study. Average n = 3 ± s.d.
2.3. Experimental procedure
To evaluate the effect of Fenton reagents on PAHs oxidation, experiments were carried out in 250 mL flasks with 15 g of fresh soil (wet weight) and 34 mL deionized water. Then, Fenton reagents contained FeSO4·7H2O solution (446 mmol L−1) and hydrogen peroxide (30%, w/w) were applied to flasks (except for control). Four treatments were then initiated: (1) zero-time Fenton oxidation, no FeSO4·7H2O solution and no H2O2 were added; (2) one-time Fenton oxidation, 2 mL FeSO4·7H2O solution and 3.33 mL H2O2 were added; (3) two-times Fenton oxidation, 4 mL FeSO4·7H2O solution and 6.66 mL H2O2 were added; (4) three-times Fenton oxidation, 6 mL FeSO4·7H2O solution and 10 mL H2O2 were added up. Each treatment included 3three replicates. The experimental setups are summarized in Table . The soil samples in the flasks were placed in an orbital shaking incubator (100 rpm min−1) at 25 °C in the dark. Soil mud in flasks was filtered after 6 h of incubation and soil was wrapped in pre-cleaned aluminium foil and immediately stored at −20 °C until analysis.
Table 2. Parameters and conditions used in our experiments. Reagents (surfactant Tween 80, Fenton reagents or deionized water) were added at 0 h, 2 h, 4 h and 12 h. The experiments were performed in 250 mL glass flask at 25 °C in orbital shaking incubator (100 rpm min−1).
To evaluate the effect of surfactant Tween 80 on desorption and Fenton oxidation efficiency of PAHs, both desorption and Fenton oxidation experiments were carried out in 250 mL flasks. The experimental setups also are summarized in Table . Fifteen grams fresh soil (wet weight) and 34 mL deionized water were applied to each flask. Then 2 mL FeSO4·7H2O (446 mmol L−1) and 3.33 mL H2O2 (30%, w/w) were added to each flask. After two hours, this procedure was repeated. After four hours, 10 mL Tween 80 solution was transferred to flasks to make the system contain 0, 800 and 6400 mg L−1 Tween 80, respectively. For desorption experiments, the flasks were hermetically sealed and incubated at 25 °C in an orbital shaking incubator (100 rpm min−1) for 8 h to reach soil–liquid equilibrium. The mud in the flasks was filtered to obtain soil water solution. The soil water solution was then filtered under vacuum through pre-ashed glass fibre filters (Whatman, GF/F) to obtain dissolved samples. For Fenton oxidation experiments, additional 3.33 mL H2O2 and 2 mL FeSO4·7H2O (446 mmol L−1) were applied to the flasks to further reduce concentration of PAHs. Then the mud in the flasks were filtered to obtain the soil, which was wrapped in pre-cleaned aluminium foil and immediately stored at −20 °C until analysis.
2.4. PAH extraction and analysis
PAHs of soil samples were characterized using modified mechanical shaking method (Schwab, Su, Wetzel, Pekarek, & Banks, Citation1999). Briefly, 5 g soil sample blended with moderate anhydrous Na2SO4, was then extracted for 1 h in 100 mL acetone/dichloromethane (1:1, v/v) in an orbital shaking incubator (200 rpm min−1). This procedure was repeated, and the two extracts were combined and dehydrated using anhydrous Na2SO4. Water samples were extracted using a liquid-liquid extraction (LLE) method (Fernandez, Garcia, Garcia-Villanova, & Gomez, Citation1996). Water sample (30 mL) was extracted successively with three volumes of 60 mL dichloromethane. The three extracts were collected and dehydrated using anhydrous Na2SO4. The combined extracts were concentrated in eggplant-type bottle in a vacuum rotary evaporator at a temperature below 40 °C.
The identification and quantification of PAHs in the extracts were accomplished by gas chromatograph mass spectrometer (GC–MS) (Thermo Trace DSQII) using a DB-5MS capillary column (30 m × 0.32 mm id, 0.25 μm film thickness; Varian, Walnut Creek, CA, USA). Helium (99.999%) was used as the GC carrier gas at a constant flow of 1.5 mL min−1. The injected volume was 1 μL in split mode. For the GC/MS, the injection port, interface line and ion temperature were maintained at 300 °C, 300 °C and 275 °C, respectively. The column temperature was programmed at 40 °C and held for 5 min, then increased at 10 °C min−1 to 280 °C and held for 4 min, 10 °C min−1 to 300 °C and held for 5 min. The quantification of PAHs was accomplished by a six-point internal calibration curve using peak area. A mix of deuterated PAH containing naphthalene-d8, anthracene-d10, phenanthrene-d10, crysene-d12 and perylene-d12 (Accustandard Inc.) was used as internal standard.
3. Results and discussion
3.1. Sole Fenton oxidation of PAHs in aged soil
The apparent removal of PAHs had not been observed in the control treatment without Fenton reagents (Figure ), which presumably represents the result of natural degradation by microbial action or variability in PAHs concentration. The percentage removal of PAHs was less than 10%. After one-time Fenton oxidation, the addition of Fenton reagents could significantly facilitate oxidation of 3- and 4-ring PAHs, while the addition of Fenton reagents could facilitate the oxidation of 5-rings PAHs to a certain extent (Figure ). The recalcitrance of PAHs increases with increasing number of aromatic rings and thus molecular weight is an important reason (Henner, Schiavon, Morel, & Lichtfouse, Citation1997). Furthermore, the transfer of low molecular weight (LMW) PAHs from the soil is known to be more rapid than HMW PAHs due to the strong sorption of high molecular weight (HMW) PAHs onto microporous of particulates and their hydrophobicity (high Kow) (Rivas, Citation2006; Venny et al., Citation2012).
Figure 1. Changes of PAHs concentration in contaminated soil before Fenton oxidation (untreated soil) and after Fenton oxidation (zero-time, one-time, two-times and three-times oxidation) under soil/slurry conditions. Two mL FeSO4·7H2O solution (446 mmol L−1) and 3.33 mL hydrogen peroxide (30%, w/w) are added to soil/slurry system in each oxidation process. Error bars indicate standard deviations (n = 3). The different letters indicate significant differences (p < 0.05).
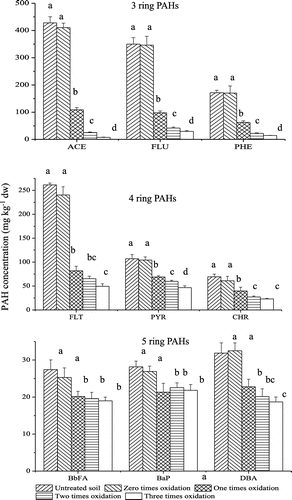
Two-times Fenton oxidation enhanced the percentage oxidation of 3- and 4-ring PAHs to a certain extent, but could not further significantly enhance Fenton oxidation of 5-ring PAHs in this study (p < 0.05) (Figure ). Compared with two-times Fenton oxidation, apparent removal of 3-, 4- and 5-ring PAHs was not observed after three-times Fenton oxidation (Figure ). In a word, during one-time Fenton oxidation, PAHs concentrations decreased rapidly and the removal efficiency was relatively high. Conversely, during two- and three-times Fenton oxidation, the PAHs in soil exhibited a slow removal trend and decreased at an extremely slow rate. It is evident that the removal of PAHs has the “drag tail” phenomenon during three-time Fenton oxidation.
In classical Fenton reaction, when H2O2 is present in excess, more ·OH radical is available to react with the contaminants (Venny et al., Citation2012). In this study, 2 mL FeSO4·7H2O (446 mmol L−1) and 3.33 mL H2O2 (30%, w/w) were added to each flask in each oxidation process, the mass ratio of hydrogen peroxide and total PAHs in soil is greater than seventy. Thus, H2O2 is present in excess and there are enough ·OH radicals to oxidize PAHs in soil. However, the removal of PAHs has the “drag tail” phenomenon during three-time Fenton oxidation. The limited mass transfer of PAHs from the sorbed phase into the aqueous phase might be an important factor because limited mass transfer results in limited PAH available for the hydroxyl radical (·OH) which was generated in the aqueous phase (Yap et al., Citation2011).
3.2. Effect of Tween 80 on desorption and oxidation of PAHs in pre-treated soil
Surfactant enhanced remediation (SER) is thought to be an effective, economic and quick method for the remediation of soils polluted with hydrophobic organic compounds (Chong et al., Citation2014). Many studies have observed that surfactants can facilitate the solubility and Fenton oxidation of PAHs in soil markedly (Martens & Frankenberger, Citation1995; Rosas et al., Citation2013; Saxe et al., Citation2000). Surfactants were introduced as a pre-treatment step with Fenton oxidation, and soil was not pre-treated with Fenton reagents in their studies. In this study, surfactant Tween 80 could not significantly improve the Fenton oxidation efficiency of 5-ring PAHs in soil was pre-treated with Fenton reagents in this study (p < 0.05), but enhanced the Fenton oxidation efficiency of 3-, and 4- ring PAHs (13–22%) (Figure ).
Figure 2. Changes of PAHs concentration in pre-oxidation soil as affected by Fenton oxidation alone or in combination with Tween 80 (0, 800 and 6400 mg L−1, respectively). Error bars indicate standard deviations (n = 3). The different letters indicate significant differences (p < 0.05).
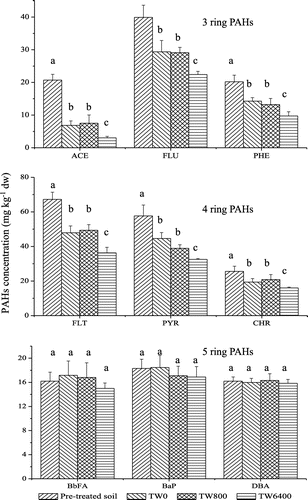
Desorption experiments results in this study, showed that little effect of surfactant Tween 80 on the desorption of ACE, FLU, PHE, FLT, PRY, CHR, BbFA, BaP and DBA was observed in treatment with low dose of Tween 80 (800 mg L−1) (Table ). High dose of Tween 80 (6400 mg L−1) had little effect on the 5-ring PAHs desorption (<1%) (Table ), but facilitated desorption of 3- and 4-ring PAHs to a certain extent in this study (10–18%) (Table ). However, the desorption rate of PAHs in this study is lower than that of the previous reports, (Ahn et al., Citation2008; Chong et al., Citation2014; López-Vizcaíno et al., Citation2012; Peng, Wu, & Chen, Citation2011; Zhou & Zhu, Citation2008) in which the soil was not pre-treated with Fenton reagents. More than 80% of PAHs in soils could be removed by surfactants in their studies under suitable conditions.
Table 3. The desorption ratios (%) of PAHs from aged soil pre-treated with Fenton reagents into water solution after surfactant Tween 80 added with different concentrations (0, 800 and 6400 mg L−1 respectively) was used to accelerate the desorption of PAHs in soil. Average n = 3 ± s.d.
Ageing of hydrophobic organic contaminants (HOCs) is an important attenuation process which often entails an initially rapid and reversible sorption process followed by a period of slow diffusion occurring over weeks, months or even years when they released into the environment (Alexander, Citation2000; Luo, Lin, Huang, & Zhang, Citation2012). Ageing of hydrophobic organic contaminants (HOCs) leads to three distinct contaminant “pools,” equating to “rapid,” “slow” and “very slow” desorption domains (Bosma, Middeldorp, Schraa, & Zehnder, Citation1996). This behaviour is thermodynamically driven, with movement towards the very-slow domain (Cornelissen, van Noort, Parsons, & Govers, Citation1997). Therefore, high desorption rate of PAHs may be observed in soil was not pre-treated with Fenton reagents, while an even much stronger effect of resistant desorption is expected in soil after Fenton oxidation due to the reason that strongly sorbed pollutants tend to be more resistant to oxidation and vice versa (Venny et al., Citation2012). In this study, although the desorption rate of PAHs is lower than that of the previous reports (Ahn et al., Citation2008; Chong et al., Citation2014; López-Vizcaíno et al., Citation2012; Peng et al., Citation2011; Zhou & Zhu, Citation2008) in which the soil was not pre-treated with Fenton reagents, high dose of Tween 80 (6400 mg L−1) could improve desorption of 3- and 4-PAHs (10–18%) in soil pre-treated with Fenton reagents, therefore improve the degradation efficiency of 3- and 4-PAHs relative to control (13–22%), which offer a possible alternative to the removal of PAHs from soils.
4. Conclusion
In this study, successive Fenton oxidation (up to three times) was conducted to enhance the removal efficiency of nine USEPA priority PAHs (ACE, FLU, PHE, FLT, PRY, CHR, BbFA, BaP and DBA) in aged soil highly contaminated with PAHs. Results showed that the removal of PAHs has the “drag tail” phenomenon during three-time Fenton oxidation. In order to increase desorption of PAHs in soil and increase the amount of PAH available for the hydroxyl radical (·OH), the application of surfactant Tween 80 was investigated to accelerate desorption and Fenton oxidation of PAH in soil after two times Fenton oxidation in this study. Results showed that little effect of Tween 80 on desorption of ACE, FLU, PHE, FLT, PRY, CHR, BbFA, BaP and DBA was observed in treatment with low dose of Tween 80 (800 mg L−1). Although the desorption rate of PAHs in this study is lower than that of the previous reports in which the soil was not pre-treated with Fenton reagents, high dose of Tween 80 (6400 mg L−1) could improve desorption of 3- and 4-PAHs (10–18%) in soil pre-treated with Fenton reagents, therefore Tween 80 could significantly improve degradation efficiency of 3- and 4-PAHs relative to control (13–22%).
Disclosure statement
No potential conflict of interest was reported by the authors.
Funding
This work was supported by the National Natural Science Foundation of China [grant number 41201516]; China National Special Research Fund for Non-Profit Sector of Environmental Protection [grant number 201409047].
References
- Ahn, C. K., Kim, Y. M., Woo, S. H., & Park, J. M. (2008). Soil washing using various nonionic surfactants and their recovery by selective adsorption with activated carbon. Journal of Hazardous Materials, 154, 153–160.10.1016/j.jhazmat.2007.10.006
- Alexander, M. (2000). Aging, bioavailability, and overestimation of risk from environmental pollutants. Environmental Science & Technology, 34, 4259–4265.10.1021/es001069+
- Antizar-Ladislao, B., Lopez-Real, J. M., & Beck, A. J. (2004). Bioremediation of polycyclic aromatic hydrocarbon (PAH)-contaminated waste using composting approaches. Critical Reviews in Environmental Science and Technology, 34, 249–289.10.1080/10643380490434119
- Bosma, T. N. P., Middeldorp, P. J. M., Schraa, G., & Zehnder, A. J. B. (1996). Mass transfer limitation of biotransformation: Quantifying bioavailability. Environmental Science and Technology, 31, 248–252.
- Chong, Z. Y., Liao, X. Y., Yan, X. L., Sun, L., Zhao, D., & Liang, T. (2014). Enhanced desorption of PAHs from manufactured gas plant soils using different types of surfactants. Pedosphere, 24, 209–219.10.1016/S1002-0160(14)60007-2
- Cornelissen, G., van Noort, P. C. M., Parsons, J. R., & Govers, H. A. J. (1997). Temperature dependence of slow adsorption and desorption kinetics of organic compounds in sediments. Environmental Science & Technology, 31, 454–460.10.1021/es960300+
- Diyáuddeen, B. H., Aziz, A. R. A., & Daud, W. M. A. W. (2012). Oxidative mineralisation of petroleum refinery effluent using Fenton-like process. Chem Eng Res Des., 90, 298–307.
- Fernandez, M. J., Garcia, C., Garcia-Villanova, R. J., & Gomez, J. A. (1996). Evaluation of liquid−solid extraction with a new sorbent and liquid−liquid extraction for multiresidue pesticides. determination in raw and finished drinking waters. Journal of Agricultural and Food Chemistry, 44, 1790–1795.10.1021/jf9405766
- Gan, S., Lau, E. V., & Ng, H. K. (2009). Remediation of soils contaminated with polycyclic aromatic hydrocarbons (PAHs). Journal of Hazardous Materials, 172, 532–549.10.1016/j.jhazmat.2009.07.118
- Gan, S., Yap, C. L., & Ng, H. K. (2013). Investigation of the impacts of ethyl lactate based Fenton treatment on soil quality for polycyclic aromatic hydrocarbons (PAHs)-contaminated soils. Journal of Hazardous Materials, 262, 691–700.10.1016/j.jhazmat.2013.09.023
- Henner, P., Schiavon, M., Morel, J. L., & Lichtfouse, E. (1997). Polycyclic aromatic hydrocarbon (PAH) occurrence and remediation methods. Analusis, 25, 56–59.
- Jonsson, S., Persson, Y., Frankki, S., van Bavel, B., Lundstedt, S., & Haglund, P. (2007). Degradation of polycyclic aromatic hydrocarbons (PAHs) in contaminated soils by Fenton’s reagent: A multivariate evaluation of the importance of soil characteristics and PAH properties. Journal of Hazardous Materials, 149, 86–96.10.1016/j.jhazmat.2007.03.057
- Lemaire, J., Buès, M., Kabeche, T., Hanna, K., & Simonnot, M. O. (2013). Oxidant selection to treat an aged PAH contaminated soil by in situ chemical oxidation. Journal of Environmental Chemical Engineering, 1, 1261–1268.10.1016/j.jece.2013.09.018
- Lemaire, J., Laurent, F., Leyval, C., Schwartz, C., Buès, M., & Simonnot, M. O. (2013). PAH oxidation in aged and spiked soils investigated by column experiments. Chemosphere, 91, 406–414.10.1016/j.chemosphere.2012.12.003
- Li, Z. M., Peterson, M. M., Comfort, S. D., Horst, G. L., Shea, P. J., & Oh, B. T. (1997). Remediating TNT-contaminated soil by soil washing and Fenton oxidation. Science of The Total Environment, 204, 107–115.10.1016/S0048-9697(97)00158-7
- López-Vizcaíno, R., Sáez, C., Cañizares, P., & Rodrigo, M. A. (2012). The use of a combined process of surfactant-aided soil washing and coagulation for PAH-contaminated soils treatment. Separation and Purification Technology, 88, 46–51.10.1016/j.seppur.2011.11.038
- Luo, L., Lin, S., Huang, H., & Zhang, S. (2012). Relationships between aging of PAHs and soil properties. Environmental Pollution, 170, 177–182.10.1016/j.envpol.2012.07.003
- Martens, D. A., & Frankenberger, W. T. (1995). Enhanced degradation of polyaromatic hydrocarbons in soil treated with an advanced oxidative process-Fenton’s reagent. Journal of Soil Contamination, 4, 175–190.
- Munter, R. (2001). Advanced oxidation processes-current status and prospects. Proceedings of the Estonian Academy of Sciences, Chemistry, 32, 91–101.
- Nam, K., Rodriguez, W., & Kukor, J. J. (2001). Enhanced degradation of polycyclic aromatic hydrocarbons by biodegradation combined with a modified Fenton reaction. Chemosphere, 45, 11–20.10.1016/S0045-6535(01)00051-0
- Peng, S., Wu, W., & Chen, J. J. (2011). Removal of PAHs with surfactant-enhanced soil washing: Influencing factors and removal effectiveness. Chemosphere, 82, 1173–1177.10.1016/j.chemosphere.2010.11.076
- Rivas, F. J. (2006). Polycyclic aromatic hydrocarbons sorbed on soils: A short review of chemical oxidation based treatments. Journal of Hazardous Materials, 138, 234–251.10.1016/j.jhazmat.2006.07.048
- Rosas, J. M., Vicente, F., Santos, A., & Romero, A. (2013). Soil remediation using soil washing followed by Fenton oxidation. Chemical Engineering Journal, 220, 125–132.10.1016/j.cej.2012.11.137
- Saxe, J. K., Allen, H. E., & Nicol, G. R. (2000). Fenton oxidation of polycyclic aromatic hydrocarbons after surfactant-enhanced soil washing. Environmental Engineering Science, 17, 233–244.10.1089/10928750050137589
- Schwab, A. P., Su, J., Wetzel, S., Pekarek, S., & Banks, M. K. (1999). Extraction of petroleum hydrocarbons from soil by mechanical shaking. Environmental Science & Technology, 33, 1940–1945.10.1021/es9809758
- Valderrama, C., Alessandri, R., Aunola, T., Cortina, J. L., Gamisans, X., & Tuhkanen, T. (2009). Oxidation by Fenton’s reagent combined with biological treatment applied to a creosote-comtaminated soil. Journal of Hazardous Materials, 166, 594–602.10.1016/j.jhazmat.2008.11.108
- Venny, Gan, S., Ng, H. K. (2012). Current status and prospects of Fenton oxidation for the decontamination of persistent organic pollutants (POPs) in soils. Chemical Engineering Journal, 213: 295–317.10.1016/j.cej.2012.10.005
- Wang, W. H., Hoag, G. E., Collins, J. B., & Naidu, R. (2013). Evaluation of surfactant-enhanced in situ chemical oxidation (S-ISCO) in contaminated soil. Water, Air, & Soil Pollution, 224, 1713–1721.10.1007/s11270-013-1713-z
- Yap, C. L., Gan, S., & Ng, H. K. (2011). Fenton based remediation of polycyclic aromatic hydrocarbons-contaminated soils. Chemosphere, 83, 1414–1430.10.1016/j.chemosphere.2011.01.026
- Zhou, W. J., & Zhu, L. Z. (2008). Enhanced soil flushing of phenanthrene by anionic-nonionic mixed surfactant. Water Research, 42, 101–108.10.1016/j.watres.2007.07.021