ABSTRACT
The main aim of the study was to understand the present status of cobalt in different agro-climatic zones. The soil samples were collected from different locations and topo-sequences of three agro-climatic zones of Jharkhand, India, viz. zone-IV (Baliapur, Jharia, and Dhanbad), zone-V (Bagru, Pakharpat, Kisko, and Lohardaga), and zone-VI (Moshabani, Jadugonda, and Chandil). The soil samples were analyzed in a laboratory to estimate the total cobalt and Diethylene-triamine pentaacetic acid (DTPA) extractable cobalt. Results show mean concentration of DTPA extractable cobalt in zones-IV, V, and VI have been determined as 0.65, 0.5, and 1.03 mg kg−1, whereas the mean total cobalt content in different agro-climatic zones was 109.17, 107.58, and 102.58 mg kg−1, respectively. The work highlights the higher amount of DTPA-extractable and the total content of cobalt was observed in lowland against the different topo-sequences. Further, the results of multiple regression equations have revealed that the distribution of extractable cobalt is primarily controlled by pH, clay, and organic carbon. Whereas, organic carbon controls the distribution of total cobalt content hence, the organic carbon plays a critical role in the distribution of cobalt in the soil.
Introduction
The Jharkhand state of India ranks as 3rd in terms of mineral production in the country and holds 40% of India’s mineral wealth. It accounts for 27% coal, 26% iron ore (haematite), 27% apatite rock phosphate, 20% cobalt, and 18% copper ore as a resource of the country. Soil is a natural resource (Paudel, Thakur, Singh, & Srivastava, Citation2015). Cobalt is a widely distributed element and is essential for animals and plants (Kabata-Pendias, Citation2011). Literature indicates many researchers around the globe have assessed both for diethylene-triamine pentaacetic acid (DTPA) extractable cobalt and total cobalt in soil (Davies & White, Citation1981; Chen, Ma, & Harris, Citation1999; Collins & Kinsela, Citation2010; Ewetola, Oyediran, Owoade, & Ojo, Citation2010; Huwait, Kumosani, Moselhy, Mosaoa, & Yaghmoor, Citation2015; Kayika, Siachoono, Kalinda, & Kwenye, Citation2017). The DTPA extractable cobalt is available to plants, whereas total cobalt is exchangeable and fixed fraction, with time the fixed portion is available to plants. The parent rocks as a lithogenic control (higher correlation with soil properties), chemical industries, mineral fertilizers, untreated industrial effluents, sewage, and mine wastewater are the major sources of cobalt and other minerals in soil and water (Gautam et al., Citation2015). In addition, to heavy metals that come from anthropogenic sources are more mobile than lithogenic metals (Burt, Wilson, Mays, & Lee, Citation2003).
In the natural environment, the distribution of cobalt has many adverse effects on plants and human. However, due to pedogenic and biogeochemical process and anthropogenic inputs, the concentration of heavy metals continuously rises to such an elevated level that it becomes phytotoxic (Kirkham, Citation1983). Surface soils with higher cobalt content are found in arid and semi-arid regions. The previous works show that the Egyptian soils have cobalt content as 16.5–26.8 mg/kg (Nasseem & Abdalla, Citation2003), Australian ferralsols (122 mg/kg) and Japanese soils (116 mg/kg). It may be attributed to either pollution or specific enrichment process (Kabata-Pendias, Citation2011). Mico´, Recatala´, Peris, and Sa´nchez (Citation2006) have assessed heavy metals sources in agricultural soils of a European Mediterranean area, and they found that the cobalt content is associated with the parent rocks. Levels of cobalt in soils were found to be as high as 12,700 mg kg−1 in the Vicinity of a Cemented Tungsten Carbide Tool Grinding Plant in the United States (Abraham & Hunt, Citation1995). Researchers have also investigated the environmental effects of mining and related industries of Jharkhand on soil, sediment, and water (Singh, Maiti, & Ghosh, Citation2009; Singh et al., Citation2012; Tiwari, Singh, Singh, & Marina, Citation2016). A systematic survey for delineation of cobalt from soil was initiated by a few workers in India to understand the sources, levels, and enrichment processes (Kumar & Kumar, Citation2010b; Kumar, Kumar, Karmakar, & Agarwal, Citation2010a; Kumar, Kumar, Rawat, & Yadav, Citation2012a).
Cobalt toxicity is affected by the physicochemical properties of the soil environment such as structure, organic matter, pH, and complex compounds (Luo, Zheng, Chen, Wang, & Fenghua, Citation2010). Soil quality criteria must consider the bioavailability of metals (Peijnenburg, Posthuma, Eijsackers, & Allen, Citation1997); these criteria can be used to establish maximum tolerable levels of metals that can be accommodated in soils. Many divalent metal cations (e.g., Mn2+, Fe2+, Co2+, Ni2+, Cu2+ and Zn2+) are structurally very similar and substitute for each other and create disorder in soil (Bruins, Kapil, & Oehme, Citation2000; Kumar, Kumar, Rawat, & Yadav, Citation2012b; Nies, Citation1999). The elevated concentration of heavy metals in soil could constitute a threat to human health and wildlife through the food web and should be investigated in the agricultural soils (Kayika et al., Citation2017; Krishna & Govil, Citation2007; Reimann, Demetriades, Eggen, & Filzmoser, Citation2009, Citation2011). This would require analysis of the extractable cobalt contents in soils or heavy metal contents in vegetables and crops, especially where high levels were found in soils and in urban areas the vegetables are irrigated by untreated sewage wastewater (Bharose, Singh, & Srivastava, Citation2013; Gautam, Sharma, Tripathi, Ahirwar, & Singh, Citation2013). Richardson, George, Hens, and Simpson (Citation2005) observed that the root elongation of plants was reduced by 30% when they were exposed for 3 days to 10 ppm cobalt concentration. Further, they found that the local environment contamination with cobalt as a result of re-suspended dust resulting from an uncontrolled mound of debris or emitted from the factory vents was considerable.
The cobalt distribution in soil depends on geogenic, anthropogenic, and climate. The different agro-climatic zones refer land unit in terms of its major climate and growing period which is climatically suitable for a certain range of crops and cultivars. The highlands are generally devoid of dense vegetation and have less infiltration, hence the soil of these areas have less concentration of cobalt in soil compared to lowlands. Upland soils had less total cobalt in upper horizons than in lower horizons (Mcintosh, Sherrell, & Prema, Citation1986). The upland soils are known to be more leached than the lowland soils (Mcintosh et al., Citation1986), due to higher rainfall and probably overall moisture conditions. The effect of leaching is most obvious in the case of upland soils with an iron pan. In these soils, cobalt has accumulated in the zone of maximum iron accumulation. Because the altitude effect is probably largely a leaching effect, and aspect is probably another topographic factor affecting Co extractability (Mcintosh et al., Citation1986). Altitude has had a major effect on the overall pattern of Co and the distribution of these elements down the profile; parent material and drainage have clearly had an influence (Lago-Vila, Arenas-Lago, Rodríguez-Seijo, Andrade Couce, & Vega, Citation2015).
Soil management problems in India and around the world are a thrust area of research in the field of soil resource management. The major soil degradation problems in India are: soil erosion, salinization, alkalinization and waterlogging, land degradation, loss of nutrients, soil moisture deficits, soil fertility depletion, and soil compaction. These problems are stratified according to slope, agro-climatic zones, soil types, and land use. The Planning Commission of India has delineated 15 agro-climatic regions to form the basis for agricultural planning in the Eighth Plan (Bhattacharyya et al., Citation2015). The review of Bhattacharyya et al. (Citation2015) discussed the salient mitigation techniques for reversing land degradation in India and their applicability in major agro-climates such as soil erosion control, water harvesting, terracing and other engineering structures, bank erosion control, intercropping and, contour farming, subsoiling, watershed approach, participatory resource, conservation and management, integrated nutrient management and organic manuring, reclamation of salt affected soils, water management and pollution control, irrigation management for improving input use efficiency, and intensive cropping and integrated farming systems.
The main aim of the present study was to assess the topographical distribution of cobalt in the soil of different agro-climatic zones of Jharkhand. The specific objectives of the work were as follows: (i) to understand the distribution and extent of cobalt in different agro-climatic zones, (ii) to find out factors responsible for the large-scale variability in cobalt distribution, and (iii) to develop regression model and finally prepare cobalt distribution maps.
Material and methods
Study area
The Jharkhand state of India has been divided into three agro-climatic zones or regions viz. Central north-eastern plateau (Region-I), Western plateau (Region-II), and South-eastern plateau (Region-III) (). Dhanbad, Jharia, and Baliapur (zone-IV) areas are geologically comprised of Archean granites and gneisses. Soil orders namely Entisols, Inceptisols, and Alfisols are present in these areas. The temperature shows variations as winter temperature (8.4–34°C) and summer (13.3–45.5°C), respectively. The annual average rainfall is 1270 mm.
Bagru, Kisko, Pakharpat, and Lohardaga (zone-V) areas are geologically comprised of Archean granites and gneisses. In the uplands, considerable thickness of laterite of Pleistocene age is found in the granite and gneisses tracts. Alluvium of the recent to sub-recent age is reported in the river valley. The mineral bauxite, feldspar, fire clay and china clay are present in the region. The annual average temperature is 23°C; the temperature goes to 36°C in summer and 10°C in winter seasons, respectively. The district receives annual average rainfall as 1000–1600 mm and it increases from west to east, and majority of rainfall during the southwest monsoon period.
Mosabani, Jandugonda, and Chandil (zone-VI) areas are geologically comprised of granites gneiss and schist. Formations of igneous, sedimentary, and metamorphic rocks of the Dharwarian period are reported in these areas. Three soil orders namely Entisols, Inceptisols, and Alfisols are present in these areas. The Jamshedpur receives an annual rainfall of 1500 mm, and maximum in the four months (June–September). The temperature ranges from 16°C (in winter) to 44°C (in summer months).
Sampling and samples analysis
From each zone, 75 soil samples were collected (0–20 cm depth) from topo-sequence namely Upland, Midland, and Lowland from three agro-climatic zones namely (i) Central and north-eastern plateau i.e. zone-IV (Baliapur), (ii) Western plateau i.e. zone-V (Bagru) and (iii) South-eastern plateau i.e. zone-VI (Moshabani). The soil samples were analyzed for various physico-chemical properties, viz. organic carbon, pH, electrical conductivity (EC) (1:2.5: soil: water), cation-exchange capacity (CEC), CaCO3, clay, and silt content, by using standard laboratory procedures. The available cobalt in soil samples, was extracted with a solution of 0.005 M DTPA-0.01 M CaCl2 − 0.1 M tri-ethanolamine (adjusted to pH 7.3) as outlined by Lindsay and Norvell (Citation1978). Total cobalt content was determined after digestion of soil with perchloric-hydrofluoric acid (Hesse, Citation1994). The concentration of metal was measured through atomic absorption spectrophotometer (AAS) (Analyst-2000, Perkin Elmer).
Regression analysis
Multiple regression analysis was performed through stepwise method to analyze the relative impacts of different factors that control the distribution of cobalt in soils of different zones. In the process of regression analysis, the dependent variables were categorized as available cobalt, and total cobalt whereas the independent variables or the explanatory variables as X1 (pH), X2 (EC), X3 (Organic carbon), X4 (CaCO3), X5 (Silt), X6 (Clay), and X7 (CEC) (Panse & Sukhatme, Citation1961).
Results and discussions
DTPA-extractable cobalt in upland soils has very low mean value in all studied zones with low standard deviation. The upland of the different agro-climatic zone has the mean value of cobalt as 0.52, 0.27, and 0.77 mg kg−1, respectively ( and A and B). Midland soils of areas of zone IV, V, and VI, ranges from 0.32 to 1.94 mg kg−1, 0.12 to 1.08 mg kg−1 and below detection limit (bdl) to 3.72 mg kg−1 with a mean value of 0.84, 0.37, and 0.98 mg kg−1, respectively. Whereas in lowland soils ranged from 0.3 to 0.9 mg kg−1, 0.28 to1.3 mg kg−1 and 0.32 to 3.04 mg kg−1 with a mean value of 0.59, 0.87, and 1.35 mg kg−1, respectively. The content and distribution of cobalt in soil profiles are dependent on soil-forming processes and therefore differ for soils of various climatic zones (Kabata-Pendias, Citation2011). Usually, higher cobalt contents of surface soils are observed for arid and semi-arid regions. Significant sources of cobalt pollution are related to nonferrous metal smelters, whereas coal and other fuel combustions are of considerably less importance.
Table 1. DTPA-extractable cobalt contents (mg kg−1) in soils of different topo-sequences (agro-climatic zones) of Jharkhand state, India.
Figure 2. A, B, C, D, E and F 3D representation of total and available cobalt content in agro-climatic zones IV, V and VI, respectively.
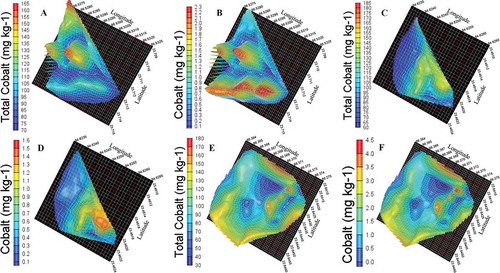
Huwait et al. (Citation2015) results indicated that the levels of Co in surface soil (0–15 cm) were higher than in sub-surface soil (>15-45 cm). The contribution of anthropogenic deposition of particles from urban sources (industrial emission, traffic, waste disposal; roadside soils and street dust) enriched heavy metals (cobalt and others) in soils (Chen et al., Citation1999; Collins & Kinsela, Citation2010; De Miguel, Llamas, Chacón, & Mazadiego, Citation1999; Krishna & Govil, Citation2007). There is a growing potential public health risk over the consumption of food crops growing on the mining waste disposal sites on the Copper belt in Zambia (Kayika et al., Citation2017). The cobalt concentration in mango fruit was less compared to soil it may be attributed to reduction in the translocation of the metal from the roots to the plant (Davies and White, Citation1981).
The upland soils hold the low concentration of cobalt and the main source is lithogenic because the upland area is less affected by the enrichment of cobalt and anthropogenic source. Lowland profiles show a very low range of variation of soil pH as compared to upland and midland whereas upland profiles show more variation of CaCO3 and less variation of electrical conductivity (Kabata-Pendias, Citation2011). Overall, DTPA-extractable cobalt under areas of zone IV, V, and VI ranged from bdl to 2.08 mg kg−1, bdl to 1.3 mg kg−1, and bdl to 3.72 mg kg−1, respectively. The decreasing order of cobalt was as follows zone VI (Lowland)>zone V (Lowland)>zone IV (Midland). The higher amount of available cobalt attributed to soil reaction. A similar observation was also reported by Roy, Acharya, Roy, Lahiri, and Sen (Citation1988). The mean value of DTPA-extractable cobalt in Lowland was higher than Midland followed by Upland except zone IV soils due to drainage. In poorly drained soils, amount of DTPA-extractable cobalt was greater than adjoining midland and upland which were comparatively well drained (Adams & Honeysett, Citation1964; Berrow & Mitchell, Citation1980; Mitchell, Reith, & Johnston, Citation1957; Walsh, Vessey, & Layzell, Citation1987). Mitchell et al. (Citation1957) attributed these effects due to the difference in the type of clay mineral and organic complexes formed under the different drainage conditions. In zone IV (Lowland), soil contained the lower value of DTPA-extractable cobalt than zone V (Midland), probably it might be due to more increase of soil pH because cobalt adsorption increased as soil pH increased (Baddesha, Chhabra, & Ghuman, Citation1997). Mcintosh et al. (Citation1986) study suggested that the evidence for altitude-related factors being a major influence on EDTA-extractable Co.
Total cobalt content in upland soils of zone IV, V, and VI ranges from 72 to 113, 61 to 131, and 65 to 142 mg kg−1 with a mean value of 94.76, 103, and 93.8 mg kg−1, respectively (). Midland soils of areas under zone IV, V, and VI, total cobalt content ranged from 83 to 146, 71 to 132, and 66 to 152 mg kg−1 with a mean value of 110.04, 106.4, and 102.84 mg kg−1, respectively, whereas in lowland soils, ranges from 91 to 163, 80 to 141, and 68–155 mg kg−1 with a mean value of 122.72, 113.36, and 111.12 mg kg−1, respectively. Overall, total cobalt content in areas of zone IV, V, and VI range from 72 to 163, 61–141, and 65–155 mg kg−1, respectively. The upland soils exhibited higher bulk density, particle density and lower water holding capacity; midland and lowland soils were recorded higher infiltration rate and clay percentage (Khan & Kamalakar, Citation2012). In all soils, pH, OC, and CEC consistently increased with depth. A similar observation was reported by Khan and Kamalakar (Citation2012) during the soil analysis of newly established Agro-biodiversity Park of Acharya NG Ranga Agricultural University, Hyderabad, Andhra Pradesh. The organic carbon content and CEC were higher in lowland soils which might be due to the clay translocation and organic carbon content accumulation. In all the pedons, calcium was most dominant cation followed by magnesium, sodium, and potassium throughout the profile. The upland pedons has low exchangeable cations than lowland soils because of excessive drainage from uplands (Khan and Kamlakar Citation2012). Data showed that midland and lowland soils of zone IV have the higher amount of total cobalt content than zone V and VI while upland soils of zone V contained the maximum amount of total cobalt. With the perusal of data, it showed that mean value of total cobalt in lowland soils was higher than midland followed by upland which might be due to higher content of organic matter and clay content (Aubert and Pinta Citation1977). Organic matter and clay percentage were generally more in lowland in comparison to midland and upland (Khan & Kamalakar, Citation2012) due to gentle slope, high infiltration rate and low run-off and forests.
Table 2. Total cobalt contents (mg kg−1) in soils of different topo-sequences (agro-climatic zones) of Jharkhand state, India.
In upland soils, according to Ewetola et al. (Citation2010), a relationship between slope position and soil properties where middle slope showed the highest clay content and the major pedogenic processes influenced the relationship between slope position and soil properties were mineral weathering erosion and eluvation-illuvation processes.
The 3D representation and contour plots of available cobalt and total cobalt in zone IV has been evidently identified, categorize, and quantify the specific areas where the enrichment of cobalt is higher. The available cobalt was observed low in the center as 30 mg kg−1 ( C and D). The total cobalt concentration was observed very low in those regions. In the zone V, it was observed that in the majority of areas, soils have low available cobalt whereas the total cobalt has slightly fewer values as compared to zone IV. In the agro-climatic zones, VI the total and available cobalt content is illustrated in (E and F) which shows similar patterns as zone V.
has the result of stepwise multiple regression and enabled us to choose the statistically most relevant equation stating the relationship between cobalt and the factors determining them. Coefficient of determination (R2) suggest that DTPA extractable cobalt (available cobalt) has 0.42; it is relatively determined by pH and clay. In case of total cobalt content, it was determined by organic carbon up to 0.38. The pH and clay are the dominant factors in controlling the distribution of cobalt in the soil. Soil texture and soil organic matter play an important role in the cobalt behavior in soils. The impact of soil organic matter is variable and depends on the kind of organic matter and pH. Although, soils rich in organic matter usually have low cobalt contents (Kabata-Pendias, Citation2011). The mobility of cobalt is strongly related to kind of soil organic matter. A significant correlation was found between lithogenic metals and some soil properties such as soil organic matter, clay content, and carbonates, indicating an important interaction among them (Mico´ et al., Citation2006; Naidu et al., Citation2008).
Table 3. Predictability of available and total content of cobalt with relation to soil characteristics figure in parenthesis indicates standard error of coefficient.
The prediction equation for total cobalt content has revealed that it can be determined by organic carbon which has the positive impact on the total cobalt content. In the lowland presence of a large amount of organic carbon controls the distribution total cobalt content.
The metal concentration and soil properties are known to influence metal bioavailability (pH, organic carbon, clay content, and effective CEC) in agricultural and grazing land soil in Europe (Reimann et al., Citation2009, Citation2011).
Conclusion
In conclusion, the cobalt distribution largely depends on the topography and agro-climatic zones. Outline of work suggested that DTPA-extractable cobalt was traced to 2.08 mg kg−1, in zone IV; trace to 1.3 mg kg−1 in zone V, whereas trace to 3.72 mg kg−1 in zone VI, respectively. Regardless lowland has the higher amount of DTPA-extractable cobalt; compared to highland with a mean value of 0.59 in zone IV; 0.87 in zone V, and 1.35 in zone VI, respectively. Due to steep slope at highlands, the cobalt is washed away during rainfall. Hence, the grass of highlands may be deficient of cobalt and ultimately affects the livestock. The total content of cobalt was 72–163 mg kg−1 in zone IV; 61–141 mg kg−1, in zone V whereas 65–155 mg kg−1 in zone VI, respectively. However, the higher amount of total content of cobalt was noted in lowland topo-sequence with a mean value of 122.72 mg kg−1 in zone IV; 113.36 mg kg−1 in zone V, and 111.12 mg kg−1 in zone VI, respectively. The study of stepwise multiple regression equations showed more impact of soil pH, clay, and organic carbon on the availability of cobalt than other soil parameters, whereas, in case of total content, organic carbon was the important determining factor. The areas where the soils have low amount of the cobalt needs cobalt salts, e.g. cobalt sulfate, as a feed additive to keep cattle and crops healthy in areas where there is insufficient natural bio-available cobalt. The metal concentrations and soil properties are known to influence metal bioavailability (pH, organic carbon, clay content, and effective CEC) in agricultural and grazing land soil.
Acknowledgments
Authors are grateful to the authorities of their respective organization for allowing them to undertake parts of the whole analysis/ground truthing/collection of secondary information, etc. All help and information received from known and unknown sources is also duly acknowledged.
Disclosure statement
No potential conflict of interest was reported by the authors.
References
- Abraham, J. L., & Hunt, A. (1995). Environmental contamination by cobalt in the vicinity of a cemented tungsten carbide tool grinding plant. Environmental Research, 69(1), 67–74.
- Adams, S. N., & Honeysett, J. L. (1964). Some effects of soil water logging on Co and Cu status of pasture plants grown in pots. Australian Journal of Agricultural Research, 15, 357–361.
- Aubert, H., & Pinta, M. (1977). Trace elements in soils (Vol. 395, pp. 19–25). Amsterdam: Elsevier.
- Baddesha, H. S., Chhabra, R., & Ghuman, B. S. (1997). Changes in soil chemical properties and plant nutrient content under eucalyptus irrigated with sewage water. Journal Industrial Social Soil Sciences, 45, 358–362.
- Berrow, M. L., & Mitchell, R. L. (1980). Location of trace elements in soil profiles: Total and extractable contents of individual horizons. Transactions of the Royal Society of Edinburgh: Earth Sciences, 71(2), 103–121.
- Bharose, R., Singh, S. K., & Srivastava, P. K. (2013). Heavy metals pollution in soil-water-vegetation continuum irrigated with ground water and untreated sewage. Bulletin Environment Sciences Researcher, 2, 1–8.
- Bhattacharyya, R., Ghosh, B. N., Mishra, P. K., Mandal, B., Rao, C. S., Sarkar, D., … Franzluebbers, A. J. (2015). Soil degradation in India: Challenges and potential solutions. Sustainability, 7, 3528–3570.
- Bruins, M. R., Kapil, S., & Oehme, F. W. (2000). Microbial resistance to metals in the environment. Ecotoxicology and Environmental Safety, 45, 198–207.
- Burt, R., Wilson, M. A., Mays, M. D., & Lee, C. W. (2003). Major and trace elements of selected pedons in the USA. Journal of Environment Quality, 32, 2109–2121.
- Chen, M., Ma, L. Q., & Harris, W. G. (1999). A saline concentration of fifteen trace elements in Florida surface soils. Journal of Environment Quality, 28, 1173–1181.
- Collins, R. N., & Kinsela, A. S. (2010). The aqueous phase speciation and chemistry of cobalt in terrestrial environments. Chemosphere, 79(8), 763–771.
- Davies, B. E., & White, H. M. (1981). Trace elements in vegetables grown on soils contaminated by base metal mining. Journal of Plant Nutrition, 3, 387–396.
- De Miguel, E., Llamas, J. F., Chacón, E., & Mazadiego, L. F. (1999). Sources and pathways of trace elements in urban environments: A multi-elemental qualitative approach. The Science of the Total Environment, 235, 355–357.
- Ewetola, E. A., Oyediran, G. O., Owoade, F. M., & Ojo, O. I. (2010). Variations in soil physical properties along toposequence of an alfisol in Southern Guinea Savanna of Nigeria. International Journal of Agriculture, Environment and Biotechnology, 3(3), 303–305.
- Gautam, S. K., Maharana, C., Sharma, D., Singh, A. K., Tripathi, J. K., & Singh, S. K. (2015). Evaluation of groundwater quality in the Chotanagpur plateau region of the Subarnarekha river basin, Jharkhand state, India. Sustainability of Water Quality and Ecology, 6, 57–74.
- Gautam, S. K., Sharma, D., Tripathi, J. K., Ahirwar, S. K., & Singh, S. (2013). A study of the effectiveness of sewage treatment plants in Delhi region. Applications Water Sciences, 3, 57–65.
- Hesse, P. R. (1994). A text book of soil chemical analysis.CBS. New Delhi, India: publishers and distributors.
- Huwait, E. A., Kumosani, T. A., Moselhy, S. S., Mosaoa, R. M., & Yaghmoor, S. (2015). Relationship between soil cobalt and vitamin B12 levels in the liver of livestock in Saudi Arabia: Role of competing elements in soils. African Health Sciences, 15(3), 993–998.
- Kabata-Pendias, A. (2011). Trace elements in soils and plants (4th ed.). Boca Raton, Florida: CRC Press.
- Kayika, P., Siachoono, S. M., Kalinda, C., & Kwenye, J. M. (2017). An investigation of concentrations of copper, cobalt and cadmium minerals in soils and mango fruits growing on Konkola copper mine tailings dam in Chingola, Zambia. Archives Des Sciences / Editees Par La Societe De Physique Et D’histoire Naturelle De Geneve, 1(1), 1–4.
- Khan, M. A. A., & Kamalakar, J. (2012). Physical, physico-chemical and chemical properties of soils of newly established Agro-biodiversity Park of Acharya NG Ranga Agricultural University, Hyderabad, Andhra Pradesh. International Journal Far Sciences, 2(2), 102–116.
- Kirkham, M. B. (1983). Problems using wastewater on vegetable crops. Horti Sciences, 21, 24–27.
- Krishna, A. K., & Govil, P. K. (2007). Soil contamination due to heavy metals from an industrial area of Surat, Gujarat, Western India. Environmental Monitoring and Assessment, 124, 263–275.
- Kumar, R., & Kumar, R. (2010b). Status of heavy metals (Co, Ni & Pb) in different agro-climatic zones of Jharkhand. Indian Journal of Agricultural Chemistry, 43, 183–190.
- Kumar, R., Kumar, R., Karmakar, S., & Agarwal, B. K. (2010a). Vertical and topographical distribution of Co, Ni & Pb in relation to soil characteristics in different agro-climatic zones of Jharkhand. Journal Industrial Social Soil Sciences, 58, 293–298.
- Kumar, R., Kumar, R., Rawat, K. S., & Yadav, B. (2012a). Vertical distribution of physico-chemical properties under different topo-sequence in soils of Jharkhand. Journal of Agricultural Physics, 12, 63–69.
- Kumar, R., Kumar, R., Rawat, K. S., & Yadav, B. (2012b). Effect of organic matter and lime in remediation of heavy metals (Co, Ni & Pb) in Alfisols. Annals Agricultural Researcher New Series, 33(4), 261–265.
- Lago-Vila, M., Arenas-Lago, D., Rodríguez-Seijo, A., Andrade Couce, M. L., & Vega, F. A. (2015). Cobalt, chromium and nickel contents in soils and plants from a serpentinite quarry. Solid Earth, 6, 323–335.
- Lindsay, W. L., & Norvell, W. A. (1978). Development of a DTPA soil test for zinc, iron, manganese, and copper1. Soil Science Society of America Journal, 42, 421–428.
- Luo, D., Zheng, H., Chen, Y., Wang, G., & Fenghua, D. (2010). Transfer characteristics of cobalt from soil to crops in the suburban areas of Fujian province, southeast China. Journal of Environmental Management, 91, 2248–2253.
- Mcintosh, P. D., Sherrell, C. G., & Prema, P. G. (1986). Distribution of cobalt, copper and zinc in upland and lowland soils of the Kaiwera district, eastern Southland, New Zealand (NZ Soil Bureau Scientific Report 78). 19. New Zealand: New Zealand Soil Bureau.
- Mico´, C., Recatala´, L., Peris, M., & Sa´nchez, J. (2006). Assessing heavy metal sources in agricultural soils of an European Mediterranean area by multivariate analysis. Chemosphere, 65, 863–872.
- Mitchell, R. L., Reith, J. W. S., & Johnston, I. M. (1957). Trace element uptake in relation to soil content. Journal of Science of Food and Agriculture, 8, 51–59.
- Naidu, R., Bolan, N. S., Megharaj, M., Juhasz, A. L., Gupta, S. K., Clothier, B. E., & Schulin, R. (2008). Chemical bioavailability in terrestrial environments. In A. E. Hartemink, A. B. McBratney, & R. Naidu (edited by), Developments in soil science (Vol. 32, pp. 1–6). Oxford, UK: Elsevier.
- Nasseem, M. G., & Abdalla, Y. H. (2003). Cobalt status in the north western coast soils of Egypt in relation to cobalt content of barley for ruminants. 16 international symposium on environmental biogeochemistry, Edinburgh, Scotland.
- Nies, D. H. (1999). Microbial heavy-metal resistance. Applied Microbiology and Biotechnology, 51, 730–750.
- Panse, V. G., & Sukhatme, P. V. (1961). Statistical methods for agricultural workers. New Delhi, India: ICAR.
- Paudel, D., Thakur, J. K., Singh, S. K., & Srivastava, P. K. (2015). Soil characterization based on land cover heterogeneity over a tropical landscape: An integrated approach using earth observation data-sets. Geocarto International, 30(2), 218–241.
- Peijnenburg, W. J. G. M., Posthuma, L., Eijsackers, H. J. P., & Allen, H. E. (1997). A conceptual framework for implementation of bioavailability of metals for environmental management purposes. Ecotoxicology and Environmental Safety, 37, 163–172.
- Reimann, C., Demetriades, A., Eggen, O. A., & Filzmoser, P. and The EurogGeoSurveys Geochemistry expert group (2009). The EuroGeoSurveys geochemical mapping of agricultural and grazing land soils project (GEMAS)–evaluation of quality control results of aqua regia extraction analysis (NGU Report 2009.49). 94. Trondheim, Norway: Geological Survey of Norway.
- Reimann, C., Demetriades, A., Eggen, O. A., & Filzmoser, P. and The EurogGeoSurveys Geochemistry expert group (2011). The EuroGeoSurveys geochemical mapping of agricultural and grazing land soils project (GEMAS)–evaluation of quality control results of total C and S, total organic carbon (TOC), cation exchange capacity (CEC), XRF, pH, and particle size distribution (PSD) analysis (NGU Report 11.043). 90. Trondheim, Norway: Geological Survey of Norway.
- Richardson, A. E., George, T. S., Hens, M., & Simpson, R. J. (2005). Utilization of soil organic phosphorus by higher plants. In B. L. Turner, E. Frossard, & D. S. Baldwin (eds), Organic phosphorus in the environment (pp. 165–184). Wallingford, UK: CABI.
- Roy, D. K., Acharya, S. K., Roy, K. K., Lahiri, T. C., & Sen, M. K. (1988). Nature of occurrence age and depositional environment of the oceanic pelagic sediments associated with the ophiolite assemblege, south Andaman Islands. Indian Minerals, 42, 31–56.
- Singh, A. K., Mondal, G. C., Singh, T. B., Singh, S., Tewary, B. K., & Sinha, A. (2012). Hydrogeochemical processes and quality assessment of groundwater in Dumka and Jamtara districts, Jharkhand, India. Environment Earth Sciences, 67, 2175–2191.
- Singh, G., Maiti, S. K., & Ghosh, S. P. (2009). Heavy metal contamination in roadside soil and vegetation: A review. Industrial Journal Environment Protection, 29(4), 334–341.
- Tiwari, A. K., Singh, P. K., Singh, A. K., & Marina, D. M. (2016). Estimation of heavy metal contamination in groundwater and development of a heavy metal pollution index by using GIS technique. Bulletin of Environmental Contamination and Toxicology, 96, 508–515.
- Walsh, K. B., Vessey, J. K., & Layzell, D. B. (1987). Carbohydrate supply and N2 fixation in soybean: The effect of varied day length and stem girdling. Plant Physiology, 85, 137–144.