ABSTRACT
Glaciated sediments are a significant source to know the glacier environment processes. Glacial meltwater streams draining from the Himalayan glaciers carry with a different type of sediment sizes, because of the supraglacial, englacial, and subglacial debris, as well as formation of sediments from erosion by the movement of the ice. This study examines mineralogical and textural characteristics of sediments transported in the selected Indian Himalayan glacier meltwater streams. The results indicate that various sedimentological diversity occurring within proximity of active glaciations due to glacio-hydrological processes. Sedimentological and mineralogical characteristics suggest that sediments of the proglacial area are polygenic in nature. A comparative change in feldspar/quartz among bedrock, sand, and clay suggested that the active geochemical weathering regime at a low-temperature environment maturing the sand composition. The less clay fraction in glacier sediment indicates limited generation of clay minerals in the glacier environment. Change in clay composition from supraglacial moraine to proglacial environment indicated a chemical alteration in glaciated valley assisted by low slope glacio-fluvial environment.
Introduction
Sediments derived from various Himalayan uplands, which form the biggest Indus-Ganga-Brahmaputra floodplains in the world. The sources of this sediment come through different geo-morphological processes in the upland area which are covered with snow and ice. Glaciological processes are a significant source of chemically immature sediments that contribute to the fertility of the soil in floodplain areas (Ahmad & Hasnain, Citation2007). The supraglacial sediments lay over the glacier play a major role in the melting of snow and ice below the glacier. Debris cover protects the glacier melting from thickly covered sediments and enhances the ice melting due to thinly covered sediments. It has long been recognized that physical weathering act as essential processes in producing vast volumes of sediments because glaciers are very active agents of weathering and erosion. Plucking, quarrying, crushing, shearing, and abrasion are the physical weathering processes in alpine basins.
Along with active glaciological processes high seismicity, steep valleys with frequent avalanching, intense monsoonal rainfall, and glacial activities support high erosion rates in the Himalayan catchments (Pandey et al., Citation1999; Hasnain & Thayyen, Citation1999; Singh & Hasnain, Citation1998). Himalayan glaciers also produced a large amount of rock debris consist of gravel, sand, silt/clay, etc., stored on supra-glacier and paleo moraines compared to other glacier regions. Such glaciers are thought to be the main active agents of erosion and transporting sediment in regional denudation systems (Gardner, Citation1986). Numbers of studies have demonstrated that specific yield may increase downstream due to the remobilization of sediments pushed by the glaciers (Ferguson, Citation1984; Hasnain & Chauhan, Citation1993; Warburton, Citation1990). Although plenty of literature and data are available on sediment and soil attributes in the middle and lower catchments of the Himalayan rivers (Pandey et al., Citation1999). The sediment characteristics in river water at upland catchments are extremely sparse. For mountain studies, textural, mineralogical, and geochemical characteristics of the sediments at glacier surface are extremely important for revealing the tectonic process and also dealing with the problems of soil erosion, flash flooding, quality of water supply in the downstream regions, transport of chemical pollutants and degrading of natural habitats. To recognize the relationship between glacier-hydrological-sedimentological interactions processes a detailed analysis has studied. The sedimentological characteristics and mineralogical composition of various types of sediments were chosen to reveal these interactions processes. The supra-glacier, old recessional moraine of the pro-glacial stream bed, and meltwater suspended sediments were collected in glacier environment at some selected locations in the Indian Himalaya. The present studies can also be used to interpret ancient landforms and sediments for paleo-environmental reconstructions, and aid in understanding different processes which are operating on glacier sediments.
Study area
The study area comprises of five Himalayan glaciers which are basically Parkhachik and Drang-Drung glacier in western Himalaya, Pin-Parbati and Gangotri glacier in middle Himalaya, and Zero point glacier in eastern Sikkim Himalaya. All these Himalayan glaciers bounded by latitudes (26°34ʹ41”–34°51ʹ23”) and longitudes (75°59ʹ12”–89°34ʹ45”) in the northern peninsular region of India ().
Figure 1. Location map of the sediments samples site in the active glacial region of Indian Himalaya (After Carosi et al. 2018).
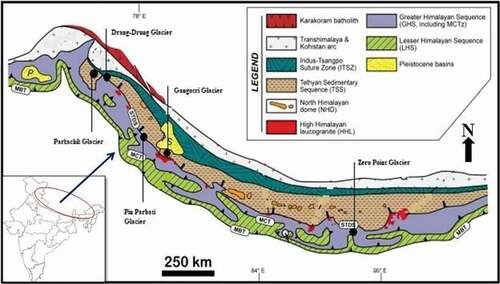
For collecting glacier surface samples, numerous field campaigns were conducted in these selected Himalayan glaciers. The samples were collected from Gangotri glacier basin (30.926074°, 79.080370°), Bhagirathi valley, Garhwal Himalaya, Pin-Parbati glacier (31.799362°, 77.374058°), Parbati valley, Himachal Pradesh in central Himalaya. In western Himalaya, the samples were collected from Parkhachik glacier (34.050942°, 76.024245°), Drang-Drung glacier (33.861900°, 76.362755°), Indus valley, Kashmir Himalaya, and Zero point glacier in eastern, Sikkim Himalaya.
Geologically, the basement rocks of all these glaciers are leucogranite and gneisses of the central crystalline zone. These extensive glaciers were characterized by variable size typically ranging from 30-km-long Gangotri glacier, 9-km Pin-Parbati glacier, 14 km Parkhachik glacier, 22 km Drang-Drung glacier, and 5-km-long Zero point glacier. All these glaciers were densely covered with debris except Drang-Drung glacier. The frontal portion of this glacier was also covered with debris along with a small size lake at glacier front.
Methods
To know the textural and mineralogical characteristics of glacier sediments we have collected a different fraction of sediment samples from glaciated moraines and stream bed sediments during the post-monsoon period. Samples were assembled in zip-lock polythene bags using a plastic scoop to avoid any types of contamination. The grain size distributions were determined by sieve shaker (Fritsch Analysette 03.502) and textural analysis was carried out using Folk and Ward (Citation1957).
The medium grains sand fraction of sediments samples were analyzed to determine the major mineral composition, and Trinocular Stereo zoom microscope (Nikon-SMZ1500) were used for this purpose. In each sample, minerals were identified and performed mineral counting analysis based on a physical method for the 200 particles in each sample (Ingersoll et al., Citation1984). The mineralogy of clay fraction of sediment samples was studied using an X-ray diffractogram instrument (Carrols, Citation1970; Gibbs, Citation1970). The samples were used as a powder and run diffractometer along with Ni-filter and Cu, Kα-radiation with the chart drive speed of 1 cm−1/min.
The pebbles size fraction of bed sediment samples were examined under petrography analysis under thin section studies. These thin sections were prepared by cutting a section of the rock sample followed by grinding and polishing before fixing on a petrography slide (Hamphries, Citation1992). The different gravel samples were viewed under thin sections and photographed under the microscope with a magnification factor of 20 µm and 50 µm.
Results and discussion
Sedimentological characteristics of the glaciated sediments
Basic statistical grain size parameters like graphics mean, standard deviation skewness, and kurtosis were calculated for different sediments fraction from Gangotri, Pin-Parbati, Parkhachik, Drang-Drung, and zero-point glaciers regions ().
Table 1. Sedimentological characteristic in the Himalayan glacier environment.
The mean grain size is an important parameter that defines the overall grain size characteristics. The mean values of sediments from supraglacial moraines, old recession moraines, snout, and suspended sediments are 0.71ɸ, 2.01ɸ, 2.12ɸ, and 4.95ɸ respectively (). The mean size of supraglacial moraine ranges from 0.38 to 1.24ɸ indicating a predominance of coarse sand which was larger than among all collected sediment samples in different glaciated regions.
The supraglacial moraines are characterized by loose rock debris on the surface of glacier and dust settling out from the atmosphere. Sorting of supraglacial moraines ranges from 0.99 to 1.9ɸ with STD (Standard Deviation) of 0.36ɸ showing poorly sorted. The average sorting value of suspended sediments is 1.5ɸ with STD of 0.11ɸ indicating very well-sorted sediments; it may be due to low energy conditions prevailing in the glacial environment during post-monsoon seasons (October to November). The other researchers have reported that the poor sorting in the glacial meltwater of suspended sediments due to the high flow conditions of glaciers meltwater (Pandey et al., Citation2002; V.B. Singh et al., Citation2014).
The skewness values of sediments indicate negatively skewed near snout area and old lateral moraine, which shows the admixture of relatively coarse to strongly coarse skewed sediments (). Supraglacial moraine sediments tend to be near symmetrical (>0.5) with a relatively small abundance of fine particles which indicates a large admixture of medium to fine sediments while suspended sediments are characterized by positive/fine skewed (0.26) which indicates a relatively high proportion of fine sediments.
The kurtosis expresses the peakedness of the grain-size distribution. If the central portion is better sorted than the tails, the frequency curve is said to be excessively peaked or leptokurtic. If the tails are better sorted, the curve is said to be flat peaked or platykurtic. The average kurtosis of supraglacial sediments is 0.71ɸ, followed by 1.3ɸ for side old moraine, 1.9ɸ near the snout, and 0.9ɸ for suspended sediments (). The individual location is showing local variation because suspended sediments fall in the mesokurtic category and sediments from the near snout area are very leptokurtic in character (>1.9).
Mineralogical characteristics of the glaciated sediments
Average sediments mineralogy of the gravel fraction from the Pin-Parbati, Drang-Drung, Parkhachik, Gangotri, and Zero point glaciers areas were subjected to the petrological microscopic studies. The minerals identified were quartz, plagioclase biotite, and muscovite. The volume percentages of identified minerals in the thin section are presented in (). Quartz is the most dominant mineral comprising 57% followed by biotite 18%, muscovite 8%, and plagioclase 13% ().
Table 2. Mineral composition % of gravel in the selected Himalayan glacier and pro-glacial environment.
Figure 5. Average mineral percentages showing of different sediment fraction in glaciated environment.
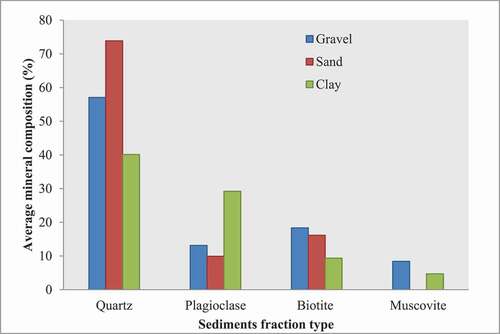
The average mineralogy of the sand fraction of the sediments from the Pin-Parbati, Drang-Drung, Parkhachik, Gangotri, and Zero point glaciers were subjected to the stereomicroscope studies in order to determine the bulk mineralogy. The volume percentage of identified minerals was presented in (). Quartz is the most dominant mineral, comprising 73%, followed by muscovite 16% and plagioclase 10%.
Table 3. Mineral composition % of sand in the selected Himalayan glacier and pro-glacial environment.
The average sediments mineralogy of silt/clay fraction of the Pin-Parvati, Parkhachik, and Drang-Drung glaciers meltwater were subject to the X-ray Diffraction studies in order to the bulk mineralogy determination. The volume percentage of identified minerals in clay fraction (< 63 µm) is given in (). It shows that quartz is the most dominant mineral, comprising 40%, followed by micas 9%, plagioclase 29%, and chlorite 5%.
Table 4. Mineral composition % of Clay/silt fraction in the selected Himalayan glacier and pro-glacial environment.
The volume percentages of identified minerals of suspended sediments in glacier streams are presented in (). Minerals percentages of suspended sediments were compiled from various studies (Ahmad, Citation1995, Citation2000; Chauhan, Citation1995; Pandey, Citation1999; Raju, Citation1993). Quartz is the most dominant mineral, comprising 30.3%, followed by mica 20%, plagioclase 31.2%, Sericite/illite 18.2%, and calcite/dolomite 5%. The comparative analysis of the mineral composition from different fractions of the sediment suggests that the bedrock and the fine fraction are near the same concerning mineral composition. However, a contrast was found for the composition of the sand fraction that was associated with a high percentage of quartz. The changes in the relative abundance of minerals in fine fractions have been used to reveal the earth surface processes (Haldar & Tišljar, Citation2014; Montgomery et al., Citation2000; Nicholas, Citation1997; Viers et al., Citation2007). A change in relative intensities of peaks of the minerals was recognized along with the new peak X-rays diffractograms. The X-rays diffractograms peak of sediments indicates that the bulk mineralogy may change significantly from supra-glacier sediments to pro-glacier bed sediments.
Table 5. Mineral Composition of suspended sediments draining from different glaciated regions.
Discussion
Numerous workers particularly in Western Himalaya, Nepal Himalaya, and the Khumbu Himalaya of Nepal have described the characteristic of supra-glacial debris on glaciers (Bishop et al., Citation1999, Citation1995; Kick, Citation1962; Owen, Citation1988; Scott, Citation1992) and the Khumbu Himalaya of Nepal (Inoue, Citation1977; Iwata et al., Citation2000; Moribayashi & Higuchi, Citation1977). Bishop et al. (Citation1995, Citation1999), and Shroder et al. (Citation2000) highlighted the importance of supra-glacial debris cover in the glacial system. Facies over the glaciers particularly the lateral and terminal sediment deposits were described by (Benn & Owen, Citation2002). Supraglacial processes are responsible for the sedimentological characteristics of glacier surface debris. Much of the supraglacial debris on Himalaya was derived by valley sides mainly lateral, medial, and old moraines, either falling directly on ablation zone or undergoing englacial transport. The steep slopes of lateral and median moraine continuously supply the sediment on the glacier surface. Further, this debris interacts with supra-glacial melting processes that redistribute the sediment and associated with numerous ponds, lakes, Moulin, crevasse, and related supraglacial channels and spread with meltwater. The finer sediments are removed by the continuous flow of supraglacial streams channels due to a steep gradient that promotes unstable situation for the accumulation of finer sediments on the glacier surface. The selective removal of the fine particle from debris results in coarsening of the supraglacial sediments (–d)).
Delivering of the meltwater through supra-glacier channels into Moulin add the fine sediments to fully developed a subglacial system in peak ablation system (Hasnain et al., Citation2001). The fine size sediments provided by supraglacial channels, the subglacial abrasion activity, unstable moraines, and alluvial cones at snout region, which reflected positively skewed and highly leptokurtic nature of the sediments. During the ablation season, huge quantities of sediment are released from the margin of debris-covered glacier deposits by a combination of mass movement and glacio-fluvial processes (Hewitt, Citation1967). Sometimes these sediments are under-covered by subglacial till which exposed by deglaciation and erosion processes. This subglacial till associated with fine silt and contribute into glacier meltwater stream and result in fine skewed glacier fluvial deposits in front of the glacier snout (). The glacio-fluvial environment of the Himalayan region is mostly surrounded by paleo lateral moraine. The silt and clay from lateral moraine also mobilized along with slope and contributed fine sediments in the proglacial environment.
Figure 7. (a) Supraglacial debris derived by valley side erosion and falling on ablation zone. (b) Lateral erosion contribute the sediments on glacier surface. (c) Medial moraine contribute the sediments near glacier surface. (d) Fine material removed by Moline and coarsening the sediments at the glacier surface.
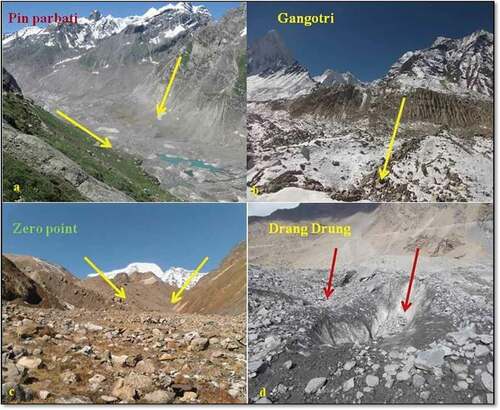
Sedimentological and mineralogical characteristics suggest that sediments of the proglacial area are polygenic in nature. The reworking of the old and new supraglacial sediments processes indicates mature sand composition. The location of the sand in Dickenson plot suggested, the stable shield area are the sources of the sediments, but the maturing of sand composition in glacier environment due to the recycling of available quaternary glacial sediments near glacier area (). The high percentage of quartz in sand fraction than gravel fraction suggests that the reworking of sand grade sediment in meltwater stream drain from glacier environments. The detritus mineralogy of the bed sediments of river Ganga in central Ganga basin also suggested that the detritus mineral mainly derived from recycled orogen provenance with granite-gneisses (S.K. Singh et al., Citation2008).
Figure 8. Subglacial till associated with fine silt and contribute into glacier meltwater stream showing in front of the Pin-Pārbati glacier snout.
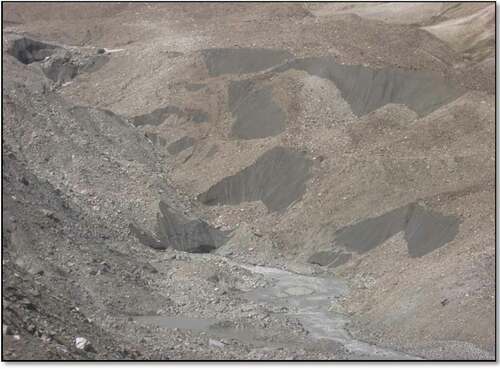
Geochemical weathering processes were assessed by using feldspar/quartz minerals ratios of different sediments fractions. The mean values were shown 0.34ɸ, 0.16 ɸ, and 0.8 ɸ for the bed rock, sand, and clay/silt fraction samples respectively. Loss of feldspar in sand fraction of sediment indicates a geochemical weathering of the active minerals in the glaciated environment. The high viscosity of meltwater at low temperature, high hardness, and less geochemical activity of quartz grain has limited production of fine particles of quartz. During these processes, it leads to higher feldspar/quartz ratio in clay/silt fraction at glacier region environment. Further, the high feldspar/quartz ratios of the suspended sediment in the glacial stream were observed that the selective removal of the feldspar, biotite, and muscovite and increase the fine particle in glacier meltwater. The less clay fraction in glacier sediment indicates limited generation of clay minerals in the glacier environment. The X-ray diffractograms showing a variation in mineralogy near the glacier snout and downstream regions ()). The result indicates that the decomposition of clay mineral in the proglacial stream caused by the sediment moisture and stable hydraulic condition in low slope area in most of the year. In low slope regions near the glaciated environment were shown that the strong leveling of the topography by the glacier action.
Conclusions
The present study provide a research platform to analysis the sediments characteristics of glacial meltwater streams and their origin. The major findings of this study summarized as follows:
Supraglacial-meltwater interaction processes are responsible for the sedimentological characteristics of glacier surface debris. The selective removal of a fine particle from debris results in coarsening of the supraglacial debris. These fine particle sediments further mixes with the weathered subglacial material, unstable moraines, and alluvial cones at snout region, which results in a positive skewed and highly leptokurtic nature of sediment in glacio-fluvial region.
The reworking of the old and new supraglacial sediments results in mature sand composition in glacier environment.
A comparative change in feldspar/quartz ratio for the bed rock, sand, and clay/silt fraction indicate the geochemical weathering and limitation of a generation of the clay-size particle in glacier environment.
Moisture content in glacial, fluvial sediments, and stable hydraulic condition along with low slope in the glaciated area provides an ideal place for the change in minerals composition in the proglacial stream.
Therefore, based on present investigations the current results suggest that, textural and mineralogical characteristics of Himalayan glaciers sediment provides detailed information on glacier dynamics, erosion rates, sediment transport, hydrological processes, etc. Therefore, future studies should follow homogenous time periods that are analogous to other studies during the ablation season over the Indian Himalayan glaciers.
Acknowledgments
The authors would like to express their thanks to Chairman, Department of Geology, Aligarh Muslim University for providing the laboratory and library facilities. The authors would like to appreciate the financial assistance provided by under DRS–II (SAP–I) program, UGC, Ministry of HRD, Govt. of India to conduct the present research work (F. No. 41-1041/2012(SR).
Disclosure statement
The authors declare that they have no conflict of interest.
Additional information
Funding
References
- Ahmad, S. (1995). Hydro-geochemical characteristics of meltwater draining from Bagni glacier, Dhauliganga basin, Garhwal Himalaya, Uttar Pradesh [M.Phill. Dissertation]. School of Environmental Sciences JNU New Delhi, 100.
- Ahmad, S. (2000). Ecohydrological Studies of Harsil sub-basin, Ganga headwater, Bhagirathi valley, Garhwal Himalaya, Uttar Pradesh, India [Ph.D. Thesis]. School of Environmental Sciences JNU New Delhi, 288.
- Ahmad, S., & Hasnain, S. I. (2007). Sediment transport in Alpine to sub-alpine stream and forest stream in Harsil sub-basin, Uttarkashi, Garhwal Himalayas, Uttar Pradesh. Journal of the Indian Association of Sedimentologists, 26(1–2), 81–89.
- Ahmad, S.(2000) Ecohydrological Studies Of Harsil Sub-Basin, Ganga Headwater, Bhagirathi Valley, Garhwal Himalaya, Uttar Pradesh, India. Phd thesis, School of Environmental Sciences, Jawaharlal Nehru University, New Delhi, (pp. 200).
- Benn, D. I., & Owen, L. A. (2002). Himalayan glacial sedimentary environments: A framework for reconstructing and dating the former extent of glaciers in high mountains. Quaternary International, 97–98, 3–25. https://doi.org/10.1016/S1040-6182(02)00048-4
- Bishop, M. P., Shroder, J. F., & Hickman, B. L. (1999). SPOT panchromatic imagery and neural networks for information extraction in a complex mountain environment. Geocarto International, 14(2), 19–28. https://doi.org/10.1080/10106049908542100
- Bishop, M. P., Shroder, J. F., & Ward, J. L. (1995). SPOT multispectral analysis for producing supra-glacial debris-load estimates for Batura glacier, Pakistan. Geocarto International, 10(4), 81–90. https://doi.org/10.1080/10106049509354515
- Carrols, D. (1970). Clay minerals - a guide to their X-ray identification. The Geological Society of America, 126, 48–65. https://doi.org/10.1130/SPE126-p1
- Chauhan D. S. (1993) Chemical characteristics of snow, ice and meltwater from Satopanth and Bhagirathi Kharak Glacier basin, Alaknanda Valley, India. Phd thesis, School of Environmental Sciences, Jawaharlal Nehru University, New Delhi, (pp. 96).
- Chauhan, D. S. (1995). Hydrochemical characteristics of the meltwater stream draining from Satopanth –Bhagirath Kharak glacier, Alaknanda basin, Garhwal Himalaya, India [Ph.D. Thesis]. School of Environmental Sciences JNU New Delhi, 142.
- Ferguson, R. I. (1984). Sediment load of the Hunza river. In K. J. Miller (Ed.), International karakoram project (pp. 581–598). Cambridge University Press.
- Folk, R. L., & Ward, W. C. (1957). Brazos river bar: A study in the significance of grain size parameters. Journal of Sedimentary Research, 27(1), 3–26. https://doi.org/10.1306/74D70646-2B21-11D7-8648000102C1865D
- Gardner, J. S. (1986). Recent fluctuations of the Rakhiot glacier, Nanga Parbat, Punjab Himalaya, Pakistan. Journal of Glaciology, 32(112), 527–529. https://doi.org/10.1017/S0022143000012247
- Gibbs, R. J. (1970). Mechanisms controlling world water chemistry. Science, 170(3962), 1088–1090. https://doi.org/10.1126/science.170.3962.1088
- Haldar, S. K., & Tišljar, J. (2014). Sedimentary rocks. In Introduction to mineralogy and petrology (pp. 121–212). Elsevier, Amsterdam.
- Hamphries, D. W. (1992). The preparation of thin section of rocks, minerals, and ceramics. Royal microscopical society. Oxford University Press.
- Hasnain, S. I., & Chauhan, D. S. (1993). Sediment transfer in the glacio-fluvial environment - a Himalayan perspective. Environmental Geology, 22(3), 205–211. https://doi.org/10.1007/BF00767405
- Hasnain, S. I., Jose, P. G., Ahmad, S., & Negi, D. C. (2001). Character of the sub-glacial drainage system in the ablation area of Dokriani glacier, India, as revealed by dye-tracer studies. Journal of Hydrology, 248(1–4), 216–223. https://doi.org/10.1016/S0022-1694(01)00404-8
- Hasnain, S. I., & Thayyen, R. J. (1999). Controls on the major ion chemistry of Dokriani glacier meltwaters, Ganga basin, Garhwal Himalaya, India. Journal of Glaciology, 45(149), 87–92. https://doi.org/10.3189/S0022143000003063
- Hewitt, K. (1967). Ice-front sedimentation and the seasonal effect: A Himalayan example. Transactions of the Institute of British Geographers, 42(42), 93–106. https://doi.org/10.2307/621374
- Ingersoll, R. V., Bullard, T. F., Ford, R. L., Grimm, J. B., Pickle, J. D., & Sales, S. W. (1984). The effect of grain size on detrital modes: A test of the Gazzi-Dickinson point-counting method. Journal of Sedimentary Research, 54(1), 103–116.
- Inoue, J. (1977). Mass Budget of Khumbu Glacier. Journal of the Japanese Society of Snow and Ice, 39(32), 15–19. https://doi.org/10.5331/seppyo.39.Special_15
- Iwata, S., Aoki, T., Kadota, T., Seko, K., & Yamaguchi, S. (2000). Morphological evolution of the debris covers on Khumbu Glacier, Nepal, between 1978 and 1995. In M. Nakawo, C. F. Raymond & A. Fountain (Eds.), Debris-Covered Glaciers, International Association of Hydrological Sciences, 264, 3–11.Proc. Seattle Workshop.
- Kick, W., 1962. Variations of some central asiatic glaciers. Symposium of Obergurgl, variations of the regime of existing glaciers (Vol. 58, pp. 223–229.). IASH.
- Montgomery, D. R., Zabowski, D., Ugolini, R. O., & Hallberg, H.and Spaltenstein, H., (2000). Soils, watershed processes, and marine sediments. International Geophysics, 72(, 159–194.
- Moribayashi, S., & Higuchi, K. (1977). Characteristics of glaciers in the Khumbu region and their recent variations. Journal of the Japanese Society of Snow and Ice, 39(30), 3–6. https://doi.org/10.5331/seppyo.39.Special_3
- Nicholas, P. (1997). Principles of geology. In Groundwater remediation and treatment technologies (pp. 2003–258). https://doi.org/10.1016/B978-081551411-4.50008-9
- Owen, L. A. (1988). Terraces, uplift and climate, Karakoram Mountains, Northern Pakistan [Ph.D. Thesis]. University of Leicester.
- Pandey, S. K. (1999). Aspects of weathering processes and chemical characteristics ofPindari glacier meltwater, Kumaon Himalaya, India [Ph.D. Thesis]. School of Environmental Sciences JNU, 184.
- Pandey, S. K., Singh, A., & Hasnain, S. I. (2002). Grain-size distribution, morphoscopy and elemental chemistry of suspended sediments of Pindari Glacier, Kumaon Himalaya, India. Hydrological Sciences Journal, 47(2), 213–226. https://doi.org/10.1080/02626660209492925
- Pandey, S. K., Singh, A. K., & Hasnain, S. I. (1999). Weathering and geochemical processes controlling solute acquisition in Ganga headwater Bhagirathi river, Garhwal Himalaya, India. Aquatic Geochemistry, 5(4), 357–379. https://doi.org/10.1023/A:1009698016548
- Raju, A. (1993). Chemical characteristics of snow and ice meltwater from Gangotri glacier basin, Bhagirathi valley, India [Ph.D. Thesis]. JNU, 134.
- Scott, C. H. (1992). Contemporary sediment transfer in Himalayan glacial systems [Ph.D. Thesis]. University of Leicester, 352.
- Shroder, J. F., Bishop, M. P., Copland, L., & Sloan, V. F. (2000). Debris covered glaciers and rock glaciers in the Nanga Parbat Himalaya, Pakistan. Geografiska Annaler, 82(1), 17–31. https://doi.org/10.1111/j.0435-3676.2000.00108.x
- Singh, A. K., & Hasnain, S. I. (1998). Major ion chemistry and weathering control in a high altitude basin: Alaknanda River, Garhwal Himalaya, India. Hydrological Sciences Journal, 43(6), 825–843. https://doi.org/10.1080/02626669809492181
- Singh, S. K., Rai, S. K., & Krishnaswami, S. (2008). Sr and Nd isotopes in river sediments from the Ganga basin: Sediment provenance and spatial variability in physical erosion. Journal of Geophysical Research, 113(3), 1–18. https://doi.org/10.1029/2007JF000909
- Singh, V. B., Ramanathan, A. L., & Pottakkal, J. G. (2014). Seasonal variation of the solute and suspended sediment load in Gangotri glacier meltwater, central Himalaya, India. Journal of Asian Earth Science, 79(A), 224–234. https://doi.org/10.1016/j.jseaes.2013.09.010
- Thayyen, R. I., Gergan, J. T., & DobhaL, D. P. (1999). particle size characteristics of suspended sediments and subglacial hydrology of Dokriani glacier. Garhwal Himalaya. India. Hydrological Science Journal, 44(1), 47–61. https://doi.org/10.1080/02626669909492202
- Viers, J., Oliva, P., Dandurand, J. L., Dupré, B., & Gaillardet, J. (2007). Chemical weathering rates, CO2 consumption, and control parameters deduced from the chemical composition of rivers. Treatise on Geochemistry, 5(2), 175–194. https://doi.org/10.1016/B978-0-08-095975-7.00506-4.
- Warburton, J. (1990). An alpine proglacial fluvial sediment budget. Geografiska Annular, 72(3/4), 261–272. https://doi.org/10.1080/04353676.1990.11880322