Abstract
Fish remains are abundant and easily recognizable in many archaeological sites. The origin of such assemblages is generally poorly known. The ability to create small fish bone accumulations is restricted to a small number of predators (including raptors, carnivores and humans). In order to recognize the agents responsible for these concentrations in archaeological sites, we have investigated the taphonomy of the fish remains from otter spraints to determine the potential role of the Eurasian otter, Lutra lutra, in the formation of fossil assemblages as a piscivorous mammal. Currently, few data are available to characterize the accumulation of otter remains in natural or archaeological sites. We analyzed a collection of 29 otter spraints from Bugeat (Corrèze) in central France. We identified the remains of eight fish species: brown trout (Salmo trutta, Salmonidae), perch (Perca fluviatilis, Percidae), pumpkinseed (Lepomis gibbosus, Centrarchidae), and several Cyprinidae, rudd (Scardinius erythrophthalmus), minnow (Phoxinus phoxinus), bullhead (Cottus gobio), and the indeterminate pair chub/dace (Squalius cephalus/Leuciscus leuciscus). The species and the body parts present, along with the reconstruction of the fish weight and observations of any modifications, such as deformation, rounding and polishing, give us insight into otter prey categories and their transformation after digestion. Among the ca. 200 bones constituting the whole fish skeleton, only between six and 39 bones survived depending on the different species. Cyprinids display a high-moderate digestion grade and seem to be the taxon most affected by digestion (64.67%). Based on surface modifications, such as breakage and digestion traces, as well as bone element representation, we produced a set of criteria as a new tool to be applied to the fossil record.
Introduction
The origin of small vertebrate deposits in caves occupied by man during Paleolithic times is a recurrent problem. There are multiple predators that may be involved, separately or together, including birds, carnivores and humans (Bon et al. Citation2011). In the case of fish remains, very few studies have been done to help identify the bone accumulator. This aspect of taphonomy, i.e. the study of processes affecting the remains after death, has been neglected in fish. The first studies on the effects of digestion on fish skeletal elements focused on domestic animals (dogs and pigs) and humans (Jones Citation1984, Citation1986; Nicholson Citation1991), despite the knowledge that some bird and mammalian predators are able to capture and eat fish in great quantities. Erlandson and Moss (Citation2001) identified a large number of predator species that could potentially deposit fish remains in caves located close to the coastline: canids (Vulpes sp., Canis sp.), bear (Ursus sp.) and birds (cormorants, condors, eagles, gulls and Eurasian eagle owl). Nicholson (Citation1991), Le Gall (Citation1999) and Russ (Citation2010) also identified the otter (Lutra lutra) as a potential contributing predator.
There are many descriptions of current assemblages originating from various predators such as nocturnal and diurnal birds of prey (Mayhew Citation1977; Dodson & Wexlar Citation1979; Andrews Citation1990; Laroulandie Citation2002), crocodiles (Fisher Citation1981) and carnivores (Andrews & Evans Citation1983; Andrews Citation1990; Mondini Citation2001; Campmas & Beauval Citation2008). Modern carnivores produce feces containing bones that display varying degrees of damage and digestion. Carnivores, like Mustelidae, produce feces in which the bones tend to be less broken and digested (Andrews Citation1990), but only Meles meles (Mallye et al. Citation2008; Mallye Citation2011) and Martes martes (Andrews Citation1990) have been studied from a taphonomic aspect. Another member of the family, the Eurasian otter (Lutra lutra), lives close to rivers and is a frequent predator of fish, but surprisingly has been poorly studied from a taphonomic point of view (Nicholson Citation1991).
The otter is a semi-aquatic mustelid and is probably the main freshwater fish predator among European mammals. Originally present all over Europe and in North Africa and Asia, it is currently considered a Near Threatened species in many European countries (UICN Citation2016) and of Least Concern in France (UICN France & MNHN Citation2016). It can measure up to 130 cm in length and weigh between 6 and 11 kg. The otter uses its spraints to mark its territory. Generally, spraints are deposited along the riverbanks or on prominent points such as rocks or tree stumps (Moors Citation1980; Bouchardy Citation1986; Rosoux Citation1995). Although the otter is mainly a piscivore, it will also eat crayfish and other aquatic invertebrates, amphibians, reptiles or birds. Its diet varies depending on the season and biotope (Bouchardy Citation1986; Kuhn & Jacques Citation2011). The otter lives close to all aquatic habitats: rivers, lakes, ponds, marshes, and even on the seashore (Nicholson Citation2000).
Within its territory, the otter will use several dens, referred to as holts. They are usually burrows in the banks of rivers in dense forest areas, but otters may also shelter in caves. Some holts are assigned for giving birth and rearing young. Holts can be located up to 249 m away from the river (Ruiz-Olmo et al. Citation2005).
Despite its behavior and diet based on fish, the otter has rarely been considered a potential accumulator of fish remains in cave deposits (Nicholson Citation1991), possibly because its presence is not specifically documented during the Paleolithic.
The aim of this article is to present the first taphonomic analysis of the fish skeletal elements during the consumption and digestion processes in the Eurasian otter, the main piscivorous mammal in Central France. This study is intended to give a picture of the potential role of otters in the formation of fossil assemblages. This will constitute an aid for understanding fish archaeological accumulations and will provide supplementary data to previously existing taphonomic references.
Materials and methods
A total of 29 spraints of Lutra lutra were collected on the banks of the “Petite Vézère”, a small stream close to Bugeat (45°35ʹ54″N, 1°56ʹ58″E, altitude 720 m, Corrèze, France) between 15 December 2011 and 25 January 2012. This river has woody riparian vegetation and is classified as “1st category”. It is populated with fishes such as trout, minnows, stone loach, gudgeon, bullhead and chub.
To clean spraints, several preparation methods have been cited in the literature: Denys et al. (Citation1995), Ludwig et al. (Citation2002), Lemarchand (Citation2007), Fernández-Jalvo and Marín-Monfort (Citation2008) and Kortan et al. (Citation2010).
In the present study, the spraints were cleaned through a sieve (0.5 mm mesh) with fresh tap water and then air-dried on filter paper.
Each bone was identified by anatomic and taxonomic comparison with the reference collection of freshwater fishes of the Muséum National d’Histoire Naturelle (MNHN, Paris), and also by using published keys (Lepiksaar Citation1994; Radu Citation2005). All the remains were examined both macroscopically and microscopically. A binocular microscope was used for the identification of small skeletal elements and for the observation of marks caused by digestion.
For the quantitative analysis, we used the number of identified specimens (NISP) and the minimum number of individuals (MNI). The MNI was estimated from the number of first vertebrae or paired bones and according to the differences in the size of the bones (Poplin Citation1976). Scales were counted separately. Prey sizes were estimated by direct comparison with the specimens from the osteological reference collection of the MNHN.
We also used the formula of Dodson and Wexlar (Citation1979) to calculate the percentage of representation. The numbers of skeletal elements are usually expressed as follows: PR = FO/FT × MNI, where PR is the skeletal representation, FO is the number of skeletal elements in the sample, and FT is the number of skeletal elements in the prey skeleton. This method was adapted for fish skeletal elements and gives an overview, since the results obtained for each spraints were merged to perform a global analysis by family.
For this study, we studied the extent of bone preservation. Surface modification was classified following Nicholson’s method (2000). The percentage of visible surface was adapted from Villa and Mahieu (Citation1991) (). The proportions of bone damage on bone surface are:
Absent: bone is not altered.
Minimal: only 25% of the surface is attacked. The shape of the bone is unchanged.
Moderate: only 50% of the bone’s surface area is preserved. A slight to moderate pitting appears on teeth.
Heavy or extreme: more than 75% of the bone is destroyed. The greater degree of digestion of teeth is observable through damage of the enameloid (Bergot Citation1975).
Results
Species composition of the otter’s diet
Altogether, 9775 bone remains were recovered from the 29 spraints (). Fish represented 82% of the identified remains. Several spraints also contained remains of birds (Passeriformes, NISP = 41 i.e. 0.4% of identified remains), amphibians (Ranidae, NISP = 70; 0.7%), insects (Trichopteridae and Carabidae, NISP = 73; 0.7%) and plants (Caprifoliaceae and Betulaceae, NISP = 44; 0.5%).
Table I. Number of identified specimens per Eurasian otter spraint. NISP: number of identified specimens.
Of all the remains attributed to fish (NISP = 7999), only 48% were identified anatomically and specifically. Among them, 11% belong to the cranial skeleton, and 19% (1529) to the axial skeleton. Bone fragments, neural and hemal spines, and scales represented 70% (5586) of all fish remains.
Eight fish species were identified: brown trout (Salmo trutta), perch (Perca fluviatilis), pumpkinseed (Lepomis gibbosus), rudd (Scardinius erythrophthalmus), minnow (Phoxinus phoxinus), bullhead (Cottus gobio) and the indeterminate pair chub (Squalius cephalus)/dace (Leuciscus leuciscus).
The most frequently represented family was Percidae (NISP = 2142; MNI = 15), followed by Salmonidae (NISP = 1191; MNI = 56), Cyprinidae (NISP = 501; MNI = 29) and Centrarchidae (NISP = 8; MNI = 3).
Size estimations indicated that the fish eaten by otters had a fresh weight ranging between 10 and 200 g. The most abundant weight categories were: less than 10 g for centrarchids (~10 cm); from 10 to 30 g for cyprinids and percids (~10–20 cm); from 100 to 200 g for salmonids (~30 cm).
Bone deformation
Deformation was recorded for vertebrae that were compressed or twisted (2.32%; ), or bearing tooth marks (0.63%). This deformation is the result of bone collapse under tooth pressure and produces a more or less oval morphology of the vertebra centrum. The compressed vertebrae showed a clear alteration of their shape when compared with reference specimens.
Figure 2. Deformation: (a) fresh caudal vertebra of Salmo trutta without compression; (b) compressed caudal vertebra of Salmo sp. from Eurasian otter spraint.
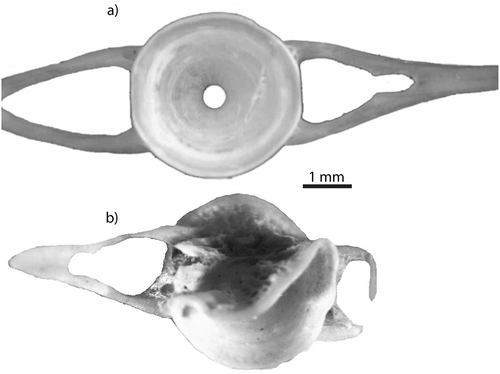
Several bones from each spraint assemblage were chewed or crushed. This damage occurred more often in vertebrae than in head bones. Puncture marks were often seen on the articulating surface of chewed vertebrae.
Rounding and polishing
The action of the gastric juices results in a smooth and polished surface (Andrews Citation1997), but prolonged transportation of bones in water also produces rounding and polishing. In our otter spraints, only digestion can cause such effects. Non-digested fish skeletal elements represented 9.8% of the faunal material. The remains of fish skeletal elements were either moderately (56%) or strongly (34%) altered by gastric juices. The remains examined showed a polished bone surface. The amount of material bearing modification was approximately 90%. The cranial elements appeared to be more sensitive to acid attacks than the vertebrae (32.20% against 67.79%). This criterion may be biased because there were a large number of unspecified skeletal elements, which were not taken into account in this study.
On the scanning electron microscope (SEM) picture (), we recognized advanced rounding of the tooth (Figure 2d) and digestion of most of the enameloid and lightly digested tip teeth (enameloid surface) (). The base of the teeth was heavily digested; enameloid was removed from the base of the teeth and we identified penetration of the enameloid.
Figure 3. Modification of a premaxillary of Salmo trutta (scanning electron microscope; scale: 1 mm): (a) bone after digestion; (b) hole made by gastric juice; (c) enameloid removal; (d) smooth surface on the top of tooth; (e) reference bone of Salmo trutta without digestion.
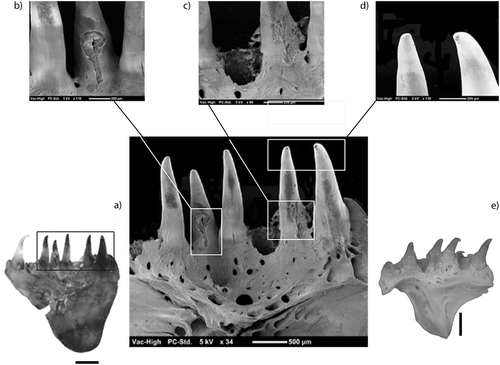
We observe that Cyprinidae, Percidae and Centrarchidae remains display moderate digestion, while Salmonidae ones are more affected by extreme digestion (). The eight preserved remains that are attributed to Centrarchidae were affected by moderate digestion (100%). Cyprinids display high rates of digested skeletal elements, and they are all affected by moderate digestion (65%), followed by percids (63%) and salmonids (51%). Salmonidae was the taxon most affected by heavy digestion (43%).
Table II. Percentage and classes of digestion (without perch scales) for fish taxon by number of remains (NR) and percentage.
Fragmentation and loss of skeletal elements
The otter accumulations are here characterized by the simultaneous presence of complete and fragmented skeletal elements in the same spraint (). In total there are 9.7% complete skeletal elements and 90.3% fragmented elements in the samples.
Survival rate
In our study, only 39 skeletal elements, from approximately 200 bones, survived the digestive process, and showed strong evidence of having been partially digested (; ). Considering the total number of skeletal elements in the entire skeleton of fish and the number of individuals concerned, this was a very low survival rate (19%).
Figure 5. Skeletal elements before and after digestion in otter spraints (Perch skeleton, modified from Coutureau Citation2005). For abbreviations see .
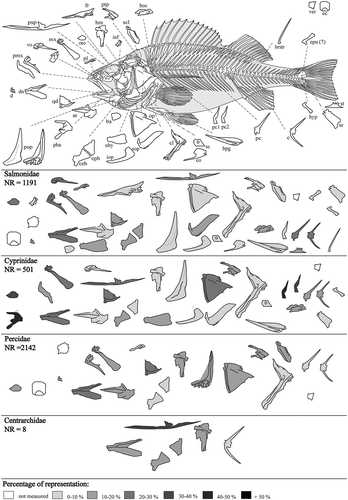
Table III. Number of identified specimens (NISP) by bones and fish species in the 29 Eurasian otter spraints.
The percentage of representation was calculated according to Dodson and Wexlar (Citation1979) and adapted to fish osteology. Overall, the percentage of representation in all species is low. Bone representation is characterized by good preservation of the parasphenoid in Centrarchidae (PR = 33.33%), the dentary and quadrate in Percidae (PR = 23.33%), the pharyngeal bone and first vertebra in Cyprinidae (PR = 44.82 %), and the dentary in Salmonidae (PR = 28.57%). In total, the axial skeleton represents 54% of the studied material. The axial skeleton is better preserved in Salmonidae, at 69%. In the other taxa the preservation of the axial skeleton is 24% for Cyprinidae, 7% for Percidae and 0.3% for Centrarchidae. The cranial elements are more abundant, with 44% for Salmonidae, 38% for Cyprinidae, 17% for Percidae and only 0.7% for Centrarchidae.
Discussion
Otter is an opportunistic carnivore that adapts to food availability. Many studies concerning its diet have been carried out in France. According to Libois et al. (Citation1991), eels dominate the food spectrum in the Marais Poitevin. In the Massif Central, Libois (Citation1997) found a more diversified diet, dominated by small size species: bullhead (Cottus gobio), stickleback (Gasterosteidae), minnow (Phoxinus phoxinus), loach (Barbatula barbatula) and gudgeon (Gobio gobio), followed by trout and various cyprinids. In all these studies, evidence of consumption of amphibians, mostly Rana spp. and Pelophylax spp., is attested (Libois & Rosoux Citation1991; Lanszki et al. Citation2006). Toads and urodeles are usually not consumed because of their poisonous glands (Adrian & Delibes Citation1987). Invertebrates such as crayfish are commonly eaten. The species consumed are not necessarily confined to the aquatic environment. Consumption of snakes, lizards or turtles is also rarely mentioned (Libois et al. Citation1991; Lemarchand Citation2007). Birds represent a small part of the diet, and consist mostly of anatids and rallids. Mammals are infrequent. The Eurasian otter can also eat carrion. The presence of pork, deer and dog in spraints (Libois Citation1997; Lanszki et al. Citation2001; Georgiev Citation2006) probably originates from scavenging.
Erlinge (Citation1968) indicates that the fish eaten by otters measure between 15 and 17 cm. However, in the wild, Eurasian otters do not display such prey size selection; they consume mainly small- and medium-sized fish, as well as bigger specimens. The proportion of the different types of prey in an otter’s diet does not necessarily reflect their abundance in nature since the otter may exert selection (Libois & Rosoux Citation1989, Citation1991). In the Marais Poitevin, otters preferentially consume eel, tench and rudd, but underexploit roach, which exists in large numbers in this environment (Libois et al. Citation1991). Erlinge (Citation1968) postulates that otters have a preference for easy prey.
All species present in our study are coherent with the general diet of otters and with fish fauna currently living in French rivers. We found a predominance of trout remains, a species which is rather lively and hard to catch. This may seem contradictory to the idea that otters favor easy prey, but trout seems to be the dominant species in the small stream we studied.
Otters consume fish starting from the head. If they do not eat the fish in the water, they drag it on the ground, sometimes over several tens of meters (P. Martin, pers. comm.). Bouchardy (Citation1986) indicates that in otters, the method of ingestion depends on the fish species, the size of the fish, and the age of the individual that consumes it. Only the fleshy parts are consumed. The partial consumption of large-sized fish may induce a bias in the faunal inventory of spraints as well as in archaeological sites. Hewson (Citation1995) indicated that, in Scotland, otters consume the flesh from salmon carcasses after spawning. The skeletal elements of these specimens will therefore not be found in spraints, and the consumption does not leave traces on the bones because they are not digested. This scavenging behavior may be related to opportunism or a difficulty in finding live prey (Kuhn & Jacques Citation2011).
Nicholson (Citation2000) studied the traces left by otters frequenting the coasts of Northern Scotland and the Northern Isles. She demonstrated that chewed or crushed bones “were more often vertebrae than head bones” and vertebrae also showed signs of puncture marks. She found no evidence of dissolution, but many of the bones were stained by the mucus that held the spraints together.
The impact of digestion on the skeleton varies according to the enzymes and acidity (pH) of the digestive system of the predator (Denys et al. Citation1995). The age of the predator and the spraints, the type of bone, and the duration of exposure to acids and enzymes can alter this impact.
Following Dodson and Wexlar (Citation1979) and Andrews (Citation1990), the representation of anatomical elements, fragmentation, and state of bone surfaces and dental characteristics of a type of predator has led to the organization of five broad categories of modifications (light, intermediate, moderate, great and extreme modifications), depending on the intensity of the digestion and the type of predator. The smaller, less dense or more porous bones will degrade more rapidly than will the more highly mineralized bone of large adults, and might be under-represented in archaeological studies (Lyman Citation1984; Nicholson Citation1996). Several experiments on the survival of fish skeletal elements during digestion were carried out in particular by Jones (Citation1986) and Nicholson (Citation1993), with examples of digestion in dog, pig and man. These studies found that 85 to 100% of fish skeletal elements had been destroyed by digestion in these mammals. The bones that had survived showed signs of compression, dissolution and rounding.
In our study, we observed some of the modifications mentioned by Nicholson (chewed and crushed bones). The bone examination with an SEM showed traces of dissolution, but puncture marks were not observed. Pitting was not seen on fish bones and is in any case difficult to distinguish on salmonids because their vertebrae are naturally porous. Due to the varying size of bones, samples are affected in a different way; however, it seems that there is no correlation with fish size or species. Eurasian otter should be included in Andrews’ (Citation1990) category 5. Several studies have demonstrated that element destruction is strongly correlated with the structural density of bones (Butler Citation1996). Lyman and O’Brien (Citation1987) explained that other properties, including shape and size, affect identification. Differences in skeletal design, as well as in the histological structure of fish bone, may affect the density of bone (Nicholson Citation1992). This could explain the difference of representation between species. We also noticed that the majority of the bones that were preserved were vertebrae or the most compact cranial bone parts. Other cranial bones were broken into very small pieces and were more digested. Sometimes, they were very difficult to identify, a fact that contributes to an underestimated survival rate. The under-represented regions may reflect their low survivorship due to environmental factors (Lyman Citation1984; Zohar et al. Citation2001). The porosity of fish bone is particularly hard to define because of the “foliate” appearance of many skeletal elements, which demonstrate an irregular design, with frequent folds and cavities. It is difficult therefore to generalize about the relative density of fish when only a restricted range of bones is considered (Nicholson Citation1992).
Our study of 29 spraints, which were produced by Eurasian otter, has permitted us to characterize the damage caused to bones during digestion. The assemblage studied consisted of 3842 identified remains. The most abundant identifiable skeletal parts were vertebrae. The fish specimens identified in our sample were generally small, and the most frequently found species was the trout. Most of the remains (90%) showed some characteristic signs of biochemical modifications, especially rounding and polishing. We also found fragmentation and loss of bones. This study confirms previous work by finding the same modifications and in the present case a high percentage of altered bones (from 56% for moderate digestion to 34% for heavy digestion) (Nicholson Citation1991; Russ Citation2010). Digestion in the otter produces feces in which the bones tend to be broken and digested like in most other mammalian carnivores. For example, Andrews and Evans (Citation1983), Andrews (Citation1990) and Matthews (Citation2002) have shown that feces of small felids generally contain extremely broken bones with high levels of rounding and polishing produced by digestion. They also found that scats of small canids contain digested bones, but the degree of breakage is slightly less than in small felids. Claw marks and traces of digestion can also be seen.
The study of modern predator behavior offers important insights into potential predators at archaeological sites. During the Paleolithic, when the caves were not occupied by humans, other species could potentially create fish bone deposits in front of or in the cave. Fish are included in the diets of many potential occupants; therefore, identifying the accumulation agent is complex. Erlandson and Moss (Citation2001) have identified a large number of species that could potentially deposit fish remains in caves located close to the coast, including bears and Eurasian eagle owl (Bubo bubo). Russ and Jones’s (Citation2011) experimental research on four female brown bears showed that bears eat salmonids of greater than 30 cm in total length. Bears selected head elements, but whole fish were also consumed (Russ & Jones Citation2011). The capture occurs during the spawning season (Robbins et al. Citation2004), and bears consume fish close to the water. Undigested parts are deposited in or close to the water, and feces are deposited in the wider environment. The results of Russ and Jones (Citation2011) may not be representative of the impacts of digestion because the number of bears in their sample is low, but they provide an image of a feeding event.
The bone assemblages produced by raptors are characterized by their degree of digestion. Fragmentation is secondary in comparison to that in mammals. Some pitting can be observed, and broken edges are rounded. Sea birds eating fish tend to regurgitate pellets near the feeding zone (Nicholson Citation1991). The Eurasian eagle owl has been identified as an accumulation agent at several archaeological cave sites (Andrews Citation1990; Laroulandie Citation2002).
Russ and Jones’s (Citation2011) experiment focused on the feeding habits of three eagle owls. Only one of the three eagle owls consumed a fish, and this was achieved by disguising a fish inside a rat. Russ and Jones (Citation2011) indicated minimal damage to any bone element, but their encasing of the fish into a rat body during ingestion potentially protected the fish bones. The whole fish was eaten, head first. The fish specimens were small and medium in size, up to 40 cm in total length. In their natural environment, the eagle owl captures fish from spring to autumn. Also for Bubo bubo, digestion is less destructive than in mammal carnivores; fish bones display lower rates of digestion and are less broken because these birds generally swallow the whole fish without cracking it. The accumulation of fish bones, in this case, was concentrated in the nest or immediately surrounding area (Russ Citation2010). In constrast, the otter marks prominent rocks with its spraints, which are more dispersible. Otters seem to consume fish near the shore. There is no mention of the discovery of spraints in living areas. However, naturalists have observed occasional otter occupation at the entrance to caves or rock-shelters that are located near rivers (Rosoux et al. Citation2015).
The accumulation agent can be identified by establishing taphonomic signatures for any possible agent of deposition. It is necessary to know the habits of potential accumulation species, as well as the element representation, fragmentation, surface modification and spatial distribution of the bones, and the size of digested fish. The comparative analysis of bone accumulations provides criteria for distinguishing potential accumulators.
Conclusion
Fish remains from archaeological cave sites are generally considered to be the result of human activities. However, when caves were not occupied by human groups, other species could enter and potentially create fish bone deposits. The present work proposes criteria for analyzing remains from current spraints of otters, a potential fish bone accumulator. Our study shows that digestive processes often modify fish skeletal elements through rounding, deformation or fragmentation. Focusing a taphonomic study only on the presence of digestive remains is insufficient to identify the predator. We need to establish a complete taphonomic signature. Several criteria need to be taken into account, such as bone representation, surface modification and fragmentation, size of fishes, species representation, and the spatial distribution of bone accumulations. One of the problems in archaeology is that fish remains will often be identified and interpreted separately from other faunal remains. In order to identify the potential predator we must, however, consider the faunal register as a whole, especially when fish is mixed with the microfauna.
Acknowledgements
This study was funded by a doctoral fellowship granted by the Muséum National d’Histoire Naturelle (Paris). Many people deserve thanks for their contributions. We would like to particularly thank Marie-Pierre Ruas, Salvador Bailon and Jean-Bernard Huchet for their help with the taxonomic identification of plants, amphibians and insects, respectively. We would also like to thank Michel Lemoine for making the SEM pictures with the neoscope of the “plateau archébotanique de l’UMR 7209 équipement programme CoBota-IdF”. We also thank Philip Hindley and Eréndira Quintana Morales for the English proofreading and for their valuable comments.
The authors express their thanks also to the anonymous reviewers for their valuable critical comments.
Additional information
Funding
References
- Adrian MI, Delibes M. 1987. Food habits of the otter (Lutra lutra) in two habitats of the Doñana National Park, SW Spain. Journal of Zoology 212:399–406. DOI: 10.1111/jzo.1987.212.issue-3.
- Andrews P. 1990. Owls, caves and fossils. Chicago, IL: University of Chicago Press.
- Andrews P. 1997. What taphonomy can and cannot tell us. Cuernos de Geología Ibérica 32:53–72.
- Andrews P, Evans EN. 1983. Small mammal bone accumulations produced by mammalian carnivores. Paleobiology 9:289–307. DOI: 10.1017/S0094837300007703.
- Bergot C. 1975. Morphogenèse et structure des dents d’un téléostéen (Salmo fario L.). Journal de biologie buccale 3:301–321.
- Bon C, Berthonaud V, Fosse P, Gély B, Maksud F, Vitalis R, Philippe M, Van der Plicht J, Elalouf J-M. 2011. Low regional diversity of late cave bears mitochondrial DNA at the time of Chauvet Aurignacian paintings. Journal of Archaeological Science 38:1886–1895. DOI: 10.1016/j.jas.2011.03.033.
- Bouchardy C. 1986. La loutre d’Europe. Paris: Sang de la Terre.
- Butler VL. 1996. Tui chub taphonomy and the importance of marsh resources in the western Great Basin of North America. American Antiquity 61:699–717. DOI: 10.2307/282012.
- Campmas É, Beauval C. 2008. Consommation osseuse des carnivores : résultats de l’étude de l’exploitation de carcasses de bœufs (Bos taurus) par des loups captifs. Annales de Paléontologie 94:167–186. DOI: 10.1016/j.annpal.2008.06.001.
- Coutureau M. 2005. La perche. ArcheoZoo.org.
- Denys C, Fernández-Jalvo Y, Dauphin Y. 1995. Experimental taphonomy: Preliminary results of the digestion of micromammal bones in the laboratory. Comptes rendus de l’Académie des sciences 321:803–809.
- Dodson P, Wexlar D. 1979. Taphonomic investigations of owl pellets. Paleobiology 5:275–284. DOI: 10.1017/S0094837300006564.
- Erlandson JM, Moss ML. 2001. Shellfish feeders, carrion eaters and the archaeology of aquatic adaptations. American Antiquity 66:413–432. DOI: 10.2307/2694242.
- Erlinge S. 1968. Food studies on captive otters Lutra lutra L. Oikos 19:259–270. DOI: 10.2307/3565013.
- Fernández-Jalvo Y, Marín-Monfort MD. 2008. Experimental taphonomy in museums: Preparation protocols for skeletons and fossil vertebrates under the scanning electron microscopy. Geobios 41:157–181. DOI: 10.1016/j.geobios.2006.06.006.
- Fisher DC. 1981. Crocodilian scatology, microvertebrate concentrations and enamel less teeth. Paleobiology 7:262–275. DOI: 10.1017/S0094837300004048.
- Georgiev D. 2006. Diet of the otter Lutra lutra in different habitats of South Eastern Bulgaria. IUCN/SCC Otter Specialist Group Bulletin 23:5–11.
- Hewson R. 1995. Use of salmonid carcasses by vertebrate scavengers. Journal of Zoology 235:53–65. DOI: 10.1111/jzo.1995.235.issue-1.
- Jones AKG. 1984. Some effects of the mammalian digestive system on fish bones. In: Desse-Berset N, editor. 2nd fish osteoarchaeology meeting. Paris: Centre National de la Recherche Scientifique. pp. 61–65.
- Jones AKG. 1986. Fish bone survival in the digestive systems of the pig, dog and man: Some experiments. In: Brinkhuizen DC, Clason AT, editors. Fish and archaeology; studies in osteometry, taphonomy, seasonality and fishing methods. BAR international series 294. Oxford: BAR International. pp. 53–61.
- Kortan D, Adámek Z, Vrána P. 2010. Otter, Lutra lutra, feeding pattern in the Kamenice river (Czech Republic) with newly established atlantic salmon, Salmo salar, population. Folia Zoologica 59:223–230.
- Kuhn R, Jacques H. 2011. La loutre d’Europe Lutra lutra (Linnaeus, 1758). Encyclopédie des carnivores de France 8. Bourges: Société Française pour l’Etude et la Protection des Mammifères.
- Lanszki J, Körmendi S, Hancz C, Martin T. 2001. Examination of some factors affecting selection of fish prey by otters (Lutra lutra) living by eutrophic fish ponds. Journal of Zoology 255:97–103. DOI: 10.1017/S0952836901001145.
- Lanszki J, Molnár M, Molnár T. 2006. Factors affecting the predation of otter (Lutra lutra) on European pond turtle (Emys orbicularis). Journal of Zoology 270:219–226. DOI: 10.1111/jzo.2006.270.issue-2.
- Laroulandie V. 2002. Damage to pigeon long bones in pellets of the eagle owl Bubo bubo and food remains of peregrine Falcon Falco peregrinus: Zooarchaeological implications. In: Bocheński ZM, Bocheński Z, Stewart J, editors. Proceedings of the 4th Meeting of the ICAZ Bird Working Group, Kraków, Poland, 11–15 September, 2001. Acta Zoologica Cracoviensia 45(special issue):331–339.
- Le Gall O. 1999. Ichtyophagie et pêches préhistoriques. Quelques données de l’Europe occidentale. Unpublished PhD thesis, Bordeaux I University.
- Lemarchand C. 2007. Etude de l’habitat de la loutre d’Europe (Lutra lutra) en région Auvergne (France) : relations entre le régime alimentaire et la dynamique de composés essentiels et éléments toxiques. Unpublished PhD thesis, Clermont-Ferrand University.
- Lepiksaar J. 1994. Introduction to osteology of fishes for paleozoologists. Göteborg.
- Libois RM. 1997. Régime et tactique alimentaire de la loutre (Lutra lutra) dans le Massif Central. Vie et Milieu 47:33–35.
- Libois RM, Rosoux R. 1989. Ecologie de la loutre (Lutra lutra) dans le Marais Poitevin-I. Etude de la consommation d’anguille (Anguilla anguilla). Vie et Milieu 39:191–197.
- Libois RM, Rosoux R. 1991. Ecologie de la loutre (Lutra lutra) dans le Marais Poitevin-II. Aperçu général du régime alimentaire. Mammalia 55:35–47.
- Libois RM, Rosoux R, Delooz E. 1991. Ecologie de la loutre (Lutra lutra) dans le Marais Poitevin-III. Variations du régime et tactique alimentaire. Cahiers d’Ethologie 11:31–50.
- Ludwig GX, Hokka V, Sulkava R, Ylönen H. 2002. Otter Lutra lutra predation on farmed and free-living salmonids in boreal freshwater habitats. Wildlife Biology 8:193–199.
- Lyman RL. 1984. Bone density and differential survivorship of fossil classes. Journal of Anthropological Archaeology 3:259–299. DOI: 10.1016/0278-4165(84)90004-7.
- Lyman RL, O’Brien MJ. 1987. Plow-zone zooarchaeology: Fragmentation and identifiability. Journal of Field Archaeology 14:493–498. DOI: 10.2307/530237.
- Mallye JB. 2011. Taphonomie et archéozoologie d’un animal fouisseur: méthode de caractéristation des accumulations de restes de Blaireau. In: Laroulandie V, Mallye JB, Denys C, editors. Taphonomie des Petits Vertébrés: Référentiels et transferts aux fossiles. Actes de la Table ronde du RTP Taphonomie. BAR International Series 2269. Oxford: British Archaeological Reports. pp. 43–56.
- Mallye J-B, Cochard D, Laroulandie V. 2008. Accumulations osseuses en périphérie de terriers de petits carnivores : les stigmates de prédation et de fréquentation. Annales de Paléontologie 94:187–208. DOI: 10.1016/j.annpal.2008.06.002.
- Matthews T. 2002. South African micromammals and predators: Some comparative results. Archaeometry 44:363–370. DOI: 10.1111/arch.2002.44.issue-3.
- Mayhew DF. 1977. Avian predators as accumulators of fossil mammal material. Boreas 6:25–31. DOI: 10.1111/j.1502-3885.1977.tb00693.x.
- Mondini M. 2001. Taphonomic action of foxes in Punta Rockshelters: A case study in Antofogasta de la Sierra (Province of Catamarca, Argentina). In: Kuznar LA, editor. Ethnoarchaeology of Andean South America. Contributions to archaeological method and theory. Ethnoarchaeological series 4. Ann Arbor, MI: International Monographs in Prehistory. pp. 266–295.
- Moors PJ. 1980. Sexual dimorphism in the body size of mustelids (Carnivora): The roles of food habits and breeding systems. Oikos 34:147–158. DOI: 10.2307/3544175.
- Nicholson RA. 1991. An investigation into variability within archaeologically recovered assemblages of faunal remains: The influence of pre-depositional taphonomic factors. Unpublished PhD thesis, York University.
- Nicholson RA. 1992. An assessment of the value of bone density measurements to archaeoichtyological studies. International Journal of Osteoarchaeology 2:139–154. DOI: 10.1002/oa.1390020206.
- Nicholson RA. 1993. An investigation into the effects on fish bone on passage through the human gut: Some experiments and comparisons with archaeological material. Circaea 10:38–51.
- Nicholson RA. 1996. Bone degradation, burial medium and species representation: Debunking the myths, an experiment-based approach. Journal of Archaeological Science 23:513–533. DOI: 10.1006/jasc.1996.0049.
- Nicholson RA. 2000. Otter (Lutra lutra L.) spraint: An investigation into possible sources of small fish bones at coastal archaeological sites. In: Huntley JP, Stallibrass S, editors. Taphonomy and interpretation. Oxford: Oxbow. pp. 55–64.
- Poplin F. 1976. A propos du Nombre de Restes et du Nombre d’Individus dans les échantillons d’ossements. Cahiers du Centre de Recherches Préhistoriques 5:61–74.
- Radu V. 2005. Atlas for the identification of bony fish bones from archaeological sites, Asociatia romana de archeologie, studii de preistorie supplementum. Bucarest: Editura contrast.
- Robbins CT, Schwartz CC, Felicetti LA. 2004. Nutritional ecology of ursids: A review of newer methods and management implications. Ursus 15:161–171. DOI: 10.2192/1537-6176(2004)015<0161:NEOUAR>2.0.CO;2.
- Rosoux R. 1995. Cycle journalier d’activités et utilisation des domaines vitaux chez la loutre d’Europe (Lutra lutra) dans le marais poitevin (France). Cahiers d’Ethologie 15:283–306.
- Rosoux R, De Bellefroid MN, Libois R. 2015. Le mystère des loutres d’Arcy-sur-Cure. Revue scientifique Bourgogne Nature 21/22:99–108.
- Ruiz-Olmo J, Batet A, Jiménez J, Martínez D. 2005. Habitat selection by female otters with small cubs in freshwater habitats in northeast Spain. Lutra 48:45–56.
- Russ H. 2010. The Eurasian eagle owl (Bubo bubo): A fish bone accumulator on Pleistocene cave sites? Journal of Taphonomy 8:281–290.
- Russ H, Jones AKG. 2011. Fish remains in cave deposits; how did they get there? Cave and Karst Science 38:57–60.
- UICN (International Union for Conservation of Nature). 2016. The IUCN red list of threatened species. Version 2016-2. Available: http://www.iucnredlist.org. Accessed Sep 2016 4.
- UICN France & MNHN. 2016. La Liste rouge des espèces menacées en France. Paris: UICN France & MNHN (2008–2015).
- Villa P, Mahieu E. 1991. Breakage patterns of human long bones. Journal of Human Evolution 21:27–48. DOI: 10.1016/0047-2484(91)90034-S.
- Zohar I, Dayan T, Galili E, Spanier E. 2001. Fish processing during the early Holocene: A taphonomic case study from coastal Israel. Journal of Archaeological Science 28:1041–1053. DOI: 10.1006/jasc.2000.0630.