Abstract
The present study investigates the aspects of endocrine cells secreting cholecystokinin, gastrin, insulin, glucagon, somatostatin and serotonin in the enteropancreatic system of Tropidurus torquatus. The specimens were collected in Marambaia Island, Sepetiba Bay, Rio de Janeiro State, Brazil. The animals’ intestine and pancreas were removed, fixed, processed and then subjected to immunohistochemical techniques. Three cell types, immunoreactive (IR) to gastrin, cholecystokinin-8 and serotonin, were identified in the intestinal mucosa. Serotonin was found in the small and large intestines, whereas gastrin and cholecystokinin-8 cells were only observed in the large intestine of T. torquatus. Glucagon, somatostatin and insulin were not observed in the intestines at all. Only glucagon, somatostatin and insulin were identified in the pancreas of the studied lizards. The dorsal lobe showed a large number of endocrine cells scattered throughout the exocrine parenchyma and in the exocrine duct walls. The present study shows that the intestine and pancreas of T. torquatus hold most of the regulatory peptides, presenting a structure similar to that in other vertebrates such as birds and mammals. The endocrine cells identified in the lizards’ enteropancreatic system evidences the good phylogenetic preservation of the regulatory peptides’ molecular structure.
Introduction
Reptiles are among the most ecologically and evolutionarily remarkable groups of living organisms since they have successfully colonized most of the planet, including oceans and some of the harshest and most environmentally unstable ecosystems on Earth (Pincheira-Donoso et al. Citation2013). Reptiles have accumulated a vast diversity of morphological, behavioral, ecological, life-history and defensive strategies to cope with the selective demands they have encountered due to exposure to hundreds of millions of years of radiation (Pianka & Vitt Citation2003; Pough et al. Citation2004; Fry et al. Citation2006). Apparently, reptiles are a useful future model – even more useful than other commonly used experimental animals such as rats, rabbits and pigs – for studies focusing on the physiological regulation of the digestive process, because of their good responses to feeding (Secor & Diamond Citation1998); therefore, they have become an important study object in different fields.
Tropidurus is one of the most characteristic genera occupying open environments in South America (Frost et al. Citation2001). The diversity of species belonging to this genus has grown over the years; recently the total number of species increased to 23 (Passos et al. Citation2011). This is one of the most broadly distributed lizard genera in South America, since the species in this genus are found from Northern Venezuela (and one locality by the Colombian border), Guyana, Suriname and French Guiana to Northern Argentina and Southern Uruguay. Their distribution covers almost the entire Brazilian territory and extends west to Bolivia and Paraguay (Carvalho Citation2013). Tropidurus torquatus (Wied-Neuwied 1820) is a species of general habits, and opportunistic foraging of the “sit-and-wait” type, and it may change its habits depending on the environment. Although its diet is little restricted, studies show its eating preference for arthropods and, mostly, for vegetal contents (Teixeira & Giovanelli Citation1999; Siqueira et al. Citation2013; Vitt & Caldwell Citation2014).
Histological and immunohistochemical studies may help in understanding the species’ habits since the anatomy of reptiles’ gastrointestinal tract (GIT) changes according to order, studied species and foraging habitats. Many hormones, such as the regulator peptides, appear to act in the integration of the innumerable functions performed by the body; besides, they perform important regulatory actions in the physiological function of digestive tract cells (Fujita Citation1990; Larsson Citation2000; Huang & Wu Citation2005; Pereira et al. Citation2015). Moreover, secretory cells play an important role in lubricating the organ and protecting it from pro-teolytic degeneration and pathogenic microorganisms (Reid et al. Citation1988).
Several functions of different GIT segments are controlled by endocrine cells, forming a complex system able to secrete physiologically active polypeptide hormones and amines, which are disseminated among the epithelial components (Carvalheira et al. Citation1968). According to Deveney and Way (Citation1983), the gastrointestinal hormones secreted by endocrine cells have important functions in the overall regulation of the digestive process, such as nutrient absorption, gut motility and intestinal blood flow. The endocrine cells in the lizard’s guts can vary in frequency and distribution depending on the species. Therefore, the aim of the present study was to determine the regional distribution and quantitative frequency of endocrine cells in the intestine and pancreas of T. torquatus through immunohistochemistry, as well as to improve the knowledge of the cell composition of the gastroenteropancreatic (GEP) system of reptiles.
Materials and methods
Collecting and preparing the biological material
Seven adult animals (four females and three males) were used in the experiment. They were captured in restingas found in the Marambaia peninsula (23°04ʹS, 43°53ʹW), Sepetiba Bay, Rio de Janeiro State, Brazil. The collected specimens were taken to the Histology and Embryology Laboratory of Rio de Janeiro Federal Rural University (UFRRJ) where they were sexed. The subjects were euthanized using 0.5% lidocaine, as recommended for reptile sacrifice in Resolution 714 from 2002, which was issued by the Federal Council of Veterinary Medicine. The current research was approved by the Ethics Research Committee of UFRRJ – process number 23083.012480/2010-64.
The animals’ coelomic cavities were opened, completely exposing the viscera, and intestine and pancreas were removed. The intestine was divided into small and large (). The pancreas was divided into two lobes, the dorsal lobe along the small intestine, and the ventral lobe close to the spleen. The tissues were fixed in Bouin’s liquid for 6 hours and dehydrated through a graded series of ethanol solutions, and embedded in Histosec (Merk, Darmstadt, Germany) using routine protocols. Histological sections were cut with a rotary microtome to 5 μm thickness and mounted on glass slides pre-coated with 0.1% poly-l-lysine (Sigma-Aldrich, Inc. USA).
Immunohistochemical study
The sections for the immunohistochemical procedure were dewaxed and rehydrated according to the routine protocol. They were incubated in citrate buffer (pH 6.0–0.01 M) and placed in a microwave oven for 15 min, for antigen recovery. Subsequently, the sections were incubated in 3% H2O2 solution in methanol for 15 min to block any endogenous peroxidase. Next, they were incubated in 1:100 bovine serum albumin diluted (B4287; Sigma–Aldrich, Inc. USA) in phosphate-buffered saline (PBS) solution, for 30 min, in a humid chamber, at room temperature.
The intestine and pancreas sections were first incubated overnight at 4°C in the respective primary antibodies (). Next, the sections were incubated in biotinylated “Universal” secondary antibody diluted at 1:200 for 30 min, and in avidin-biotin-peroxidase complex (ABC), diluted at 1:200, for 30 min (both from PK 6200, Vector Laboratories, Inc., USA). Subsequently, the peroxidase label was revealed through the reaction with Stable DAB/Plus (K 047, Diagnostic BioSystems, Inc., USA), which was prepared according to the instructions of the manufacturer. All dilutions and thorough washes, between stages, were performed using PBS (pH 7.4). The sections were counterstained in Harris’ hematoxylin, rinsed in deionized water, dehydrated through a series of ethanol and methylcyclohexane solutions, and mounted in Entellan (Merck & Co., USA) medium.
Table I. Details of primary antibodies used in this study.
The polyclonal antisera produced by mammals were used because of the difficulty in obtaining T. torquatus antibodies against the studied proteins, and because these proteins are conserved at the phylogenetic scale (Huang & Wu Citation2005; Zhang & Wu Citation2009; Chandavar & Naik Citation2011; Hao et al. Citation2012).
Negative and positive controls were used to investigate the specificity of the reactions. Bat intestine sections were used as positive controls, because they presented positive responses to these reactions in previous studies (e.g., Santos et al. Citation2008a, Citation2008b; Machado-Santos et al. Citation2009). The negative control was prepared by replacing the primary antibody with non-immune serum and PBS (pH 7.4).
Observation, photomicrography and cell counting
was made in a Leica M205C Stereomicroscope equipped with a photographic camera. The final photo is a result of the stacking process made with the software Leica Application Suite Version 4.5.
Figure 1. Anatomy of the gastroenteropancreatic system of Tropidurus torquatus. The esophagus, stomach, pancreas and intestine (small intestine and large intestine) are depicted.
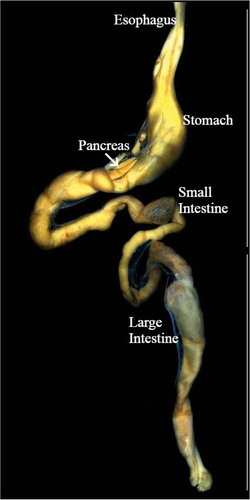
The intestine and pancreas photomicrographs from seven specimens were taken using a digital camera (Nikon Coolpix 4300) attached to the microscope (Olympus BX41). Ten fields per 10 (20× objective) intestinal mucosa, islet-like and exocrine region sections from each specimen were analyzed. The relative endocrine cell immunoreactive (IR) frequency was measured in a computerized image analyzer (Image-J software). The immunoreactive cell frequency was expressed as mean ± standard deviation (SD) per unit area (mm2) of mucosa.
Results
Gastrin-, cholecystokinin-8- and serotonin (5-hydroxytryptamine)-IR cells were identified in the intestine of T. torquatus, but glucagon-, somatostatin- and insulin-IR cells were not observed there. Glucagon-, somatostatin- and insulin-IR cells were identified in the pancreas. Two different endocrine cell types were found in the enteropancreatic system. One cell type was classified as spindle-shaped (open-typed cells), because of its elongated spindle and pyriform shapes, as well as the narrow apex pointing toward the lumen. The other cell type was classified as spherical (closed-type cells or interstitial) due to its round and spherical features.
Intestine
The distribution patterns and relative frequencies of the endocrine cells in the intestine of Tropidurus torquatus are shown in .
Table II. Distribution and frequency of the endocrine cells of the intestine and pancreas of Tropidurus torquatus (mean ± standard deviation). NF = not found; CCK: cholecystokinin.
Gastrin-IR cells were detected in the small intestine only of T. torquatus ()–()). These IR cells were the second most predominant cell type in the intestine of the lizards studied herein (). The gastrin-stained cells changed from spherical to spindle-shaped. The spindle-shaped gastrin-positive cells, which present relatively short cytoplasmic processes, were detected in the epithelia of the small intestine (b)). Spherical gastrin-positive cells were observed in the epithelial intestinal mucosa (c)).
Figure 2. Photomicrographs of gastrin-immunoreactive (IR) cells in the intestine of Tropidurus torquatus. (A–C) Small intestine. A, Gastrin-IR cells between the epithelial cells (arrows) (270×). B, Gastrin-IR cells of the epithelium of the intestinal folds, a few of the open type (arrow) and most of the closed type (740×). C, Highlighting the existence of closed cell types (arrows; 1000×).
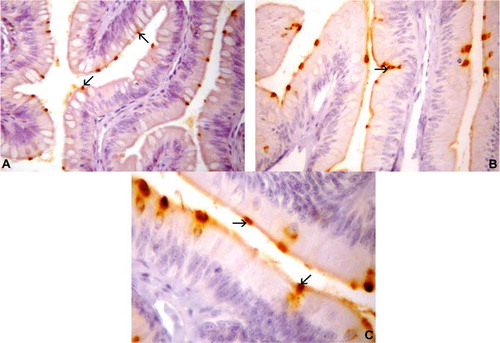
Cholecystokinin-8-IR cells were the least abundant cell type in the intestine of the studied lizards (); their shape changed from spherical to spindle-like, similar to the gastrin-IR cells. Some cells were of the open type presenting cytoplasmic processes, which were detected in the epithelia of the small intestine ()), but most cells were of the closed type (b)).
Figure 3. Photomicrographs of cholecystokinin-immunoreactive (CCK-IR) cells in the intestine of Tropidurus torquatus. (A–B) Small intestine. A, CCK-IR cells between the cells of the intestinal epithelium, most of the closed type with a few of the open type (arrows; 870×). B, The presence of CCK-IR cells between the cells of the intestinal epithelium, all of the closed type, can be observed (740×).
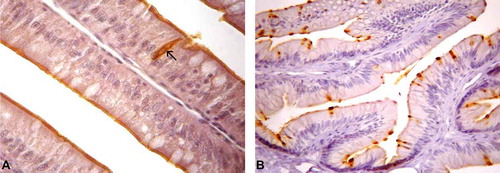
Serotonin-positive cells showed different relative frequencies throughout the intestinal tract, including the small (–()) and large intestines ((d) and (e)) of T. torquatus. These cells were the most predominant type found in the current study (). The spindle-shaped cells had relatively long cytoplasmic processes, which ended in the lumen; they were detected in the epithelia of the small and large intestines (a) and (e)). Spherical cells were dispersed in the small and large intestinal mucosa (b) and (c)).
Figure 4. Photomicrographs of serotonin-immunoreactive (IR) cells in the intestine of Tropidurus torquatus. (A–C) Small intestine. A, Highlighting the cytoplasmatic process of serotonin-IR cells of the open type (1040×). B, The presence of serotonin-IR cells at the base of the epithelium, some of the open type (arrow) and others of the closed type, can be observed (1000×). C, Serotonin-IR cells between the cells of the intestinal epithelium, all of the closed type (arrow; 870×). (D and E) Large intestine. D, Serotonin-IR cells at the base of the gland, all of the closed type (arrow; 870×). E, Closed-type cells at the base of the gland and epithelium (arrows) and open-type cells (arrowhead) between the cells of the intestinal epithelium (1040x×.
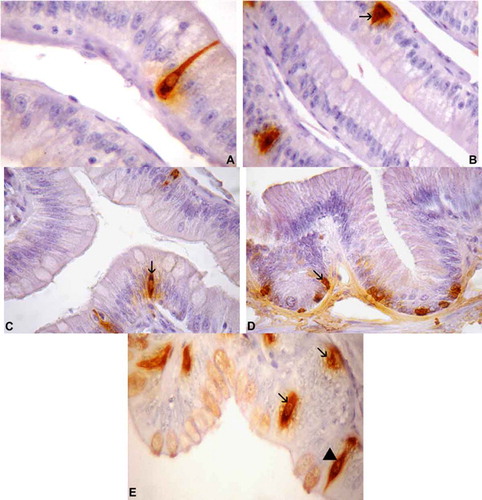
Pancreas
The pancreas was divided into exocrine and islet regions. The distribution patterns and relative frequencies of the endocrine cells found in the pancreas of Tropidurus torquatus are shown in .
Somatostatin-IR cells were the second most predominant type in the pancreas of T. torquatus (); they were found between the exocrine acinar cells – as solitary cells – and, occasionally, in the pancreatic duct ()). These IR cells were found in the peripheral parts of the islet regions and presented predominantly irregular shape (open-type cell) in the islet regions (b)), and elongated or oval shape (closed-type cells) in the parenchyma and in the exocrine duct walls (c)).
Figure 5. (A–C). Photomicrographs of somatostatin-immunoreactive (IR) cells in the pancreas of Tropidurus torquatus. A, The presence of somatostatin-IR cells between exocrine acinar cells can be observed (arrow; 870×). B, Note the islet-like regions; spindle-shaped somatostatin-IR cells were found in the peripheral portion (arrow; 870×). C, View of spherical somatostatin-IR cells in the parenchyma and on the exocrine ducts (arrow; 1040×).
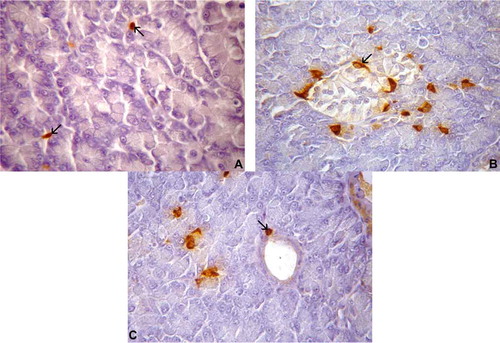
Glucagon-IR cells were the most predominant type in the pancreas of T. toquatus (); they were located in the exocrine parenchyma, as single cells, or in two- to three-cell clusters between the exocrine acinar cells. All these IR cells were oval-shaped (closed-type cells) () in the islet regions (a)).
Figure 6. (A–B). Photomicrographs of glucagon-immunoreactive (IR) cells in the pancreas of Tropidurus torquatus. A, The glucagon-IR cells at the islet-like regions can be observed (arrow; 230×). B, Note the presence of glucagon-IR cells at the exocrine parenchyma (arrow) closed-type cells (500×).
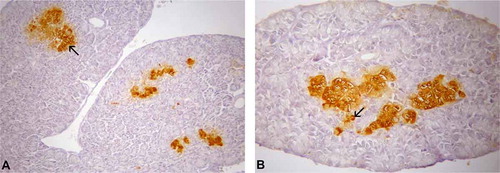
Insulin-IR cells were the least abundant type in the pancreas of T. torquatus (); they were found throughout the exocrine parenchyma, between the exocrine acinar cells – as solitary cells, or in three- to five-cell clusters (). These IR cells were located in the cell-cords (b)), in the islet-like regions; they were oval-shaped (closed-type cells) in the islet regions, and presented irregular shape (open-type cell) in the pancreatic parenchyma (c)).
Figure 7. (A–C). Photomicrographs of insulin cells in the pancreas of Tropidurus torquatus. A, Insulin-immunoreactive (IR) cells are closed-type cells islet-like regions (arrowhead) and open-type cells at the exocrine parenchyma (arrow; 870×). B. View of insulin-IR cells in the islet-like regions (arrow; 500×). C, Insulin-IR cells in the pancreatic parenchyma (arrow; 740×).
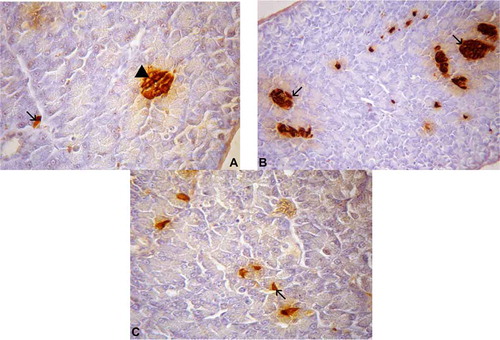
Discussion
Intestine
Gastrin-IR, CCK-IR, serotonin-IR, somatostatin-IR, glucagon-IR and insulin-IR cells are six important endocrine cell types found in vertebrates’ digestive tract. The regional distributions and relative frequencies of the endocrine cells found in the alimentary tracts can vary remarkably with animal species and feeding habits (Solcia et al. Citation1989; D’Este et al. Citation1994). The present study aimed at investigating the density of endocrine cells distributed in the enteropancreatic tract of Tropidurus torquatus.
The endocrine cells were divided into two types: round to spherical closed-type cells mainly located in the mucosal regions, and spherical to spindle-shaped open-type cells found in the epithelium (Ku et al. Citation2006). The closed-type cells assessed in the present study were mainly located in mucosal regions, whereas most of the open-type cells were found in the epithelial regions of T. torquatus.
Apparently, gastrin and CCK-8 come from the same ancestor, and a large fraction of these cells are seen in the human duodenum. They react with non-C terminal CCK antibodies and C-terminal gastrin/CCK antibodies, show immunoreactivity with C-terminal gastrin-34 antibodies and are co-localized with CCK in a varying portion of secretory granules (Solcia et al. Citation1989). Gastrin, which is secreted by G cells of the gastrointestinal tract, is the main physiological gastric acid secretion regulator (Solcia et al. Citation1987). It can also promote mucosal epithelial cell proliferation (Hernández et al. Citation2012), whereas the cholecystokinin secreted by endocrine intestinal mucosa cells (I cells) stimulates pancreatic enzyme secretion (Liddle Citation2000).
Gastrin-IR cells were restricted to the small intestine of T. torquatus in the present study, and are prevalent in the small intestine of reptile species such as the chelonians Chrysemys picta (Gapp et al. Citation1985), Testudo gracea, Mauremys caspica and Lacerta lepida (Perez-Tomas et al. Citation1989); the crocodilian Caiman latirostris (Yamada et al. Citation1987); and the lizards E. kingii (Arena et al. Citation1990), Podarcis hispanica (Burrell et al. Citation1992), T. wolteri (Lee & Ku Citation2004), G. japonicus, E. chinensis, S. indicus and E. elegans (Huang & Wu Citation2005). However, the gastrin-IR cells were found in the large intestine of some reptilian species such as the Testudines, including Mauremys caspica (Tarakçi et al. Citation2005) and Trachemys scripta elegans (Zhang & Wu Citation2009).
Cholecystokinin-8-IR cells were only observed in the small intestine of T. torquatus, similarly to reptilian species such as the crocodilian C. latirostris (Yamada et al. Citation1987), and the lizards P. hispanica (Burrell et al. Citation1992) and T. wolteri (Lee & Ku Citation2004). Some of the open-type cholecystokinin-8-IR cells in the intestine of T. torquatus present cytoplasmic processes that reach the adjacent cells possibly involved in paracrine secretion, as previously described by Chandra et al. (Citation2010). However, most of the closed-type cholecystokinin-8-IR cells were found in the small intestine.
Serotonin is a neurotransmitter synthesized by the serotonergic neurons of the central nervous system and by the endocrine cells in the GEP system (Rodrigues et al. Citation2005). This hormone has a strong effect on the regulation of digestive functions (Grundy Citation2008). The 5-HT could stimulate gastrointestinal mucosa secretion, smooth muscle contraction and the expansion of blood vessels, thus accelerating digestive tract movements (Wang et al. Citation2007). El-Salhy et al. (Citation1985) reported that these IR cells are found in the digestive tract of all vertebrate species, a fact that suggests their establishment in this region at an early phase of vertebrate evolution.
Serotonin-IR cells were found in the intestine of T. torquatus and were the most predominant endocrine cells there. The same result was observed in different reptilian species, including in the crocodilians C. latirostris (Yamada et al. Citation1987) and Alligator sinensis (Wu et al. Citation1999); the chelonians M. caspica, T. gracea, L. lepida (Perez-Tomas et al. Citation1989; see also Tarakçi et al. Citation2005 for M. caspica) and Ocadia sinensis (Liu et al. Citation2007); and the squamates E. kingii (Arena et al. Citation1990), C. chalcides, Z. madagascariensis (Morescalchi et al. Citation1997), T. wolteri (Lee & Ku Citation2004), G. japonicus, E. chinensis, S. indicus, E. elegans (Huang & Wu Citation2005), and T. scripta (Zhang & Wu Citation2009).
Most serotonin-IR cells presenting cytoplasmic processes reaching the intestinal lumen (open type) were observed in the intestine of T. torquatus, and this suggests that serotonin may be secreted through the exocrine pathway to the intestinal lumen. However, some of the closed types of these IR cells, reaching the adjacent cells, were also observed in the basal portion of the glands, thus indicating that these cells may secrete this hormone through the paracrine pathways. These different cell-signaling modes were previously described by Huang and Wu (Citation2005) in the intestines of four squamates, namely G. japonicus, E. chinensis, S. indicus and E. elegans.
The somatostatin-, glucagon- and insulin-IR cells were not observed in any of the T. torquatus intestine regions. Lee et al. (Citation1995) also reported the absence of somatostatin-IR cells in the GIT of the amur lizard. Glucagon-IR cells were not detected in the alimentary tract of the King’s skink species (Arena et al. Citation1990) or in the small intestine of the snakes (Masini Citation1986). However, these three IR cell types were found in the intestine of other lizard species, including L. lepida (Perez-Tomas et al. Citation1989), Chalcides chalcides, Zoonosaurus madagascariensis (Morescalchi et al. Citation1997) and T. wolteri (Lee & Ku Citation2004). The aforementioned studies suggest that the regional distribution of these cells changes among reptilians, and the present results seem to corroborate this pattern.
Pancreas
Somatostatin is secreted not only by the D cells of the pancreatic islets, but also by the hypothalamus and by certain intestinal cells; it also inhibits the secretion of other neuroendocrine hormones (Hsu & Crump Citation1989). The somatostatin cells scattered in the exocrine parenchyma, as well as the long protrusions exhibited by these cells, suggest paracrine functions performed by the zymogen cells. There are reports on somatostatin inhibiting the exocrine secretion by the pancreas (Raptis et al. Citation1978).
Somatostatin-IR cells were located in the exocrine pancreas and pancreatic duct, and around the periphery of cell-cords in the islet-like regions in the pancreas of T. torquatus. These IR cells were also located in the pancreas of different reptilian species such as A. carolinensis (Rhoten & Hall Citation1981), M. quinquetaeniata, U. aegyptia (El-Salhy & Grimelius Citation1981), C. ocellatus (El-Salhy et al. Citation1983), C. chalcides and Z. madagascariensis (Morescalchi et al. Citation1997); and in the Testudines C. picta (Gapp et al. Citation1985) and M. caspica (Ayala et al. Citation1989).
The glucagon secreted by A cells in the pancreas increases the blood glucose levels (Foa et al. Citation1957), whereas the insulin secreted by the B cells of the pancreas regulates the serum glucose levels (Hsu & Crump Citation1989).
The glucagon-IR and insulin-IR cells were observed in the islet-like regions and randomly located throughout the pancreatic parenchyma of T. torquatus. This result is similar to that previously reported for different reptilian species, including the squamates A. carolinensis (Rhoten & Hall Citation1981), M. quinquetaeniata, U. aegyptia (El-Salhy & Grimelius Citation1981), C. chalcides, Z. madagascariensis (Morescalchi et al. Citation1997), T. wolteri (Ku & Lee Citation2004) and E. carinata (Chandavar & Naik Citation2011); and the Testudines M. caspica (Ayala et al. Citation1989), T. scripta (Ku et al. Citation2001) and Melanochelys trijuga (Chandavar & Naik Citation2008).
The glucagon-IR and insulin-IR cell arrangements in the islets and isolated cells represent an endocrine network that may regulate the exocrine function in a paracrine fashion (Putti et al. Citation1992). Thus, the study showed that the glucagon and insulin cells do not necessarily need to form islets in all lizard species. Epple and Brinn (Citation1987) concluded that the islets were scattered throughout the pancreas in turtles, crocodiles and in certain Squamata; actually, they are either individual or formed in smaller groups comprising two to four cells within the exocrine pancreas.
The density of endocrine cell distribution is related to the species’ feeding habits, food components and living environment. The morphologies of endocrine cells are in compliance with the endocrine and exocrine functions (Zhitao et al. Citation2011). Therefore, the different endocrine cell types identified in the enteropancreatic system of T. torquatus are similar to those found in other reptiles and other vertebrate species, which is a characteristic of vertebrate phylogeny.
Acknowledgements
The authors thank I. L. C. Meirelles for her technical assistance, the Rio de Janeiro State Research Foundation (FAPERJ) for financial support and UFRRJ for the exchange of Program Internal Exchange Scientific Initiation – PROIC granted to the first author. The research was approved by the Ethics Research Committee of the Federal Rural University of Rio de Janeiro, Seropédica, Brazil (process number 23083.012480/2010-64). The authors thank H. R. Silva for help in the animals’ collection. License number for wild animal capture: SISBIO 506961. No conflict of interest is reported.
Additional information
Funding
References
- Arena PC, Richardson KC, Yamada J. 1990. An immunohistochemical study of endocrine cells of the alimentary tract of the King’s skink (Egernia kingii). Journal of Anatomy 170:73–85.
- Ayala AG, Lozano MT, Agulleiro B. 1989. Comparative study on the endocrine cells in the pancreas of Mauremys caspica (Chelonia) in summer and winter. General and Comparative Endocrinology 75:363–375. DOI:10.1016/0016-6480(89)90171-8.
- Burrell AC, Villaro P, Sesma G, Rindi DV. 1992. Evidence for the colocalization of Gas/CCK- and PYY/PP-immunoreactive substances in the small intestine of the lizard Podarcis hispanica: Immunocytochemical and ultrastructural study. General and Comparative Endocrinology 88:40–49. DOI:10.1016/0016-6480(92)90192-M.
- Carvalheira AF, Welsch U, Pearse AG. 1968. Cytochemical and ultrastructural observations on the argentaffin and argyrophil cells of the gastro-intestinal tract in mammals, and their place in the APUD series of polypeptide-secreting cells. Histochemie 14:33–46. DOI:10.1007/BF00268031.
- Carvalho ALG. 2013. On the distribution and conservation of the South American lizard genus Tropidurus Wied-Neuwied, 1825 (Squamata: Tropiduridae). Zootaxa 3640:042–056. DOI:10.11646/zootaxa.3640.1.3.
- Chandavar VR, Naik PR. 2008. Immunocytochemical detection of glucagon and insulin cells in endocrine pancreas and cyclic disparity of plasma glucose in the turtle Melanochelys trijuga. Journal of Biosciences 33:239–247. DOI:10.1007/s12038-008-0041-8.
- Chandavar VR, Naik PR. 2011. Immunocytochemical localization of glucagon and insulin cells in Eutropis carinata with respect to reproductive cycle. Asian Herpetological Research 2:103–109. DOI:10.3724/SP.J.1245.2011.00103.
- Chandra R, Samsa L, Vigna S, Liddle R. 2010. Pseudopod-like basal cell processes in intestinal cholecystokinin cells. Cell and Tissue Research 341:289–297. DOI:10.1007/s00441-010-0997-1.
- D’Este L, Buffa R, Pelagi M, Siccardi AG, Renda T. 1994. Immunohistochemical localization of chromogranin A and B in the endocrine cells of the alimentary tract of the green frog, Rana esculenta. Cell & Tissue Research 277:341–349. DOI:10.1007/BF00327782.
- Deveney CW, Way LW. 1983. Regulatory peptides of gut. In: Greenspan FS, Forsham PH, editors. Basic and clinical endocrinollogy. Asian ed. Singapore: Maruzen. pp. 479–499.
- El-Salhy M, Abu-Sinna G, Wilander E. 1983. The endocrine pancreas of a squamate reptile, the desert lizard (Chalcides ocellatus). A histological and immunohistochemical investigation. Histochemistry 78:391–397. DOI:10.1007/BF00496625.
- El-Salhy M, Grimelius L. 1981. Histological and immunohistochemical studies of the endocrine pancreas of lizards. Histochemistry 72:237–247. DOI:10.1007/BF00517137.
- El-Salhy M, Winder E, Lundqvist M. 1985. Comparative studies of serotonin-like immunoreactive cells in the digestive tract of vertebrates. Biomedical Research 6:371–375.
- Epple A, Brinn JE. 1987. The comparative physiology of the pancreatic islets. Berlin: Springer.
- Foa PP, Galansino G, Pozza G. 1957. Glucagon, a second pancreatic hormone. Recent Progress in Hormone Research 13:473–503.
- Frost DR, Rodrigues MT, Grant T, Titus TA. 2001. Phylogenetics of the lizard Genus Tropidurus (Squamata: Tropiduridae: Tropidurinae): Direct optimization, descriptive efficiency, and sensitivity analysis of congruence between molecular data and morphology. Molecular Phylogenetics and Evolution 21:352–371. DOI:10.1006/mpev.2001.1015.
- Fry BG, Vidal N, Norman JA, Vonk FJ, Scheib H, Ramjan RSF, Kuruppu S, Fung K, Hedges SB, Richardson MK, Hodgson WC, Ignjatovic V, Summerhayes R, Kochva E. 2006. Early evolution of the venom system in lizards and snakes. Nature 439:584–588. DOI:10.1038/nature04328.
- Fujita T. 1990. Gut hormone secreting cells- unsolved problems. Human Cell 3:27–30.
- Gapp DA, Kenny MP, Polak JM. 1985. The gastro-entero-pancreatic system of the turtle, Chrysemys picta. Peptides 6:347–352. DOI:10.1016/0196-9781(85)90396-1.
- Grundy D. 2008. 5-HT system in the gut: Roles in the regulation of visceral sensitivity and motor functions. European Review for Medical and Pharmacological Sciences 12:63–67.
- Hao W, Shulan L, Zhitao L, Peng L, Wenge Z. 2012. Immunohistochemical study of the four kinds of endocrine eells in the digestive tract of Lacerta vivipara. Chinese Agricultural Science Bulletin 28:56–60.
- Hernández DR, Vigliano FA, Sánchez S, Bermúdez R, Domitrovic HA, Quiroga MI. 2012. Neuroendocrine system of the digestive tract in Rhamdia quelen juvenile: An immunohistochemical study. Tissue & Cell 44:220–226. DOI:10.1016/j.tice.2012.03.005.
- Hsu WH, Crump MH. 1989. The endocrine pancreas. In: McDonald LE, Pineda MH, editors. Veterinary endocrinology and reproduction. Philadelphia: Lea & Febiger. pp. 186–201.
- Huang XG, Wu XB. 2005. Immunohistochemical study on gastrointestinal endocrine cells of four reptiles. World Journal of Gastroenterology 11:5498–5505. DOI:10.3748/wjg.v11.i35.5498.
- Ku S-K, Lee H-S. 2004. The distribution and frequency of endocrine cells in the splenic lobe of grass lizard (Takydromus wolteri): An immunohistochemical study. European Journal of Histochemistry 48:429–436. DOI:10.4081/917.
- Ku SK, Lee HS, Lee JH. 2006. The regional distribution and relative frequency of gastrointestinal endocrine cells in the nude mice, Balb/c-nu/nu: An immunohistochemical study. Anatomia, Histologia, Embryologia: Journal of Veterinary Medicine Series C 35:104–110. DOI:10.1111/ahe.2006.35.issue-2.
- Ku S-K, Lee H-S, Lee J-H, Park K-D. 2001. Immunohistochemistry of the pancreatic endocrine cells of the red-eared slider (Trachemys scripta elegans). Korean Journal of Biological Sciences 4:187–193. DOI:10.1080/12265071.2000.9647544.
- Larsson L-I. 2000. Developmental biology of gastrin and somatostatin cells in the antropyloric mucosa of the stomach. Microscopy Research and Technique 48:272–281. DOI:10.1002/(ISSN)1097-0029.
- Lee HS, Ku SK. 2004. An immunohistochemical study of endocrine cells in the alimentary tract of the grass lizard, Takydromus wolteri Fischer (Laceridae). Acta Histochemica 106:171–178. DOI:10.1016/j.acthis.2003.10.008.
- Lee MS, Lee JH, Lee HS. 1995. An histological and immunohistochemical study of the endocrine cells in the gastrointestinal tract of the Amur lizard (Takydromus amurensis). Korean Journal of Veterinary Research 35:67–73.
- Liddle RA. 2000. Regulation of cholecystokinin secretion in humans. Journal of Gastroenterology 35:181–187. DOI:10.1007/s005350050328.
- Liu C, Zhen L, Li S, Zhao WG. 2007. Distribution and morphological observation of 5-HT immunoreactive endocrine cells in digestive tract of Mauremys sinensis. Sichuan Journal of Zoology 26:22.
- Machado-Santos C, Peracchi AL, Mikalauskas JS, Rocha PA, Sales A. 2009. Distributions of the endocrine cells in the gastrointestinal tract of nectarivorous. Tissue Cell 41:222–229. DOI:10.1016/j.tice.2008.11.004.
- Masini MA. 1986. Immunohistochemical localization of gut peptides in the small intestine of snakes. Basic and Applied Histochemistry 30:317–324.
- Morescalchi AM, Gaccioli M, Faraldi G, Tagliafierro G. 1997. The gastroenteric-pancreatic neuroendocrine system in two reptilian species, Chalcides chalcides and Zoonosaurus madascariensis (Sauridae). European Journal of Histochemistry 41:29–40.
- Passos DC, Lima DC, Borges-Nojosa DM. 2011. A new species of Tropidurus (Squamata, Tropiduridae) of the semitaeniatus group from a semiarid area in Northeastern Brazil. Zootaxa 2930:60–68.
- Pereira JG, Silva SR, Gonçalves MTC, Melo FA, Viana DC, Oliveira AS, Machado CR. 2015. Immunolocalization of enteroglucagon in endocrine cells in the stomach of scorpion mud turtle Kinosternon scorpioides (Reptilia, Chelonia, Kinosternidae). Ciência Animal Brasileira 16:448–455. DOI:10.1590/1089-6891v16i330969.
- Perez-Tomas R, Ballesta J, Pastor LM, Madrid JF, Polak JM. 1989. Comparative immunohistochemical study of the gastroenteropancreatic endocrine system of three reptiles. General and Comparative Endocrinology 76:171–191. DOI:10.1016/0016-6480(89)90148-2.
- Pianka ER, Vitt LJ. 2003. Lizards. Windows to the evolution of diversity. Berkeley, Los Angeles & London: University of California Press.
- Pincheira-Donoso D, Bauer AM, Meiri S, Uetz P. 2013. Global taxonomic diversity of living reptiles. Plos ONE 8:e59741. DOI:10.1371/journal.pone.0059741.
- Pough FH, Andrews RM, Cadle JE, Crump ML, Savitzky AH, Wells KD. 2004. Herpetology. New Jersey: Pearson, Prentice Hall.
- Putti R, Rossa A, Varano L, Laforgia V, Cavagnuolo A. 1992. An immunocytochemical study of the endocrine pancreas in three genera of lacertids. General and Comparative Endocrinology 87:249–259. DOI:10.1016/0016-6480(92)90029-J.
- Raptis S, Schlegel W, Lehmann E, Dollinger HC, Zoupas CH. 1978. Effects of somatostatin on the exocrine pancreas and the release of duodenal hormones. Metabolism 27:1321–1328. DOI:10.1016/0026-0495(78)90066-5.
- Reid PE, Volz D, Cho KY, Owen DA. 1988. A new method for the histochemical demonstration of O-acyl sugars in human colonic epithelial glycoproteins. The Histochemical Journal 20:510–518. DOI:10.1007/BF01002649.
- Rhoten WB, Hall CE. 1981. Four hormones in the pancreas of the lizard, Anolis carolinensis. The Anatomical Record 199:89–97. DOI:10.1002/(ISSN)1097-0185.
- Rodrigues SS, Fonseca CC, Neves MTD. 2005. Células endócrinas do sistema gastroenteropancreático: Conceitos, distribuição, secreções, ação e controle. Arquivos de Ciências Veterinárias e Zoologia da Unipar 8:171–180.
- Santos CM, Nascimento AA, Peracchi AL, Dias D, Ribeiro TP, Sales A. 2008b. A comparative immunohistochemical study of endocrine cells in the digestive tract of two frugivorous bats: Artibeus cinerius and Sturnira lilium. Acta Histochemica 110:134–142. DOI:10.1016/j.acthis.2007.10.002.
- Santos CM, Nascimento AA, Peracchi AL, Sales A, Mikalauskas JS, Gouveia SF. 2008a. Immunocytochemical study of gastrintestinal endocrine cells in insectivorous bats (Mammalia: Chiroptera). Brazilian Journal of Biology 68:663–669. DOI:10.1590/S1519-69842008000300026.
- Secor SM, Diamond J. 1998. A vertebrate model of extreme physiological regulation. Nature 395:659–662. DOI:10.1038/27131.
- Siqueira CC, Kiefer MC, Van Sluys M, Rocha CFD. 2013. Variation in the diet of the lizard Tropidurus torquatus along its coastal range in Brazil. Biota Neotropica 13:93–101. DOI:10.1590/S1676-06032013000300012.
- Solcia E, Capella C, Buffa R, Usellini L, Fiocca R, Sessa F. 1987. Endocrine cells of the digestive system. In: Johnson LR, editor. Physiology of the gastrointestinal tract. New York: Raven Press. pp. 111–130.
- Solcia E, Usellini L, Buffa R, Rindi G, Villani L, Aguzzi A, Silini E. 1989. Endocrine cells producing regulatory peptides. In: Polak JM, editor. Regulatory peptides. Basel: Birkhäuser Verlag. pp. 220–246.
- Tarakçi BG, Köprücü SS, Yaman M. 2005. An Immunohistochemical study on the endocrine cells in the gastrointestinal tract of the freshwater turtle, Mauremys caspica caspica. Turkish Journal of Veterinary and Animal Sciences 29:581–587.
- Teixeira RL, Giovanelli M. 1999. Ecologia de Tropidurus torquatus (Sauria: Tropiduridae) da restinga de Guriri, São Mateus, ES. Revista Brasileira de Biologia (Brazil) 59:11–18. DOI:10.1590/S0034-71081999000100002.
- Vitt LJ, Caldwell JP. 2014. Herpetology: an introductory biology of amphibians and reptiles. 4rd ed. Burlington: Elsevier.
- Wang SH, Dong L, Luo JY, Gong J, Li L, Lu XL, Han SP. 2007. Decreased expression of serotonin in the jejunum and increased numbers of mast cells in the terminal ileum in patients with irritable bowel syndrome. World Journal of Gastroenterology 13:6041–6047. DOI:10.3748/wjg.13.6041.
- Wu XB, Zhang SZ, Chen BH, Deng ZP, Zhou Z, Wang CL, Nie JS, Xie WS. 1999. Immunohistochemical studies on endocrine cells in the digestive tract of Alligator sinensis. Dongwu Xuebao 45:155–161.
- Yamada J, Campos VI, Kitamura N, Pacheco AC, Yamashita T, Yanaihara N. 1987. An immunohistochemical study of the endocrine cells in the gastrointestinal mucosa of the Caiman latirostris. Archives of Histology and Cytology 50:229–241. DOI:10.1679/aohc.50.229.
- Zhang ZG, Wu XB. 2009. Immunohistochemical study on endocrine cells in the digestive tract of Trachemys scripta elegans. Chinese Journal of Histochemistry and Cytochemistry 03:14.
- Zhitao L, Shulan L, Xin G, Peng G, Wenge Z, Yuguo X. 2011. The research of somatostatin immunoreactve endocrine cells in digestive tract of Lacerta vivipara. Chinese Agricultural Science Bulletin 27:225–228.