Abstract
In the last few decades, findings of freshwater limpets belonging to the genus Ferrissia have been frequent and widely distributed throughout the Palaearctic and beyond. The widespread presence of a Nearctic alien taxon was proved, but no consensus was achieved about the possible existence of autochthonous Ferrissia taxa in the area, an occurrence which would be supported by the presence of gastropod fossils attributed to the genus throughout Eurasia and North Africa. In order to test the hypothesis of a possible persistence of autochthonous Ferrissia taxa in the Palaearctic to the present day, all the published data on the genetic diversity of Ferrissia populations occurring in the area were reviewed, expanding also the currently available dataset through dedicated sampling surveys in Italy, Malta and Spain. Here, based on currently published and novel large ribosomal subunit 16S rRNA (16S) and cytochrome oxidase sub-unit 1 (COI) sequences, the presence of the allochthonous Ferrissia californica in the whole Palaearctic was confirmed. Conversely, no evidence supporting the presence of autochthonous Ferrissia taxa was obtained. Ferrissia californica proved to be a highly invasive taxon (in spite of its extremely low genetic diversity) throughout the invaded regions, which is possibly related to the species’ ability of asexual reproduction. Finally, the need to investigate the possible impact exerted by F. californica on the autochthonous Palaearctic biota is briefly stressed.
Introduction
Fossil records of the planorbid genus Ferrissia Walker, 1903 are known to occur throughout the Palaearctic region, with the genus being recorded from the Middle Miocene of Hungary, Austria, southern Germany and Switzerland (Schlickum Citation1976; Kókay Citation2006; Harzhauser et al. Citation2012, Citation2014b); from Pliocene deposits of France (Wautier Citation1974); and from the Early and Middle Pleistocene of Israel (Mienis & Ashkenazi Citation2011; Mienis & Rittner Citation2013). In addition, there is doubtful fossil evidence of the presence of Ferrissia sp. from interglacial Pleistocene and Holocene deposits in the Netherlands (Meijer Citation1987; Van Der Velde Citation1991) and Egypt (Bernasconi & Stanley Citation1994). Oddly, no evidence of the presence of extant Ferrissia limpets was collected in the Old World until the 20th century, when a few occurrences of representatives of this genus were found in France (Roger & Calas Citation1944), in Germany (Boettger Citation1949) and in Russia (reported by Son Citation2007). Afterwards, several findings occurred, and the reported taxa were identified mostly under the binomia Ferrissia wautieri (Mirolli, 1960) in Europe and F. clessiniana (Jickeli, 1882) in Africa and the Middle East. In fact, no consensus was reached on the taxonomic identity of Palaearctic Ferrissia until molecular evidence (e.g. Walther et al. Citation2006b,Citationc; Marrone et al. Citation2011b) proved that all the studied Ferrissia populations occurring in the Palaearctic region belonged to a single allochthonous taxon of Nearctic origin, initially reported as F. fragilis (Tryon, 1863) and later as F. californica (Rowell, 1863), in accordance with the Principle of Priority of the International Code of Zoological Nomenclature (Christensen Citation2016).
From the beginning of the 21st century, an ever-increasing body of evidence (Walther et al. Citation2006a, Citationb and Citationc, Citation2010; Beran & Horsak Citation2007; Son Citation2007; Semenchenko & Laenko Citation2008; Spyra Citation2008; Cilia Citation2009; Raposeiro et al. Citation2011; Marrone et al. Citation2011b, Citation2014; Tokinova Citation2012; Albrecht et al. Citation2013) has proved that the invasive North American Ferrissia californica widely invaded Europe, Asia and South America, while a molecular identification of the taxa occurring in Oceania (Greke Citation2011) and Africa (Brown Citation1965; Khalloufi & Boumaiza Citation2007) is, to date, lacking. However, based on the presence of Quaternary fossils of Ferrissia in the Palaearctic, the persistence of pre-Quaternary autochthonous Ferrissia populations in the region cannot be ruled out.
The present study aims to investigate the possible presence of autochthonous Ferrissia taxa currently living in the Palaearctic area, through the aggregate analysis of all the Palaearctic Ferrissia mtDNA sequences currently available in public repositories, and adding new data collected in the Central Mediterranean area.
Materials and methods
Ferrissia specimens (one to four from each sampled site) were collected during field surveys between 2008 and 2012 on the Italian mainland (including Lake Maggiore, the type locality of Ferrissia wautieri), Sardinia, Sicily, Malta and Spain (Table S1); the locations of the newly studied populations and of those for which published sequences are available were mapped using the QGIS software v. 2.18.2 (Citation2016) (http://www.qgis.org) and are reported in .
Water bodies were sampled with a 200-μm-mesh-sized hand net swept through the littoral vegetation and on the bottom of the studied water bodies. Collected samples were fixed in situ in 80% ethanol, and sorted under a stereomicroscope. The specimens, identified as Ferrissia sp. according to Girod et al. (Citation1980), were then dipped in double-distilled water for 1 hour, and processed for DNA extraction using the Qiagen “DNeasy Animal Tissue Kit” following the manufacturer’s instructions. Some specimens were deposited in the collection of the Museum of Natural Science of the University of Florence (Zoology Section “La Specola”, Italy; see Table S1 for the catalogue numbers); others are stored in the molluscan collection of Federico Marrone at the University of Palermo (Italy) and are available for loan on request.
A partial sequence of the cytochrome oxidase subunit 1 (COI) gene was amplified using the universal primer pair LCO1490 and HCO2198 (Folmer et al. Citation1994). A portion of the large ribosomal subunit 16S rRNA gene was amplified using the primer pair “Skisto-1” and “Skisto-2” (see Marrone et al. Citation2011a). Composition of the polymerase chain reaction (PCR) mix and thermal cycles were performed according to Marrone et al. (Citation2013).
After PCR amplification, 5 μL of each PCR product were separated by electrophoresis on a 2% agarose gel at 70 V for 1 h and visualised with a UV Transilluminator. When PCR products showed a clear and single band of the correct expected length, the whole PCR product was purified using the Exo-SAP purification kit and single-strand sequenced by Macrogen Inc. (Seoul, South Korea) with an ABI 3130xL (Applied Biosystems) sequencer with the same primers used for PCR amplification. Sequences were analysed and manually proofread with the DNA sequencing software Chromas v. 2.6.2 (Technelysium, Pty. Ltd. 1998, Queensland, Australia), and aligned with ClustalX v. 2.1 (Larkin et al. Citation2007).
In addition, in order to compare the new sequences obtained with those available for other countries, all of the 65 available COI and 17 16S rRNA Ferrissia spp. sequences from the Palaearctic and the Neotropical regions were downloaded from GenBank. Moreover, all the available F. californica (= F. fragilis) sequences from the Nearctic were downloaded for comparison, and eight Ferrissia rivularis (Say, 1917) and two Pettancylus sp. sequences were downloaded to be included as outgroups (see Albrecht et al. Citation2006; Walther et al. Citation2010; further information on the downloaded sequences is given in Table S1). The poor quality of the available COI sequences of Ferrissia from Denmark (Accession Number [AN] AY577502, erroneously labelled as Acroloxus lacustris (Linnaeus, 1758)) and COI and 16S rRNA sequences of Ferrissia clessiniana from Uganda (AN AY577509 and AY577469) did not allow us to include them in the analyses.
Analyses were conducted in two steps. Firstly, the molecular identification of the Palaearctic Ferrissia populations was performed through the reconstruction of phylogenetic trees based on all the available sequences for each mtDNA gene fragment, respectively. Afterwards, the COI genetic diversity observed in the Palaearctic and Neotropical biogeographical regions, where the single observed species (i.e. Ferrissia californica) proved to be allochthonous, were compared with that observed in the Nearctic, where F. californica finds its native range.
The genetic identification of the studied specimens and the reconstruction of the phylogenetic relationships among the taxa were performed with Bayesian inference (BI) and maximum likelihood (ML) methods as implemented in MrBayes v. 3.2.6 (Ronquist et al. Citation2012) and PhyMl v. 3 (Guindon & Gascuel Citation2003), respectively. As a measure of branch support, bootstrap values (Felsenstein Citation1985) were calculated with 1000 replicates in the ML trees. For both markers, two independent Markov Chain Monte Carlo analyses were run with 1 million generations (temp.: 0.2; default priors). Trees and parameter values were sampled every 100 generations resulting in 10,000 saved trees per analysis; in both analyses convergence was reached (Effective Sample Size (ESS) above 937.52 and 1161.86, respectively, for COI and 16S rRNA); 2500 trees were conservatively discarded as “burn-in”. Nodes’ statistical support of BI was evaluated by their posterior probabilities. The choice of the best evolutionary model was made using PartitionFinder v. 1.0.1 (Lanfear et al. Citation2012) according to the Akaike information criterion (AIC; Akaike Citation1974). For both the COI and 16S rRNA datasets, BI and ML analyses were performed using a Hasegawa–Kishino–Yano (HKY) model of sequence evolution for molecular data.
A haplotype network for all the available COI sequences was constructed with the software Popart (v. 1.7, http://popart.otago.ac.nz), using the median-joining method (Bandelt et al. Citation1999); conversely, due to the low haplotype diversity observed for 16S rRNA, no network was built based on this marker.
Based on the output of the phylogenetic analyses, the sampled populations were partitioned into two groups: (i) autochthonous populations (USA; 36 COI and three 16S rRNA sequences); (ii) allochthonous populations (Asia, Europe and South America; 40 COI and 23 16S rRNA sequences).
Analysis of molecular variance (AMOVA) (Excoffier et al. Citation1992), mismatch analysis, haplotype diversity (Hd) and nucleotide diversity (π) were computed in order to detect possible population differentiation with DnaSP v. 5 (Librado & Rozas Citation2009). Furthermore, in order to estimate the significance of the pairwise differences in haplotype diversity between the two groups, the functions described by Thomas et al. (Citation2002) and available at http://www.ucl.ac.uk/mace-lab/resources/software (“TEST_h_DIFF”) were used through the statistical software “R” (v. 3.2.1, R Core Team Citation2015, available at https://www.R-project.org).
Results
Excluding the outgroups, 76 COI sequences (percentage of variable and parsimony-informative sites: 6.22% and 4.84%, respectively) and 26 16S rRNA sequences (percentage of variable and parsimony-informative sites, 0.63% and 0%, respectively) were analysed overall.
Both phylogenetic methods (ML and BI) showed the presence of a well-supported clade (1.00 posterior probability, pp, and 84% bootstrap support, bs, in the Bayesian and ML analyses for the COI marker, and 1.00 pp and 85% bs for the 16S rRNA marker; see and ), which includes all the autochthonous North American Ferrissia californica populations along with all the Ferrissia sp. specimens to date investigated from the Palaearctic and Neotropical regions ( and ). Conversely, the North America F. rivularis clade is separated from the F. californica one by deep genetic distances and well-supported nodes, in accordance with its status of bona species (Walther et al. Citation2010).
Figure 2. Bayesian phylogram (95% majority rule consensus tree) of the focal Ferrissia spp. using the mitochondrial cytochrome oxidase 1 (COI) dataset. Ferrissia rivularis and Pettancylus sp. were used as outgroups. Node statistical support is reported as nodal posterior probabilities (Bayesian Inference of phylogeny, BI) and bootstrap values (Maximum Likelihood, ML). Asterisks indicate a bootstrap support value lower than 50. •: Novel sequences.
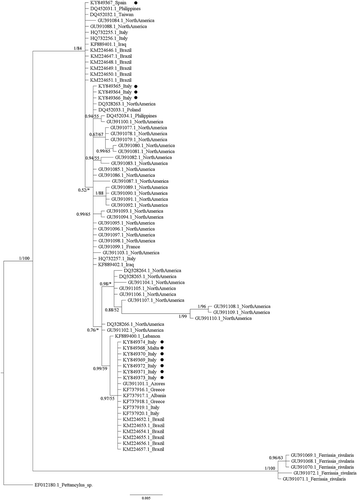
Figure 3. Bayesian phylogram (95% majority rule consensus tree) of the focal Ferrissia spp. using the mitochondrial 16S ribosomal subunit (16S rRNA) dataset. Ferrissia rivularis and Pettancylus sp. were used as outgroups. Node statistical support is reported as nodal posterior probabilities (Bayesian Inference of phylogeny, BI) and bootstrap values (Maximum Likelihood, ML). Asterisks indicate a bootstrap support value lower than 50. •: Novel sequences.
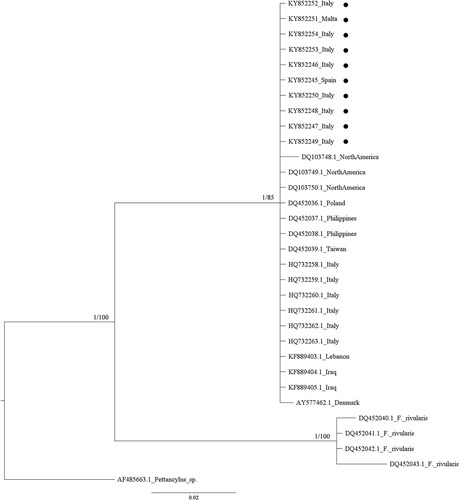
Overall, 21 COI haplotypes were obtained (Table S1). The haplotype network () indicates the existence of three main and widespread haplotypes, and some rarer haplotypes shared by one or a few individuals mostly restricted to single continents. Most of the haplotypes belong to, or include at least one of, the North American samples excepting the haplotypes H11 and H12, which were observed solely in “South America and Europe” and in “Asia”, respectively.
Figure 4. Median-joining haplotype network based on a fragment of mtDNA cytochrome oxidase sub-unit 1 (COI). Dashes indicate substitution steps.
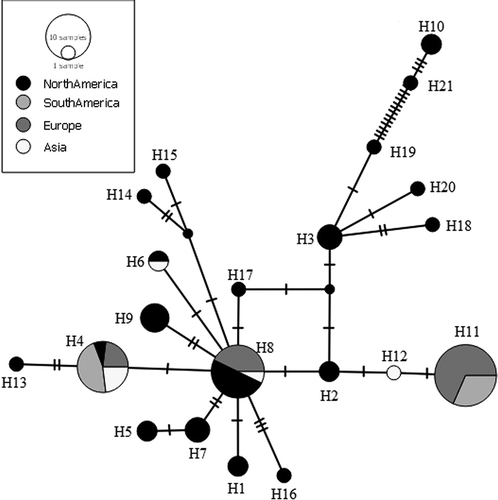
The comparison of the two groups showed that both nucleotide and haplotype diversity is higher in the “autochthonous” group than in the “allochthonous” one, and this applies for both markers (). AMOVA test results () show that the percentage of variation among the two geographical groups is 17.37%, and within the groups it is 82.63%, with an Fixation index (FST) value of 0.173 and a p value of 0.000. The mismatch analysis showed an observed number of pair-wise differences which differs significantly from the expected values (Sum of Square Deviations (SSD) p value = 0.011; raggedness index = 0.028; raggedness p value = 0.039, ).
Table I. Nucleotide diversity (Pi) and haplotype diversity (Hd) of the “autochthonous” and “allochthonous” Ferrissia groups based on the mitochondrial cytochrome oxidase 1 (COI) and 16S ribosomal subunit (16S rRNA) gene fragments.
Table II. AMOVA FST results based on 578-bp-long fragments of the mtDNA COI gene. “Va” is the variance among groups, “Vb” is the variance among populations within groups.
Discussion
In spite of the fossil evidence indicating the presence of autochthonous Ferrissia species in the Palaearctic until the late Pleistocene or early Holocene, the tree topologies obtained using fragments of both COI and 16S rRNA mtDNA markers strongly support the molecular homogeneity of all the current Eurasian (and South American) Ferrissia populations, and their conspecificity with F. californica from North America.
The AMOVA results and the topology of the haplotype network show the presence of a substantially higher diversity within the group of autochthonous (i.e. Nearctic) populations than in the group of the allochthonous (i.e. Palaearctic and Neotropical) ones. Moreover, the haplotypes found in North America are also present in South America and Eurasia, which host an impoverished subset of the molecular diversity scored in the Nearctic. The only exceptions are the H11 and H12 haplotypes, occurring in Europe, Asia and South America, which are, to date, absent from the Nearctic area. However, the lack of these haplotypes in North America is likely to be ascribed to subsampling of Ferrissia californica in its native range (e.g. along the west coast of the USA, cf. Walther et al. Citation2010), and it is likely that the H11 and H12 haplotypes could be found there when a higher sampling effort is performed. This evidence supports the allochthnous status of Ferrissia californica in Eurasia, and its North American origin. Moreover, the mismatch analysis suggests that the entire population of Ferrissia californica is probably under recent demographic expansion.
The occurrence of Ferrissia californica out of its native range may be ascribed to the unwitting anthropochorus dispersal of this species along with the aquarium trade, followed by its release by the aquarists through aquarium waste disposal – e.g. Duggan (Citation2010) reports the finding of F. californica (as F. fragilis) in the sediments of aquariums in New Zealand. It has been verified that the aquarium trade is responsible for the establishment of a large number of species into non-native areas (e.g. Mackie Citation1999; Rixon et al. Citation2005). In addition, there are several well-documented examples showing the global invasiveness of “aquarium-dispersed” gastropod species, such as Potamopyrgus antipodarum (Gray, 1843), Melanoides tuberculata (Müller, 1774) and Haitia acuta (Draparnaud, 1805) which invaded almost every freshwater ecosystem of the world in less than two centuries (e.g. Dillon et al. Citation2002; Taylor Citation2003; Bousset et al. Citation2004; Wethington & Lydeard Citation2007; Albrecht et al. Citation2009; Naser et al. Citation2011; Ladd & Rogowski Citation2012; Collado Citation2014; Marrone & Naselli-Flores Citation2015).
Despite the low genetic diversity observed in non-native areas, Ferrissia californica displays a noteworthy invasive capacity in markedly different areas with suitable habitats and climates, from the temporary ponds of the Mediterranean area through rivers and lakes of Northern Europe, to tropical environments in the Philippines and Brazil (e.g. Raposeiro et al. Citation2011; Tokinova Citation2012; Albrecht et al. Citation2013; Marrone et al. Citation2014; Lacerda et al. Citation2015; and references therein). This is in line with the so-called “genetic paradox of invasions” (Roman & Darling Citation2007; Caron et al. Citation2014; Schrieber & Lachmuth Citation2017), based on the disappointments of the expectation that a greater invasive capacity should be attributed to higher genetic diversity. In fact, rather than hinder invasion, bottlenecks that occur during man-mediated introduction events might increase the invasive capacity of the invader by depleting deleterious alleles so that even inbred individuals have a high fitness (see Estoup et al. Citation2016). Furthermore, those taxa which have a non-gonochoric reproduction mode (as in the case of Ferrissia californica) are often advantaged during the colonisation of new habitats because they have a higher potential for demographic growth (and subsequent monopolisation of resources) compared to those having a gonochoric reproduction (see Incagnone et al. Citation2015, and references therein). Once it invaded a new region, Ferrissia californica proved to be able to disperse locally using other physical and/or biological vectors. Genetic diversity and life-history traits (e.g. reproduction mode, see above) are known to determine the invasive potential of a species and its interactions with other taxa, and the combination of the two factors seems to have favoured the spreading of Ferrissia californica throughout invaded continents.
In conclusion, we could not find support for the current presence of indigenous Ferrissia species in the Old World, and it seems that the autochthonous taxa previously occurring in the area did not survive the Pleistocene or the early Holocene epochs. However, the possible presence of autochthonous Ferrissia species in North Africa and the Middle East, where Holocene subfossil records of Ferrissia were found (see also Marrone et al. Citation2014), cannot currently be ruled out. Thus, retrieving and analysing samples from these areas is now crucial in order to assess the possible presence of autochthonous Ferrissia in the Palaearctic, and to test the possible synonymy of F. clessiniana (which was described from northern Egypt) with F. californica.
Furthermore, taking into account the current lack of studies focusing on the impact exerted by Ferrissia californica on the autochthonous biota of invaded water bodies, future studies should ideally focus on this issue, evaluating whether, where, and how it might be necessary to monitor and manage this species in order to minimise the possibility of this documented “cryptic invasion” to evolve into an “evident trivialisation” of the Palaearctic biodiversity.
Supplemental TableS1
Download MS Excel (20.8 KB)Acknowledgements
Some Ferrissia samples were kindly provided by G. Alfonso (University of Salento, Italy), M. Bodon (University of Genova, Italy), S. Cianfanelli (Museum of Natural History, University of Florence, Italy), D. Cilia (St Paul’s Missionary College, Rabat, Malta), G. Manganelli (University of Siena, Italy), N. Riccardi (Italian National Research Council, Institute of Ecosystem Study, Verbania, Italy), J. Sala (Institute of Aquatic Ecology, University of Girona, Spain) and F. Stoch (Evolutionary Biology and Ecology Unit, Université Libre de Bruxelles, Belgium). The comments of two anonymous reviewers allowed us to improve a first draft of the manuscript.
Supplemental data
Supplemental data for this article can be accessed here.
References
- Akaike H. 1974. A new look at the statistical model identification. IEEE Transactions on Automatic Control 19:716–723. doi:10.1109/TAC.1974.1100705
- Albrecht C, Föller K, Clewing C, Hauffe T, Wilke T. 2013. Invaders versus endemics: Alien gastropod species in ancient Lake Ohrid. Hydrobiologia 739:163–174. doi:10.1007/s10750-013-1724-1
- Albrecht C, Kroll O, Terrazas EM, Wilke T. 2009. Invasion of ancient Lake Titicaca by the globally invasive Physa acuta (Gastropoda: Pulmonata: Hygrophila). Biological Invasions 11:1821–1826. doi:10.1007/s10530-008-9360-9
- Albrecht C, Kuhn K, Streit B. 2006. A molecular phylogeny of Planorboidea (Gastropoda, Pulmonata): Insights from enhanced taxon sampling. Zoological Scripta 36:27–39. doi:10.1111/j.1463-6409.2006.00258.x
- Bandelt H, Forster P, Röhl A. 1999. Median-joining networks for inferring intraspecific phylogenies. Molecular Biology and Evolution 16:37–48. doi:10.1093/oxfordjournals.molbev.a026036
- Beran L, Horsak M. 2007. Distribution of the alien freshwater snail Ferrissia fragilis (Tryon, 1863) (Gastropoda: Planorbidae) in the Czech Republic. Aquatic Invasions 2:45–54. doi:10.3391/ai.2007.2.1.5
- Bernasconi MP, Stanley DJ. 1994. Molluscan biofacies and their environmental implications, Nile Delta Lagoons, Egypt. The Journal of Coastal Research 10:440–465.
- Boettger CR. 1949. Die Einschleppung einer nordamerikanischen Süsswasserschnecke der Gattung Ferrissia nach Deutschland. Archiv Für Molluskenkunde 78:187.
- Bousset L, Henry PY, Sourrouille P, Jarne P. 2004. Population biology of the invasive freshwater snail Physa acuta approached through genetic markers, ecological characterization and demography. Molecular Ecology 13:2023–2036. doi:10.1111/j.1365-294X.2004.02200.x
- Brown DS. 1965. Freshwater gastropod Mollusca from Ethiopia. Bulletin of British Museum Natural History Zoology 12:37–94.
- Caron V, Ede FJ, Sunnucks P. 2014. Unravelling the Paradox of Loss of Genetic Variation during invasion: Superclones may explain the success of a clonal invader. PLoS One 9:e97744. doi:10.1371/journal.pone.0097744
- Christensen CC. 2016. Change of status and name for a Hawaiian freshwater limpet: Ancylus sharpi Sykes, 1900, is the invasive North American Ferrissia californica (Rowell, 1863), formerly known as Ferrissia fragilis (Tryon, 1863) (Gastropoda: Planorbidae: Ancylinae). British Museum Occasional Paper 118:5–8.
- Cilia DP. 2009. On the presence of the alien freshwater gastropod Ferrissia fragilis (Tryon, 1863) (Gastropoda: Planorbidae) in the Maltese Islands (Central Mediterranean). Bollettino Malacologico 45:123–127.
- Collado GA. 2014. Out of New Zealand: Molecular identification of the highly invasive freshwater mollusk Potamopyrgus antipodarum (Gray, 1843) in South America. Zoological Studies 53:1–9. doi:10.1186/s40555-014-0070-y
- Dillon RT, Wethington AR, Rhett JM, Smith TP. 2002. Populations of the European freshwater pulmonate Physa acuta are not reproductively isolated from American Physa heterostropha or Physa integra. Invertebrate Biology 121:226–234. doi:10.1111/j.1744-7410.2002.tb00062.x
- Duggan IC. 2010. The freshwater aquarium trade as a vector for incidental invertebrate fauna. Biological Invasions 12:3757–3770. doi:10.1007/s10530-010-9768-x
- Estoup A, Ravignè V, Hufbauer R, Vitalis R, Gautier M, Facon B. 2016. Is there a genetic paradox of biological invasion? Annual Review of Ecology, Evolution, and Systematics 47:51–72. doi:10.1146/annurev-ecolsys-121415-032116
- Excoffier L, Smouse PE, Quattro JM. 1992. Analysis of molecular variance inferred from metric distances among DNA haplotypes: Application to human mitochondrial DNA restriction data. Genetics 131:479–491.
- Felsenstein J. 1985. Confidence limits on phylogenies: An approach using the bootstrap. Evolution 39:783–791. doi:10.1111/evo.1985.39.issue-4
- Folmer O, Black M, Hoeh W, Lutz R, Vrijenhoek R. 1994. DNA primers for amplification of mitochondrial cytochrome c oxidase subunit 1 from diverse metazoan invertebrates. Molecular Marine Biology and Biotechnology 3:294–299.
- Girod A, Bianchi I, Mariani M. 1980. Guide per il riconoscimento delle specie animali delle acque interne italiane. Gasteropodi, 1 (Gastropoda: Pulmonata; Prosobranchia: Neritidae, Viviparidae Bithyniidae, Valvatidae). Vol. 7. Verona: Consiglio Nazionale delle Ricerche.
- Greke K. 2011. First record of the genus Ferrissia Walker, 1903 (Gastropoda: Planorbidae) from the Papuan region. Biodiversity, Biogeography and Nature Conservation in Wallacea and New Guinea. Vol. 1. Dmitry Telnov (Editor). Riga: The Entomological Society of Latvia. ISBN-13: 9789984976846.
- Guindon S, Gascuel O. 2003. A simple, fast, and accurate algorithm to estimate large phylogenies by maximum likelihood. Systematic Biology 52:696–704. doi:10.1080/10635150390235520
- Harzhauser M, Neubauer TA, Gross M, Binder H. 2014b. The early Middle Miocene mollusc fauna of Lake Rein (Eastern Alps, Austria). Palaeontographica Abteilung A: Palaeozoology – Stratigraphy 302:1–71. doi:10.1127/pala/302/2013/1
- Harzhauser M, Neubauer TA, Mandic O, Zuschin M, Ćorić S. 2012. A Middle Miocene endemic freshwater mollusc assemblage from an intramontane Alpine lake (Aflenz Basin, Eastern Alps, Austria). Palaontologische Zeitschrift 86:23–41. doi:10.1007/s12542-011-0117-x
- Incagnone G, Marrone F, Barone R, Robba L, Naselli-Flores L. 2015. How do freshwater organisms cross the “dry ocean”? A review on passive dispersal and colonization processes with a special focus on temporary ponds. Hydrobiologia 750:103–123. doi:10.1007/s10750-014-2110-3
- Khalloufi N, Boumaiza M. 2007. Première citation et description de quatre pulmonés dulcicoles de Tunisie (Mollusca, Orthogastropoda). Bulletin De La Société Zoologique De France 132:191–204.
- Kókay J. 2006. Nonmarine mollusc fauna from the Lower and Middle Miocene, Bakony Mts, W Hungary. Geologica Hungarica, Series Palaeontologica 56:3–196.
- Lacerda LEM, Richau CS, Amaral CRI, Silva DA, Carvalho EF, Santos SB. 2015. Ferrissia fragilis (Tryon, 1863): A freshwater snail cryptic invader in Brazil revealed by morphological and molecular data. Aquatic Invasions 10:157–168. doi:10.3391/ai
- Ladd HLA, Rogowski DL. 2012. Egg predation and parasite prevalence in the invasive freshwater snail, Melanoides tuberculata (Müller, 1774) in a west Texas spring system. Aquatic Invasions 7:287–290. doi:10.3391/ai.2012.7.2.016
- Lanfear R, Calcott B, Ho SYW, Guindon S. 2012. PartitionFinder: Combined selection of partitioning schemes and substitution models for phylogenetic analyses. Molecular Biology and Evolution 29:1695–1701. doi:10.1093/molbev/mss020
- Larkin MA, Blackshields G, Brown NP, Chenna R, McGettigan PA, McWilliam H, Valentin F, Wallace IM, Wilm A, Lopez R, Thompson JD, Gibson TJ, Higgins DG. 2007. Clustal W and Clustal X version 2.0. Bioinformatics 23:2947–2948. doi:10.1093/bioinformatics/btm404
- Librado P, Rozas J. 2009. DnaSP version 5: A software for comprehensive analysis of DNA polymorphism data. Bioinformatics 25:1451–1452. doi:10.1093/bioinformatics/btp187
- Mackie GL. 1999. Mollusc invasions through the aquarium trade. In: Claudi R, Leach JH, editors. Nonindigenous freshwater organisms: vectors, biology and impacts. Boca Raton: Lewis Publishers. pp. 135–147.
- Marrone F, Alfonso G, Miserocchi D, Lo Brutto S. 2011a. First record of Hemidiaptomus (Gigantodiaptomus) superbus (Schmeil, 1895) in Italy, with notes on distribution and conservation status (Copepoda, Calanoida, Diaptomidae). Journal of Limnology 70:149–155. doi:10.3274/JL11-70-1-18
- Marrone F, Lo Brutto S, Arculeo M. 2011b. Cryptic invasion in Southern Europe: The case of Ferrissia fragilis (Pulmonata: Ancylidae) Mediterranean populations. Biologia - Section Zoology 663:484–490. doi:10.2478/s11756-011-0044-z
- Marrone F, Lo Brutto S, Hundsdoerfer AK, Arculeo M. 2013. Overlooked cryptic endemism in copepods: Systematics and natural history of the calanoid subgenus Occidodiaptomus Borutzky 1991 (Copepoda, Calanoida, Diaptomidae). Molecular Phylogenetics and Evolution 66:190–212. doi:10.1016/j.ympev.2012.09.016
- Marrone F, Naselli-Flores L. 2015. A review on the animal xenodiversity in Sicilian inland waters (Italy). Advances in Oceanography and Limnology 6:5451. doi:10.4081/aiol.2015.5451
- Marrone F, Naser MD, Amaal GY, Sacco F, Arculeo M. 2014. First record of the North American cryptic invader Ferrissia fragilis (Tryon, 1863) (Mollusca: Gastropoda: Planorbidae) in the Middle East. Zoology in the Middle East 60:39–45. doi:10.1080/09397140.2014.892332
- Meijer T. 1987. Ferrissia wautieri fossiel in Nederland. Correspondentieblad Van De Nederlandse Malacologische Vereniging 238:332–337.
- Mienis HK, Ashkenazi S. 2011. Lentic Basommatophora molluscs and hygrophilous land snails as indicators of habitat and climate in the Early-Middle Pleistocene (0.78 Ma) at the site of Gesher Benot Ya’aqov (GBY), Israel. Journal of Human Evolution 60:328–340. doi:10.1016/j.jhevol.2010.03.009
- Mienis HK, Rittner O. 2013. On the distribution and status of the River limpet Ancylus fluviatilis O. F. Müller, 1774 (Mollusca, Gastropoda, Planorbidae) in Israel. MalaCo 9:460–462.
- Naser MD, Son MO, Yasser AG. 2011. Assessing the risks of invasions of aquatic invertebrates in the Shatt Al-Arab River. Russian Journal Biological Invasions 2:120–125. doi:10.1134/S2075111711020081
- QGIS Development Team. 2016. QGIS Geographic Information System, version 2.18.2. Open Source Geospatial Foundation Project. Available: http://www.qgis.org/. Accessed April 2017 1
- R Core Team. 2015. R: A language and environment for statistical computing, version 3.2.1. Vienna, Austria: R Foundation for Statistical Computing. Available: https://www.R-project.org. Accessed April 2017 1.
- Raposeiro PM, Costa AC, Martins AF. 2011. On the presence, distribution and habitat of the alien freshwater snail Ferrissia fragilis (Tryon, 1863) (Gastropoda: Planorbidae) in the oceanic islands of the Azores. Aquatic Invasions 6(SUPPL.1):S13–S17. doi:10.3391/ai.2011.6.S1.003
- Rixon CAM, Duggan IC, Bergeron NMN, Ricciardi A, MacIsaac HJ. 2005. Invasion risks posed by the aquarium trade and live fish markets to the Laurentian Great Lakes. Biodiversity and Conservation 14:1365–1381. doi:10.1007/s10531-004-9663-9
- Roger J, Calas P. 1944. Quelques mots sur les Ancylidae. Bulletins Mensuels - Société Linnéenne De Lyon 13:31–32. doi:10.3406/linly.1944.13136
- Roman J, Darling JA. 2007. Paradox lost: Genetic diversity and the success of aquatic invasions. Trends in Ecology & Evolution 22:454–464. doi:10.1016/j.tree.2007.07.002
- Ronquist F, Teslenko M, Van der Mark P, Ayres DL, Darling A, Höhna S, Larget B, Liu L, Suchard MA, Huelsenbeck JP. 2012. MrBayes v. 3.2: Efficient Bayesian phylogenetic inference and model choice across a large model space. Systematic Biology 61:539–542. doi:10.1093/sysbio/sys029
- Schlickum WR. 1976. Die in der pleistozänen Gemeindekiesgrube von Zwiefaltendorf a.d. Donau abgelagerte Molluskenfauna der Silvanaschichten. Archiv Für Molluskenkunde 107:1–31.
- Schrieber K, Lachmuth S. 2017. The Genetic Paradox of Invasions revisited: The potential role of inbreeding × environment interactions in invasion success. Biological Reviews 92:939–952. doi:10.1111/brv.12263
- Semenchenko V, Laenko T. 2008. First record of the invasive North American gastropod Ferrissia fragilis (Tryon, 1863) from the Pripyat River basin, Belarus. Aquatic Invasions 3:80–82. doi:10.3391/ai.2008.3.1.12
- Son MO. 2007. North American freshwater limpet Ferrissia fragilis (Tryon, 1863) (Gastropoda: Planorbidae) - A cryptic invader in the Northern Black Sea Region. Aquatic Invasions 2:55–58. doi:10.3391/ai.2007.2.1.6
- Spyra A. 2008. The septifer form of Ferrissia wautieri (Mirolli, 1960) found for the first time in Poland. Mollusca 26:95–98.
- Taylor DW. 2003. Introduction to Physidae (Gastropoda: Hygrophila); biogeography, classification, morphology. Revista De Biología Tropical 51:1–287.
- Thomas MG, Weale ME, Jones AL, Richards M, Smith A, Redhead N, Torroni A, Scozzari R, Gratrix F, Tarekegn A, Wilson JF, Capelli C, Bradman N, Goldstein DB. 2002. Founding mothers of Jewish communities: Geographically separated Jewish groups were independently founded by very few female ancestors. The American Journal of Human Genetics 70:1411–1420. doi:10.1086/340609
- Tokinova RP. 2012. The first finding of the North American freshwater limpets Ferrissia fragilis (Tryon, 1863) (Mollusca, Gastropoda) in the Middle Volga basin. Russian Journal of Biological Invasions 3:52–55. doi:10.1134/S2075111712010134
- Van Der Velde G. 1991. Population dynamics and population structure of Ferrissia wautieri (Mirolli, 1960) (Gastropoda, Ancylidae) in a pond near Nijmegen (The Netherlands). Hydrobiological Bulletin 24:141–144. doi:10.1007/BF02260431
- Walther AC, Burch JB, Foighil DÓ. 2010. Molecular phylogenetic revision of the freshwater limpet genus Ferrissia (Planorbidae: Ancylinae) in North America yields two species: Ferrissia (Ferrissia) rivularis and Ferrissia (Kincaidilla) fragilis. Malacologia 53:25–45. doi:10.4002/040.053.0102
- Walther AC, Lee T, Burch JB, Foighil DÓ. 2006a. Acroloxus lacustris is not an ancylid: A case of misidentification involving the cryptic invader Ferrissia fragilis (Mollusca: Pulmonata: Hygrophila). Molecular Phylogenetics and Evolution 39:271–275. doi:10.1016/j.ympev.2005.08.014
- Walther AC, Lee T, Burch JB, Foighil DÓ. 2006b. E Pluribus Unum: A phylogenetic and phylogeographic reassessment of Laevapex (Pulmonata: Ancylidae), a North American genus of freshwater limpets. Molecular Phylogenetics and Evolution 40:501–516. doi:10.1016/j.ympev.2006.03.019
- Walther AC, Lee T, Burch JB, Ó Foighil D. 2006c. Confirmation that the North American ancylid Ferrissia fragilis (Tryon, 1863) is a cryptic invader of European and East Asian freshwater ecosystems. Journal of Molluscan Studies 72:318–321. doi:10.1093/mollus/eyl009
- Wautier J. 1974. Premières données sur la répartition en Europe de Ferrissia wautieri (Gastropoda, Ancylidae). Bulletin De La Société Zoologique De France 99:715–723.
- Wethington AR, Lydeard C. 2007. A molecular phylogeny of Physidae (Gastropoda: Basommatophora) based on mitochondrial DNA sequences. Journal of Molluscan Studies 73:241–257. doi:10.1093/mollus/eym|021