Abstract
It is known that the freshwater heterotrich ciliate Blepharisma japonicum uses five pigments called blepharismins, stored in its extrusive pigment granules, for both light perception and chemical defense against predators. In this work we focused our attention on the defensive strategies of two additional pigmented species of Blepharisma, B. stoltei and B. undulans. In particular: (1) we observed the predator–prey interactions of B. stoltei or B. undulans against one multicellular and two unicellular predators; (2) we clarified the nature of B. stoltei and B. undulans pigments by means of High Performance Liquid Chromatography (HPLC) and Liquid Chromatography Mass Spectrometry (LC-MS); and (3) we demonstrated and compared the toxicity of the purified pigments on a panel of ciliated protists, and against one metazoan predator. The results indicate that the chemical defense mechanism present in B. stoltei and B. undulans is mediated by the same five blepharismins previously characterized for B. japonicum, although produced in different proportions.
Introduction
Blepharismins (BPs) are multifunctional quinone derivatives identified in the species of heterotrich ciliates belonging to the genus Blepharisma Perty, 1849. In B. japonicum Suzuki, 1954, the best-studied photophobic species of the genus, BPs are produced as a mixture of five compounds (BP-A, -B, -C, -D and -E) structurally related to hypericin, a photodynamic toxin of Hypericum perforatum Linnaeus, 1753, and stentorin, produced by Stentor coeruleus Ehrenberg, 1830, another negatively phototactic ciliate (Lobban et al. Citation2007; Matsuoka et al. Citation2010). As in other toxin-producing heterotrichs, BPs are stored in membrane-bound extrusive organelles (pigment granules) usually 0.3–0.6 μm diameter, arranged in stripes between the rows of cilia. To date, two primary functions of BPs have been clarified: light perception, and defense against predators (Miyake et al. Citation1990; Harumoto et al. Citation1998; Muto et al. Citation2001; Lobban et al. Citation2007; Uruma et al. Citation2007; Matsuoka et al. Citation2010).
With regard to light perception, B. japonicum shows a temporal backward swimming or rotating movement (step-up photophobic response) if exposed to a sudden increase in light intensity. The step-up photophobic response helps the cells avoid strongly illuminated regions, and lethal damage due to the photodynamic action of BPs (Kato & Matsuoka Citation1995). It was demonstrated that the photosensitive region is localized in the anterior end of the cell body where only BP-B is present, suggesting that this pigment may represent the photoreceptor mediating the step-up photophobic response (Matsuoka et al. Citation1997).
In addition to light perception, BPs were found to act as chemical weapons via their light-independent cytotoxic effect against predatory protozoans and methicillin-resistant Gram-positive bacteria (Miyake et al. Citation1990; Pant et al. Citation1997; Harumoto et al. Citation1998). A possible explanation for this cytotoxicity can be found in the capability of BPs to form cation-selective channels in planar phospholipid bilayers (Muto et al. Citation2001), a phenomenon also expected to occur in the cell membranes of microorganisms exposed to toxic concentrations of ciliate pigments.
From a more general point of view, the toxic secondary metabolites from ciliates have recently attracted the attention of an increasing number of researchers also for their pharmacological and medical applications (Petrelli et al. Citation2012; Catalani et al. Citation2016; Perrotta et al. Citation2016).
In this work: (1) we investigated whether the chemical defense mechanism known in B. japonicum was also present in B. stoltei Isquith, 1966 and B. undulans Stein, 1868, exposing both intact cells and pigment-deficient cells of these two species to unicellular and multicellular predators; (2) we clarified the nature of the pigments produced by B. stoltei and B. undulans using High Performance Liquid Chromatography (HPLC) and Liquid Chromatography Mass Spectrometry (LC-MS) analyses; and (3) we demonstrated and compared the toxicity of the purified pigments on a panel of free-living and raptorial ciliates, and against one microturbellarian predator.
Materials and methods
Organisms and culture methods
Blepharisma japonicum strain R1072 (Harumoto et al. Citation1998), Blepharisma stoltei strain HT-IV and Blepharisma undulans strain K78 (Kobayashi et al. Citation2015) were cultured in Synthetic Medium for Blepharisma (SMB) medium (Miyake Citation1981) and fed with the flagellate Chlorogonium elongatum Dangeard, 1899, cultivated as described in Buonanno et al. (Citation2005), or in Jaworski’s Medium (JM) solution. Light microscopy observations were carried out in vivo, in SMB or in a solution of methyl cellulose in SMB (2%) to slow cilia motility. Coleps hirtus Müller, 1786 (clone PC-4) (Buonanno et al. Citation2014), Euplotes aediculatus Pierson, 1943 (Buonanno et al. Citation2017), Paramecium multimicronucleatum Powers & Mittchell, 1910 (clone TL-2, collected in Lake Trasimeno, Perugia, Italy) and Stentor roeseli Ehrenberg, 1835 (clone TL-4) (Buonanno et al. Citation2017) were also cultured in SMB and fed with C. elongatum. Stenostomum sphagnetorum Luther, 1960 (Platyhelminthes: Turbellaria), a common freshwater ciliate predator (Buonanno Citation2005), was cultured as described in Buonanno et al. (Citation2013).
Removal of pigment granules and toxicity tests of BPs
In this work, the cold shock-based method (Buonanno & Ortenzi Citation2016) was applied to B. japonicum, B. stoltei or B. undulans in order to obtain the pigment granule-deficient (PGD) cells. These cells appear partially or totally bleached, and are substantially healthy as control cells (data not shown). The same procedure allows us also to collect the toxin-enriched supernatant (TES) containing the discharge from the pigment granules.
To evaluate the toxicity of the purified BPs, triplicate samples of 10 ciliate cells or metazoan specimens were placed in depression slides containing 250 µL SMB and increasing concentrations of each toxin (from 1 to 100 µg/mL). The number of surviving organisms (normal morphology and locomotion) was counted, after 24 h, and the median lethal concentrations (LC50) were estimated on the basis of a concentration–survival curve, essentially according to the procedure described by Buonanno (Citation2009). The data were evaluated by nonlinear regression analysis using GraphPad Prism 6 software (GraphPad Software, San Diego, CA, USA) with the 95% level of confidence.
Predator–prey experiments
Samples of five or 10 cells of either untreated or PGD cells of B. japonicum, B. stoltei or B. undulans were mixed with samples of 500 cells of C. hirtus, 20 cells of S. roeseli or 10 specimens of S. sphagnetorum in 250 µL of SMB. The mixtures involving C. hirtus as predator are here termed 500C-5J, 500C-5S, 500C-5U and 500C-10J, 500C-10S, 500C-10U, respectively, for those involving five or 10 cells of B. japonicum, B. stoltei or B. undulans. Each mixture was prepared in six replicates, for both untreated and PGD cells of the three Blepharisma species, and observed under a stereomicroscope after 2 or 24 h. The data represent the means ± standard error (SE) of six independent determinations, and the significance of the differences between the mean values was examined by Student’s t-test with the significance threshold set at p < 0.005.
Chemical characterization of BPs by LC-MS
Lyophilized ethanol extracts of Blepharisma cell cultures or aliquots of lyophilized TES were resuspended in ethanol, sonicated in an ultrasonic bath for 15 min (Sonorex Super, Bandelin electronics, Berlin, Germany), and centrifuged at 3000 × g for 10 min at room temperature. The procedure was repeated 3 times, and the supernatant was dried down in a rotatory evaporator and re-suspended in 500 μL of methanol.
Ten-microliter aliquots were injected and analyzed in negative ionization mode on a Hewlett-Packard Model 1100 Series liquid chromatograph (Hewlett-Packard Development Company, L.P., Palo Alto CA, USA) coupled both to a Bruker Esquire-LC quadrupole Ion Trap Mass Spectrometer (IT-MS) equipped with an Electrospray Ionization (ESI) source (Bruker Optik GmbH, Ettlingen, Germany) and to a photo diode-array detector (DAD) (Agilent Technologies, Milan, Italy, Agilent 1100). Chromatographic separation was carried out on a Zorbax Eclipse XDB-C18 column (150 × 4.6 mm inner diameter, pore size 200 Å, particle size 3.5 µm; Hewlett Packard, Palo Alto, CA, USA), with a linear gradient of solvent A (methanol: water 7:3, containing 12 mM ammonium acetate) and solvent B (methanol containing 12 mM ammonium acetate) from 0%B to 100%B in 30 min, at a constant flow rate of 1.0 mL/min. Final conditions were kept for at least 30 min. Mass range was 50–1200 m/z, and high-voltage capillary was set at −4000 V. DAD was operated at 205, 215, 254, 574 and 590 nm. High-resolution MS was conducted using same chromatographic setup on an Orbitrap Fusion™ Tribrid™ Mass Spectrometer (Thermo Fisher Scientific, Waltham, MA, USA).
Results and discussion
Predator–prey interactions
It is known that one of the main functions of BPs in B. japonicum is chemical defense against unicellular predators (Harumoto et al. Citation1998; Terazima & Harumoto Citation2004). To investigate whether this function was also present in B. stoltei and B. undulans (), we observed the predator–prey interaction of untreated or PGD cells of the Blepharisma species against one multicellular (S. sphagnetorum) predator and two unicellular predators (C. hirtus and S. roeseli). A significant difference in prey survival between untreated and PGD cells of B. japonicum, B. stoltei of B. undulans was observed in mixtures involving C. hirtus as predator. After 2 h, all untreated B. japonicum (5, n = 6), B. stoltei (5, n = 6), and B. undulans cells (5, n = 6) lived, while the surviving PGD cells of B. japonicum (3.33 ± 0.2, n = 6), B. stoltei (3.67 ± 0.2, n = 6) and B. undulans (1.00 ± 0.4, n = 6) were significantly fewer, strongly suggesting the presence of a chemical defense system in untreated Blepharisma cells, against C. hirtus ().
Figure 1. Micrographs of living cells of A, Blepharisma stoltei and B, Blepharisma undulans. The pigment granules are slightly visible as red/pink dots (arrows) located in the region between ciliary lines, in correspondence to large and transparent contractile vacuoles at the posterior end of the ciliates. Scale bars = 50 µm.
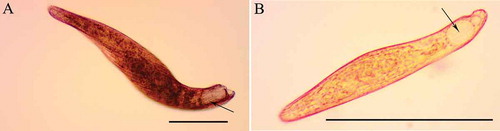
Figure 2. Effect of pigment granule removal in cells of Blepharisma japonicum, Blepharisma stoltei and Blepharisma undulans, obtained by cold-shock treatment, on the predator–prey interaction against Coleps hirtus. Groups of five or 10 cells of either pigment granule-deprived (PGD) or untreated cells of B. japonicum, B. stoltei or B. undulans were mixed with groups of 500 cells of C. hirtus (the 500C-5J, 500C-5S, 500C-5U and 500C-10J, 500C-10S, 500C-10U, respectively). Each bar represents the mean (± standard error, SE) of six independent experiments. *P < 0.005 and **P < 0.0001.
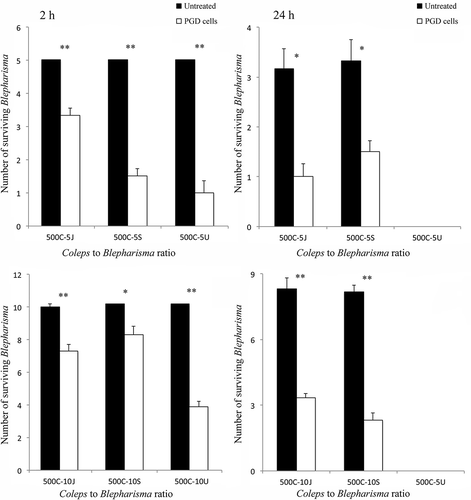
Also in the mixtures involving groups of 10 prey, after 2 h, significant differences were observed in survival values between untreated cells of B. japonicum (9.83 ± 0.17, n = 6), B. stoltei (10, n = 6), B. undulans (10, n = 6) and PGD cells of B. japonicum (7.17 ± 0.40, n = 6), B. stoltei (8.17 ± 0.48, n = 6), B. undulans (3.83 ± 0.31, n = 6). The apparent reduction in the predator’s efficiency, if compared to the data obtained with groups of five prey, appears to be linked to the different ratios between predators and prey as already observed in interactions involving other ciliates (Buonanno et al. Citation2012, Citation2014). However, the predatory action of C. hirtus was appreciably more effective on PGD of Blepharisma cells than on control cells, with statistically significant results, in any observed ratios.
A comparable result is also observed after 24 h for B. japonicum and B. stoltei cells, while both untreated and PGD groups of cells of B. undulans disappeared, probably because in long-period interactions, the predator is able to overcome the defense of B. undulans ().
Parallel predator–prey experiments were also performed using the microturbellarian S. sphagnetorum and the ciliate S. roeseli as predators. In the mixtures involving S. sphagnetorum, all untreated or PGD cells of the three Blepharisma species disappeared after 2 h, suggesting that the chemical defense is ineffective against this predator (data not shown).
S. roeseli is a sedentary ciliate which beats its adoral cilia rhythmically to drive food, such as bacteria or other protists, into its cytostome. It has been systematically observed (20 independent experiments) that this ciliate is not able to ingest specimens of B. japonicum or B. stoltei, due to the large cell body of these two species, whereas it is able to ingest the substantially smaller specimens of B. undulans (both untreated and PGD cells). Although S. sphagnetorum and S. roeseli seem to overcome Blepharisma’s chemical defense, it was observed that after the ingestion of intact cells of the toxic ciliates these predators are not able to reproduce, suggesting the presence of post-ingestion toxicity phenomena. To investigate whether S. sphagnetorum could be affected by the toxic pigments of B. undulans, one specimen of the predator was fed with 150 cells of B. undulans or with 150 cells of Paramecium multimicronucleatum (a non-toxic prey was used as control) in 500 µL of SMB, and the number of specimens of S. sphagnetorum was counted after 120 h. As shown in , S. sphagnetorum fed with B. undulans can reproduce only when fed with P. multimicronucleatum, whereas this ability is lost when it is fed with B. undulans and some predator specimens start to die after 48, strongly suggesting the presence of post-ingestion toxicity phenomena in the turbellarian. Similar results were also obtained using S. roeseli as predator (data not shown).
Figure 3. Comparison between growth curves of Stenostomum sphagnetorum fed either with Blepharisma undulans or with Paramecium multimicronucleatum. One specimen of S. sphagnetorum was mixed with 150 cells of B. undulans, or with 150 cells of P. multimicronucleatum as control. The specimens of S. sphagnetorum were counted for 120 h. Each point represents the mean (± standard error, SE) of nine independent experiments. Inset, a specimen of S. sphagnetorum with engulfed cells of B. undulans (arrow) visible as a dark area in the intestine. Scale bar = 50 µm.
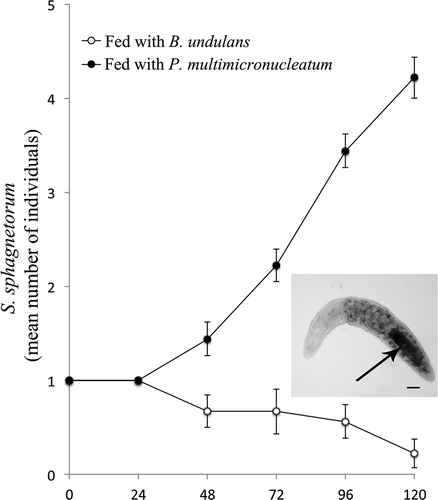
These results are in apparent contrast with the data collected in a previous study, where it was observed that S. sphagnetorum is able to recognize and avoid toxic prey such as the ciliates Spirostomum teres Claparede et Lachmann, 1858 or Spirostomum ambiguum Ehrenberg, 1835 (Buonanno Citation2011). Nevertheless, in the interaction with Blepharisma, the toxic pigments do not immediately affect the microturbellarian as in the case reported above involving S. teres or S. ambiguum, and a long time of “exposure” to the ingested prey is needed to damage S. sphagnetorum.
Overall, these results show that like B. japonicum, B. stoltei and B. undulans also present a chemical defense mechanism against predators.
BPs of B. stoltei and B. undulans
Toxin-enriched supernatants (TES) containing the discharge from the pigment granules of B. japonicum, B. stoltei and B. undulans were fractionated by reverse-phase chromatography (RP-HPLC) to obtain the elution profiles reported in . We can reasonably state that pigments isolated from B. stoltei and B. undulans are equivalent to BPs found in B. japonicum, as they showed (a) the same retention times under RP-HPLC, (b) the same m/z under low- and high-resolution MS, and (c) the same UV-visible spectra recorded for BPs of B. japonicum.
Figure 4. HPLC fractionation of the pigmented toxin enriched supernatant (TES) of (a) Blepharisma japonicum, (b) Blepharisma stoltei, and (c) Blepharisma undulans.
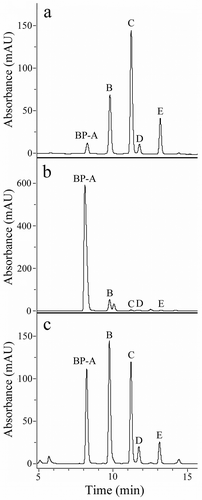
For each species, the mean relative abundances of BPs obtained from a suspension of 100,000 cells were as follows:
B. japonicum: BP-A, 76 µg; BP-B, 287 µg; BP-C, 331 µg; BP-D, 58 µg; BP-E, 133 µg. B. stoltei: BP-A, 1.3 mg; BP-B, 179 µg; trace amounts (≥1% of total BPs) of BP-C, -D, and – E. B. undulans: BP-A, 285 µg; BP-B, 322 µg; BP-C, 297 µg; BP-D, 69 µg; BP-E, 87 µg.
It is worth noting that, differently from the situation described for other ciliate genera, such as Euplotes Ehrenberg, 1831, Pseudokeronopsis Borror & Wicklow, 1983, Spirostomum Ehrenberg, 1834 or Stentor Oken, 1815, in which different species can produce diverse or even biogenetically distant specific secondary metabolites (Guella et al. Citation2010; Buonanno Citation2011; Buonanno et al. Citation2012, Citation2017; Höfle et al. Citation2014; Sera et al. Citation2015; Anesi et al. Citation2016), in the genus Blepharisma different species appear to conserve equivalent structures, although produced in different proportions.
Toxicity of BPs
To evaluate the contribution of each of the five BPs present in the pigment granules of Blepharisma to the toxic effects of their discharge, we analyzed the effects of purified aliquots of these molecules on four species of grazing and raptorial ciliates, and on one microturbellarian predator. For this purpose, a set of dose–response experiments was performed and the results indicated that all BPs exerted an appreciable toxic effect on all species examined ().
Table I. Comparison of the toxic effects of the five purified blepharismins (BP-A; BP-B; BP-C; BP-D and BP-E) on the ciliates Coleps hirtus, Euplotes aediculatus, Paramecium multimicronucleatum and Stentor roeseli and on the microturbellarian Stenostomum sphagnetorum. Viability was assessed after 24 h of incubation and the LC50 values were obtained by nonlinear regression analysis of three independent experiments, with the 95% confidence limits calculated using GraphPad Prism 6 software.
BP-A proved to be the most toxic compound against ciliates, with an LC50 ranging from 0.8 to 5.15 μg/mL. Similar to BP-A, BP-B also showed high toxicity against target ciliates (LC50 from 1.01 to 10.94 μg/mL), whereas BP-C, -D and -E showed a weaker toxicity (LC50 from 1.03 to 24.28 μg/mL), particularly against E. aediculatus and S. roeseli (LC50 from 11.38 to 24.28 μg/mL). Comparable values of toxicity were observed for all BPs against S. sphagnetorum (LC50 from 20.45 to 23.89 μg/mL). In addition, it was observed that B. japonicum, B. stoltei and B. undulans are substantially immune to all BPs, showing an LC50 > 100 µg/mL (data not shown).
Interestingly, BP-A is particularly abundant in B. stoltei that also produces an appreciable amount of BP-B, and trace amounts of the other BPs. It is likely that in this ciliate the defensive role is essentially played by BP-A, especially in consideration of its relative abundance, with the less-represented BP-B primarily mediating the step-up photophobic response of the ciliate, according to the function proposed for this BP in B. japonicum by Matsuoka et al. (Citation1997).
Even if the two most hydrophilic BPs, BP-A and BP-B, showed the highest activity, it appears that exposure to toxic concentrations of each purified BP can result in lethal damage to target organisms, as shown in . This observation is in full accord with the cytotoxic mechanism previously proposed for all BPs, which proves capable of aggregating in clusters of different sizes and forming channels of different conductance in planar phospholipid bilayers (Muto et al. Citation2001). Assuming that the same action mechanism may also occur in vivo, resulting in the formation of transmembrane pores and subsequent cell lysis, channel formation might be indicated as the main physiological effect of BPs on both unicellular and multicellular organisms.
Conclusion
The data collected in this work show that three species of Blepharisma share a common panel of multifunctional secondary metabolites (BPs), whereas intra- and interspecific molecular diversity appears to be a constant for the secondary metabolites characterized in the other genera of ciliates investigated previously. The conservation of this panel of secondary metabolites can be observed even in the limited case of B. stoltei, where two BPs are predominant, but the presence of all five BPs is confirmed, suggesting that distinct roles for these molecules are likely required at least for the fine control of photophobic reactions, as proposed by Matsuoka et al. (Citation1997). Otherwise, with regard to the defensive role ascribed to the title compounds, our data confirm that each of the five BPs can exert an effective toxic/lethal effect on raptorial ciliates or multicellular predators, in full accord with the action mechanism proposed by Muto et al. (Citation2001). Here we focused our attention on three pigmented species of Blepharisma, but an extension of the analyses among this genus to secondary metabolites produced by colorless species, such as B. hyalinum Perty, 1852, may pave the way to an increased body of data and to obtain a more representative picture of the metabolomics of single-celled ciliated eukaryota.
Acknowledgements
We thank Dr. Terue Harumoto (Nara Women’s University, Japan) for sending us the strains of B. stoltei and B. undulans. We also thank Dr. Graziano Di Giuseppe (University of Pisa, Italy) for the determination of P. multimicronucleatum used in this work, and Dr. Gill Philip (University of Macerata, Italy) for the linguistic revision of the text.
Additional information
Funding
References
- Anesi A , Buonanno F , Di Giuseppe G , Ortenzi C , Guella G. 2016. Metabolites from the euryhaline ciliate Pseudokeronopsis erythrina . European Journal of Organic Chemistry 7:1330–1336. DOI:10.1002/ejoc.201501424.
- Buonanno F. 2005. Variations in the efficiency of ciliate extrusomal toxins against a common ciliate predator, the catenulid Stenostomum sphagnetorum . Italian Journal of Zoology 72:293–295. DOI:10.1080/11250000509356688.
- Buonanno F . 2009. Antipredator behavior of freshwater microturbellarian Stenostomum sphagnetorum against the predatory ciliate Dileptus margaritifer . Zoological Science 26:443–447. DOI:10.2108/zsj.26.443.
- Buonanno F . 2011. The changes in the predatory behavior of the microturbellarian Stenostomum sphagnetorum on two species of toxin-secreting ciliates of the genus Spirostomum . Biologia 66/4:648–653. DOI:10.2478/s11756-011-0061-y.
- Buonanno F , Anesi A , Di Giuseppe G , Guella G , Ortenzi C . 2017. Chemical defense by erythrolactones in the euryhaline ciliated protist, Pseudokeronopsis erythrina . Zoological Science 34:42–51. DOI:10.2108/zs160123.
- Buonanno F , Anesi A , Guella G , Kumar S , Bharti D , La Terza A , Quassinti L , Bramucci M , Ortenzi C . 2014. Chemical offence by means of toxicysts in the freshwater ciliate, Colpes hirtus . Journal of Eukaryotic Microbiology 61:293–304. DOI:10.1111/jeu.12106.
- Buonanno F , Guella G , Strim C , Ortenzi C . 2012. Chemical defence by mono-prenyl hydroquinone in a freshwater ciliate, Spirostomum ambiguum . Hydrobiologia 684:97–107. DOI:10.1007/s10750-011-0972-1.
- Buonanno F , Harumoto T , Ortenzi C . 2013. The defensive function of trichocysts in Paramecium tetraurelia against metazoan predators compared with the chemical defense of two species of toxin-containing ciliates. Zoological Science 30:255–261. DOI:10.2108/zsj.30.255.
- Buonanno F , Ortenzi C . 2016. Cold-shock based method to induce the discharge of extrusomes in ciliated protists and its efficiency. Journal of Basic Microbiology 56:586–590. DOI:10.1002/jobm.201500438.
- Buonanno F , Saltalamacchia P , Miyake A . 2005. Defense function of pigmentocysts in the karyorelictid ciliate Loxodes striatus . European Journal Protistology 41:151–158. DOI:10.1016/j.ejop.2005.01.001.
- Catalani E , Proietti Serafini F , Zecchini S , Picchietti S , Fausto AM , Marcantoni E , Buonanno F , Ortenzi C , Perrotta C , Cervia D . 2016. Natural products from aquatic eukaryotic microorganisms for cancer therapy: Perspectives on anti-tumour properties of ciliate bioactive molecules. Pharmacological Research 113(A):409–420. DOI:10.1016/j.phrs.2016.09.018.
- Guella G , Skropeta D , Di Giuseppe G , Dini F . 2010. Structures, biological activities and phylogenetic relationships of terpenoids from marine ciliates of the genus Euplotes . Marine Drugs 8:2080–2116. DOI:10.3390/md8072080.
- Harumoto T , Miyake A , Ishikawa N , Sugibayashi R , Zenfuku K , Iio H . 1998. Chemical defense by means of pigmented extrusomes in the ciliate Blepharisma japonicum . European Journal Protistology 34:458–470. DOI:10.1016/S0932-4739(98)80014-X.
- Höfle G , Reinecke S , Laude U , Kabbe K , Dietrich S . 2014. Amethystin, the coloring principle of Stentor amethystinus . Journal of Natural Products 77:1383−1389. DOI:10.1021/np5001363.
- Kato Y , Matsuoka T . 1995. Photodynamic action of the pigment in ciliated protozoan Blepharisma . Journal of Protozoology Research 5:136–140.
- Kobayashi M , Miura M , Takusagawa M , Sugiura M , Harumoto T . 2015. Two possible barriers blocking conjugation between different megakaryotypes of Blepharisma . Zoological Science 32:53–61. DOI:10.2108/zs140151.
- Lobban CS , Hallam SJ , Mukherjee P , Petrich JW . 2007. Photophysics and multifunctionality of hypericin-like pigments in heterotrich ciliates: A phylogenetic perspective. Photochemistry and Photobiology 83:1074–1094. DOI:10.1111/j.1751-1097.2007.00191.x.
- Matsuoka T , Kotsuki H , Muto Y . 2010. Multi-functions of photodynamic pigments in ciliated ptotozoans. In: Méndez-Vilas A , editor. Current research, technology and education topics in applied microbiology and microbial biotechnology. Badajoz: Formatex. pp. 419–426.
- Matsuoka T , Satol M , Maeda M , Naoki H , Tanaka T , Kotsuki H . 1997. Localization of Blepharismin photosensors and identification of a photoreceptor complex mediating the step-up photophobic response of the unicellular organism, Blepharisma . Photochemistry and Photobiology 65:915–921. DOI:10.1111/php.1997.65.issue-5.
- Miyake A . 1981. Cell interaction by gamones in Blepharisma . In: O’Day DH , Horgen PA , editors. Sexual interaction in eukaryotic microbes. New York: Academic Press. pp. 95–129.
- Miyake A , Harumoto T , Salvi B , Rivola V . 1990. Defensive function of pigment granules in Blepharisma japonicum . European Journal of Protistology 25:310–315. DOI:10.1016/S0932-4739(11)80122-7.
- Muto Y , Matsuoka T , Kida A , Okano Y , Kirino Y . 2001. Blepharismins, produced by the protozoan, Blepharisma japonicum, form ion-permeable channels in planar lipid bilayer membranes. FEBS Letters 508:423–426. DOI:10.1016/S0014-5793(01)03110-6.
- Pant B , Kato Y , Kumagai T , Matsuoka T , Sugiyama M . 1997. Blepharismin produced by a protozoan Blepharisma functions as an antibiotic effective against methicillin-resistant Staphylococcus aureus . FEMS Microbiology Letters 155:67–71. DOI:10.1111/j.1574-6968.1997.tb12687.x.
- Perrotta C , Buonanno F , Zecchini S , Giavazzi A , Proietti Serafini F , Catalani E , Guerra L , Belardinelli MC , Picchietti S , Fausto AM , Giorgi S , Marcantoni E , Clementi E , Ortenzi C , Cervia D . 2016. Climacostol reduces tumour progression in a mouse model of melanoma via the p53-dependent intrinsic apoptotic programme. Scientific Reports 6:27281. DOI:10.1038/srep27281.
- Petrelli D , Buonanno F , Vitali LA , Ortenzi C . 2012. Antimicrobial activity of the protozoan toxin climacostol and its derivatives. Biologia 67:525–529. DOI:10.2478/s11756-012-0030-0.
- Sera Y , Masaki ME , Doe M , Buonanno F , Miyake A , Usuki Y , Iio H . 2015. Spirostomin, defense toxin of the ciliate Spirostomum teres: Isolation, structure elucidation, and synthesis. Chemistry Letters 44:633–635. DOI:10.1246/cl.150044.
- Terazima MN , Harumoto T . 2004. Defense function of pigment granules in the ciliate Blepharisma japonicum against two predatory protists, Amoeba proteus (Rhizopodea) and Climacostomum virens (Ciliata). Zoological Science 21:823–828. DOI:10.2108/zsj.21.823.
- Uruma Y , Sakamoto K , Takumi K , Doe M , Usuki Y , Iio H . 2007. Assignment of 13C NMR spectrum for blepharismin C based on biosynthetic studies. Tetrahedron 63:5548–5553. DOI:10.1016/j.tet.2007.04.015.