Abstract
Historically, the harvest and consumption of sea cucumbers have been largely restricted to Asian countries. Recently, however, the western world has witnessed a rapid growth of holothurian-related industries, and Holothuria mammata Grube, 1840 and H. sanctori Delle Chiaje, 1823 are now being commercially harvested in European and North African countries. The identification of holothurian species tends to be difficult and time-consuming, and in many cases needs experienced taxonomists. We suggest a simple non-destructive DNA sampling, using tube feet or oral tentacles from these two species. We also describe a simple and fast method of diagnose based on polymerase chain reaction-restriction fragment length polymorphism (PCR-RFLP) using restriction nuclease Sau3AI on 16S rRNA fragments. The use of RFLPs to rapidly and inexpensively identify species with no need for taxonomical or genetic expertise could prove a valuable asset for conservation and fishery purposes targeting commercially harvested animals such as the holothurians in the north-east Atlantic and the Mediterranean Sea.
Introduction
The sea cucumbers, class Holothuroidea, one of the five extant classes of the exclusively marine phylum Echinodermata (Pawson Citation2007), are a conspicuous presence throughout all oceans and seas, at all latitudes and depths, occurring in virtually any marine habitat, from corals to rocky, sandy, muddy and mangrove habitats (Purcell et al. Citation2012). Roughly 1400 extant species are known worldwide (Pawson Citation2007) but only 66 are commercially harvested, comprising predominantly species belonging to the order Aspidochirotida from tropical shallow waters (Purcell Citation2010). The processed products (cooked or dried), also known as “beche-de-mer”, “trepang” or “haishen”, are chiefly exported to Asian markets (Purcell et al. Citation2012). Possibly due to the over-exploitation of the typical valuable holothurians and in response to the growing demand for sea cucumber products (Purcell Citation2010), several Atlanto–Mediterranean holothurians are now being increasingly harvested, such as Holothuria mammata Grube, 1840 and H. sanctori Delle Chiaje, 1823 (see González-Wangüemert et al. Citation2016). This could pose a potential threat to many important ecosystems (e.g. seagrass beds) as many of the targeted species are viewed as key species, contributing to the recycling of bottom detritus as continuous deposit-feeders and, thus, playing an important role in the soft-sediment biochemical cycles (Mezali et al. Citation2003).
The identification of even well-known holothurian species tends to be difficult and time-consuming, and in many cases needs experienced taxonomists, particularly if dealing with preserved specimens (e.g. in ethanol, dried or frozen), which tend to suffer from varying degrees of shrinkage and deformation, and loss of internal organs through evisceration (Tortonese Citation1965; Borrero-Pérez et al. Citation2009). Furthermore, in sea-cucumber industries, many critical morphological characters tend to be destroyed or discarded (Wen et al. Citation2010). DNA-based genetic analyses have emerged as a popular and inexpensive tool within the echinoderm research community, used for a variety of objectives including monitoring populations in conservation and fishery studies (e.g. Uthicke et al. Citation2010; Wen et al. Citation2010). Problems associated with the identification of holothurian species in “beche-de-mer” transformed products, and the high incidence of fraud and illegal trading (e.g. marketing less-valuable species under the identification of high-value species), have led to multiple efforts in the development of genetic tools for the rapid identification and assessment (Uthicke et al. Citation2010). Polymerase chain reaction (PCR) amplification followed by restriction fragment length polymorphism (RFLP) techniques, particularly based on a fragment of the slow-evolving 16S region, have been presented as viable methods for identification of commercial species and authentication of highly valuable species by Asian markets (Wen et al. Citation2010).
Conservation and management of marine resources are increasingly demanding the development of tissue-sampling techniques that are as non-intrusive as possible (Beebee & Rowe Citation2008). For holothurians, the most widely targeted tissues are the muscles, gonads and gut (e.g. Uthicke et al. Citation2004; Borrero-Pérez et al. Citation2010; So et al. Citation2011), though other structures such as tentacles (Kerr et al. Citation2005) or the body wall (Uthicke et al. Citation2004; Borrero-Pérez et al. Citation2010) sporadically appear in the literature. Sampling any of these tissues does not necessarily result in the death of the specimen, due to the holothurian’s natural capacity for regeneration (García-Arrarás & Greenberg Citation2001). However, a non-destructive sampling method should favour: (1) a selection of tissues that do not imply the dissection of the specimens; (2) easy identification by untrained personnel; and, (3) if dealing with live specimens, the rapid regeneration of the specimens. The use of any internal structures could also prove to be an issue, as many shallow-water north-eastern Atlantic species naturally eviscerate (Tortonese Citation1965). Furthermore, internal parts are generally discarded in sea-cucumber fisheries (Purcell Citation2010). The tentacle tissue or the tube feet appear to be the best alternative, for they comply with any of the needs listed above – more so if dealing with collection specimens or commercialised animals, allowing for a fast assessment of several individuals (and easy storage) without specimen destruction (including of taxonomical characters).
We describe an easy, fast, non-intrusive method of DNA sampling and identification of two edible north-eastern Atlantic holothurian species, using RFLPs on 16s PCR products derived from tube feet and tentacle tissue.
Materials and methods
Sampled area and ecological considerations
The Azores is an archipelago composed of nine volcanic oceanic islands and several islets, spreading around 600 km in the north-east Atlantic (). Among the shallow-water echinoderms reported from the archipelago (Pereira Citation1997; Wirtz Citation2009), four species are currently considered edible: Parastichopus regalis (Cuvier, 1817), Holothuria forskali Delle Chiaje, 1823, H. mammata and H. sanctori (Ramón et al. Citation2010; Sicuro & Levine Citation2012; González-Wangüemert et al. Citation2016). The last two species are very common inhabitants of Azorean low intertidal and shallow subtidal areas, achieving occasionally spectacular densities in calm areas, such as protected bays, marine lagoons, ports and marinas (authors’ personal observation). These two species are also found throughout the Mediterranean and north-eastern Atlantic, from the Bay of Biscay to North Africa, including the archipelagos of Madeira and Canaries (Tortonese Citation1965). Additionally, H. sanctori extends its distribution southward and was reported in Cape Verde, Ascension Island and Santa Helena Island (Tortonese Citation1965).
Figure 1. Sampling sites (stars) in the Azores for Holothuria mammata and H. sanctori: eastern group (Caloura, São Miguel Island; N37°42ʹ25”, W25°30ʹ32”), central group (São Roque, Pico Island; N38°31ʹ39”, W28°19ʹ08”) and western group (Santa Cruz, Flores; N39°27ʹ17”, W31°07ʹ29”).
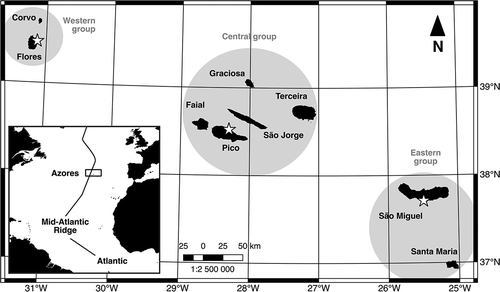
Figure 2. PCR products of 16S rRNA gene and respective RFLP profiles generated by restriction nucleases Sau3AI; marker (8). Holothuria sanctori (lanes 1–7): 16S rRNA PCR product (1) and RFLP profiles by island group, western group (2, 3), central group (4,5) and eastern group (6,7). Holothuria mammata (lanes 9 to13): 16S rRNA PCR product (9) and RFLP profiles by island group, western group (10, 11), central group (12) and eastern group (13). Note: Polymerase chain reaction (PCR); ribosomal ribonucleic acid (rRNA); restriction fragment length polymorphism (RFLP).
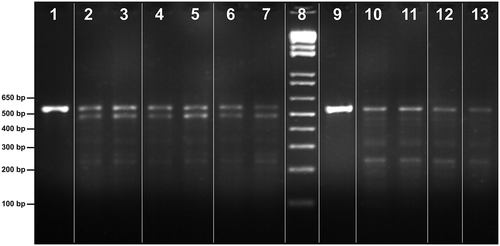
Holothuria mammata and H. sanctori are perfect candidates for a study of this nature in the Azores considering their easy access and abundance in the archipelago, as well as their geographical range and commercial potential. Individuals of these two species were collected during low tide or by snorkelling in four islands of the Azores, between 2010 and 2012, covering the eastern group (Caloura, São Miguel Island; N37°42ʹ25”, W25°30ʹ32”), the central group (São Roque, Pico Island; N38°31ʹ39”, W28°19ʹ08”) and the western group (Santa Cruz, Flores; N39°27ʹ17”, W31°07ʹ29”) (see . The identification was based on gross morphological characters and later confirmed by the observation of the ossicles under the binocular microscope, following Tortonese (Citation1965).
Tissue sampling and DNA extraction
For comparison purposes, several tissues were initially sampled in each individual: body wall, longitudinal muscle, intestine, tube feet and tentacle. Each sampled tissue was fixed in ethanol. DNA extraction was obtained on 15 mg of tissue using E.Z.N.A.® Mollusc DNA Kit (Omega, USA), with minor adaptations to the instructions in the supplier’s manual (vortex steps were replaced by manual agitation, and DNA elution buffer was replaced by purified water). DNA concentration was measured using a NanoVue Spectrophotometer (GE Healthcare Bio-Sciences).
PCR
A pair of invertebrate universal primers (16Sar: 5ʹ-CGCCTGTTTATCAAAAACAT-3ʹ and 16Sbr: 5ʹ-CTCCGGTTTGAACTCAGATCA-3ʹ) was used for PCR amplification. The conserved 16S region discriminates between species and this molecular marker is extensively used in invertebrate phylogenetic studies, including holothurian species targeted in this study (Borrero-Pérez et al. Citation2009, Citation2010). The thermal cycling profile started at 95°C, with an initial denaturation time of 5 min, followed by 35 cycles of 60 s denaturation at 95°C, 60 s annealing at 50°C and 60 s extension at 72°C, and a final extension of 10 min at 72°C. PCR products were screened by 1% agarose gel electrophoresis at 90 V for 60 min and visualised under UV exposure. The length of fragments was determined by comparison with commercial 1-kb DNA ladder (Invitrogen, UK).
RFLP analysis
The restriction nuclease Sau3AI (Promega) was tested on amplified PCR products without previous purification, from 10 sampled individuals (see . Following Stefanni et al. (Citation2009), reaction mixtures were slightly modified from the protocol proposed by the manufacturer: 2 μL of PCR product, 2 μL of restriction enzyme buffer (RE), 0.2 μL of bovine serum albumin (BSA), 1 μL of enzyme solution and, finally, purified water to a final volume of 20 μL. All mixtures were incubated at 37°C for 4 h. Digest products were separated by electrophoresis in a 2.0% agarose gel at 90 V for 120 min. The sizes of the resulting DNA fragments were estimated by comparison with a commercial 1-kb DNA ladder (Invitrogen, UK). After the electrophoresis, the gel was visualised under UV light.
Results and discussion
The final concentrations of DNA extraction product were similar in the two species, but varied according to the sampled tissues (). Though it was possible to extract DNA from any of the sampled tissues, the muscle or body wall produced less clean extracts. In the particular case of the body wall, the presence of inhibitors in the extract at higher concentrations caused the frequent failure to successfully produce any PCR products. The choice of using either tissue will depend ultimately on the target species and on the available material. For example, holothurian species such as the ones used here introvert and retract the tentacles when inactive or stressed. Though it is easy to force the appearance of the tentacles by gently squeezing the oral area, the same is not possible when dealing, for example, with preserved animals (e.g. dry or frozen). And of course, when dealing with highly processed “beche-de-mer” products, in which none of the referenced structures will have survived, the traditionally used longitudinal muscle will have to be the chosen tissue.
Table I. Final concentrations of genomic DNA extraction eluted in 150 μL and A260/A280 ratios for each sampled tissue.
The direct digestion of the 16S PCR products with Sau3AI resulted in species-specific restriction patterns, as shown in . The digestion of Sau3AI, the 16S PCR product for H. mammata (lane 9), yielded one fragment, close to 552 bp in length (lanes 10–13), and for H. sanctori (lane 1) yielded two fragments, approximately 565 bp and 488 bp in length, respectively (lanes 2 and 7). The RFLP digestion produced fragments about 234 bp long for both species, but only H. mammata revealed a reasonable clear banding. These results showed that the PCR-RFLP is a suitable technique to identify the sea-cucumber species included in this work.
PCR-RFLP is one of the most widely used DNA-based methods in species identification, for its simplicity, rapidity, reproducibility, limited equipment requirements and cost-effective results (Rasmussen & Morrissey Citation2011); thus, routine and field-oriented methodologies of genetic screening and discrimination (“diagnostic kits”) are now available for a wide range of commercial marine species (e.g. Aranishi Citation2005; Stefanni et al. Citation2009; Wen et al. Citation2010; Guerao et al. Citation2011) and product authentication in food-related industries (e.g. Martinez et al. Citation2005; Rasmussen & Morrissey Citation2009; Sawicki & Klein Citation2010). Simultaneously, coupling such protocols with non-destructive DNA sampling techniques combines the advantages mentioned above with a more environmentally sensitive collection method. Though this study was restricted to two common species in the Azorean shallow waters, considering the simplicity of the methodology herein described, efforts should be made to contemplate applying it to other European commercial species.
Acknowledgements
P. Madeira was supported by FCT (the Portuguese Foundation for Science and Technology) under grant SFRH/BD/61146/2009. S. P. Ávila was funded by FCT (IF 00465/2015) and by FEDER funds through the Operational Programme for Competitiveness Factors–COMPETE, and by National Funds (FCT): UID/BIA/50027/2013 and POCI-01-0145-FEDER-006821. We gratefully acknowledge the support from the funding agency Direcção Regional da Ciência e Tecnologia (DRCT, Governo Regional dos Açores). We also want to thank Diana Catarino and colleagues from DOP for their support. We thank the associate laboratory LARSyS #9, with financial support from OE and COMPETE, through FCT and DRCT/GovAzores.
Additional information
Funding
References
- Aranishi F. 2005. Rapid PCR-RFLP method for discrimination of imported and domestic mackerel. Marine Biotechnology 7:571–575. DOI: 10.1007/s10126-004-4102-1.
- Beebee T, Rowe G. 2008. An introduction to molecular ecology. 2nd ed. New York: Oxford University Press.
- Borrero-Pérez GH, Gómez-Zurita J, González-Wangüemert M, Marcos C, Pérez-Ruzafa A. 2010. Molecular systematics of the genus Holothuria in the Mediterranean and Northeastern Atlantic and a molecular clock for the diversification of the Holothuriidae (Echinodermata: Holothuroidea). Molecular Phylogenetics and Evolution 57:899–906. DOI: 10.1016/j.ympev.2010.08.019.
- Borrero-Pérez GH, Pérez-Ruzafa A, Marcos C, González-Wangüemert M. 2009. The taxonomic status of some Atlanto-Mediterranean species in the subgenus Holothuria (Echinodermata: Holothuroidea: Holothuriidae) based on molecular evidence. Zoological Journal of the Linnean Society 157:51–69. DOI: 10.1111/zoj.2009.157.issue-1.
- García-Arrarás JE, Greenberg MJ. 2001. Visceral regeneration in holothurians. Microscopy Research and Technique 55:438–451. DOI: 10.1002/jemt.v55:6.
- González-Wangüemert M, Valente S, Henriques F, Domínguez-Godino JA, Serrão EA. 2016. Setting preliminary biometric baselines for new target sea cucumbers species of the NE Atlantic and Mediterranean fisheries. Fisheries Research 179:57–66. DOI: 10.1016/j.fishres.2016.02.008.
- Guerao G, Andree K, Froglia C, Simeó CG, Rotllant G. 2011. Identification of European species of Maja (Decapoda: Brachyura: Majidae): RFLP analyses of COI mtDNA and morphological considerations. Scientia Marina 75:129–134. DOI: 10.3989/scimar.2011.75n1.
- Kerr AM, Janies DA, Clouse RM, Samyn Y, Kuszak J, Kim J. 2005. Molecular phylogeny of coral-reef sea cucumbers (Holothuriidae: Aspidochirotida) based on 16S mitochondrial ribosomal DNA sequence. Marine Biotechnology 7:53–60. DOI: 10.1007/s10126-004-0019-y.
- Martinez I, James D, Loréal H. 2005. Application of modern analytical techniques to ensure seafood safety and authenticity. FAO Fisheries Technical Paper 455. Rome: FAO. 73 pp.
- Mezali K, Chekaba B, Zupo V, Asslah B. 2003. Comportement alimentaire de cinq especes d’holothuries aspidochirotes (Holothuroidea: Echinodermata) de la presqu’ile de Sidi-Fredj (Algerie). Bulletin de la Société zoologique de France 128:49–62.
- Pawson DL. 2007. Phylum Echinodermata. Zootaxa 1668:749–764.
- Pereira MOR. 1997. Checklist of the littoral echinoderms of the Azores. Açoreana 8:331–337.
- Purcell SW. 2010. Managing sea cucumber fisheries with an ecosystem approach. FAO Fisheries and Aquaculture Technical Paper 520. Rome: FAO. 157 pp.
- Purcell SW, Samyn Y, Conand C 2012. Commercially important sea cucumbers of the world. FAO Species Catalogue for Fishery Purposes 6. Rome: FAO. 150 pp.
- Ramón M, Lleonart J, Massutí E. 2010. Royal cucumber (Stichopus regalis) in the northwestern Mediterranean: Distribution pattern and fishery. Fisheries Research 105:21–27. DOI: 10.1016/j.fishres.2010.02.006.
- Rasmussen RS, Morrissey MT. 2009. Application of DNA-based methods to identify fish and seafood substitution on the commercial market. Comprehensive Reviews in Food Science and Food Safety 8:118–154. DOI: 10.1111/crfs.2009.8.issue-2.
- Rasmussen RS, Morrissey MT. 2011. Advances in DNA-based techniques for the detection of seafood species substitution on the commercial market. JALA: Journal of the Association for Laboratory Automation 16:308–321.
- Sawicki W, Klein D. 2010. Sea fish and shellfish authentication issues. In: Daczkowska-Kozon E, Sun Pan B, editors. Environmental effects on seafood availability, safety and quality. Boca Raton, FL: Taylor & Francis Group. pp. 275–294.
- Sicuro B, Levine J. 2012. Sea cucumber: A new candidate species for aquaculture in Mediterranean Sea. Reviews in Fisheries Science 19:299–304. DOI: 10.1080/10641262.2011.598249.
- So JJ, Uthicke S, Hamel J-F, Mercier A. 2011. Genetic population structure in a commercial marine invertebrate with long-lived lecithotrophic larvae: Cucumaria frondosa (Echinodermata: Holothuroidea). Marine Biotechnology 158:859–870.
- Stefanni S, Bettencourt R, Knutsen H, Menezes G. 2009. Rapid PCR-RFLP method for discrimination of the two Atlantic cryptic deep-sea species of scabbardfish. Molecular Ecology Resources 9:528–530. DOI: 10.1111/j.1755-0998.2008.02470.x.
- Tortonese E. 1965. Fauna D’Italia – Echinodermata. Bologna: Edizioni Calderini. pp. 422.
- Uthicke S, Byrne M, Conand C. 2010. Genetic barcoding of commercial Bêche-de-mer species (Echinodermata: Holothuroidea). Molecular Ecology Resources 10:634–646. DOI: 10.1111/j.1755-0998.2009.02826.x.
- Uthicke S, Welch D, Benzie JAH. 2004. Slow growth and lack of recovery in overfished holothurians on the Great Barrier Reef: Evidence from DNA fingerprints and repeated large-scale surveys. Conservation Biology 18:1395–1404. DOI: 10.1111/cbi.2004.18.issue-5.
- Wen J, Hu C, Zhang L, Luo P, Zhao Z, Fan S, Su T. 2010. The application of PCR–RFLP and FINS for species identification used in seacucumbers (Aspidochirotida: Stichopodidae) products from the market. Food Control 21:403–407. DOI: 10.1016/j.foodcont.2009.06.014.
- Wirtz P. 2009. Ten new records of marine invertebrates from the Azores. Arquipelago 26:45–49.