Abstract
A rapid temperature increase in the 1980-90s has been accompanied by dramatic and unprecedented changes in the biota and communities of the Ligurian Sea. This review uses existing historical series (a few of which have been purposely updated) to assess extent and consequences of such changes. A number of warm-water species, previously absent or occasional in the comparatively cold Ligurian Sea, has recently established thanks to warmer winters. Occurrence among them of invasive alien species is causing concern because of their capacity of outcompeting autochthonous species. Summer heatwaves, on the other hand, caused mass mortalities in marine organisms, some of which found refuge at depth. New marine diseases appeared, as well as other dysfunctions such as the formation of mucilage aggregates that suffocated and entangled benthic organisms. Human pressures have combined with climate change to cause phase shifts (i.e., abrupt variations in species composition and community structure) in different habitats, such as the pelagic environment, seagrass meadows, rocky reefs, and marine caves. These phase shifts implied biotic homogenization, reduction of diversity, and dominance by invasive aliens, and may be detrimental to the resilience of Ligurian Sea ecosystems. Another phase of rapid warming has possibly started in the 2010s and there are clues pointing to a further series of biological changes, but data are too scarce to date for proper assessment. Only well addressed long-term studies will help understanding the future dynamics of Ligurian Sea ecosystems and their possibilities of recovery.
Introduction
The first evidences of anthropogenic global warming date back to the 1970s (Broecker Citation1975), and the temperature increase of ocean waters is now amply documented (Lima & Wethey Citation2012). Climate change is modifying the properties of the world’s ocean at a fast rate (Gattuso et al. Citation2015), leading to unprecedented biological shifts (Beaugrand et al. Citation2019). Change threatens all components of biodiversity, from individual organisms to the biological communities providing ecosystem services (Hooper et al. Citation2005; Bellard et al. Citation2012). The climate-induced loss of biodiversity has thus become a major scientific and societal issue (Cardinale et al. Citation2012; Urban et al. Citation2016). Contrarily to a diffuse opinion in the past (Boudouresque & Bianchi Citation2013, and references therein), recent studies indicate that marine species may be more vulnerable to warming than are terrestrial ones (Richardson & Schoeman Citation2019).
The semi-enclosed nature of the Mediterranean Sea, together with its smaller inertia due to the relative short residence time of its water masses, makes it highly reactive to climate change (Durrieu de Madron et al. Citation2011), so that it ranks among the world’s regions for which the strongest warming rates are predicted (Giorgi Citation2006). The Mediterranean Sea is also a biodiversity hotspot (Bianchi & Morri Citation2000a; Coll et al. Citation2010), threatened by many anthropogenic forcings (Claudet & Fraschetti Citation2010; Lejeusne et al. Citation2010; Fraschetti et al. Citation2011). In addition to direct human impacts, climate change is driving the sensitive Mediterranean Sea ecosystems towards an unpredictable future (Turley Citation1999; Bianchi et al. Citation2012; Boero Citation2015a, Citation2015b).
The ongoing increase of water temperature is influencing the Mediterranean marine biota by a combination of direct (survival, reproduction, recruitment, etc.) and indirect effects, mediated by biotic interactions (predation, parasitism, diseases, etc) or the altered emphasis of marine current fluxes between adjacent basins (Bianchi Citation1997). Species distribution within the Mediterranean Sea is modifying in response to climate change (Gambi et al. Citation2008; Bianchi et al. Citation2013), with tropical taxa taking over communities, thus triggering - together with sea water warming - a process of “tropicalization” (Bianchi & Morri Citation2003; Bianchi Citation2007; Raitsos et al. Citation2010).
The Ligurian Sea () is one of the northerner and colder sectors of the Mediterranean Sea, and its biota is somewhat different from that of the rest of the basin, with a dearth of thermophilic species and the comparative abundance of cold temperate boreal species (Bianchi et al. Citation2012). The Ligurian Sea is also one of the most anthropized parts of the Mediterranean Sea, due to huge urban, touristic and industrial development, harbour activities and construction of artificial structures, building of coastal roads and railways, dumping and drainages, professional and recreational fisheries, and beach replenishments (Mangialajo et al. Citation2007; Misic et al. Citation2011; Parravicini et al. Citation2012, Citation2013; Prato et al. Citation2016; Cánovas-Molina et al. Citation2016a; Venturini et al. Citation2017, Citation2019; Enrichetti et al. Citation2019a, Citation2019b).
Figure 1. Geographical setting of the Ligurian Sea, with its position within the Mediterranean (inset). Localities cited in the text are indicated.
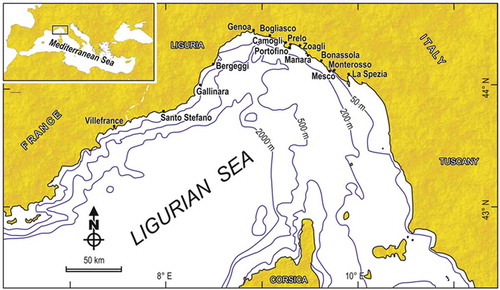
There is a wealth of studies, spanning over a century, on the Ligurian Sea ecology (Bianchi et al. Citation1987), which altogether offer a baseline to evaluate the footprint of global change on its ecosystems (Cattaneo-Vietti et al. Citation2010). It has therefore been possible to describe the modifications undergone by the biodiversity of Ligurian Sea in the last decades (Morri & Bianchi Citation2001; Cattaneo-Vietti Citation2018). There is growing evidence that a major shift in Ligurian Sea climate and ecosystem regime occurred around the 1980-90s (Gatti et al. Citation2015, Citation2017), in coincidence with similar change observed in other European seas and perhaps globally (Conversi et al. Citation2010; Reid et al. Citation2016). A proper evaluation of ecosystem change requires the availability of long-time series (Hampton et al. Citation2019), which are unfortunately rare (Bianchi & Morri Citation2004a; Boero et al. Citation2015). The Italian part of the Ligurian Sea, facing the coast of Liguria (an administrative region in NW Italy), represents a partial exception, as historical data are available for a number of taxa and habitats.
This review aims at describing the most important consequences of the 1980-90s shift on the marine biodiversity of Liguria, searching for common patterns of change through the comparative analysis of existing data series, a few of which have been purposely updated for this review using unpublished information. Some of these data series span several decades (Morri et al. Citation2001; Bavestrello et al. Citation2015; Cattaneo-Vietti et al. Citation2015; Gatti et al. Citation2015, Citation2017; Parravicini et al. Citation2015; Burgos-Juan et al. Citation2016; Betti et al. Citation2017a; Costa et al. Citation2018; Montefalcone et al. Citation2018), and a few use retrospective techniques to reconstruct trends for up the last 3000 years (Peirano et al. Citation2004; Bertolino et al. Citation2012, Citation2014a, Citation2017).
Ligurian Sea water warming
Since the second half of the 1980s, clues of Mediterranean Sea water warming came from both temperature measurements and, even more clearly, biological indicators (Bianchi & Morri Citation1993; Francour et al. Citation1994). A major climatic event has been the so-called Eastern Mediterranean Transient (EMT), which started in the mid 1980s and spanned the whole 1990s, implying important changes in water circulation (Malanotte-Rizzoli et al. Citation1999; Beuvier et al. Citation2010). The effects of the EMT had been especially observed in the Levant and Ionian seas, but the consequences extended over the adjacent basins (Manca Citation2000) to reach the Western Mediterranean (Gasparini et al. Citation2005; Schröder et al. Citation2006). Starting from the mid 1990s, hydrological changes were detected in the Ligurian Sea (Marty & Chiavérini Citation2010).
Series of water temperature data in the Ligurian Sea have been recently analysed by Parravicini et al. (Citation2015) and Bianchi et al. (Citation2018a), among others. Parravicini et al. (Citation2015) used data collected fortnightly off Villefranche-sur-Mer (43°41.10ʹ N, 7°18.94ʹ E) at 0 m, 10 m, 20 m, 30 m, 50 m and 75 m depth between January 1958 and December 2010. An increase in water temperature over the whole period was observed at all depths, but while at 75 m this increase appeared continuous and gradual, at the shallowest depths warming showed three main phases: a cooling phase between 1958 and the 1980s, a phase of rapid warming from the 1980s to the 1990s, and a phase of more gentle warming from the 1990s to 2010. The existence of the break in the 1980-90s, representing a shift between a comparatively cool and a warm period, had already been documented by studies that analysed either air temperature (Astraldi et al. Citation1995; Morri & Bianchi Citation2001) or sea surface temperature (SST) data (Lelieveld et al. Citation2002). The rapid warming phase of the 1980-90s mostly coincided with the EMT (Lejeusne et al. Citation2010). The findings of Parravicini et al. (Citation2015) were consistent with these earlier results and indicated that water warming did not concern the sea surface alone, but the entire water column down to at least 75 m depth.
Bianchi et al. (Citation2018a) examined SST data derived from NOAA satellite records at 42°54ʹ N and 9°24ʹ E (freely available at www.esrl.noaa.gov/psd/cgi-bin/data/timeseries/timeseries1.pl), calibrated with field measurements (Bianchi & Morri Citation2004b). Despite calibration, these data were considered as representative mostly of offshore conditions, whilst in shallow coastal waters air temperature data are often better climatic proxies than ocean temperature data (Morri & Bianchi Citation2001). Thus, the annual means of air temperature obtained from the Meteorological Observatory of Genoa were also used. Both air temperature and SST raised in the last decades, mean temperatures in the 2000s being up to half a degree higher than in the 1970s (Gatti et al. Citation2017). At Portofino, where detailed sea truth data are available, SST increased of 0.1°C on annual average between 2002 and 2013 (Cattaneo-Vietti et al. Citation2015). As a consequence, the surface isotherms of February (the coldest month in the year) in the Ligurian Sea moved northward (), causing warmer winters. Bianchi et al. (Citation2018a), however, observed that the rise of temperature was not regular with time and four main phases were distinguished, notwithstanding large year-to-year variability: 1) a comparatively cold phase from the late 1960s to the early 1980s; 2) a rapid warming phase in the second half of the 1980s; 3) a warmer, stabilized phase since the 1990s; 4) another apparent rapid temperature rise after 2010. The recent update to 2018 of both air temperature and SST data confirms the existence of this fourth phase, 2014 to 2018 having been the warmest years on records () and thus conforming to the expected further rapid warming over the whole Mediterranean Sea (Shaltout & Omstedt Citation2014). Numerical experiments suggest that the future Mediterranean thermohaline circulation will replicate the EMT (Adloff et al. Citation2015).
Figure 2. Multidecadal (1980–2018) trends of yearly averages of temperatures in Liguria. (a) Air temperature, measured at the meteorological observatory of Genoa university. (b) Sea surface temperature (SST), from NOAA satellite data (www.esrl.noaa.gov/psd/cgi-bin/data/timeseries/timeseries1.pl). In both graphs, the smoothed thick lines depict the 6th degree polynomial fit.
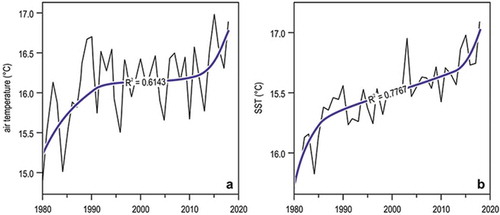
Figure 3. Surface isotherms of February in the Ligurian Sea. (a) Climatological means from the historical dataset 1906–1985. (b) Means for 1985–2006. Redrawn and modified from Bianchi et al. (Citation2012).
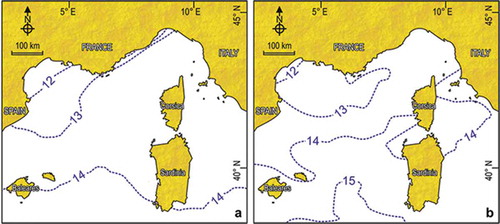
Changes in the marine biota
The biota of the Ligurian Sea has been the object of studies since the second half of the XVIII century, when the famous biologist Lazzaro Spallanzani (1729–1799) carried out observations in the Gulf of La Spezia. The well-known feather-duster worm Sabella spallanzani, described in 1791, has been dedicated to him by Johann Friedrich Gmelin (1748–1804). In the XIX century, the monumental work “Descrizione di Genova e del Genovesato”, published in 1846, contained the first organic lists of the marine fauna and flora of Liguria (Tortonese Citation1971). In the XX century, the marine biota has been studied extensively, allowing Rossi (Citation1969) to outline the zoogeography of the Ligurian Sea.
In the last decades, however, the Ligurian Sea has witnessed unprecedented modifications in the composition of its biota due to a combination of human impacts and climate change (Morri & Bianchi Citation2001; Bianchi & Morri Citation2004b; Parravicini et al. Citation2013). Three main processes can be recognized: i) the arrival and establishment of southern species; ii) mass mortalities; and iii) increased incidence of diseases and other dysfunctions (Boero Citation2011; Cattaneo-Vietti Citation2018).
Southern species
Occasional records of warm-water species, whose range was normally restricted to the Tyrrhenian Sea and other southern sectors of the Mediterranean, had been explained in the past with the occurrence of “thermophilic oases” along the Ligurian coasts (Sarà Citation1985). These alleged thermophilic oases may derive from eddies created along the coast by the current circulation in the Ligurian Sea (Casella et al. Citation2011). The comparatively stable Ligurian Circuit pushes against the coast the much more variable Tyrrhenian Current () so that in certain years lens of warm water may persist along the Ligurian coast (Astraldi et al. Citation1995; Vignudelli et al. Citation2000): here, southern organisms whose motile stages are transported by the Tyrrhenian Current (Aliani & Meloni Citation1999; Aliani & Molcard Citation2003) can settle and withstand winter conditions. Such a process explains the historical variability of southern species records in the Ligurian Sea.
Figure 4. Surface circulation in the Ligurian Sea. (a) Scheme of the main current systems, with the steady Ligurian Circuit (colder) and the inflowing Tyrrhenian Current (warmer). (b) Satellite infra-red thermal imagery (AVHRR NOAA 9): the whitest areas are the warmest.
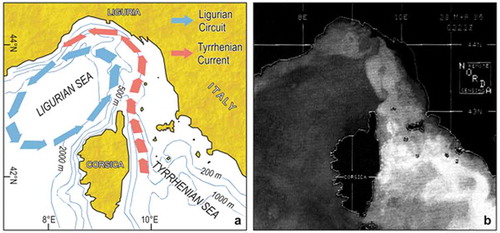
Starting from the late 1980s, records of southern species have become more frequent and numerous (Bianchi & Morri Citation1993, Citation1994a). Reduced winter cooling and increased average temperature have allowed these southern species to establish and diffuse in the Ligurian Sea (Parravicini et al. Citation2015; Bianchi et al. Citation2018a). The ornate wrasse Thalassoma pavo, for example, has been proved to reproduce in the Ligurian Sea (Vacchi et al. Citation1999, Citation2001; Sara et al. Citation2005), even if it does not reach population densities comparable to those observed in the warmer Tyrrhenian Sea (Guidetti et al. Citation2002). Several other fish species of southern origin are now to be considered as established in the Ligurian Sea, such as the zebra seabream Diplodus cervinus, the pearly razorfish Xyrichtys novacula, the Atlantic lizardfish Synodus saurus, the yellowmouth barracuda Sphyraena viridensis, the parrotfish Sparisoma cretense and the cryptobenthic Molly Miller combtooth blenny Scartella cristata (Parravicini et al. Citation2008; Bianchi et al. Citation2018a; Cattaneo-Vietti Citation2018). The same holds for a larger number of algae and invertebrates (Parravicini et al. Citation2015; Bianchi et al. Citation2018a).
All the above-mentioned southern species are native of the Mediterranean Sea. Together with them, however, came also numerous species of extra-Mediterranean origin. The arrival of these non-indigenous species (NIS) has been directly or indirectly favoured by human activities, but their successful establishment has once again been made possible by sea water warming (Bianchi et al. Citation2018a). Both the fish NIS, such as the bluespotted cornetfish Fistularia commersonii and the blunthead pufferfish Sphoeroides pachygaster (Orsi Relini Citation2010), and the invertebrate NIS, such as the calcisponge Paraleucilla magna (Bertolino et al. Citation2014b; Ulman et al. Citation2017), the four-colour nudibranch Godiva quadricolor (Betti et al. Citation2015), the sabellid polychaete Branchiomma luctuosum (Betti et al. Citation2018), and the crab Percnon gibbesi (Bianchi et al. Citation2018a), have been recorded irregularly (both in space and time) to date. On the contrary, the algal NIS, such as the rhodophytes Acrothamnion preissii, Asparagopsis armata and Womersleyella setacea, and the chlorophytes Caulerpa cylindracea and Caulerpa taxifolia (Gatti et al. Citation2015; Montefalcone et al. Citation2015; Parravicini et al. Citation2015) are causing concern because of their fast spreading and wide distribution. Fishermen have been harmed by the abundance of these algae, which clog their traps and nets, but have also involuntarily contributed to their diffusion by cleaning their fishing gears at sea. Due to its impact on native ecosystems since the mid 1980s, especially along the French tract of the Ligurian Sea, C. taxifolia gained the nickname of “killer alga” (Meinesz Citation1999). However, by 2000 its dispersal rate slowed down and the species did not persist in areas formerly colonized (Montefalcone et al. Citation2015). In contrast, C. cylindracea, first recorded in 1996 in the Ligurian Sea (Bussotti et al. Citation1996; Modena et al. Citation2000), exhibited an impressive and constant expansion of its range, becoming invasive in most coastal habitats of the Ligurian Sea, marine protected areas included (Morri et al. Citation2019).
The numbers of both native southerners and NIS have increased in recent times, but with a different trend (Bianchi et al. Citation2018a). Collating information from scuba diving records indicated that the number of the former somehow fluctuated with climate variability, whereas that of the latter increased steadily, with an apparent acceleration after the 2000s ()). Regular monitoring at Genoa between 2009 and 2015 evidenced that the number of the native southerners increased linearly, that of the NIS increased exponentially ()). The ever growing incidence of warm-water species, either native or non-indigenous, is changing the biogeography of the Ligurian Sea (Bianchi et al. Citation2013).
Figure 5. Trends in southern species occurrence in the Ligurian Sea. (a) Number of native or non-indigenous southern species recorded by diving in several localities of Liguria per 5-year period; the curves represent the second degree polynomial fits. (b) Yearly trends in the number of native or non-indigenous southern species in Genoa sites regularly monitored. The best fit is linear for native southerners and exponential for non-indigenous species. Redrawn and modified from Bianchi et al. (Citation2018a).
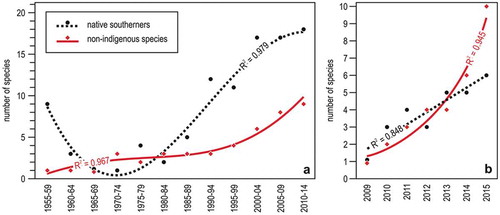
Mass mortalities
While allochthonous warm-water species made their way into the Ligurian Sea, many autochthonous benthic invertebrates suffered mass mortalities correlated with summer heatwaves (Cerrano & Bavestrello Citation2009a; Rivetti et al. Citation2014). Increased frequency of marine heatwaves (discrete periods of extreme local sea water warming) is concurrent with long-term persistent warming, and is having deleterious impacts on many species in the world’s ocean (Smale et al. Citation2019).
In the Ligurian Sea, the first documented mass mortality episodes occurred in the 1980s. The red coral Corallium rubrum and the gorgonians Eunicella cavolini and Paramuricea clavata were heavily struck (Bavestrello & Boero Citation1986; Rivoire Citation1991; Bavestrello et al. Citation1994; Harmelin & Marinopoulos Citation1994; Garrabou et al. Citation2001), as well as Spongia officinalis and other sponges (Gaino et al. Citation1992). In the second half of the 1990s, mass mortalities were observed in the bivalve Spondylus gaederopus, in the scleractinian corals Balanophyllia europaea, Cladocora caespitosa ()) and Oculina patagonica (Rodolfo-Metalpa et al. Citation2000, Citation2005) and in the sponge Petrosia ficiformis (Cerrano et al. Citation2001).
Figure 6. Examples of invertebrate mortality in the Ligurian Sea. (a) Necrosis in the sponge Spongia officinalis (Portofino, 5 m depth). (b) Partial mortality of the scleractinian coral Cladocora caespitosa (Portofino, 10 m depth). (c) Branch necrosis in the gorgonian Eunicella cavolini (Portofino, 30 m depth). (d) Empty shell, still in living position, of the bivalve Pinna nobilis killed by the pathogen Mycobacterium (Bergeggi, 15 m depth).
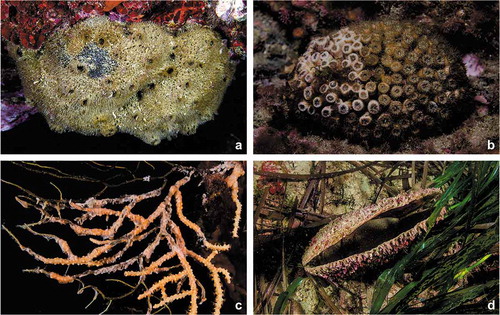
The most severe and widespread episode of mass mortality ever recorded occurred at the end of the summer 1999, and affected 27 species of sessile invertebrates including sponges, gorgonians, corals, bryozoans and ascidians, from the Tuscan Archipelago, Italy, to Marseille, France (Cerrano et al. Citation2000; Pérez et al. Citation2000; Garrabou et al. Citation2001). Quantitative data on gorgonians (Eunicella cavolini, E. singularis, Paramuricea clavata) indicated that the proportion of colonies affected ranged from 60% to 100%, which implied that millions of gorgonians died in the Ligurian Sea ()). Mortality did not similarly hit all colonies. Small colonies (<10 cm) were less affected than larger ones, and females more intensely than males, causing a shift in sex ratio in favour of males of about 3:1, in contrast with the original ratio of 1:1 (Cerrano et al. Citation2005). The mortality followed an unusual meteorological period, with a decrease of the NW winds from July to October 1999 and an anomalous increase of the sea water temperature of up to 2-5°C for several weeks, with a major peak at the end of August in surface waters and at the end of September at 32 m depth ()); the mixed layer deepened to 40 m, instead of the normal 20–25 m (Romano et al. Citation2000; Laubier Citation2001).
Figure 7. The heatwave of summer 1999 and gorgonian mortality in the Ligurian Sea. (a) Trend in sea water temperature at 0 m and 32 m depth, between August and October. Lines are weekly averages, bands the confidence limits (± 95%) obtained from the secular (1909–1987) trend calculated on Ligurian Sea records. (b) Percentage of partially (grey) and totally (black) dead colonies in three common gorgonian species. Redrawn and modified from Cerrano et al. (Citation2000).
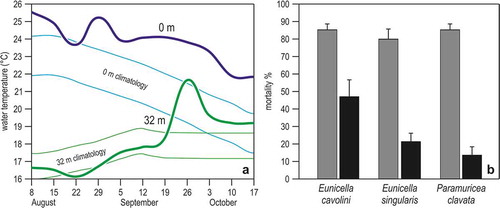
Another well documented case of mass mortality, which involved not less than 25 rocky benthic macroinvertebrates (mainly sponges and gorgonians), took place in summer 2003. The heatwave of June to September 2003 in Europe caused an anomalous warming of sea water, which reached between 1° and 3°C above the climatic values (Sparnocchia et al. Citation2006), causing mortality in up to 80% of the Ligurian Sea gorgonian colonies (Garrabou et al. Citation2009).
The effects of these catastrophic heatwaves remained evident for years. Recovery of populations was slow, with most species showing only partial recovery (Linares et al. Citation2005; Cupido et al. Citation2008; Cerrano & Bavestrello Citation2009b). Notwithstanding high recruitment rates (Sara et al. Citation2003), gorgonian populations attained only half their previous density and remained far from their original size/age structure (Cupido et al. Citation2009). The reproductive output fell dramatically (Cupido et al. Citation2012) and biomass values remained low for several years, mainly because new colonies were small and the survived ones reduced their sizes due to the breaking of necrotic branches (Cerrano et al. Citation2005). Loss or reduction of the gorgonian canopies and of the assemblages dominated by long-lived macroinvertebrates led to the dominance of turf-forming species, which in turn facilitated the proliferation and spread of the invasive alga Caulerpa cylindracea (Gatti et al. Citation2015; Verdura et al. Citation2019).
Quantitative data on the precious coral Corallium rubrum collected in 1964 (Marchetti Citation1965), 1990 and 2012 (Bavestrello et al. Citation2015) at Portofino indicated a dramatic shift in population structure. This species had been subjected to strong harvesting pressure from the 1950s to the early 1970s, but recent protection measures allowed for an increase in colony weight (from 3 g to 8 g on average). Thus, the red coral population switched from a “grass plain-like” towards a “forest-like” appearance.
The population of the bryozoan Pentapora fascialis, a large erect and perennial species, was monitored since 1997 in the Gulf of La Spezia (Cocito et al. Citation1998), thus allowing evaluating the impacts and consequences of the 1999 and 2003 warming events (Cocito & Sgorbini Citation2013). Cover declined rapidly, with larger colonies being the most affected. Deeper colonies recovered to pre-disturbance levels, while shallower colonies underwent necrosis and algal epibiosis.
Diseases and other dysfunctions
Marine disease outbreaks caused by opportunistic pathogens are increasing in frequency and severity worldwide and there is a growing body of evidence that points to links with climatic change (Harvell et al. Citation1999; Hayes et al. Citation2001; Burge et al. Citation2014; Cohen et al. Citation2018). Thermal stress may reduce the resistance of animals and plants to microrganisms (virus, bacteria, protozoans, fungi) that otherwise would have remained non virulent (Cerrano & Bavestrello Citation2009a).
No etiological agent was unequivocally identified in the mass mortalities of invertebrates observed in the Ligurian Sea, but the bacterium Vibrio coralliilyticus, originating from warmer seas, possibly played a role in the death of the gorgonian Paramuricea clavata (Martin et al. Citation2002; Bally & Garrabou Citation2007; Vezzulli et al. Citation2010). Summer mortality events of the scleractinian coral Oculina patagonica (Rodolfo-Metalpa et al. Citation2006) were demonstrated as due to the combined effect of high temperature and infection by the bacterium Vibrio shiloi (Rosenberg & Falkovitz Citation2004). The abundance of several other species of Vibrio, such as V. cholerae, V. vulnificus and V. parahaemolyticus (potentially pathogenic to humans), has been recently observed in the Ligurian Sea when the sea surface temperature exceeded 25°C (Vezzulli et al. Citation2009). Aspergillus sydowii, a fungus that has been responsible for the mass destruction of coral in the Caribbean Sea over the last 15 years, has been recently recorded in the Port of Genoa and may represent a risk for Ligurian corals, gorgonians and sponges (Greco et al. Citation2017).
A microbial infection had been invoked as the causal agent of mortality in the bivalve Spondylus gaederopus (Meinesz & Mercier Citation1983) and in the sea urchins Arbacia lixula, Paracentrotus lividus and Sphaerechinus granularis (Boudouresque et al. Citation1980). The populations of the giant pen shell Pinna nobilis living in Bergeggi and Portofino were infected in late summer 2018 by a species of Mycobacterium (Carella et al. Citation2019), with 30-100% animals dead ()). The Ligurian Sea populations of the zoanthid Parazoanthus axinellae have been suffering from a summer disease since 2000: from June to October, the polyps became covered with dense mats of the filamentous cyanobacterium Porphyrosiphon, which compromised polyp activity (Cerrano et al. Citation2006). Scuba diving observations at Bergeggi and Portofino in late summer 2018 evidenced that diseased colonies were localized above the thermocline, whereas those living below the thermocline were healthy, suggesting the combined action between microbial attack and elevated temperature.
Diseases in sponges have been studied in detail. The sponges most seriously affected were those characterized by a skeleton of spongin fibres, and a spongin fibre-perforating bacterium was hypothesized to be the causative agent of the disease (Gaino & Pronzato Citation1989; Cerrano et al. Citation2000). Generally, the disease started as small necrotic areas on the surface of the sponge ()), which increased in size until they affected the whole sponge. The progression of the disease was influenced by the growth form, with massive sponges damaged faster than the branching forms. Rates of tissue regeneration depended on the species and amount of damage. Recovery was facilitated when individual sponges were able to isolate the necrotic tissue by means of a layer of new pinacoderm between healthy and necrotic tissue, as seen in Petrosia ficiformis (Cerrano et al. Citation2001).
The list of tropical species whose range expansion in the Ligurian Sea has been favoured by climate warming also includes the toxic dinophyte Ostreopsis ovata. It produces ovatoxin-a, a form of palytoxin, one of the most toxic non-proteic molecules occurring in nature (Ciminiello et al. Citation2008). Ovatoxin-a causes disease in the marine fauna (Faimali et al. Citation2012; Privitera et al. Citation2012) and irritations by contact or cough, fever and respiratory problems by inhalation of marine aerosols in humans (Abbate et al. Citation2012, and references therein). The first massive bloom of O. ovata in the Ligurian Sea occurred in August 1998 (Abbate et al. Citation2007). Since then, blooms were reported almost every summer (with a major episode in 2005) in shallow waters during periods of warm and calm weather (Mangialajo et al. Citation2008a, Citation2010; Cohu et al. Citation2013; Bianchi et al. Citation2018a). Urbanized areas and degraded habitats have been shown to be more prone to its proliferation (Meroni et al. Citation2018).
Blooms of mucilaginous aggregates of filamentous algae have been another dysfunction linked to hot summers in the last decades. The algae producing these aggregates included both Phaeophyceae and Chrysophyceae, but in the Ligurian Sea the former were dominant, with the species Acinetospora crinita. The mucilage aggregates were able to cover wide extents of the sea floor, suffocating benthic organisms. A major mucilaginous event occurred at Portofino in summer 2003, affecting macroalgae, sponges and scleractinian corals (Schiaparelli et al. Citation2007). A possibly stronger event was observed in summer 2018. Aggregates started forming just below the summer thermocline in June ()) and arrived at covering completely the sea floor in July and August ()). A period of calm weather in August caused an anomalous warming of the surface layer (as already described by Sparnocchia et al. Citation2006), leading to the formation of a second thermocline and to the development of mucilage aggregates also in shallow water ()). Filaments sunk to deeper water (down to more than 40 m) entangled gorgonians (), causing their necrosis ()) as observed in other regions of the Western Mediterranean (Giuliani et al. Citation2005; Piazzi et al. Citation2018).
Figure 8. Phenology of the mucilage event of 2018 at Portofino. (a) Abundance of mucilage aggregated according to time and depth. Circles are roughly proportional to mucilage cover. Approximate positions of summer thermoclines are also indicated. (b) 100% cover of mucilage aggregates at about 20 m depth in July. (c) Mucilage filaments entangling the gorgonian Eunicella cavolini at about 30 m depth in August. (d) Mucilage filaments entangling the gorgonian Paramuricea clavata at about 40 m depth in September.
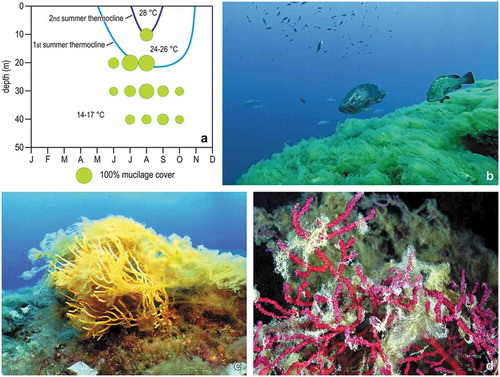
Modification of marine habitats
All marine habitats of Liguria have been thoroughly investigated during the XX century, so that it was possible to produce a comprehensive compendium in the 1980s (Cattaneo-Vietti et al. Citation1988). A comparative dearth of information on deep rocky reefs has been partially remedied in recent years (Cánovas-Molina et al. Citation2016a; Enrichetti et al. Citation2019c). However, historical data-series that encompass the climate change of the last decades are available for only four habitats: the pelagic environment; seagrass meadows; rocky reefs; and marine caves.
The pelagic environment
The disappearance of some species and the arrival of others, even of commercial interest, have characterized the pelagic fish assemblages of the Ligurian Sea in the last decades. Inter-annual variations in sea surface temperatures affected the abundance of anchovies: in 1985, 1986 and 1993, high egg productivities were observed, together with temperature trends exhibiting a fast increase of sea surface temperature in May and a reduced temperature variability during the reproductive period, June to August (Petrillo et al. Citation1998). On the contrary, in 1991, a year with low temperature increase in spring and high temperatures in the following summer, the lowest abundances of anchovy eggs were reported. Also the increased abundance of the round sardinella Sardinella aurita, a thermophilic species, could be related to temperature increase in the Ligurian Sea (Cattaneo-Vietti et al. Citation2010).
The long period of activity of a small tuna trap, the “tonnarella” of Camogli (Balestra et al. Citation1976; Cattaneo-Vietti & Bava Citation2009), allowed analysing the yearly differences in the fish catches in comparison with change in sea surface temperature (Morri & Bianchi Citation2001; Cattaneo-Vietti et al. Citation2015). A number of thermophilic species, such as the dolphinfish Coryphaena hippurus, the yellowmouth barracuda Sphyraena viridensis and the greater amberjack Seriola dumerili ()), have numerically increased, while boreal species, such as the bullet tuna Auxis rochei ()), have decreased. Large pelagic predators, such as sharks and in particular the blue shark Prionace glauca, once frequent in the Ligurian Sea, also decreased. Sharks have become increasingly rare everywhere in the Mediterranean Sea (Ferretti et al. Citation2008; Britten et al. Citation2014) due to overfishing, intense use of pelagic longlines or impoverishment of their prey, but their reduced occurrence in the Ligurian Sea has been probably also due to the noise disturbance caused by boat traffic during summer (Cattaneo-Vietti Citation2018).
Figure 9. Two examples of fish species catched in the tuna trap (“tonnarella”) of Camogli. (a) The greater amberjack Seriola dumerili has been first recorded in the 1990s. (b) The bullet tuna Auxis rochei has halved in abundance with respect to the 1950s.
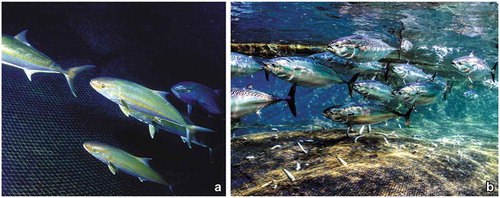
Regarding cetaceans, MacLeod (Citation2009) predicted that, at world level, the distribution of 88% of the species may be affected by changes in water temperature. In the Ligurian Sea, now included in the Pelagos Sanctuary for marine mammals (Notarbartolo Di Sciara et al. Citation2008), the striped dolphin Stenella coeruleoalba has increased in abundance, while the common dolphin Delphinus delphis has decreased in the last decades (Meliadò et al. Citation2019) but the relationships of these evidences with the variations of SST are not clear. However, a study conducted in this area (Azzellino et al. Citation2008) evidenced that fin whales and striped dolphins respond to the spatial variability of the SST and are both attracted by thermal anomalies. On the contrary, sperm whales show a lower preference for the thermal anomalies.
The abundance of jellyfish, and especially of the stinging scyphozoan Pelagia noctiluca, in the Ligurian Sea has increased starting from the 1980s, with major peaks in 1981–1985 and 1994–2008 (Bernard et al. Citation2011). Outbreaks were possibly linked to the climatic variability of the Ligurian Current, which may push P. noctiluca swarms towards the coast (Ferraris et al. Citation2012). Similarly, the strandings of the by-the-wind sailor Velella velella, known in the Ligurian Sea since at least 1923 (Pellerano Citation2016), have apparently increased in recent years (Betti et al. Citation2017b). In 2009, a bloom of the alien combjelly Mnemiopsis leidyi was recorded in the eastern Ligurian Sea, but the species apparently did not get established (Boero et al. Citation2009).
Seagrass meadows
Seagrass meadows are important coastal ecosystems in tropical and temperate seas worldwide, providing essential ecosystem services (Cullen-Unsworth et al. Citation2014). The Mediterranean endemic species Posidonia oceanica represents a most valuable example of natural capital (Vassallo et al. Citation2013) and is protected by several international initiatives and national laws, which call for its management and monitoring for conservation (Montefalcone Citation2009). Despite its importance as foundation species, its meadows are experiencing a well-documented regression in the Mediterranean due to multiple anthropogenic pressures and invasive species, worsened by the effect of climate change (Chefaoui et al. Citation2018, and references therein).
The first studies on P. oceanica meadows of Liguria date back to the beginning of the XX century (Issel Citation1918) but the first detailed maps of their distribution became available only in the mid 1990s (Bianchi & Peirano Citation1995), evidencing a generalized regressive condition of the meadows. Combining a morphodynamic model, which predicts the extent of P. oceanica meadows in absence of any human influence (Vacchi et al. Citation2014, Citation2017, and references therein), with the available historical data (Burgos-Juan et al. Citation2016; Burgos et al. Citation2017), it has been possible to estimate that more than 50% of the original surface of the meadows of Liguria has been lost under the influence of human impacts (Peirano & Bianchi Citation1997; Peirano et al. Citation2005; Montefalcone et al. Citation2006a, Citation2006b, Citation2007a, Citation2008a, Citation2008b, Citation2009, Citation2010a). A similar trend has been observed in the French part of the Ligurian Sea (Holon et al. Citation2015).
In the last decades, possibly thanks to the adoption of protection measures, regression apparently halted, with even some signs of recovery ()), as observed also in other regions (Carmen et al. Citation2019). Regular monitoring activities on the P. oceanica meadow of Monterosso-al-Mare from 1991 to 2007 (Peirano et al. Citation2001, Citation2011) and occasional surveys between 1991 and 2017 showed a sudden and lasting increase of cover and shoot density, respectively, in coincidence with the sea water warming of the 1980-90s (). The relationship between sea water warming and P. oceanica growth, however, is controversial (Montefalcone et al. Citation2013; Pergent et al. Citation2014).
Figure 10. Changes in seagrass meadows of Liguria. (a) Time trend of Posidonia oceanica meadow extent since the mid of the XIX century, combining modelling, estimations, and quantitative cartographic measurements. The curve depicts the second degree polynomial fit of the data. (b) Time trend of the proportion of Cymodocea nodosa out of the total areal extent of seagrass meadows, and of the proportion of the linear extent of artificial structures on the coastline.
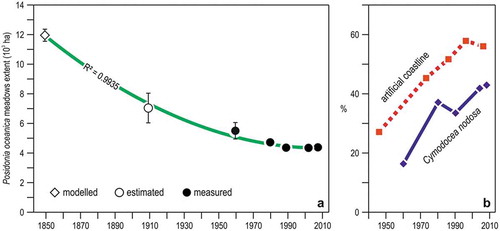
Figure 11. Trends in the Posidonia oceanica meadow at Monterosso. The curves depict the third degree polynomial fit of the data. (a) Yearly means (± se) of cover values, 1991–2006. (b) Discontinuous measures of mean shoot density (± se), 1991–2017. Data from Peirano et al. (Citation2011) and Regione Liguria (http://www.cartografiarl.regione.liguria.it/SiraQualMare/script/PubRetePuntoParam.asp?_ga=2.97453724.2042002155.1518772812-601063746.1517569932&fbclid=IwAR00qk1ShUaKXOxg0Hdi3lTtzAjMvUir-ryolOenpw9-v_yTP4wcI3wI2yk).
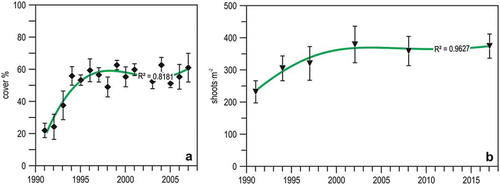
Decline of P. oceanica in Liguria has often been coupled with its replacement by the more tolerant seagrass Cymodocea nodosa (Bianchi & Peirano Citation1995; Montefalcone et al. Citation2006a; Barsanti et al. Citation2007): such a replacement might be favoured by sea water warming (Ontoria et al. Citation2019), but showed an obvious positive relationship with the increased artificialization of the coastline ()). In recent years, C. nodosa was in turn partly replaced first by the native green alga Caulerpa prolifera and then by its two alien congeneric C. taxifolia and, especially, C. cylindracea (Montefalcone et al. Citation2007c, Citation2010b, Citation2015).
The modifications underwent by the meadow of Prelo Cove in the last century represent a good example of such a series of substitutions. In the 1930s, the meadow of Prelo Cove had been severely impacted by the building of a jetty for pleasure craft (Lasagna et al. Citation2006a, Citation2006b, Citation2011). By the late 1950s, the areas of the cove’s sea floor deprived of P. oceanica were colonized by C. prolifera (Tortonese Citation1962). By 1979, C. prolifera disappeared to leave place to C. nodosa, which lasted there to at least 1986 (Morri et al. Citation1986). At this stage, the regressed meadow was therefore apparently following the secondary successional pattern envisaged by Molinier and Picard (Citation1952), suggesting the possibly of recovery in the following years. By 1991, however, the pattern was reverted: C. nodosa was not found anymore, whilst C. prolifera was back again (Tunesi & Vacchi Citation1994). Being a thermophilic species, C. prolifera had been possibly favoured by the water warming of the 1980-90s, to remain abundant till at least 2004 (Montefalcone et al. Citation2006b). Notwithstanding persisting warm-water conditions, recent surveys in the 2010s did not find C. prolifera but C. cylindracea (Montefalcone et al. Citation2015), as predicted by the phase-shift model of Montefalcone et al. (Citation2007c) and contrarily to both the successional and the warming hypotheses. The multidecal change observed in the Prelo meadow was mirrored in the modifications of the associated sponge fauna, as reconstructed through the analysis of the siliceous spicules trapped within the matte (Bertolino et al. Citation2012).
The longest data series available on a Ligurian seagrass meadow, that of Bergeggi (Montefalcone et al. Citation2007b; Oprandi et al. Citation2014a, Citation2014b), illustrates well the present dualism of the health state of Ligurian P. oceanica meadows: on the one hand, the meadow has been recovering (in term of areal extent) since 2009 ()); on the other hand, it has been suffering from substitution by C. cylindracea, which is deviating the recovery trajectory of the ecosystem towards a different basin of attraction ()). Thus, information collected to date indicates hysteresis, rather than resilience (Montefalcone et al. Citation2011). Similarly, the resilience of seagrass ecosystems worldwide is becoming compromised by a range of local to global stressors (Unsworth et al. Citation2015).
Figure 12. Changes in the Posidonia oceanica meadow of Bergeggi in 25 years. (a) Position of the meadow upper limit with respect to 1987. (b) Time trajectory of the meadow in the MDS ordination plane; pie diagrams illustrate the quantitative meadow composition. Redrawn and updated from Oprandi et al. (Citation2014a, Citation2014b).
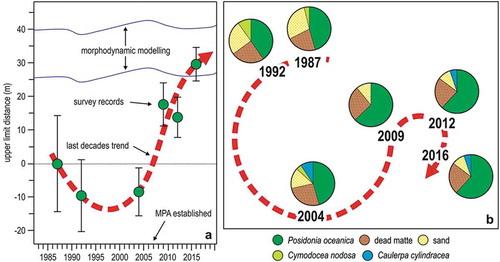
Rocky reefs
Rocky reefs are a quantitatively trivial fraction of the marine environment but hold a great scientific and economic importance (Bianchi et al. Citation2004), as they represent a major reservoir of marine biodiversity and provide ecosystem services especially for fishery and tourism (Sala et al. Citation2012; Paoli et al. Citation2017). It is not a coincidence that virtually all the Mediterranean marine protected areas are established in correspondence with rocky coasts (Sala et al. Citation2002). Reefs are listed in the European Habitats Directive among the marine habitats of interest (Thibaut et al. Citation2017) and are defined, according to the European Nature Information System (EUNIS, http://eunis.eea.europa.eu), as “submarine, or exposed at low tide, rocky substrates and biogenic concretions, which arise from the sea floor in the sublittoral zone but may extend into the littoral zone where there is an uninterrupted zonation of plant and animal communities. These reefs generally support a zonation of benthic communities of algae and animal species including concretions, encrustations and corallogenic concretions”.
The coasts bordering the Ligurian Sea are rocky for the most part, and many studies exist on the benthic communities thriving there, especially in the sublittoral (Cánovas-Molina et al. Citation2016b). On the contrary, and notwithstanding easier access, littoral communities have received little and discontinuous attention (Morri et al. Citation1986, and references therein). Betti et al. (Citation2017a) reconstructed a 59-year-long time series of occurrence of the hydroid Paracoryne huvei, an encrusting species typical of the intertidal zone, where it may cover wide expanses in late winter and early spring (February to April). Once considered rare (Boero Citation1984), P. huvei has recently increased its abundance and habitat occupancy. This stenothermal species possibly took advantage of the present phase of reduced winter cooling; the recent slow but progressive improvement of water quality (Asnaghi et al. Citation2009; De La Fuente et al. Citation2018) might also have played a role.
Sublittoral communities have been more extensively studied since the dawn of scuba diving, the Ligurian Sea having been the cradle of two major pioneer studies (Bianchi & Morri Citation2000b). Enrico Tortonese undertook fundamental research on the benthos of Portofino reefs in the late 1950s, using descriptions and samples taken by the divers of the Centro Subacqueo of Genova-Nervi, directed by Duilio Marcante (Tortonese Citation1958, Citation1962). Lucia Rossi did the same a few years later at Punta Mesco, describing the subtidal reef communities on the basis of still-frame underwater photographs taken by the diver Gianni Roghi (Rossi Citation1965). The sites studied by Tortonese-Marcante and Rossi-Roghi were revisited in the following decades, so that long-term series have become available for both Portofino and Mesco.
Gatti et al. (Citation2017) collated all the available information on Portofino reefs, starting from the pioneer studies of Tortonese, to build a large dataset of descriptive and semi-quantitative data, which allowed exploring the evolution of the rocky reef sessile assemblages across nearly 60 years. A major change of community composition was evidenced in the 1980-90s, under the combined effects of climate warming and increased human pressures, especially diving tourism (Lucrezi et al. Citation2017). Some species disappeared or got rarer, many found refuge at depth, and among the newcomers there were recently introduced alien species. The general trend included the loss of canopy-forming algae (Mangialajo et al. Citation2008b) in favour of algal turfs ().
Figure 13. Change in the rocky reef communities of Portofino at about 10 m depth. (a) Sargassum vulgare and Dictyopteris polypodioides canopy in 1981. (b) Sarpa salpa grazing in a turf-dominated environment in 2009. Modified from Parravicini et al. (Citation2013).
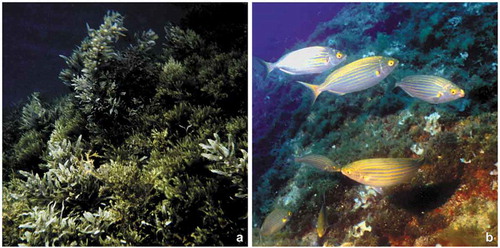
A detailed study on hydroids, first collected in 1980 (Boero & Fresi Citation1986) and then resampled in 2004 (Puce et al. Citation2009) and 2018 (Betti et al. Citation2019) with the same technique and in the same stations, evidenced changes in species composition (due to the appearance of southern species), seasonality, and depth distribution. Species richness, in particular, increased from Spring to Autumn in 1980 but exhibited the opposite trend in 2004, and remained low all year round in 2018 ()). Corydendrium parasiticum, a conspicuous species with warm-water affinity, was first recorded in 2018 in shallow water ()).
Figure 14. Change in the hydroid assemblage of Portofino reef. (a) Monthly variations in the total number of species in three years. (b) Corydendrium parasiticum, a species first recorded in 2018 in shallow waters (<5 m).
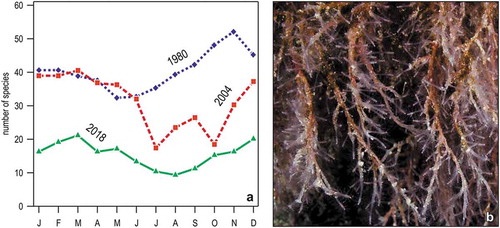
The comparison of quantitative data (still-frame underwater photographs) collected in 1987–1988 and 2012–2013 on the whole sessile community confirmed the severe depletion of macroalgal cover, while the total cover of sponges remained almost unvaried notwithstanding species substitutions (Bertolino et al. Citation2016). Photographs taken in 2002–2003 (i.e., few years after the establishment of the Marine Protected Area in 1999) and in 2013 (Betti et al. Citation2017c; Longobardi et al. Citation2017) illustrated a continued trend of modifications: frondose macroalgae (such as species of Cystoseira and Sargassum) decreased further, resulting in a significant reduction of habitat complexity and three-dimensional structure, which might affect in turn the small associate fauna (Losi et al. Citation2018; De La Fuente et al. Citation2019; Marchini et al. Citation2019). Further changes included the increased abundance of the bryozoan Bugula fulva; the decreased abundance of the barnacle Perforatus perforatus and the mussel Mytilus galloprovincialis; and the appearance of alien species (Asparagopsis armata, Caulerpa cylindracea and Paraleucilla magna).
Comparison of still-frame underwater photographs (Peirano & Sassarini Citation1992; Peirano et al. Citation2000; Bianchi et al. Citation2001; Roghi et al. Citation2010) was also used to explore change in the communities of the rocky shoal of Punta Mesco. These communities remained qualitatively and quantitatively stable from 1961 to 1990. The same result was obtained by the analysis of descriptive studies since 1937, which suggested that there had been no major modification for more than 50 years (Gatti et al. Citation2015, and references therein). Between 1990 and 1996, the communities changed dramatically, to remain subsequently stable in a new configuration to 2017. The communities passed from highly structured assemblages with a dense canopy of the gorgonian Paramuricea clavata, an understory dominated by the long-lived calcified bryozoan Cellaria fistulosa and a basal layer of encrusting corallines, such as Lithophyllum stictiforme, to a homogenized community with a reduced canopy, an understory with large hydroids and a basal layer of filamentous algae dominated by the alien species Womersleyella setacea and Caulerpa cylindracea (). Reduced canopy and scarcer calcified organisms implied loss of structural complexity, with likely consequences on the whole associate biota and ecosystem services. The two alternative states showed comparatively stable for decades (1961–1990 and 1996–2017, respectively), while the transition between them has been abrupt (1990–1996) and marked by the quantitative exuberance of a number of species that acted as “passengers” of change. Boudouresque (Citation1970) described as “Riou effect” (from the name of an island near Marseilles, France) the outburst of generalist species at the transition between two distinct species assemblages along a spatial gradient. What has been observed at Punta Mesco was apparently a Riou effect along a temporal gradient. While the drop in similarity was rapid, requiring only six years, homogenization increased continuously and gradually (Gatti et al. Citation2015).
Figure 15. Compositional change in the rocky reef community of Mesco Shoal. Others include species whose cover has always been <5%.
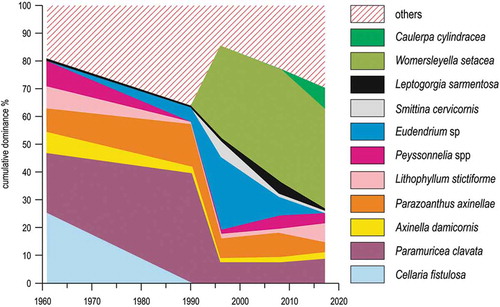
A 25-year-long data series from Gallinara Island (1991–2016) evidenced a reduction in the three-dimensional structure of the epibenthic communities and a severe decrease in species diversity (Bianchi et al. Citation2018b). Beside biodiversity loss, the most impressive outcome was biotic homogenization: deep (23–35 m) and shallow (2–9 m) communities were clearly different in 1991, but merged into an indistinct unique assemblage in 2009 and 2016 (). In this case, the modifications observed have been attributed mostly to local human impacts rather than to sea water warming, the sea floor having been littered with derelict fishing gear and other waste from boats.
Figure 16. Ordination plot on the first two axes from Correspondence Analysis of epibenthic species cover data (82 species × 78 sampling quadrats) of Gallinara Island, in 1991, 2009, and 2016. Variance explained: 47.0%. All diagrams are in the same plane and have been split for ease of reading. Station-point centroids are identified by their depth (in meters). Lines illustrate the depth gradient.
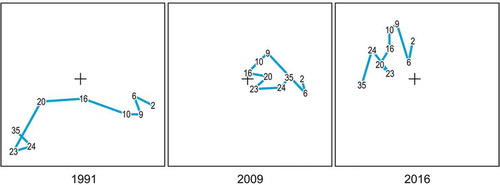
There were striking parallelisms in the biotic changes observed at Portofino, Punta Mesco and Gallinara Island. Based on the quantitative information available, species were classified as “losers” if they decreased their percent cover or disappeared over time, and as “winners” if they conversely increased or newly appeared (). The losers included the algae Cystoseira spp, Flabellia petiolata and Sargassum vulgare, the sponge Axinella damicornis, the cnidarian Alcyonium coralloides, and the bryozoan Cellaria fistulosa; some species (such as the sponges Agelas oroides and Petrosia ficiformis and the cnidarians Eunicella singularis and Parazoanthus axinellae) recovered, at least partially. The winners were represented only by the alien algae Caulerpa cylindracea and Womersleyella setacea, thus conforming to the paradigm that a few winners will replace many losers in a frame of biotic homogenization (McKinney & Lockwood Citation1999; but see Dornelas et al. Citation2019). Among the losers, stenothermal but eurybathic species found refuge at depth, to avoid the increased temperature variability in shallow waters (Morri et al. Citation2017): so did, for instance, the brown alga Dictyopteris polypodioides, the sponge Spongia officinalis, the bivalve Pinna nobilis, the bryozoan Reteporella grimaldii, and the ascidians Halocynthia papillosa and Microcosmus sabatieri at Portofino (). A. coralloides, disappeared from scuba depths (), is still found at 70–150 m (Bo et al. Citation2014). The same might be true for the small-spotted catshark Scyliorhinus canicula: although quantitative data are not available, it was frequently observed at scuba depths in the 1970s and its egg cases attached to gorgonian branches were a common sight; by the 1990s, it disappeared from scuba depths but was still found, although rarer, in deeper waters (Ferretti et al. Citation2005). Studies on larval dispersal of the gorgonian Paramuricea clavata in the Eastern Ligurian Sea (Pilczynska et al. Citation2016) detected larval exchange between different depth zones, supporting the hypothesis that deep water colonies unaffected by the surface heatwaves may provide larvae for shallow waters, thus helping population recovery.
Figure 17. Selected examples of epibenthic species that changed their mean cover (± se) with time in Ligurian rocky reefs. Note logarithmic scale on y axis.
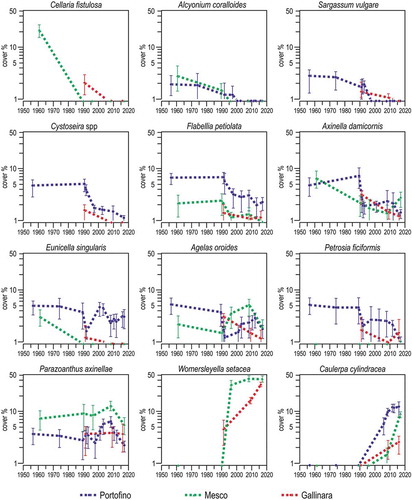
Figure 18. Selected examples of species that changed their depth range preference (mean ± se), going deeper with time, in Portofino rocky reefs.
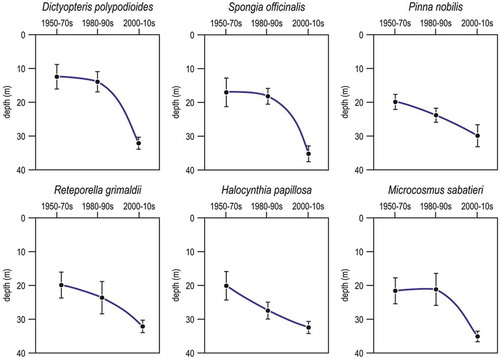
Bertolino et al. (Citation2014a, Citation2017) studied the dynamics of a sponge assemblage at decennial and millennial temporal scales analysing the siliceous spicules entrapped in the biogenic concretions of coralligenous reefs at Santo Stefano, Bogliasco, Portofino and Punta Manara. Notwithstanding changes in diversity and abundance that coincided with Holocene climate variations, the sponge assemblage remained rather stable during 3000 years. On the contrary, between 1973 and 2014 species richness decreased of about 45% on average. The decrease involved mainly massive and erect species, while encrusting, boring and cavity-dwelling species were less affected.
Marine caves
Marine caves are unique and vulnerable habitats with high aesthetic value (Rovere et al. Citation2011). The cave communities of the Mediterranean Sea are rich in biological peculiarities (including specialized organisms and deep-sea species), are poorly resilient, and act as refuges for ancestral forms (Cattaneo & Pastorino Citation1974; Bianchi et al. Citation1996). Their scientific interest made marine caves to be listed in the EU Habitats Directive and the Barcelona Convention, and calls for study and monitoring (Rastorgueff et al. Citation2015). After more than five decades of research, however, knowledge on marine caves remains fragmentary (Cicogna et al. Citation2003).
About 76 marine caves are known to exist along the Ligurian coast (Canessa et al. Citation2014), but information on their biological communities is available for only a dozen of them (Bianchi & Morri Citation1994b; Ugolini et al. Citation2003; Nepote et al. Citation2017). The sponge fauna of two semi-submerged cavities at Bonassola and Zoagli were first studied in 1961–63 (Sarà Citation1964) and again in 2015–16 (Costa et al. Citation2018). A still undetermined species of Aplysina, previously absent from the caves, was found common in the intertidal zone. The comparative analysis also evidenced an increase in species richness ()) and a significant change of growth forms ()), massive sponges having been replaced by encrusting forms.
Figure 19. Change with time in the communities of Ligurian marine caves. (a) Number of sponge species in the two semi-submerged caves of Bonassola and Zoagli in 1964 and 2016. (b) Percent ratio of massive to encrusting sponge species in the same caves and in the same years. (c) Trends of average (± se) Bray-Curtis similarity among the sessile communities of the Bergeggi marine cave from 1986–2013, using morpho-functional descriptors. (a) and (b) from data in Costa et al. (Citation2018), (c) redrawn and modified from Montefalcone et al. (Citation2018).
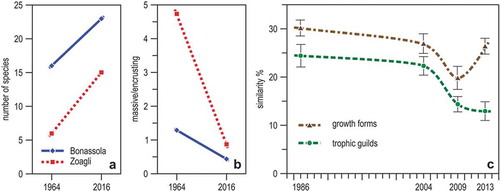
Similar results had been obtained by Parravicini et al. (Citation2010), who compared the sessile assemblages of the marine cave of Bergeggi in 1986 and 2004, i.e. before and after the summer heatwaves of 1999 and 2003: these positive thermal anomalies (with peaks up to 4°C above the climatological mean) were thought to have selectively killed erect and massive organisms (such as the sponge Petrosia ficiformis) and caused their replacement by encrusting organisms.
The marine cave of Bergeggi is unique in the Ligurian Sea – and perhaps in the whole Mediterranean – as it has been studied more or less regularly since the late 1970s (Bianchi et al. Citation1986; Sgorbini et al. Citation1988; Morri et al. Citation1994). Increased sea water temperature induced the substitution of the warm-water mysid Hemimysis margalefi for the cold-water species H. speluncola in the marine cave of Bergeggi, as well in other marine caves of the north-western Mediterranean (Chevaldonné & Lejeusne Citation2003). Such a species shift is likely to exert great influence on the energy budget of cave ecosystems (Bianchi Citation2007). Mysids stay in caves during the day but move outside at night to feed. In so doing, they import organic matter from outside into the oligotrophic cave ecosystem, but H. speluncola typically forms huge swarms while H. margalefi small groups. Thanks to the availability of a 30-year-long series of quantitative data (substratum cover from wire-frame photographs) on the sessile communities of the marine cave of Bergeggi, Montefalcone et al. (Citation2018) evaluated change in both the structural (growth forms) and functional (feeding guilds) aspects of the cave ecosystem. The most important rate of change was observed after the climate events mentioned above and coincided with major beach nourishments and the extension of a neighbouring commercial harbour. Structural aspects, but not functional ones, have been showing some recovery in recent years ()).
Concluding remarks
The present review of historical and recent data on climate and marine biodiversity of the Ligurian Sea allowed novel understanding about the nature, magnitude and direction of change in recent decades. The sea water temperature increase has been accompanied by an impressive change in the marine biota, and especially by the establishment of warm-water species of various origins and by episodes of mass mortality of sessile organisms. The most dramatic changes occurred around the 1980-90s, in correspondence with a phase of more rapid warming. Another phase of rapid warming has possibly started in the 2010s and there are clues pointing to a further series of biological changes, as already observed in other warm-temperate regions of the globe (Caputi et al. Citation2019); Ligurian Sea data, however, are too scarce to date for proper assessment.
The effects of climate change on Mediterranean marine ecosystems are multifarious (Calvo et al. Citation2011). Beside the establishment of southern species (aliens included), the most commonly effects observed in Liguria include native species finding refugia at depth, to avoid the heatwaves of shallow waters (Sparnocchia et al. Citation2006), and the biotic homogenization of the communities. Both phenomena have been observed in other Mediterranean regions and elsewhere (Bianchi et al. Citation2014; Assis et al. Citation2016; Morri et al. Citation2017). The availability of a number of historical data series allowed describing trajectories of change that have the 1980-90s as a turning point. An ecosystem shift in the same years has been recorded in other regions of the world’s ocean (Conversi et al. Citation2010; Defriecz et al. Citation2016; Reid et al. Citation2016). Linking global to regional trends is a basic step for understanding the ecological impacts of marine climate change (Popova et al. Citation2016).
Perhaps coincidentally, major changes in both climate and human pressures took place in roughly the same period (Parravicini et al. Citation2013; Gatti et al. Citation2015, Citation2017). Global climate warming and local human pressures are known to combine their effects on the marine biota (Bianchi et al. Citation2014). Local anthropogenic stressors, such as habitat degradation or destruction, pollution, sedimentation, and overfishing, together with a gradual history of changes in environmental conditions (climate, habitat quality, resource availability), can potentially exacerbate biodiversity decline and habitat loss, thus eroding the ability of ecosystems to absorb and recover from additive or synergic affectors (Montefalcone et al. Citation2011). Local and global pressures may interact in a complex way (Bianchi Citation1997), favouring, for example, the biological invasion by alien species in habitats already stressed by warming and human activities (Occhipinti-Ambrogi & Savini Citation2003; Montefalcone et al. Citation2010b). Distinguishing the modifications caused by climate change from those caused by local human disturbance is often difficult (Morri & Bianchi Citation2001). For instance, the loss of habitat-forming macroalgae (e.g., species of Cystoseira and Sargassum) observed in the Ligurian Sea (Mangialajo et al. Citation2008b; Gatti et al. Citation2017) – as well as in other regions of the Mediterranean Sea (Thibaut et al. Citation2005; Bianchi et al. Citation2014; Blanfuné et al. Citation2016; Capdevila et al. Citation2019; Catra et al. Citation2019) – has been considered by different authors as due to either climate or human impact. Similarly, the reduction of gorgonian canopies caused by sea water warming might have been enhanced by the impact of fishing, and in particular by the lost fishing gears that entangle their colonies (Bavestrello et al. Citation1997).
Mass mortalities caused by heatwaves may be equated to pulse disturbances, whereas most human pressures (e.g., pollution, turbidity) may be assimilated to a continuous stress. Despite the conceptual and practical difficulty in differentiating between disturbance and stress, the two terms, which are often used interchangeably by different authors, bear different ecological meanings: both are external agents, but the former causes subtraction of biomass, the latter reduces productivity (Montefalcone et al. Citation2011). Montefalcone et al. (Citation2017) devised two distinct indices to differentiate between the effects of disturbance and stress on marine ecosystems. Applying the two indices to a Ligurian coralligenous reef suggested that recovery following a disturbance may be rapid, while the effects of anthropogenic stress are more persistent.
The combined action of sea water warming and increased human pressure led to a phase shift in most Ligurian Sea habitats, with abrupt changes in species composition and community structure. A phase shift normally results from a regime shift, i.e., a large-scale and long-lasting change in the nature, intensity, and/or frequency of the factors that govern the dynamics of the ecosystem (Montefalcone et al. Citation2011; van Putten et al. Citation2019). A regime shift has been documented since the 1980s, when the whole Mediterranean Sea underwent a major change that encompassed atmospheric, hydrological, and ecological systems (Conversi et al. Citation2010). The phase shifts observed in Ligurian Sea communities suggest a link between basin-wide and local changes in climate and direct human pressures, to which added the absolute novelty of the invasion by alien species. Stressed ecosystems are said to be more prone to the establishment of alien invasive species (MacDougall & Turkington Citation2005). Womersleyella setacea and Caulerpa cylindracea, two of the “worst invasive species” in the Mediterranean Sea (Zenetos et al. Citation2005), were first recorded in the Ligurian Sea in the 1990s (Airoldi et al. Citation1995; Bussotti et al. Citation1996), and have recently become dominant in different habitats. The success of both invaders is favoured by sea water warming and coastal anthropization (Bianchi et al. Citation2018a). Urbanization has been proved to increase sediment delivery to Ligurian coastal waters (Mateos-Molina et al. Citation2015), and the filamentous turf formed by W. setacea is known to be favoured by high sedimentation rates (Airoldi et al. Citation1995). In turn, turf retains sediment and facilitates colonisation by C. cylindracea (Bulleri & Benedetti-Cecchi Citation2008). Such a cascade of facilitation effects likely depicts a paroxysmal positive feedback that drove the Ligurian Sea communities into an alternative state. Although some recovery from the warming-induced mass mortalities of the 1990s has been recorded, the habitat alteration caused by the invasion of the alien species leaves little scope for a reversal of the phase shift experienced by Ligurian Sea communities. Persistence of the phase shift may be detrimental to the resilience of Ligurian Sea ecosystems. Protection measures might help the return of the species that used to characterize the Ligurian Sea communities in historical times; however, they will hardly eradicate the aliens (Montefalcone et al. Citation2010b). Current management efforts to maintain or reach “good ecological status” (GES) for marine ecosystems (Boero Citation2014) should therefore consider that future marine habitats will probably lose their original peculiarities and acquire a different and unprecedented configuration partly shaped by alien species (Bianchi Citation2007). Only properly addressed long-term studies (Hampton et al. Citation2019) will help understanding the future dynamics of Ligurian Sea ecosystems and their possibility of recovery.
Acknowledgements
Change of marine climate and ecosystems was the core topic of the national research project SINAPSI (Seasonal, INterannual and decAdal variability of the atmosPhere, oceanS and related marIne ecosystems), and fell under the frame of the project ‘The impacts of biological invasions and climate change on the biodiversity of the Mediterranean Sea’, under the aegis of the Italian Ministry of the Environment. Valter Capicchioni (Genoa) communicated the air temperature data from the Meteorological Observatory of Genoa. Giampietro Gasparini (La Spezia) gave information and bibliography about water exchange between Tyrrhenian and Ligurian seas, and put at our disposal the satellite infra-red thermal imagery AVHRR NOAA 9. Vincenzo Paolillo (Genova) provided the image of Seriola dumerili in the tuna trap of Camogli. Dario Cazzella (Piacenza), Claudio De Angelis and Pino Galletta (Genova) provided information and/or images on the mucilage event of summer 2018.
Disclosure statement
No potential conflict of interest was reported by the authors.
References
- Abbate M, Bordone A, Cerrati G, Di Festa T, Melchiorre N, Pastorelli AM, Peirano A, Petruzzelli MR, Ungaro N. 2012. A new method for sampling potentially toxic benthic dinoflagellates. Cryptogamie Algologie 33:165–170. DOI: 10.7872/crya.v33.iss2.2011.165.
- Abbate M, Bordone A, Cerrati G, Lisca A, Peirano A. 2007. Differences in occurrences and density of Ostreopsis ovata in the Gulf of La Spezia (NW Mediterranean). Biologia Marina Mediterranea 14:286–287.
- Adloff F, Somot S, Sevault F, Jordà G, Aznar R, Déqué M, Herrmann M, Marcos M, Dubois C, Padorno E, Alvarez-Fanjul E, Damià G. 2015. Mediterranean Sea response to climate change in an ensemble of twenty first century scenarios. Climate Dynamics 45:2775–2802. DOI: 10.1007/s00382-015-2507-3.
- Airoldi L, Rindi F, Cinelli F. 1995. Structure, seasonal dynamics and reproductive phenology of a filamentous turf assemblage on a sediment influenced, rocky subtidal shore. Botanica Marina 38:227–273. DOI: 10.1515/botm.1995.38.1-6.227.
- Aliani S, Meloni R. 1999. Dispersal strategies of benthic species and water current variability in the Corsica Channel (Western Mediterranean). Scientia Marina 63:137–145. DOI: 10.3989/scimar.1999.63n2.
- Aliani S, Molcard A. 2003. Hitch-hiking on floating marine debris: Macrobenthic species in the Western Mediterranean Sea. Hydrobiologia 503:59–67. DOI: 10.1023/B:HYDR.0000008480.95045.26.
- Asnaghi V, Chiantore M, Bertolotto RM, Parravicini V, Cattaneo-Vietti R, Gaino F, Moretto P, Privitera D, Mangialajo L. 2009. Implementation of the European water framework directive: Natural variability associated with the CARLIT method on the rocky shores of the Ligurian Sea (Italy). Marine Ecology 30:505–513. DOI: 10.1111/j.1439-0485.2009.00346.x.
- Assis J, Castilho Coelho N, Lamy T, Valero M, Alberto F, Serrão EA. 2016. Deep reefs are climatic refugia for genetic diversity of marine forests. Journal of Biogeography 43:833–844. DOI: 10.1111/jbi.12677.
- Astraldi M, Bianchi CN, Gasparini GP, Morri C. 1995. Climatic fluctuations, current variability and marine species distribution: A case study in the Ligurian Sea (north-west Mediterranean). Oceanologica Acta 18:139–149.
- Azzellino A, Gaspari SA, Airoldi S, Lanfredi C. 2008. Biological consequences of global warming: Does sea surface temperature affect cetacean distribution in the western Ligurian Sea? Journal of the Marine Biological Association of the United Kingdom 88:1145–1152. DOI: 10.1017/S0025315408000751.
- Balestra V, Boero F, Carli A. 1976. Andamento del pescato della tonnarella di Camogli dal 1950 al 1974. Valutazioni bio-statistiche. Bollettino Pesca Piscicoltura Idrobiologia 31:105–115.
- Bally M, Garrabou J. 2007. Thermodependent bacterial pathogens and mass mortalities in temperate benthic communities: A new case of emerging disease linked to climate change. Global Change Biology 13:2078–2088. DOI: 10.1111/gcb.2007.13.issue-10.
- Barsanti M, Delbono I, Ferretti O, Peirano A, Bianchi CN, Morri C. 2007. Measuring change of Mediterranean coastal biodiversity: Diachronic mapping of the meadow of the seagrass Cymodocea nodosa (Ucria) Ascherson in the Gulf of Tigullio (Ligurian Sea, NW Mediterranean). Hydrobiologia 580:35–41. DOI: 10.1007/s10750-006-0467-7.
- Bavestrello G, Bertone S, Cattaneo-Vietti R, Cerrano C, Gaino E, Zanzi D. 1994. Mass mortality of Paramuricea clavata (Anthozoa, Cnidaria) on Portofino promontory cliffs, Ligurian Sea, Mediterranean Sea. Marine Life 4:15–19.
- Bavestrello G, Bo M, Bertolino M, Betti F, Cattaneo-Vietti R. 2015. Long-term comparison of structure and dynamics of the red coral metapopulation of the Portofino Promontory (Ligurian Sea): A case-study for a Marine protected area in the Mediterranean Sea. Marine Ecology 36:1354–1363. DOI: 10.1111/maec.12235.
- Bavestrello G, Boero F. 1986. Necrosi e rigenerazione in Eunicella cavolinii (Anthozoa, Cnidaria) in Mar Ligure. Bollettino dei Musei e degli Istituti Biologici dell’Università di Genova 52:295–300.
- Bavestrello G, Cerrano C, Zanzi D, Cattaneo-Vietti R. 1997. Damage by fishing activities to the gorgonian coral Paramuricea clavata in the Ligurian Sea. Aquatic Conservation: Marine and Freshwater Ecosystems 7:253–262. DOI: 10.1002/(ISSN)1099-0755.
- Beaugrand G, Conversi A, Atkinson A, Cloern J, Chiba S, Fonda-Umani S, Kirby RR, Greene CH, Goberville E, Otto SA, Reid PC, Stemmann L, Edwards M. 2019. Prediction of unprecedented biological shifts in the global ocean. Nature Climate Change 9:237–243. DOI: 10.1038/s41558-019-0420-1.
- Bellard C, Bertelsmeier C, Leadley P, Thuiller W, Courchamp F. 2012. Impacts of climate change on the future of biodiversity. Ecology Letters 15:365–377. DOI: 10.1111/j.1461-0248.2011.01736.x.
- Bernard P, Berline L, Gorsky G. 2011. Long term (1981-2008) monitoring of the jellyfish Pelagia noctiluca (Cnidaria, Scyphozoa) on the French Mediterranean coasts. Journal of Oceanography Research and Data 4:1–10.
- Bertolino M, Betti F, Bo M, Cattaneo-Vietti R, Pansini M, Romero J, Bavestrello G. 2016. Changes and stability of a Mediterranean hard bottom benthic community over 25 years. Journal of the Marine Biological Association of the United Kingdom 96:341–350. DOI: 10.1017/S0025315415001186.
- Bertolino M, Calcinai B, Cappellacci S, Cerrano C, Lafratta A, Pansini M, Penna A, Bavestrello G. 2012. Posidonia oceanica meadows as sponge spicule traps. Italian Journal of Zoology 79:231–238. DOI: 10.1080/11250003.2011.614641.
- Bertolino M, Calcinai B, Cattaneo-Vietti R, Cerrano C, Lafratta A, Pansini M, Pica D, Bavestrello G. 2014a. Stability of the sponge assemblage of Mediterranean coralligenous concretions along a millennial time span. Marine Ecology 35:149–158. DOI: 10.1111/maec.2014.35.issue-2.
- Bertolino M, Costa G, Carella M, Cattaneo-Vietti R, Cerrano C, Pansini M, Quarta G, Calcagnile L, Bavestrello G. 2017. The dynamics of a Mediterranean coralligenous sponge assemblage at decennial and millennial temporal scales. PloS One 12:e0177945. DOI: 10.1371/journal.pone.0177945.
- Bertolino M, Longo C, Marra MV, Corriero G, Pansini M. 2014b. Paraleucilla magna Klautau et al., 2004 (Porifera, Calcarea), an alien species extending its range in the Mediterranean Sea. Biologia Marina Mediterranea 21:109–110.
- Betti F, Bavestrello G, Bianchi CN, Morri C, Righetti E, Bava S, Bo M. 2017a. Long-term life cycle and massive blooms of the intertidal hydroid Paracoryne huvei in the North-western Mediterranean Sea. Marine Biology Research 13:538–550. DOI: 10.1080/17451000.2016.1240874.
- Betti F, Bavestrello G, Bo M, Asnaghi V, Chiantore M, Bava S, Cattaneo-Vietti R. 2017c. Over 10 years of variation in Mediterranean reef benthic communities. Marine Ecology 38:e12439. DOI: 10.1111/maec.2017.38.issue-3.
- Betti F, Bavestrello G, Bo M, Coppari M, Enrichetti F, Manuele M, Cattaneo-Vietti R. 2017b. Exceptional strandings of the purple snail Janthina pallida Thompson, 1840 (Gastropoda: Epitoniidae) and first record of an alien goose barnacle along the Ligurian coast (western Mediterranean Sea). The European Zoological Journal 84:488–495. DOI: 10.1080/24750263.2017.1379562.
- Betti F, Bo M, Bava S, Faimali M, Bavestrello G. 2018. Shallow-water sea fans: The exceptional assemblage of Leptogorgia sarmentosa (Anthozoa: Gorgoniidae) in the Genoa harbour (Ligurian Sea). The European Zoological Journal 85:290–298. DOI: 10.1080/24750263.2018.1494219.
- Betti F, Bo M, Enrichetti F, Bavestrello G. 2019. Idrozoi, sentinelle dei cambiamenti climatici sulle scogliere mediterranee. 50° Congresso della Società Italiana di Biologia Marina, 10-14 giugno 2019, Livorno. Volume pre-print. pp. 192–194.
- Betti F, Cattaneo-Vietti R, Bava S. 2015. Northernmost record of Godiva quadricolor (Gastropoda: Nudibranchia) in the SCI “Fondali Noli-Bergeggi” (Ligurian Sea). Marine Biodiversity Records 8:e26. DOI: 10.1017/S1755267215000032.
- Beuvier J, Sevault F, Herrmann M, Kontoyiannis H, Ludwig W, Rixen M, Stanev E, Béranger K, Somot S. 2010. Modeling the Mediterranean Sea interannual variability during 1961–2000: Focus on the Eastern Mediterranean transient. Journal of Geophysical Research 115:C08017. DOI: 10.1029/2009JC005950.
- Bianchi CN. 1997. Climate change and biological response in the marine benthos. Atti dell’Associazione Italiana di Oceanologia e Limnologia 12:3–20.
- Bianchi CN. 2007. Biodiversity issues for the forthcoming tropical Mediterranean Sea. Hydrobiologia 580:7–21. DOI: 10.1007/s10750-006-0469-5.
- Bianchi CN, Boudouresque CF, Francour P, Morri C, Parravicini V, Templado J, Zenetos A. 2013. The changing biogeography of the Mediterranean Sea: From the old frontiers to the new gradients. Bollettino dei Musei e degli Istituti Biologici dell’Università di Genova 75:81–84.
- Bianchi CN, Caroli F, Guidetti P, Morri C. 2018a. Seawater warming at the northern reach for southern species: Gulf of Genoa, NW Mediterranean. Journal of the Marine Biological Association of the United Kingdom 98:1–12. DOI: 10.1017/S0025315417000819.
- Bianchi CN, Cattaneo-Vietti R, Cinelli F, Morri C, Pansini M. 1996. Lo studio biologico delle grotte sottomarine: Conoscenze attuali e prospettive. Bollettino dei Musei e degli Istituti Biologici dell’Università di Genova (1994-95) 60-61:41–69.
- Bianchi CN, Cevasco MG, Diviacco G, Morri C. 1986. Primi risultati di una ricerca ecologica sulla grotta marina di Bergeggi (Savona). Bollettino dei Musei e degli Istituti Biologici dell’Università di Genova 52(suppl.):267–293.
- Bianchi CN, Cocito S, Diviacco G, Dondi N, Fratangeli F, Montefalcone M, Parravicini V, Rovere A, Sgorbini S, Vacchi M, Morri C. 2018b. The park never born: Outcome of a quarter of a century of inaction on the sea-floor integrity of a proposed but not established Marine Protected Area. Aquatic Conservation: Marine and Freshwater Ecosystems 28:1209–1228. DOI: 10.1002/aqc.v28.5.
- Bianchi CN, Corsini-Foka M, Morri C, Zenetos A. 2014. Thirty years after: Dramatic change in the coastal marine ecosystems of Kos Island (Greece), 1981–2013. Mediterranean Marine Science 15:482–497. DOI: 10.12681/mms.678.
- Bianchi CN, Morri C. 1993. Range extensions of warm-water species in the northern Mediterranean: Evidence for climatic fluctuations? Porcupine Newsletter 5:156–159.
- Bianchi CN, Morri C. 1994a. Southern species in the Ligurian Sea (northern Mediterranean): New records and a review. Bollettino dei Musei e degli Istituti Biologici dell’Università di Genova 58-59(1992–1993):181–197.
- Bianchi CN, Morri C. 1994b. Studio bionomico comparativo di alcune grotte marine sommerse: Definizione di una scala di confinamento. Memorie dell’Istituto Italiano di Speleologia Ser. II 6:107–123.
- Bianchi CN, Morri C. 2000a. Marine biodiversity of the Mediterranean Sea: Situation, problems and prospects for future research. Marine Pollution Bulletin 40:367–376. DOI: 10.1016/S0025-326X(00)00027-8.
- Bianchi CN, Morri C. 2000b. Training scientific divers - Italian style. Ocean Challenge 10:25–29.
- Bianchi CN, Morri C. 2003. Global sea warming and “tropicalization” of the Mediterranean Sea: Biogeographic and ecological aspects. Biogeographia 24:319–327.
- Bianchi CN, Morri C. 2004a. Climate change and biological response in Mediterranean Sea ecosystems – A need for broad-scale and long-term research. Ocean Challenge 2003 13:32–36.
- Bianchi CN, Morri C. 2004b. Uomo, clima e biodiversità marina: Esempi dal Mar Ligure. Uomo e Natura 9:15–23.
- Bianchi CN, Morri C, Chiantore M, Montefalcone M, Parravicini V, Rovere A. 2012. Mediterranean Sea biodiversity between the legacy from the past and a future of change. In: Stambler N, editor. Life in the Mediterranean Sea: A look at habitat changes. New York, USA: Nova Science Publishers. pp. 1–55.
- Bianchi CN, Morri C, Peirano A, Romeo G, Tunesi L. 1987. Caratterizzazione ecotipologica delle coste italiane. Bibliografia ecotipologica sul Mar Ligure. Elenco preliminare. Roma, Italy: ENEA, Collana di studi ambientali.
- Bianchi CN, Peirano A. 1995. Atlante delle fanerogame marine della Liguria: Posidonia oceanica e Cymodocea nodosa. La Spezia, Italy: ENEA, Centro Ricerche Ambiente Marino.
- Bianchi CN, Peirano A, Salvati E, Morri C. 2001. Assessing interannual and decadal changes in marine epibenthic assemblages through UW photography: An example from Punta Mesco, Ligurian Sea. Archivio di Oceanografia e Limnologia 22:83–86.
- Bianchi CN, Pronzato R, Cattaneo-Vietti R, Benedetti-Cecchi L, Morri C, Pansini M, Chemello R, Milazzo M, Fraschetti S, Terlizzi A, Peirano A, Salvati E, Benzoni F, Calcinai B, Cerrano C, Bavestrello G. 2004. Mediterranean marine benthos: A manual of methods for its sampling and study. 6: Hard bottoms. Biologia Marina Mediterranea 11(suppl. 1):185–215.
- Blanfuné A, Boudouresque CF, Verlaque M, Thibaut T. 2016. The fate of Cystoseira crinita, a forest-forming Fucale (Phaeophyceae, Stramenopiles), in France (North Western Mediterranean Sea). Estuarine, Coastal and Shelf Science 181:196–208. DOI: 10.1016/j.ecss.2016.08.049.
- Bo M, Bava S, Canese S, Angiolillo M, Cattaneo-Vietti R, Bavestrello G. 2014. Fishing impact on deep Mediterranean rocky habitats as revealed by ROV investigation. Biological Conservation 171:167–176. DOI: 10.1016/j.biocon.2014.01.011.
- Boero F. 1984. The ecology of marine hydroids and effects of environmental factors: A review. Marine Ecology 5:93–118. DOI: 10.1111/j.1439-0485.1984.tb00310.x.
- Boero F. 2011. New species are welcome, but … what about the old ones? Italian Journal of Zoology 78:1–2. DOI: 10.1080/11250003.2011.562708.
- Boero F. 2014. GES revolution. Italian Journal of Zoology 81:311. DOI: 10.1080/11250003.2014.957024.
- Boero F. 2015a. The future of the Mediterranean Sea Ecosystem: Towards a different tomorrow. Rendiconti Lincei Scienze Fisiche e Naturali 26:3–12. DOI: 10.1007/s12210-014-0340-y.
- Boero F. 2015b. Mediterranean scenarios. In: Bekken-Nielsen T, Gestwagen R, editors. The Inland Seas: Towards an ecohistory of the Mediterranean and Black Sea. Stuttgart, Germany: Franz Steiner Verlag, Geographica Historica. Vol. 35. pp. 387–398.
- Boero F, Fresi E. 1986. Zonation and evolution of a rocky bottom hydroid community. Marine Ecology 7:123–150. DOI: 10.1111/j.1439-0485.1986.tb00152.x.
- Boero F, Kraberg AC, Krause G, Wiltshire KH. 2015. Time is an affliction: Why ecology cannot be as predictive as physics and why it needs time series. Journal of Sea Research 101:12–18. DOI: 10.1016/j.seares.2014.07.008.
- Boero F, Putti M, Trainito E, Prontera E, Piraino S, Shiganova TA. 2009. First records of Mnemiopsis leidyi (Ctenophora) from the Ligurian, Thyrrhenian and Ionian Seas (Western Mediterranean) and first record of Phyllorhiza punctata (Cnidaria) from the Western Mediterranean. Aquatic Invasions 4:675–680. DOI: 10.3391/ai.
- Boudouresque CF. 1970. Recherches sur les concepts de biocénose et de continuum au niveau de peuplements benthiques sciaphiles. Vie et Milieu 21(1B):103–136.
- Boudouresque CF, Bianchi CN. 2013. Une idée neuve: La protection des espèces marines. In: Le Diréach L, Boudouresque CF, editors. GIS posidonie: Plus de 30 ans au service de la protection et de la gestion du milieu marin. Marseilles, France: GIS Posidonie. pp. 85–91.
- Boudouresque CF, Nedelec N, Sheperd SA. 1980. The decline of a population of the sea urchin Paracentrotus lividus in the Bay of Port-Cros (Var, France). Travaux Scientifiques du Parc National de Port-Cros 6:243–251.
- Britten GL, Dowd M, Minto C, Ferretti F, Boero F, Lotze H-K. 2014. Predator decline leads to decreased stability in a coastal fish community. Ecology Letters 17:1518–1525. DOI: 10.1111/ele.12354.
- Broecker WS. 1975. Climatic change: Are we on the brink of a pronounced global warming? Science 189:460–463. DOI: 10.1126/science.189.4201.460.
- Bulleri F, Benedetti-Cecchi L. 2008. Facilitation of the introduced green alga Caulerpa racemosa by resident algal turfs: Experimental evaluation of underlying mechanisms. Marine Ecology Progress Series 364:77–86. DOI: 10.3354/meps07484.
- Burge CA, Eakin CM, Friedman CS, Froelich B, Hershberger PK, Hofmann EE, Petes LE, Prager KC, Weil E, Willis BL, Ford SE, Harvell CD. 2014. Climate change influences on marine infectious diseases: Implications for management and society. Annual Review of Marine Science 6:249–277. DOI: 10.1146/annurev-marine-010213-135029.
- Burgos E, Montefalcone M, Ferrari M, Paoli C, Vassallo P, Morri C, Bianchi CN. 2017. Ecosystem functions and economic wealth: Trajectories of change in seagrass meadows. Journal of Cleaner Production 168:1108–1119. DOI: 10.1016/j.jclepro.2017.09.046.
- Burgos-Juan E, Montefalcone M, Ferrari M, Morri C, Bianchi CN. 2016. A century of change in seagrass meadows of Liguria. Biologia Marina Mediterranea 23:78–81.
- Bussotti S, Conti M, Guidetti P, Martini F, Matricardi G. 1996. First record of Caulerpa racemosa (Forsskål) J. Agardh along the coast of Genoa (North-Western Mediterranean). Doriana 6:1–5.
- Calvo E, Simó R, Coma R, Ribes M, Pascual J, Sabatés A, Gili JM, Pelejero C. 2011. Effects of climate change on Mediterranean marine ecosystems: The case of the Catalan Sea. Climate Research 50:1–29. DOI: 10.3354/cr01040.
- Canessa M, Montefalcone M, Cánovas Molina A, Coppo S, Diviacco G, Bavestrello G, Morri C, Bianchi CN. 2014. Submerged marine caves of Liguria: Updating the knowledge. In: Langar H, Bouafif C, Ouerghi A, editors. Proceedings of the 1st Mediterranean symposium on the conservation of dark habitats. Tunis, Tunisia: UNEP/MAP–RAC/SPA. pp. 27–32.
- Cánovas-Molina A, Montefalcone M, Bavestrello G, Cau A, Bianchi CN, Morri C, Canese S, Bo M. 2016a. A new ecological index for the status of mesophotic megabenthic assemblages in the Mediterranean based on ROV photography and video footage. Continental Shelf Research 121:13–20. DOI: 10.1016/j.csr.2016.01.008.
- Cánovas-Molina A, Montefalcone M, Vassallo P, Morri C, Bianchi CN, Bavestrello G. 2016b. Combining literature review, acoustic mapping and in situ observations: An overview of coralligenous assemblages in Liguria (NW Mediterranean Sea). Scientia Marina 80:7–16.
- Capdevila P, Hereu B, Salguero-Gómez R, Rovira G, Medrano A, Cebrian E, Garrabou J, Kersting DK, Linares C. 2019. Warming impacts on early life stages increase the vulnerability and delay the population recovery of a long-lived habitat-forming macroalga. Journal of Ecology 107:1129–1140. DOI: 10.1111/1365-2745.13090.
- Caputi N, Kangas M, Chandrapavan A, Hart A, Feng M, Marin M, de Lestang S. 2019. Factors affecting the recovery of invertebrate stocks from the 2011 Western Australian extreme marine heatwave. Frontiers in Marine Science 6:484. DOI: 10.3389/fmars.2019.00484.
- Cardinale BJ, Duffy JE, Gonzalez A, Hooper DU, Perrings C, Venail P, Narwani A, Mace GM, Tilman D, Wardle DA, Kinzig AP, Daily GC, Loreau M, Grace JB, Larigauderie A, Srivastava D, Naeem S. 2012. Biodiversity loss and its impact on humanity. Nature 486:59–67. DOI: 10.1038/nature11148.
- Carella F, Aceto S, Pollaro F, Miccio A, Iaria C, Carrasco N, Prado P, De Vico G. 2019. A mycobacterial disease is associated with the silent mass mortality of the pen shell Pinna nobilis along the Tyrrhenian coastline of Italy. Scientific Reports 9:2725. DOI: 10.1038/s41598-018-37217-y.
- Carmen B, Krause-Jensen D, Alcoverro T, Marbà N, Duarte CM, van Katwijk MM, Pérez M, Romero J, Sánchez-Lizaso JL, Roca G, Jankowska E, Pérez-Lloréns JL, Fournier J, Montefalcone M, Pergent G, Ruiz JM, Cabaço S, Cook K, Wilkes RJ, Moy FE, Trayter GMR, Arañó XS, de Jong DJ, Fernández-Torquemada Y, Auby I, Vergara JJ, Santos R. 2019. Recent trend reversal for declining European seagrass meadows. Nature Communications 10:3356. DOI: 10.1038/s41467-019-11340-4.
- Casella E, Molcard A, Provenzale A. 2011. Mesoscale vortices in the Ligurian Sea and their effect on coastal upwelling processes. Journal of Marine Systems 88:12–19. DOI: 10.1016/j.jmarsys.2011.02.019.
- Catra M, Alongi G, Leonardi R, Negri MP, Sanfilippo R, Sciuto F, Serio D, Viola A, Rosso A. 2019. Degradation of a photophilic algal community and its associated fauna from eastern Sicily (Mediterranean Sea). Mediterranean Marine Science 20:74–89.
- Cattaneo R, Pastorino MV. 1974. Popolamenti algali e fauna bentonica nelle cavità naturali della regione litorale mediterranea. Rassegna Speleologica Italiana 12:272–281.
- Cattaneo-Vietti R. 2018. Structural changes in Mediterranean marine communities: Lessons from the Ligurian Sea. Rendiconti Lincei Scienze Fisiche e Naturali 29:515–524. DOI: 10.1007/s12210-018-0670-2.
- Cattaneo-Vietti R, Albertelli G, Aliani S, Bava S, Bavestrello G, Benedetti Cecchi L, Bianchi CN, Bozzo E, Capello M, Castellano M, Cerrano C, Chiantore M, Corradi N, Cocito S, Cutroneo L, Diviacco G, Fabiano M, Faimali M, Ferrari M, Gasparini GP, Locritani M, Mangialajo L, Marin V, Moreno M, Morri C, Orsi Relini L, Pane L, Paoli C, Petrillo M, Povero P, Pronzato R, Relini G, Santangelo G, Tucci S, Tunesi L, Vacchi M, Vassallo P, Vezzulli L, Wurtz M. 2010. The Ligurian Sea: State of the art, problems, and perspectives. Chemistry and Ecology 26(S 1):319–340. DOI: 10.1080/02757541003689845.
- Cattaneo-Vietti R, Bava S. 2009. La tonnarella e la pesca tradizionale a Camogli. Recco, Italy: Le Mani-Microart’S.
- Cattaneo-Vietti R, Cappanera V, Castellano M, Povero P. 2015. Yield and catch changes in a Mediterranean small tuna trap: A climate change effect? Marine Ecology 36:155–166. DOI: 10.1111/maec.12127.
- Cattaneo-Vietti R, Sirigu AP, Tommei A. 1988. Sea of Liguria. Genova, Italy: Research Centre of the Union of Ligurian Chambers of Commerce.
- Cerrano C, Arillo A, Azzini F, Calcinai B, Castellano L, Muti C, Valisano L, Zega G, Bavestrello G. 2005. Gorgonian population recovery after a mass mortality event. Aquatic Conservation: Marine and Freshwater Ecosystems 15:147–157. DOI: 10.1002/(ISSN)1099-0755.
- Cerrano C, Bavestrello G. 2009a. Mass mortalities and extinctions. In: Wahl M, editor. Marine hard bottom communities. Berlin, Germany: Springer, Ecological Studies (Analysis and Synthesis). Vol. 206. pp. 295–307.
- Cerrano C, Bavestrello G. 2009b. Medium-term effects of die-off of rocky benthos in the Ligurian Sea: What can we learn from gorgonians? Chemistry and Ecology 24:73–82. DOI: 10.1080/02757540801979648.
- Cerrano C, Bavestrello G, Bianchi CN, Cattaneo-Vietti R, Bava S, Morganti C, Morri C, Picco P, Sara G, Schiaparelli S, Siccardi A, Sponga F. 2000. A catastrophic mass-mortality episode of gorgonians and other organisms in the Ligurian Sea (Northwestern Mediterranean), summer 1999. Ecology Letters 3:284–293. DOI: 10.1046/j.1461-0248.2000.00152.x.
- Cerrano C, Magnino G, Sarà A, Bavestrello G, Gaino E. 2001. Necrosis in a population of Petrosia ficiformis (Porifera, Demospongiae) in relations with environmental stress. Italian Journal of Zoology 68:131–136. DOI: 10.1080/11250000109356397.
- Cerrano C, Totti C, Sponga F, Bavestrello G. 2006. Summer disease in Parazoanthus axinellae (Schmidt, 1862) (Cnidaria, Zoanthidea). Italian Journal of Zoology 73:355–361. DOI: 10.1080/11250000600911675.
- Chefaoui RM, Duarte CM, Serrão EA. 2018. Dramatic loss of seagrass habitat under projected climate change in the Mediterranean Sea. Global Change Biology 24:4919–4928. DOI: 10.1111/gcb.14401.
- Chevaldonné P, Lejeusne C. 2003. Regional warming-induced species shift in north-west Mediterranean marine caves. Ecology Letters 6:371–379. DOI: 10.1046/j.1461-0248.2003.00439.x.
- Cicogna F, Bianchi CN, Ferrari G, Forti P, editors. 2003. Grotte marine: Cinquant’anni di ricerca in Italia. Rome, Italy: Ministero dell’Ambiente e della Tutela del Territorio.
- Ciminiello P, Dell’Aversano C, Fattorusso E, Forino M, Tartaglione L, Grillo C, Melchiorre N. 2008. Putative palytoxin and its new analogue, ovatoxin-a, in Ostreopsis ovata collected along the Ligurian coasts during the 2006 toxic outbreak. Journal of the American Society for Mass Spectrometry 19:111–120. DOI: 10.1016/j.jasms.2007.11.001.
- Claudet J, Fraschetti S. 2010. Human-driven impacts on marine habitats: A regional meta-analysis in the Mediterranean Sea. Biological Conservation 143:2195–2206. DOI: 10.1016/j.biocon.2010.06.004.
- Cocito S, Sgorbini S. 2013. Long-term trend in substratum occupation by a clonal, carbonate bryozoan in a temperate rocky reef in times of thermal anomalies. Marine Biology 161:17–27. DOI: 10.1007/s00227-013-2310-9.
- Cocito S, Sgorbini S, Bianchi CN. 1998. Aspects of the biology of the bryozoan Pentapora fascialis in the north-western Mediterranean. Marine Biology 131:73–82. DOI: 10.1007/s002270050298.
- Cohen RE, James CC, Lee A, Martinelli MM, Muraoka WT, Ortega M, Sadowski R, Starkey L, Szesciorka R, Timko SE, Weiss EL, Franks PJS. 2018. Marine host-pathogen dynamics: Influences of global climate change. Oceanography 31:182–193. DOI: 10.5670/oceanog.2018.201.
- Cohu S, Mangialajo L, Thibaut T, Blanfuné A, Marro S, Lemée R. 2013. Proliferation of the toxic dinoflagellate Ostreopsis cf. ovata in relation to depth, biotic substrate and environmental factors in the north west Mediterranean Sea. Harmful Algae 24:32–44. DOI: 10.1016/j.hal.2013.01.002.
- Coll M, Piroddi C, Steenbeek J, Kaschner K, Ben Rais Lasram F, Aguzzi J, Ballesteros E, Bianchi CN, Corbera J, Dailianis T, Danovaro R, Estrada M, Froglia C, Galil BS, Gasol JM, Gertwagen R, Gil J, Guilhaumon F, Kesner-Reyes K, Kitsos MS, Koukouras A, Lampadariou N, Laxamana E, López-fé de la Cuadra CM, Lotze HK, Martin D, Mouillot D, Oro D, Raicevich S, Rius-Barile J, Saiz-Salinas JI, San Vicente C, Somot S, Templado J, Turon X, Vafidis D, Villanueva R, Voultsiadou E. 2010. The biodiversity of the Mediterranean Sea: Estimates, patterns, and threats. PloS One 5:e11842. DOI: 10.1371/journal.pone.0011842.
- Conversi A, Fonda Umani S, Peluso T, Molinero JC, Santojanni R, Edwards M. 2010. The Mediterranean Sea regime shift at the end of the 1980s, and intriguing parallelism with other European basins. PloS One 5:e10633. DOI: 10.1371/journal.pone.0010633.
- Costa G, Betti F, Nepote E, Cattaneo-Vietti R, Pansini M, Bavestrello G, Bertolino M. 2018. Sponge community variations within two semi-submerged caves of the Ligurian Sea (Mediterranean Sea) over a half-century time span. The European Zoological Journal 85:381–391. DOI: 10.1080/24750263.2018.1525439.
- Cullen-Unsworth LC, Nordlund LM, Paddock J, Baker S, McKenzie LJ, Unsworth RKF. 2014. Seagrass meadows globally as a coupled social–Ecological system: Implications for human wellbeing. Marine Pollution Bulletin 83:387–397. DOI: 10.1016/j.marpolbul.2013.06.001.
- Cupido R, Cocito S, Barsanti M, Sgorbini S, Peirano A, Santangelo G. 2009. Unexpected long-term population dynamics in a canopy-forming gorgonian following mass mortality. Marine Ecology Progress Series 394:195–200. DOI: 10.3354/meps08260.
- Cupido R, Cocito S, Manno V, Ferrando S, Peirano A, Iannelli M, Bramanti L, Santangelo G. 2012. Sexual structure of a highly reproductive, recovering gorgonian population: Quantifying reproductive output. Marine Ecology Progress Series 469:25–36. DOI: 10.3354/meps09976.
- Cupido R, Cocito S, Sgorbini S, Bordone A, Santangelo G. 2008. Response of a gorgonian (Paramuricea clavata) population to mortality events: Recovery or loss? Aquatic Conservation: Marine and Freshwater Ecosystems 18:984–992. DOI: 10.1002/aqc.v18:6.
- De La Fuente G, Asnaghi V, Chiantore M, Thrush S, Povero P, Vassallo P, Petrillo M, Paoli C. 2019. The effect of Cystoseira canopy on the value of midlittoral habitats in NW Mediterranean, an emergy assessment. Ecological Modelling 404:1–11. DOI: 10.1016/j.ecolmodel.2019.04.005.
- De La Fuente G, Chiantore M, Gaino F, Asnaghi V. 2018. Ecological status improvement over a decade along the Ligurian coast according to a macroalgae based index (CARLIT). PloS One 13:e0206826. DOI: 10.1371/journal.pone.0206826.
- Defriecz EJ, Sheppard LW, Reid PC, Reuman DC. 2016. Climate change-related regime shifts have altered spatial synchrony of plankton dynamics in the North Sea. Global Change Biology 22:2069–2080. DOI: 10.1111/gcb.13229.
- Dornelas M, Gotelli NJ, Shimadzu H, Moyes F, Magurran AE, McGill BJ. 2019. A balance of winners and losers in the Anthropocene. Ecology Letters 22:847–854. DOI: 10.1111/ele.2019.22.issue-5.
- Durrieu de Madron X, Guieu C, Sempéré R, Conan P, Cossa D, D’Ortenzio F, Estournel C, Gazeau F, Rabouille C, Stemmann L, Bonnet S, Diaz F, Koubbi P, Radakovitch O, Babin M, Baklouti M, Bancon-Montigny C, Belviso S, Bensoussan N, Bonsang B, Bouloubassi I, Brunet C, Cadiou JF, Carlotti F, Chami M, Charmasson S, Charrière B, Dachs J, Doxaran D, Dutay JC, Elbaz-Poulichet F, Eléaume M, Eyrolles F, Fernandez C, Fowler S, Francour P, Gaertner JC, Galzin R, Gasparini S, Ghiglione JF, Gonzalez JL, Goyet C, Guidi L, Guizien K, Heimbürger LE, Jacquet SHM, Jeffrey WH, Joux F, Le Hir P, Leblanc K, Lefèvre D, Lejeusne C, Lemé R, Loÿe-Pilot MD, Mallet M, Méjanelle L, Mélin F, Mellon C, Mérigot B, Merle PL, Migon C, Miller WL, Mortier L, Mostajir B, Mousseau L, Moutin T, Para J, Pérez T, Petrenko A, Poggiale JC, Prieur L, Pujo-Pay M, Pulido-Villena E, Raimbault P, Rees AP, Ridame C, Rontani JF, Ruiz Pino D, Sicre MA, Taillandier V, Tamburini C, Tanaka T, Taupier-Letage I, Tedetti M, Testor P, Thébault H, Thouvenin B, Touratier F, Tronczynski J, Ulses C, Van Wambeke F, Vantrepotte V, Vaz S, Verney R. 2011. Marine ecosystems’ responses to climatic and anthropogenic forcings in the Mediterranean. Progress in Oceanography 91:97–166. DOI: 10.1016/j.pocean.2011.02.003.
- Enrichetti F, Bava S, Bavestrello G, Betti F, Lanteri L, Bo M. 2019a. Artisanal fishing impact on deep coralligenous animal forests: A Mediterranean case study of marine vulnerability. Ocean and Coastal Management 177:112–126. DOI: 10.1016/j.ocecoaman.2019.04.021.
- Enrichetti F, Bo M, Morri C, Montefalcone M, Toma M, Bavestrello G, Tunesi L, Canese S, Giusti M, Salvati E, Bertolotto RM, Bianchi CN. 2019b. Assessing the environmental status of temperate mesophotic reefs: A new, integrated methodological approach. Ecological Indicators 102:218–229. DOI: 10.1016/j.ecolind.2019.02.028.
- Enrichetti F, Dominguez-Carrió C, Toma M, Bavestrello G, Betti F, Canese S, Bo M. 2019c. Megabenthic communities of the Ligurian deep continental shelf and shelf break (NW Mediterranean Sea). PloS One 14:e0223949. DOI: 10.1371/journal.pone.0223949.
- Faimali M, Giussani V, Piazza V, Garaventa F, Corrà C, Asnaghi V, Privitera D, Gallus L, Cattaneo-Vietti R, Mangialajo L, Chiantore M. 2012. Toxic effects of harmful benthic dinoflagellate Ostreopsis ovata on invertebrate and vertebrate marine organisms. Marine Environmental Research 76:97–107. DOI: 10.1016/j.marenvres.2011.09.010.
- Ferraris M, Berline L, Lombard F, Guidi L, Elineau A, Mendoza-Vera JM, Lilley MKS, Taillandier V, Gorsky G. 2012. Distribution of Pelagia noctiluca (Cnidaria, Scyphozoa) in the Ligurian Sea (NW Mediterranean Sea). Journal of Plankton Research 34:874–885. DOI: 10.1093/plankt/fbs049.
- Ferretti F, Myers RA, Sartor P, Serena F. 2005. Long term dynamics of the chondrichthyan fish community in the upper Tyrrhenian Sea. International Council for the Exploration of the Sea Conferences and Meetings 25:1–34.
- Ferretti F, Myers RA, Serena F, Lotze HK. 2008. Loss of large predatory sharks from the Mediterranean Sea. Conservation Biology 22:952–964. DOI: 10.1111/j.1523-1739.2008.00938.x.
- Francour P, Boudouresque CF, Harmelin JG, Harmelin-Vivien ML, Quignard JP. 1994. Are the Mediterranean waters becoming warmer? Information from biological indicators. Marine Pollution Bulletin 28:523–526. DOI: 10.1016/0025-326X(94)90071-X.
- Fraschetti S, Guarnieri G, Bevilacqua S, Terlizzi A, Claudet J, Russo GF, Boero F. 2011. Conservation of Mediterranean habitats and biodiversity countdowns: What information do we really need? Aquatic Conservation: Marine and Freshwater Ecosystems 21:299–306. DOI: 10.1002/aqc.v21.3.
- Gaino E, Pronzato R. 1989. Ultrastructural evidence of bacterial damage to Spongia officinalis fibres (Porifera, Demospongiae). Diseases of Aquatic Organisms 6:67–74. DOI: 10.3354/dao006067.
- Gaino E, Pronzato R, Corriero G, Buffa P. 1992. Mortality of commercial sponges: Incidence in two Mediterranean areas. Bollettino di Zoologia 59:79–85. DOI: 10.1080/11250009209386652.
- Gambi MC, Barbieri F, Bianchi CN. 2008. New record of the alien seagrass Halophila stipulacea (Hydrocharitaceae) in the western Mediterranean: A further clue to changing Mediterranean Sea biogeography. Biodiversity Records 2:e84. DOI: 10.1017/S175526720900058X.
- Garrabou J, Coma R, Benssousan N, Bally M, Chevaldonné P, Cigliano M, Diaz D, Harmelin JG, Gambi MC, Kersting DK, Ledoux JB, Lejeusne C, Linares C, Marschal C, Pérez T, Ribes M, Romano JC, Serrano E, Teixido N, Torrents O, Zabala M, Zuberer F, Cerrano C. 2009. Mass mortality in NW Mediterranean rocky benthic communities: Effects of the 2003 heat wave. Global Change Biology 15:1090–1103. DOI: 10.1111/j.1365-2486.2008.01823.x.
- Garrabou J, Pérez T, Sartoretto S, Harmelin JG. 2001. Mass mortality event in red coral Corallium rubrum populations in the Provence region (France, NW Mediterranean). Marine Ecology Progress Series 217:263–272. DOI: 10.3354/meps217263.
- Gasparini GP, Ortona A, Budillon G, Astraldi M, Sansone E. 2005. The effect of the eastern Mediterranean transient on the hydrographic characteristics in the Strait of Sicily and in the Tyrrhenian Sea. Deep Sea Research Part I: Oceanographic Research Papers 52:915–935. DOI: 10.1016/j.dsr.2005.01.001.
- Gatti G, Bianchi CN, Montefalcone M, Venturini S, Diviacco G, Morri C. 2017. Observational information on a temperate reef community helps understanding the marine climate and ecosystem shift of the 1980–90s. Marine Pollution Bulletin 114:528–538. DOI: 10.1016/j.marpolbul.2016.10.022.
- Gatti G, Bianchi CN, Parravicini V, Rovere A, Peirano A, Montefalcone M, Massa F, Morri C. 2015. Ecological change, sliding baselines and the importance of historical data: Lessons from combining observational and quantitative data on a temperate reef over 70 years. PloS One 10:e0118581. DOI: 10.1371/journal.pone.0118581.
- Gattuso JP, Magnan A, Billé R, Cheung WWL, Howes EL, Joos F, Allemand D, Bopp L, Cooley SR, Eakin CM, Hoegh-Guldberg O, Kelly RP, Pörtner HO, Rogers AD, Baxter JM, Laffoley D, Osborn D, Rankovic A, Rochette J, Sumaila UR, Treyer S, Turley C. 2015. Contrasting futures for ocean and society from different anthropogenic CO2 emissions scenarios. Science 349:aac4722. DOI: 10.1126/science.aac4722.
- Giorgi F. 2006. Climate change hot-spots. Geophysical Research Letters 33:L08707. DOI: 10.1029/2006GL025734.
- Giuliani S, Virno Lamberti C, Sonni C, Pellegrini D. 2005. Mucilage impact on gorgonians in the Tyrrhenian Sea. Science of the Total Environment 353:340–349. DOI: 10.1016/j.scitotenv.2005.09.023.
- Greco G, Capello M, Cecchi G, Cutroneo L, Di Piazza S, Zotti M. 2017. Another possible risk for the Mediterranean Sea? Aspergillus sydowii discovered in the Port of Genoa (Ligurian Sea, Italy). Marine Pollution Bulletin 122:470–474. DOI: 10.1016/j.marpolbul.2017.06.058.
- Guidetti P, Bianchi CN, La Mesa G, Modena M, Morri C, Sara G, Vacchi M. 2002. Abundance and size structure of Thalassoma pavo (Pisces: Labridae) in the western Mediterranean Sea: Variability at different spatial scales. Journal of the Marine Biological Association of the UK 82:495–500. DOI: 10.1017/S0025315402005775.
- Hampton SE, Scheuerell MD, Church MJ, Melack JM. 2019. Long-term perspectives in aquatic research. Limnology and Oceanography 64:S2–S10.
- Harmelin JC, Marinopoulos J. 1994. Population structure and partial mortality of the gorgonian Paramuricea clavata (Risso) in the North-Western Mediterranean (France, Port-Cros Island). Marine Life 4:5–13.
- Harvell CD, Kim K, Burkholder JM, Colwell RR, Epstein PR, Grimes DJ, Hofmann EE, Lipp EK, Osterhaus ADME, Overstreet RM, Porter JW, Smith GW, Vasta GR. 1999. Emerging marine disease-climate links and anthropogenic factors. Science 285:1505–1510. DOI: 10.1126/science.285.5433.1505.
- Hayes ML, Bonaventura J, Mitchell TP, Propero JM, Shinn EA, Van Dolah F, Barber RT. 2001. How are climate changes and emerging marine diseases functionally linked? Hydrobiologia 460:213–220. DOI: 10.1023/A:1013121503937.
- Holon F, Boissery P, Guilbert A, Freschet E, Deter J. 2015. The impact of 85 years of coastal development on shallow seagrass beds (Posidonia oceanica L. (Delile)) in South Eastern France: A slow but steady loss without recovery. Estuarine, Coastal and Shelf Science 165:204–212. DOI: 10.1016/j.ecss.2015.05.017.
- Hooper DU, Chapin III FS, Ewel JJ, Hector A, Inchausti P, Lavorel S, Lawton JH, Lodge DM, Loreau M, Naeem S, Schmid B, Setälä H, Symstad AJ, Vandermeer J, Wardle DA. 2005. Effects of biodiversity on ecosystem functioning: A consensus of current knowledge. Ecological Monographs 75:3–35. DOI: 10.1890/04-0922.
- Issel R. 1918. Biologia marina: Forme e fenomeni della vita nel mare. Milano, Italia: Hoepli.
- Lasagna R, Montefalcone M, Albertelli G, Corradi N, Ferrari M, Morri C, Bianchi CN. 2011. Much damage for little advantage: Field studies and morphodynamic modelling highlighted the environmental impact of an apparently small coastal mismanagement. Estuarine, Coastal and Shelf Science 94:255–262. DOI: 10.1016/j.ecss.2011.07.003.
- Lasagna R, Montefalcone M, Bianchi CN, Morri C, Albertelli G. 2006a. Approccio macrostrutturale alla valutazione dello stato di salute di una prateria di Posidonia oceanica. Biologia Marina Mediterranea 13:379–385.
- Lasagna R, Montefalcone M, Bianchi CN, Morri C, Albertelli G. 2006b. Morphology of a Posidonia oceanica meadow under altered sedimentary budget. Biologia Marina Mediterranea 13:245–249.
- Laubier L. 2001. Climatic changes and trends and marine invertebrates: A need for relevant observing networks and experimental ecophysiology. Proceedings of the Italian Association of Oceanology and Limnology 14:15–24.
- Lejeusne C, Chevaldonné P, Pergent-Martini C, Boudouresque CF, Pérez T. 2010. Climate change effects on a miniature ocean: The highly diverse, highly impacted Mediterranean Sea. Trends in Ecology and Evolution 25:250–260. DOI: 10.1016/j.tree.2009.10.009.
- Lelieveld J, Berresheim H, Borrmann S, Crutzen PJ, Dentener FJ. 2002. Global air pollution crossroads over the Mediterranean. Science 298:794–799. DOI: 10.1126/science.1075457.
- Lima FP, Wethey DS. 2012. Three decades of high-resolution coastal sea surface temperatures reveal more than warming. Nature Communications 3:704. DOI: 10.1038/ncomms1713.
- Linares CL, Coma R, Diaz D, Zabala M, Hereu B, Dantart L. 2005. Immediate and delayed effects of a mass mortality event on gorgonian population dynamics and benthic community structure in the NW Mediterranean Sea. Marine Ecology Progress Series 305:127–137. DOI: 10.3354/meps305127.
- Longobardi L, Bavestrello G, Betti F, Cattaneo-Vietti R. 2017. Long-term changes in a Ligurian infralittoral community (Mediterranean Sea): A warning signal? Regional Studies in Marine Science 14:15–26. DOI: 10.1016/j.rsma.2017.03.011.
- Losi V, Sbrocca C, Gatti G, Semprucci F, Rocchi M, Bianchi CN, Balsamo M. 2018. Sessile macrobenthos (Ochrophyta) drives seasonal change of meiofaunal community structure on temperate rocky reefs. Marine Environmental Research 142:295–305. DOI: 10.1016/j.marenvres.2018.10.016.
- Lucrezi S, Milanese M, Markantonatou V, Cerrano C, Sarà A, Palma M, Saayman M. 2017. Scuba diving tourism systems and sustainability: Perceptions by the scuba diving industry in two marine protected areas. Tourism Management 59:385–403. DOI: 10.1016/j.tourman.2016.09.004.
- MacDougall AS, Turkington R. 2005. Are invasive species the drivers or passengers of change in degraded ecosystems? Ecology 86:42–55. DOI: 10.1890/04-0669.
- MacLeod CD. 2009. Global climate change, range changes and potential implications for the conservation of marine cetaceans: A review and synthesis. Endangered Species Research 7:125–136. DOI: 10.3354/esr00197.
- Malanotte-Rizzoli P, Manca BB, d’Alcala MR, Theocharis A, Brenner S, Budillon G, Ozsoy E. 1999. The Eastern Mediterranean in the 80s and in the 90s: The big transition in the intermediate and deep circulations. Dynamics of Atmospheres and Oceans 29:365–395. DOI: 10.1016/S0377-0265(99)00011-1.
- Manca BB. 2000. Recent changes in dynamics of the Eastern Mediterranean affecting the water characteristics of the adjacent basins. In: Briand F, editor. The Eastern Mediterranean climatic transient, its origin, evolution and impact on the ecosystem. Monaco: CIESM, Workshop Series n 10. pp. 27–31.
- Mangialajo L, Bertolotto R, Cattaneo-Vietti R, Chiantore M, Grillo C, Lemee R, Melchiorre N, Moretto P, Povero P, Ruggieri N. 2008a. The toxic benthic dinoflagellate Ostreopsis ovata: Quantification of proliferation along the coastline of Genoa, Italy. Marine Pollution Bulletin 56:1209–1214. DOI: 10.1016/j.marpolbul.2008.02.028.
- Mangialajo L, Chiantore M, Cattaneo-Vietti R. 2008b. Loss of fucoid algae along a gradient of urbanisation, and structure of benthic assemblages. Marine Ecology Progress Series 358:63–74. DOI: 10.3354/meps07400.
- Mangialajo L, Ganzin N, Accoroni S, Asnaghi V, Blanfuné A, Cabrini M, Cattaneo-Vietti R, Chavanon F, Chiantore M, Cohu S, Costa E, Fornasaro D, Grossel H, Marco-Miralles F, Masó M, Reñé A, Rossi AM, Montserrat Sala M, Thibaut T, Totti C, Vila M, Lemée R. 2010. Trends in Ostreopsis proliferation along the Northern Mediterranean coasts. Toxicon 57:408–420. DOI: 10.1016/j.toxicon.2010.11.019.
- Mangialajo L, Ruggieri N, Asnaghi V, Chiantore M, Povero P, Cattaneo-Vietti R. 2007. Ecological status in the Ligurian Sea: The effect of coastline urbanization and the importance of proper reference sites. Marine Pollution Bulletin 55:30–41. DOI: 10.1016/j.marpolbul.2006.08.022.
- Marchetti R. 1965. Ricerche sul corallo rosso della costa ligure e toscana. II. Il Promontorio di Portofino. Rendiconti dell’Istituto Lombardo di Scienze e Lettere B 99:279–316.
- Marchini A, Ragazzola F, Vasapollo C, Castelli A, Cerrati G, Gazzola F, Jiang C, Langeneck J, Manauzzi MC, Musco L, Nannini M, Zekonyte J, Lombardi C. 2019. Intertidal Mediterranean coralline algae habitat is expecting a shift toward a reduced growth and a simplified associated fauna under climate change. Frontiers in Marine Science 6:106. DOI: 10.3389/fmars.2019.00106.
- Martin Y, Bonnefort JL, Chancerell L. 2002. Gorgonians mass mortality during the 1999 late summer in French Mediterranean coastal waters: The bacterial hypothesis. Water Research 36:779–782. DOI: 10.1016/S0043-1354(01)00251-2.
- Marty JC, Chiavérini J. 2010. Hydrological changes in the Ligurian Sea (NW Mediterranean, DYFAMED site) during 1995-2007 and biogeochemical consequences. Biogeosciences 7:2117–2128. DOI: 10.5194/bg-7-2117-2010.
- Mateos-Molina D, Palma M, Ruiz-Valentín I, Panagos P, García-Charton JA, Ponti M. 2015. Assessing consequences of land cover changes on sediment deliveries to coastal waters at regional level over the last two decades in the northwestern Mediterranean Sea. Ocean and Coastal Management 116:435–442. DOI: 10.1016/j.ocecoaman.2015.09.003.
- McKinney ML, Lockwood JL. 1999. Biotic homogenization: A few winners replacing many losers in the next mass extinction. Trends in Ecology and Evolution 14:450–453. DOI: 10.1016/S0169-5347(99)01679-1.
- Meinesz A. 1999. Killer algae: A true tale of biological invasion. Chicago, USA: University Press.
- Meinesz A, Mercier D. 1983. Sur les fortes mortalités de Spondylus gaederopous Linné observées sur les côtes de Méditerranée. Travaux Scientifiques du Parc National de Port-Cros 9:89–95.
- Meliadò E, Bavestrello G, Gnone G, Cattaneo-Vietti R. 2019. Dolphin bounty hunting in the history of the Italian fishery. Journal of Cetacean Research and Management ( In press).
- Meroni L, Chiantore M, Petrillo M, Asnaghi V. 2018. Habitat effects on Ostreopsis cf. ovata bloom dynamics. Harmful Algae 80:64–71. DOI: 10.1016/j.hal.2018.09.006.
- Misic C, Castellano M, Covazzi-Arriague A. 2011. Organic matter features, degradation and remineralization at two coastal sites in the Ligurian Sea (NW Mediterranean) differently influenced by anthropogenic forcing. Marine Environment Research 72:67–74. DOI: 10.1016/j.marenvres.2011.05.006.
- Modena M, Matricardi G, Vacchi M, Guidetti P. 2000. Spreading of Caulerpa racemosa (Forsskål) J Agardh (Bryopsidaceae, Chlorophyta) along the coasts of the Ligurian Sea. Cryptogamie Algologie 21:301–304. DOI: 10.1016/S0181-1568(00)00114-8.
- Molinier R, Picard J. 1952. Recherches sur les herbiers de phanérogames marines du littoral méditerranéen français. Annales de l’Institut Océanographique 27:157–234.
- Montefalcone M. 2009. Ecosystem health assessment using the seagrass Posidonia oceanica: A review. Ecological Indicators 9:595–604. DOI: 10.1016/j.ecolind.2008.09.013.
- Montefalcone M, Albertelli G, Bianchi CN, Mariani M, Morri C. 2006a. A new synthetic index and a protocol for monitoring the status of Posidonia oceanica meadows: A case study at Sanremo (Ligurian Sea, NW Mediterranean). Aquatic Conservation: Marine and Freshwater Ecosystems 16:29–42. DOI: 10.1002/(ISSN)1099-0755.
- Montefalcone M, Albertelli G, Morri C, Bianchi CN. 2010b. Patterns of wide-scale substitution within meadows of the seagrass Posidonia oceanica in NW Mediterranean Sea: Invaders are stronger than natives. Aquatic Conservation: Marine and Freshwater Ecosystems 20:507–515. DOI: 10.1002/aqc.v20:5.
- Montefalcone M, Albertelli G, Morri C, Parravicini V, Bianchi CN. 2009. Legal protection is not enough: Posidonia oceanica meadows in marine protected areas are not healthier than those in unprotected areas of the northwest Mediterranean Sea. Marine Pollution Bulletin 58:515–519. DOI: 10.1016/j.marpolbul.2008.12.001.
- Montefalcone M, Amigoni E, Bianchi CN, Morri C, Peirano A, Albertelli G. 2008a. Multiscale lepidochronological analysis of Posidonia oceanica (L.) Delile production in a northwestern Mediterranean coastal area. Chemistry and Ecology 24(S1):187–193. DOI: 10.1080/02757540801970100.
- Montefalcone M, Bianchi CN, Morri C, Peirano A, Albertelli G. 2007a. Human disturbance and phase shift in the Posidonia oceanica meadows of the Ligurian Sea (NW Mediterranean). In: Pergent-Martini C, El Asmi S, Le Ravallec C, editors. Proceedings of the third Mediterranean symposium on marine vegetation. Tunis, Tunisia: RAC/SPA. pp. 124–129.
- Montefalcone M, Chiantore M, Lanzone A, Morri C, Albertelli G, Bianchi CN. 2008b. Beyond BACI design reveals the decline of the seagrass Posidonia oceanica induced by anchoring. Marine Pollution Bulletin 56:1637–1645. DOI: 10.1016/j.marpolbul.2008.05.013.
- Montefalcone M, De Falco G, Nepote E, Canessa M, Bertolino M, Bavestrello G, Morri C, Bianchi CN. 2018. Thirty year ecosystem trajectories in a submerged marine cave under changing pressure regime. Marine Environmental Research 137:98–110. DOI: 10.1016/j.marenvres.2018.02.022.
- Montefalcone M, Giovannetti E, Lasagna R, Parravicini V, Morri C, Albertelli G. 2007b. Diachronic analysis of a Posidonia oceanica meadow in the marine protected area of Bergeggi. Biologia Marina Mediterranea 14:82–83.
- Montefalcone M, Giovannetti E, Morri C, Peirano A, Bianchi CN. 2013. Flowering of the seagrass Posidonia oceanica in the NW Mediterranean: Is there a link with solar activity? Mediterranean Marine Science 14:416–423. DOI: 10.12681/mms.529.
- Montefalcone M, Lasagna R, Bianchi CN, Morri C, Albertelli G. 2006b. Anchoring damage on Posidonia oceanica meadow cover: A case study in Prelo cove (Ligurian Sea, NW Mediterranean). Chemistry and Ecology 22:207–217. DOI: 10.1080/02757540600571976.
- Montefalcone M, Morri C, Bianchi CN, Bavestrello G, Piazzi L. 2017. The two facets of species sensitivity: Stress and disturbance on coralligenous assemblages in space and time. Marine Pollution Bulletin 117:229–238. DOI: 10.1016/j.marpolbul.2017.01.072.
- Montefalcone M, Morri C, Parravicini V, Bianchi CN. 2015. A tale of two invaders: Divergent spreading kinetics of the alien green algae Caulerpa taxifolia and Caulerpa cylindracea. Biological Invasions 17:2717–2728. DOI: 10.1007/s10530-015-0908-1.
- Montefalcone M, Morri C, Peirano A, Albertelli G, Bianchi CN. 2007c. Substitution and phase shift within the Posidonia oceanica seagrass meadows of NW Mediterranean Sea. Estuarine, Coastal and Shelf Science 75:63–71. DOI: 10.1016/j.ecss.2007.03.034.
- Montefalcone M, Parravicini V, Bianchi CN. 2011. Quantification of coastal ecosystem resilience. In: Wolanski E, McLusky DS, editors. Treatise on estuarine and coastal science. Waltham, USA: Elsevier Academic Press 10 (Ecohydrology and Restoration). pp. 49–70.
- Montefalcone M, Parravicini V, Vacchi M, Albertelli G, Ferrari M, Morri C, Bianchi CN. 2010a. Human influence on seagrass habitat fragmentation in NW Mediterranean Sea. Estuarine, Coastal and Shelf Science 86:292–298. DOI: 10.1016/j.ecss.2009.11.018.
- Morri C, Bianchi CN. 2001. Recent changes in biodiversity in the Ligurian Sea (NW Mediterranean): Is there a climatic forcing? In: Faranda FM, Guglielmo L, Spezie G, editors. Mediterranean ecosystems: Structure and processes. Milano, Italy: Springer. pp. 375–384.
- Morri C, Bianchi CN, Damiani V, Peirano A, Romeo G, Tunesi L. 1986. L’ambiente marino tra punta della Chiappa e Sestri Levante (Mar Ligure): Profilo ecotipologico e proposta di carta bionomica. Bollettino dei Musei e degli Istituti Biologici dell’Università di Genova 52(suppl.):213–231.
- Morri C, Bianchi CN, Degl’Innocenti F, Diviacco G, Forti S, Maccarone M, Niccolai I, Sgorbini S, Tucci S. 1994. Gradienti fisico-chimici e ricoprimento biologico nella Grotta Marina di Bergeggi (Mar Ligure). Memorie dell’Istituto Italiano di Speleologia ser. II 6:85–94.
- Morri C, Bianchi CN, Di Camillo CG, Ducarme F, Allison WR, Bavestrello G. 2017. Global climate change and regional biotic responses: Two hydrozoan tales. Marine Biology Research 13:573–586. DOI: 10.1080/17451000.2017.1283419.
- Morri C, Montefalcone M, Gatti G, Vassallo P, Paoli C, Bianchi CN. 2019. An alien invader is the cause of homogenization in the recipient ecosystem: A simulation-like approach. Diversity 11:146. DOI: 10.3390/d11090146.
- Morri C, Peirano A, Bianchi CN. 2001. Is the Mediterranean coral Cladocora caespitosa an indicator of climatic change? Archivio di Oceanografia e Limnologia 22:139–144.
- Nepote E, Bianchi CN, Morri C, Ferrari M, Montefalcone M. 2017. Impact of a harbour construction on the benthic community of two shallow marine caves. Marine Pollution Bulletin 114:35–45. DOI: 10.1016/j.marpolbul.2016.08.006.
- Notarbartolo di Sciara G, Agardy T, Hyrenbach D, Scovazzi T, Van Klaveren P. 2008. The Pelagos sanctuary for Mediterranean marine mammals. Aquatic Conservation: Marine and Freshwater Ecosystems 18:367–391. DOI: 10.1002/aqc.855.
- Occhipinti-Ambrogi A, Savini D. 2003. Biological invasions as a component of global change in stressed marine ecosystems. Marine Pollution Bulletin 46:542–551. DOI: 10.1016/S0025-326X(02)00363-6.
- Ontoria Y, González-Guedes E, Sanmartí N, Bernardeau-Esteller J, Ruiz JM, Romero J, Pérez M. 2019. Interactive effects of global warming and eutrophication on a fastgrowing Mediterranean seagrass. Marine Environmental Research 145:27–38. DOI: 10.1016/j.marenvres.2019.02.002.
- Oprandi A, Montefalcone M, Ferrari M, Morri C, Bianchi CN. 2014a. Invasion of the alien green alga Caulerpa racemosa and phase shift within the Posidonia oceanica seagrass meadow of Bergeggi. Biologia Marina Mediterranea 21:75–78.
- Oprandi A, Montefalcone M, Vacchi M, Coppo S, Diviacco G, Morri C, Ferrari M, Bianchi CN. 2014b. Combining modelling and historical data to define the status of Posidonia oceanica meadows. In: Langar H, Bouafif C, Ouerghi A, editors. Proceedings of the 5th Mediterranean symposium on marine vegetation. Tunis, Tunisia: UNEP/MAP–RAC/SPA. pp. 119–124.
- Orsi Relini L. 2010. Non-native marine fish in Italian waters. In: Golani D, Golani-Appelbaum B, editors. Fish invasion of the Mediterranean Sea: Changes and renewal. Sofia, Bulgaria: Pensoft. pp. 267–292.
- Paoli C, Montefalcone M, Morri C, Vassallo P, Bianchi CN. 2017. Ecosystem functions and services of the marine animal forests. In: Rossi S, Bramanti L, Gori A, Orejas C, editors. Marine animal forests: The ecology of benthic biodiversity hotspots. Cham, Switzerland: Springer International. pp. 1271–1312.
- Parravicini V, Donato M, Morri C, Villa E, Bianchi CN. 2008. Date mussel harvesting favours some blennioids. Journal of Fish Biology 73:2371–2379. DOI: 10.1111/jfb.2008.73.issue-10.
- Parravicini V, Guidetti P, Morri C, Montefalcone M, Donato M, Bianchi CN. 2010. Consequences of sea water temperature anomalies on a Mediterranean submarine cave ecosystem. Estuarine, Coastal and Shelf Science 86:276–282. DOI: 10.1016/j.ecss.2009.11.004.
- Parravicini V, Mangialajo L, Mousseau L, Peirano A, Morri C, Francour P, Kulbicki M, Bianchi CN. 2015. Climate change and warm-water species at the north-western boundary of the Mediterranean Sea. Marine Ecology 36:897–909. DOI: 10.1111/maec.12277.
- Parravicini V, Micheli F, Montefalcone M, Morri C, Villa E, Castellano M, Povero P, Bianchi CN. 2013. Conserving biodiversity in a human-dominated world: Degradation of marine sessile communities within a protected area with conflicting human uses. PloS One 8:e75767. DOI: 10.1371/journal.pone.0075767.
- Parravicini V, Rovere A, Vassallo P, Micheli F, Montefalcone M, Morri C, Paoli C, Albertelli G, Fabiano M, Bianchi CN. 2012. Understanding relationships between conflicting human uses and coastal ecosystems status: A geospatial modeling approach. Ecological Indicators 19:253–263. DOI: 10.1016/j.ecolind.2011.07.027.
- Peirano A, Bianchi CN. 1997. Decline of the seagrass Posidonia oceanica in response to environmental disturbance: A simulation-like approach off Liguria (NW Mediterranean Sea). In: Hawkins LE, Hutchinson S, editors. The response of marine organisms to their environments. Southampton, UK: University Press. pp. 87–95.
- Peirano A, Bianchi CN, Savini D, Farina G. 2001. A long-term monitoring activity on a Posidonia oceanica meadow at Monterosso al Mare (Ligurian Sea). Archivio di Oceanografia e Limnologia 22:145–148.
- Peirano A, Cocito S, Banfi V, Cupido R, Damasso V, Farina G, Lombardi C, Mauro R, Morri C, Roncarolo I, Saldaña S, Savini D, Sgorbini S, Silvestri C, Stoppelli N, Torricelli L, Bianchi CN. 2011. Phenology of the Mediterranean seagrass Posidonia oceanica (L.) Delile: Medium and long-term cycles and climate inferences. Aquatic Botany 94:77–92. DOI: 10.1016/j.aquabot.2010.11.007.
- Peirano A, Damasso V, Montefalcone M, Morri C, Bianchi CN. 2005. Effects of climate, invasive species and anthropogenic impacts on the growth of the seagrass Posidonia oceanica (L.) Delile in Liguria (NW Mediterranean Sea). Marine Pollution Bulletin 50:817–822. DOI: 10.1016/j.marpolbul.2005.02.011.
- Peirano A, Morri C, Bianchi CN, Aguirre J, Antonioli F, Calzetta G, Carobene L, Mastronuzzi G, Orrù P. 2004. The Mediterranean coral Cladocora caespitosa: A proxy for past climate fluctuations? Global and Planetary Change 40:195–200. DOI: 10.1016/S0921-8181(03)00110-3.
- Peirano A, Salvati E, Bianchi CN, Morri C. 2000. Long-term change in the subtidal assemblages of Punta Mesco (Ligurian Sea, Italy) as assessed through underwater photography. Porcupine Marine Natural History Society Newsletter 5:9–12.
- Peirano A, Sassarini M. 1992. Analisi delle caratteristiche distributive di alcune facies di substrato duro dei fondali delle Cinque Terre (Mar Ligure). Oebalia 17:523–528.
- Pellerano A. 2016. Il Laboratorio Marino e la Società Ligure Sarda per la Protezione della Pesca. Biologia Marina Mediterranea 23(suppl. 1):1–108.
- Pérez T, Garrabou J, Sartoretto S, Harmelin JG, Francour P, Vacelet J. 2000. Mortalité massive d’invertébrés marins: un événement sans précédent en Méditerranée nord-occidentale. Comptes Rendus de l’Académie des Sciences Série III Sciences de la Vie 323:853–865.
- Pergent G, Bazairi H, Bianchi CN, Boudouresque CF, Buia MC, Calvo S, Clabaut P, Harmelin-Vivien M, Mateo MA, Montefalcone M, Morri C, Orfanidis S, Pergent-Martini C, Semroud R, Serrano O, Thibaut T, Tomasello A, Verlaque M. 2014. Climate change and Mediterranean seagrass meadows: A synopsis for environmental managers. Mediterranean Marine Science 15:462–473. DOI: 10.12681/mms.621.
- Petrillo M, Martorano D, Albertelli G, Della Croce N. 1998. Uova di Engraulis encrasicolus e parametri ambientali nella Zona Pilota di Chiavari, Mar Ligure (1985–1996). Biologia Marina Mediterranea 5:349–360.
- Piazzi L, Atzori F, Cadoni N, Cinti MF, Frau F, Ceccherelli G. 2018. Benthic mucilage blooms threaten coralligenous reefs. Marine Environmental Research 140:145–151. DOI: 10.1016/j.marenvres.2018.06.011.
- Pilczynska J, Cocito S, Boavida J, Serrão E, Queiroga H. 2016. Genetic diversity and local connectivity in the Mediterranean red gorgonian coral after mass mortality events. PloS One 11:e0150590. DOI: 10.1371/journal.pone.0150590.
- Popova E, Yool A, Byfield V, Cochrane K, Coward AC, Salim SS, Gasalla MA, Henson SA, Hobday AJ, Pecl GT, Sauer WH, Roberts MJ. 2016. From global to regional and back again: Common climate stressors of marine ecosystems relevant for adaptation across five ocean warming hotspots. Global Change Biology 22:2038–2053. DOI: 10.1111/gcb.13247.
- Prato G, Barrier C, Francour P, Cappanera V, Markantonatou V, Guidetti P, Mangialajo L, Cattaneo-Vietti R, Gascuel D. 2016. Assessing interacting impacts of artisanal and recreational fisheries in a small marine protected area (Portofino, NW Mediterranean Sea). Ecosphere 7:e01601. DOI: 10.1002/ecs2.1601.
- Privitera D, Giussani V, Isola G, Faimali M, Piazza V, Garaventa F, Cantamessa E, Cattaneo-Vietti R, Chiantore M. 2012. Toxic effects of Ostreopsis ovata on larvae and juveniles of Paracentrotus lividus. Harmful Algae 18:16–23. DOI: 10.1016/j.hal.2012.03.009.
- Puce S, Bavestrello G, Di Camillo CG, Boero F. 2009. Long-term changes in hydroid (Cnidaria, Hydrozoa) assemblages: Effect of Mediterranean warming? Marine Ecology 30:313–326. DOI: 10.1111/mae.2009.30.issue-3.
- Raitsos DE, Beaugrand G, Georgopoulos D, Zenetos A, Pancucci-Papadopoulou AM, Theocharis A, Papathanassiou E. 2010. Global climate change amplifies the entry of tropical species into the Eastern Mediterranean Sea. Limnology and Oceanography 55:1478. DOI: 10.4319/lo.2010.55.4.1478.
- Rastorgueff PA, Bellan-Santini D, Bianchi CN, Bussotti S, Chevaldonné P, Guidetti P, Harmelin JG, Montefalcone M, Morri C, Pérez T, Ruitton S, Vacelet J, Personnic S. 2015. An ecosystem-based approach to evaluate the ecological quality of Mediterranean undersea caves. Ecological Indicators 54:137–152. DOI: 10.1016/j.ecolind.2015.02.014.
- Reid PC, Hari RE, Beaugrand G, Livingstone DM, Marty C, Straile D, Barichivich J, Goberville E, Adrian R, Aono Y, Brown R, Foster J, Groisman P, Hélaouët P, Hsu HH, Kirby R, Knight J, Kraberg A, Li J, Lo TT, Myneni RB, North RP, Pounds JA, Sparks T, Stübi R, Tian Y, Wiltshire KH, Xiao D, Zhu Z. 2016. Global impacts of the 1980s regime shift. Global Change Biology 22:682–703. DOI: 10.1111/gcb.13106.
- Richardson AJ, Schoeman DS. 2019. Sea animals are more vulnerable to warming than are land ones. Nature 569:50–51. DOI: 10.1038/d41586-019-01193-8.
- Rivetti I, Fraschetti S, Lionello P, Zambianchi E, Boero F. 2014. Global warming and mass mortalities of benthic invertebrates in the Mediterranean Sea. PloS One 9:e115655. DOI: 10.1371/journal.pone.0115655.
- Rivoire G. 1991. Mortalité du corail et des gorgones en profondeur au large des côtes provençales. In: Boudoresque CF, Avon M, Gravez V, editors. Les especès marines à protéger en Méditerranée. Marseilles, France: GIS Posidonie. pp. 53–59.
- Rodolfo-Metalpa R, Bianchi CN, Peirano A, Morri C. 2000. Coral mortality in NW Mediterranean. Coral Reefs (Online) 19:24. DOI: 10.1007/s003380050221.
- Rodolfo-Metalpa R, Bianchi CN, Peirano A, Morri C. 2005. Tissue necrosis and mortality of the temperate coral Cladocora caespitosa. Italian Journal of Zoology 72:271–276. DOI: 10.1080/11250000509356685.
- Rodolfo-Metalpa R, Richard C, Allemand D, Bianchi CN, Morri C, Ferrier-Pagés C. 2006. Response of zooxanthellae in symbiosis with the Mediterranean corals Cladocora caespitosa and Oculina patagonica to elevated temperatures. Marine Biology 150:45–55. DOI: 10.1007/s00227-006-0329-x.
- Roghi F, Parravicini V, Montefalcone M, Rovere A, Morri C, Peirano A, Firpo M, Bianchi CN, Salvati E. 2010. Decadal evolution of a coralligenous ecosystem under the influence of human impacts and climate change. Biologia Marina Mediterranea 17:59–62.
- Romano JC, Bensoussan N, Younes W, Arlhac D. 2000. Anomalies thermiques dans les eaux du golfe de Marseille durant l’été 1999. Une explication partielle de la mortalité d’invertébrés fixés. Comptes Rendus de l’Académie des Sciences Série III Sciences de la Vie 323:415–427.
- Rosenberg E, Falkovitz L. 2004. The Vibrio shiloi/Oculina patagonica model system of coral bleaching. Annual Review of Microbiology 58:143–159. DOI: 10.1146/annurev.micro.58.030603.123610.
- Rossi L. 1965. Il coralligeno di Punta Mesco (La Spezia). Annali del Museo Civico di Storia Naturale di Genova 75:144–180.
- Rossi L. 1969. Considerazioni zoogeografiche sul bacino NW del Mediterraneo, con particolare riguardo al Mar Ligure. Archivio Botanico e Biogeografico Italiano XLV-4a serie 14:139–152.
- Rovere A, Parravicini V, Firpo M, Morri C, Bianchi CN. 2011. Combining geomorphologic, biological and accessibility values for marine natural heritage evaluation and conservation. Aquatic Conservation: Marine and Freshwater Ecosystems 21:541–552. DOI: 10.1002/aqc.v21.6.
- Sala E, Aburto-Oropeza O, Paredes G, Parra I, Barrera JC, Dayton PK. 2002. A general model for designing networks of marine reserves. Science 298:1991–1993. DOI: 10.1126/science.1075284.
- Sala E, Ballesteros E, Panagiotis D, Di Franco A, Ferretti F, Foley D, Fraschetti S, Friedlander A, Garrabou J, Guclusoy H, Guidetti P, Halpern BS, Hereu B, Karamanlidis AA, Kizilkaya Z, Macpherson E, Mangialajo L, Mariani S, Micheli F, Pais A, Riser K, Rosenberg AA, Sales M, Selkoe KA, Starr R, Tomas F, Zabala M. 2012. The structure of Mediterranean rocky reef ecosystems across environmental and human gradients, and conservation implications. PloS One 7:e32742. DOI: 10.1371/journal.pone.0032742.
- Sara G, Bianchi CN, Morri C. 2005. Mating behaviour of the newly-established ornate wrasse Thalassoma pavo (Osteichthyes: Labridae) in the Ligurian Sea (north-western Mediterranean). Journal of the Marine Biological Association of the UK 85:191–196. DOI: 10.1017/S0025315405011057h.
- Sara G, Gasparini GP, Morri C, Bianchi CN, Cinelli F. 2003. Reclutamento di gorgonie dopo un episodio di moria di massa in Mar Ligure orientale. Biologia Marina Mediterranea 10:176–182.
- Sarà M. 1964. Distribuzione ed ecologia dei Poriferi in acque superficiali della Riviera ligure di Levante. Archivio Zoologico Italiano 49:181–248.
- Sarà M. 1985. Ecological factors and their biogeographic consequences in the Mediterranean ecosystems. In: Moraitou-Apostolopoulou M, Kiortsis V, editors. Mediterranean marine ecosystems. New York, USA: Plenum Press. pp. 1–17.
- Schiaparelli S, Castellano M, Povero P, Sartoni G, Cattaneo-Vietti R. 2007. A benthic mucilage event in north-western Mediterranean Sea and its possible relationships with the summer 2003 European heatwave: Short term effects on littoral rocky assemblages. Marine Ecology 28:341–353. DOI: 10.1111/mae.2007.28.issue-3.
- Schröder K, Gasparini GP, Tangherlini M, Astraldi M. 2006. Deep and intermediate water in the western Mediterranean under the influence of the eastern Mediterranean Transient. Geophysical Research Letters 33:L21607. DOI: 10.1029/2006GL027121.
- Sgorbini S, Bianchi CN, Degl’Innocenti F, Diviacco G, Forti S, Morri C, Niccolai I. 1988. Méthodologie d’une étude hydrobiologique dans la grotte marine de Bergeggi (mer Ligure). Rapports et Procès-Verbaux des Réunions de la Commission Internationale pour l’Exploration Scientifique de la Mer Méditerranée 31:119.
- Shaltout M, Omstedt A. 2014. Recent sea surface temperature trends and future scenarios for the Mediterranean Sea. Oceanologia 56:411–443. DOI: 10.5697/oc.56-3.411.
- Smale DA, Wernberg T, Oliver ECJ, Thomsen M, Harvey BP, Straub SC, Burrows MT, Alexander LV, Benthuysen JA, Donat MG, Feng M, Hobday AJ, Holbrook NJ, Perkins-Kirkpatrick SE, Scannell HA, Gupta AS, Payne BL, Moore PJ. 2019. Marine heatwaves threaten global biodiversity and the provision of ecosystem services. Nature Climate Change 9:306–312. DOI: 10.1038/s41558-019-0412-1.
- Sparnocchia S, Schiano ME, Picco P, Bozzano R, Cappelletti A. 2006. The anomalous warming of summer 2003 in the surface layer of the Central Ligurian Sea (Western Mediterranean). Annales Geophysicae, European Geosciences Union 24:443–452.
- Thibaut T, Blanfuné A, Boudouresque CF, Personnic S, Ruitton S, Ballesteros E, Bellan-Santini D, Bianchi CN, Bussotti S, Cebrian E, Cheminée A, Culioli JM, Derrien-Courtel S, Guidetti P, Harmelin-Vivien M, Hereu B, Morri C, Poggiale JC, Verlaque M. 2017. An ecosystem-based approach to assess the status of Mediterranean algae-dominated shallow rocky reefs. Marine Pollution Bulletin 117:311–329. DOI: 10.1016/j.marpolbul.2017.01.029.
- Thibaut T, Pinedo S, Torras X, Ballesteros E. 2005. Long-term decline of the populations of Fucales (Cystoseira spp. and Sargassum spp.) in the Albères coast (France, North-western Mediterranean). Marine Pollution Bulletin 50:1472–1489. DOI: 10.1016/j.marpolbul.2005.06.014.
- Tortonese E. 1958. Bionomia marina della regione costiera fra Punta della Chiappa e Portofino (Riviera Ligure di Levante). Archivio di Oceanografia e Limnologia 11:167–210.
- Tortonese E. 1962. Recenti ricerche sul bentos in ambienti litorali del mare Ligure. Pubblicazioni della Stazione Zoologica di Napoli 32(suppl.):99–116.
- Tortonese E. 1971. Natura e naturalisti in Liguria. Atti dell’Accademia Ligure di Scienze e Lettere 28:1–16.
- Tunesi L, Vacchi M. 1994. Considerazioni sul rinvenimento di Caulerpa prolifera (Forsskål) Lamoroux sui fondali del Golfo Tigullio (Mar Ligure). Atti dell’Associazione Italiana di Oceanologia e Limnologia 10:413–419.
- Turley CM. 1999. The changing Mediterranean Sea, a sensitive ecosystem? Progress in Oceanography 44:387–400. DOI: 10.1016/S0079-6611(99)00033-6.
- Ugolini U, Ferdeghini F, Sara G, Morri C, Bianchi CN. 2003. Indagine ecologica sulla grotta sottomarina dell’Isola del Tinetto (Golfo di La Spezia, Mar Ligure orientale): Dati preliminari. Biologia Marina Mediterranea 10:48–57.
- Ulman A, Ferrario J, Occhipinti-Ambrogi A, Arvanitidis C, Bandi A, Bertolino M, Bogi C, Chatzigeorgiou G, Çiçek BA, Deidun A, Ramos-Esplá A, Koçak C, Lorenti M, Martinez-Laiz G, Merlo G, Princisgh E, Scribano G, Marchini A. 2017. A massive update of non-indigenous species records in Mediterranean marinas. PeerJ 5:e3954. DOI: 10.7717/peerj.3954.
- Unsworth RKF, Collier CJ, Waycott M, Mckenzie LJ, Cullen-Unsworth LC. 2015. A framework for the resilience of seagrass ecosystems. Marine Pollution Bulletin 100:34–46. DOI: 10.1016/j.marpolbul.2015.08.016.
- Urban MC, Bocedi G, Hendry AP, Mihoub JB, Pe’er G, Singer A, Bridle JR, Crozier LG, De Meester L, Godsoe W, Gonzalez A, Hellmann JJ, Holt RD, Huth A, Johst K, Krug CB, Leadley PW, Palmer SCF, Pantel JH, Schmitz A, Zollner PA, Travis JMJ. 2016. Improving the forecast for biodiversity under climate change. Science 353:aad8466. DOI: 10.1126/science.aad8466.
- Vacchi M, De Falco G, Simeone S, Montefalcone M, Morri C, Ferrari M, Bianchi CN. 2017. Biogeomorphology of the Mediterranean Posidonia oceanica seagrass meadows. Earth Surface Processes and Landforms 42:42–54. DOI: 10.1002/esp.v42.1.
- Vacchi M, Montefalcone M, Parravicini V, Rovere A, Vassallo P, Ferrari M, Morri C, Bianchi CN. 2014. Spatial models to support the management of coastal marine ecosystems: A short review of best practices in Liguria, Italy. Mediterranean Marine Science 15:189–197. DOI: 10.12681/mms.535.
- Vacchi M, Morri C, Modena M, La Mesa G, Bianchi CN. 2001. Temperature changes and warm-water species in the Ligurian Sea: The case of the ornate wrasse Thalassoma pavo (Linnaeus, 1758). Archivio di Oceanografia e Limnologia 22:149–154.
- Vacchi M, Sara G, Morri C, Modena M, La Mesa G, Guidetti P, Bianchi CN. 1999. Dynamics of marine populations and climate change: Lessons from a Mediterranean fish. Porcupine Marine Natural History Society Newsletter 3:13–17.
- van Putten I, Boschetti F, Ling S, Richards SA. 2019. Perceptions of system-identity and regime shift for marine ecosystems. ICES Journal of Marine Science: fsz058. DOI: 10.1093/icesjms/fsz058.
- Vassallo P, Paoli C, Rovere A, Montefalcone M, Morri C, Bianchi CN. 2013. The value of the seagrass Posidonia oceanica: A natural capital assessment. Marine Pollution Bulletin 75:157–167. DOI: 10.1016/j.marpolbul.2013.07.044.
- Venturini S, Campodonico P, Cappanera V, Fanciulli G, Cattaneo-Vietti R. 2017. Recreational fishery in Portofino Marine Protected Area (MPA), Italy: Some implications for the management. Fisheries Management and Ecology 24:382–391. DOI: 10.1111/fme.12241.
- Venturini S, Merotto L, Campodonico P, Cappanera V, Fanciulli G, Cattaneo-Vietti R. 2019. Recreational fisheries within the Portofino MPA and surrounding areas (Ligurian Sea, Western Mediterranean Sea). Mediterranean Marine Science 20:142–150.
- Verdura J, Linares C, Ballesteros E, Coma R, Uriz MJ, Bensoussan N, Cebrian E. 2019. Biodiversity loss in a Mediterranean ecosystem due to an extreme warming event unveils the role of an engineering gorgonian species. Scientific Reports 9:5911. DOI: 10.1038/s41598-019-41929-0.
- Vezzulli L, Pezzati E, Moreno M, Fabiano M, Pane L, Pruzzo C, VibrioSea Consortium. 2009. Benthic ecology of Vibrio spp and pathogenic Vibrio species in a coastal Mediterranean environment (La Spezia Gulf, Italy). Microbial Ecology 58:808–818. DOI: 10.1007/s00248-009-9542-8.
- Vezzulli L, Previati C, Pruzzo C, Marchese A, Bourne DG, Cerrano C, VibrioSea Consortium. 2010. Vibrio infections triggering mass mortality events in a warming Mediterranean Sea. Environmental Microbiology 12:2007–2019. DOI: 10.1111/j.1462-2920.2010.02209.x.
- Vignudelli S, Cipollini P, Astraldi M, Gasparini GP, Manzella G. 2000. Integrated use of altimeter and in situ data for understanding the water exchanges between the Tyrrhenian and Ligurian Seas. Journal of Geophysical Research Oceans 105:19649–19663. DOI: 10.1029/2000JC900083.
- Zenetos A, Çinar ME, Pancucci-Papadopoulou MA, Harmelin JG, Furnari G, Andaloro F, Bellou N, Streftaris N, Zibrowius H. 2005. Annotated list of marine alien species in the Mediterranean with records of the worst invasive species. Mediterranean Marine Science 6:63–118. DOI: 10.12681/mms.186.