Abstract
Neuropeptides play a central role in the regulation of reproduction, growth, development, and various other physiological processes. In our study, 26 neuropeptide precursors were identified from the pleuropedal and cerebral ganglia of Pacific abalone using transcriptome analysis. Of them, two (neuromacin and neuroglian) were potentially novel and two (myomodulin and FMRFamide) were involved in the reproductive regulation of mollusks. A BLAST search indicated that most of these neuropeptides exhibited the highest homologies with neuropeptides of other protostomian species. Myomodulin, FMRFamide, and molluscan insulin-related peptides shared 87%, 72%, and 88% sequence identities with homologous peptides of the tropical gastropod mollusk, Haliotis asinina. In silico analysis revealed that these identified neuropeptide precursors were likely to be extracellular secreted proteins. Alignment of multiple cerebrin, FFamide, and insulin-related peptide sequences illustrated that most of their biologically active residues were highly conserved with other invertebrate homologous neuropeptide residues. Three-dimensional (3D) structures of adipokinetic hormone, allatostain (A, B, and C), allatotropin, cerebrin, clionin, conopressin, elevenin, and neuromycin precursors in H. discus hannai exhibited a helix-loop-helix structure. Based on phylogenetic analysis, buccalin A1 and A2, allatostain B, and FCAP neuropeptide precursors of Pacific abalone formed a clade with other gastropod and bivalve mollusks neuropeptide precursors. This study provides a novel insight for further functional studies of abalone and other gastropod mollusks.
Introduction
Neuropeptides are ubiquitous neuron-secreted peptides that can act as hormones, neurotransmitters, and neuromodulators (Jékely Citation2013). They are the largest and most diverse class of endogenous proteinaceous molecules within the nervous system (Burbach Citation2011). These intracellular signaling molecules are derived from prepropeptides that are typically produced by protein synthesis within neurons or neurosecretory cells (Nässel & Larhammar Citation2013). Neuropeptides and peptide hormones are widely distributed throughout the nervous and endocrine systems of protostomes and deuterostomes. The biological activity of neuropeptides for regulating target cell functions is mainly mediated by G protein-coupled receptors (GPCRs) or through the phospholipase-phosphatidylinositol pathway involving a different group of second messengers (Strand Citation2003; Tanaka et al. Citation2003). Neuropeptides are synthesized from a larger, inactive precursor molecule that requires proteolytic processing sites to generate active neuropeptides that then act on target cells by interacting with specific signal-transducing membrane receptors (Zupanc Citation1996). Neuropeptides are crucial for regulating a plethora of cellular and physiological functions involved in feeding, digestion, excretion, circulation, and reproduction (Nässel & Larhammar Citation2013). These endogenous active substances play key roles in the regulation, formation, maturation, and release of gametes in both vertebrates and invertebrates (Hartenstein Citation2006).
Many studies related to neuropeptides have been performed. Some of the earliest studies were the first to identify and characterize oxytocin and vasopressin neuropeptides (Du Vigneaud et al. Citation1953; Popenoe & du Vigneaud Citation1954). Two peptides of the vasopressin-oxytocin family have been identified and characterized in marine gastropod mollusks (Cruz et al. Citation1987). In addition, neuropeptides have been identified from Lottia gigantea (Veenstra Citation2010), Deroceras reticulatum (Ahn et al. Citation2017), Charonia tritonis (Bose et al. Citation2017), and Patinopecten yessoensis (Zhang et al. Citation2018), all of which provide valuable information to understand the mechanisms of neuroendocrine regulation in mollusks.
The abalone H. discus hannai is an ecologically and economically important marine species that is naturally distributed in Korea, Japan, China, and Taiwan. Several recent studies have identified a number of reproductive and metabolic genes in H. discus hannai (Mendoza-Porras et al. Citation2014; Kim et al. Citation2017). Additionally, neuropeptide precursors that influence sexual maturation in female Pacific abalone have been recently reported by Kim et al. (Citation2019). In the present study, neuropeptide transcripts were identified and characterized from the pleuropedal and cerebral ganglia of H. discus hannai to provide valuable resources for future neuroendocrine research in abalone species.
Materials and methods
Sample collection
In July 2018, two-year-old female abalone (H. discus hannai, 10.5 cm in shell length and 148.2 g in body weight) adults were collected from Jindo Island (South Korea) and transferred to the laboratory in the Department of Fisheries Science, Chonnam National University. The abalone was euthanized by immersion in an anesthetic solution (ethyl 3-aminobenzoate methane sulfonate, MS-222) to obtain neural ganglia tissues (pleuropedal ganglion, cerebral ganglion). After collection, samples were immediately frozen in liquid N2 and stored at −80°C for subsequent use.
RNA extraction and transcriptome analysis
Total RNA derived from the ganglion tissues were extracted using an RNeasy mini kit (Qiagen, Hilden, Germany) and then treated with RNase-free DNase (Promega, Madison, WI, USA) to eliminate genomic DNA according to the manufacturer’s instructions. RNA concentration and purity were determined using a NanoDrop® NP 1000 spectrophotometer, and RNA integrity was evaluated by agarose gel electrophoresis. A cDNA library was constructed using a TruSeq Standard mRNA Sample Preparation Kit (Illumina, CA, USA) following the manufacturer’s recommendations. It was then subjected to paired end sequencing (2 × 100 bp) to produce raw reads on an Illumina HiSeq 2500 platform (Illumina, CA, USA).
Raw reads were filtered using a homemade Perl script to remove low-quality reads that contained greater than 10% skipped bases (marked as ‘N’s) or base quality scores less than 20. Filtered reads were mapped to reference genomes of related species (abalone and other molluscan species) using TopHat aligner (Trapnell Citation2009).
Sequence analysis
The amino acid sequences of neuropeptide or peptide hormones of mollusks and other invertebrates were used as queries for BLAST analysis to search for neuropeptide transcripts of H. discus hannai. The open reading frame (ORF) was used to translate sequences and then input into BLASTp as query sequences to identify neuropeptide orthologues. In silico analysis of the identified neuropeptide was performed using multiple online software tools. Basic Local Alignment Search Tool (BLASTP) (http://www.ncbi.nlm.nih.gov/BLAST/) was used to determine the homologies of H. discus hannai neuropeptide transcript to neuropeptide transcripts of other species. Signal peptides were predicted using SignalP 4.1 (www.cbs.dtu.dk/services/SignalP/). Prepropeptide cleavage sites were predicted with NeuroPred (http://stagbeetle.animal.uiuc.edu/cgi-bin/neuropred.py) (Southey et al. Citation2006). Cysteine residues of disulfide bridges were predicted with CYSPRED (Fariselli et al. Citation1999). Primary structures and subcellular localization of proteins were detected using ProtParam (http://expasy.org/tools/protparam.html) and Protcomp (http://linux1. softberry.com/berry.phtml), respectively. Multiple alignments of deduced amino acid sequences of neuropeptides were conducted using Clustal Omega (Sievers et al. Citation2011).
Phylogenetic analysis and three-dimensional protein structure
Neuropeptide precursors homologous sequences from invertebrates were retrieved to construct phylogenetic trees. The neighbor-joining algorithm was used to build phylogenetic trees using MEGA software (version 6.06) (Tamura et al. Citation2013). Numbers reported on branches were obtained using a bootstrap analysis conducted on 1000 replicates to evaluate the significance of nodes. 3D structures of neuropeptide precursors were predicted using the I-TASSER server (Yang et al. Citation2015). Visualization of the predicted 3D structure was performed using Chimera software (https://www.cgl.ucsf.edu/chimera/).
Results and discussion
Transcriptome sequencing and assembly
The ganglia transcriptome of H. discus hannai produced a total of 34,580,812 paired-end reads. After the quality filtering step, 33,548,676 clean reads were obtained, representing 97.01% recovery of all sequencing reads. Finally, the ganglia transcriptome was assembled into 41,287 transcripts with an average length of 958.71 bp. A total of 11,819 sequences were annotated either by trinotate analysis or by BLASTn against a nt database with E values below 10−15.
Identification of neuropeptide precursors
Based on NCBI BLAST search, 26 cDNAs encoding full or partial neuropeptide transcripts were identified from the pleuropedal and cerebral ganglia of Pacific abalone, H. discus hannai (). These identified genes were homologous to previously identified neuropeptide precursors of other molluscan species. Myomodulin, FMRFamide, and molluscan insulin-related peptide exhibited 87%, 72%, and 88% identities with homologous peptides of H. asinina. A catalogue of neuropeptide precursor constructed in this study and similar characterization of neuropeptides from other molluscan species are shown in Supplementary file 1.
Table I. Summary of transcripts encoding neuropeptides within the ganglia of Haliotis discus hannai. ORF = open reading frame; SP = signal peptide; MW = molecular weight; pI = isoelectric point
Adipokinetic hormone (AKH)
AKH is a small neuropeptide synthesized and stored by neurosecretory cells. It plays a pivotal role in the mobilization of both carbohydrates and lipids. This neuropeptide was first reported as an insect metabolic neuropeptide involved in the mobilization of energy substrates during physiological activities (Gäde Citation2009). In mollusks, AKH has been identified in Crassostrea gigas (Dubos et al. Citation2017) and D. reticulatum (Ahn et al. Citation2017). An 84-residue AKH precursor protein was identified that had a predicted N-terminal signal peptide and an AKH amidated peptide (pQVSFSTNWGSamide). A similar AKH mature peptide has been reported in C. gigas (Dubos et al. Citation2017). For the AKH precursor protein, its 3D structure was characterized by two alpha helices separated by a loop (helix-loop-helix structure, HLH).
Allatostatin A or buccalin
Allatostatin A is an L-amide, biologically active neuropeptide that functions in muscle contraction. This neuropeptide was first isolated from Aplysia californica (Miller et al. Citation1993). It has been also reported in L. gigantea (Veenstra Citation2010), C. gigas (Stewart et al. Citation2014), D. reticulatum (Ahn et al. Citation2017), and P. yessoensis (Zhang et al. Citation2018). In H. discus hannai, two buccalin transcripts, allatostatin A1 and A2, were identified that encode 24 and 30-residue signal peptides and 3 and 5 copies of the mature peptide, respectively. The open reading frames (ORF) of allatostatin A1 and A2 cDNA sequences encoded deduced proteins of 114 and 129 amino acids with theoretical molecular mass of 12.60 and 14.06 kDa, respectively. The peptide precursor contains GxLamide at the C-terminal end, which is in agreement with a previous report on allatostatins A in D. reticulatum and P. yessoensis (Ahn et al. Citation2017; Zhang et al. Citation2018). Phylogenetic analysis revealed that buccalin precursors A1 and A2 of H. discus hannai were most closely related to D. reticulatum buccalin A1 and C. virginica buccalin-like neuropeptide precursors, respectively (). 3D structures of allatostatin A1 revealed multiple alpha helices separated by a loop. The long helix contained 11 amino acids, and the numbers of amino acids involved in the N-terminal and C-terminal alpha helices were 4 and 7 amino acids, respectively.
Figure 1. Phylogenetic tree of allatostatin (buccalin) A and B neuropeptide precursors. The neighbor-joining method was employed to construct the phylogenetic tree using MEGA 6 based on deduced amino acid sequence similarities. Values provided at the nodes indicate bootstrap percentages for the given split among 1000 replicates
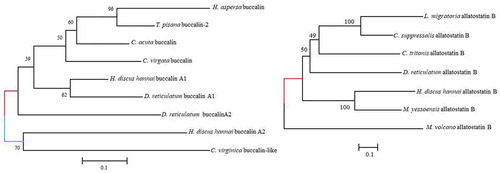
Allatostatin B
The first molluscan allatostatin B was isolated from the ganglia of the African giant snail (Achatina fulica). This neuropeptide is also called WWamide due to the presence of an N-terminal Trp (W) and a C-terminal Trp (W)-amide. In H. discus hannai, allatostatin B was identified. It was found to encode a 25-residue signal peptide followed by four putative allatostatin B peptides that contained conserved W residues at both ends, consistent with the results of previous studies (Ahn et al. Citation2017; Zhang et al. Citation2018). A phylogenetic tree analysis of allatostatin B was constructed, showing that allatostatin B H. discus hannai clustered strongly with M. yessoensis (). 3D structures of allatostatin B were characterized by multiple alpha helices separated by a helix-loop-helix structure.
Allatostatin C
Allatostatin C is a vertebrate somatostatin homolog. It was first discovered in the moth, Manduca sexta (Veenstra Citation2009). It is characterized by two conserved Cys residues surrounding a hexapeptide. Here, we report an allatostatin C preprohormone that is 101 amino acids in length. It contains one copy of the mature peptide. Its cysteine residues at positions Cys-91 and Cys-98 are highly conserved with those of other homologs of invertebrates. 3D structures revealed multiple alpha helices exhibiting a typical HLH structure. The long loop was formed by 24 amino acids and the numbers of amino acids involved in the N-terminal and C-terminal helices were 6 and 8, respectively.
Allatotropin
Allatotropin is an amidated tridecapeptide neurohormone that was originally described as a regulator of juvenile hormone production, it was isolated from the nervous system of the lepidopteran, M. sexta (Kataoka et al. Citation1989). The encoded allatotropin peptide isolated from H. discus hannai shared 50% and 46% sequence identities with those of allatotropin peptide precursors of C. tritonis and D. reticulatum, respectively. In the predicted secondary structures of H. discus hannai allatotropin precursor, multiple alpha helices were observed, with a HLH structure.
Bursicon and partner of bursicon
Bursicon is a heterodimer glycoprotein hormone that belongs to a cysteine-knot protein composed of an α subunit and a β subunit (Honegger et al. Citation2008). This peptide hormone is crucial for regulating cuticle hardening during metamorphosis (Chung et al. Citation2012). Both α and β subunits of bursicon have been reported in D. reticulatum (Ahn et al. Citation2017), P. fucata, and C. gigas (Stewart et al. Citation2014). In H. discus hannai, full-length sequences of bursicon and partner of bursicon encoding 18 and 22-residue signal peptides, respectively, were identified. Bursicon contained six cysteine residues (39, 67, 88, 90,105, and 125). The partner of bursicon contained five residues (54, 89, 93, 112, and 128). They are likely to form disulfide bridges.
Bradykinin-like neuropeptide
The presence of the bradykinin neuropeptide has been reported in the left upper quadrant cells in A. californica. These cells can regulate some renal functions (Wickham & DesGroseillers Citation1991). A bradykinin-like transcript encoding a putative 14.78 kDa precursor peptide containing a signal peptide (19-aa) and potential proteolytic cleavage sites was identified in H. discus hannai. It could generate smaller mature peptides. A BLAST search indicated that this protein shared 31% sequence identities with bradykinin-like neuropeptide precursor of A. californica.
Cerebrin
Cerebrin was first identified from the cerebral ganglia of A. californica. This protein was found to be involved in feeding behavior (Li & Floyd Citation2001). A single copy of a predicted 17-residue C-terminally amidated peptide was identified from the H. discus hannai transcriptome. Sequence alignment of bioactive peptides revealed that Gly, Asp, and two Asn residues at positions 70, 73, 68 and 77, respectively, were highly conserved among different mollusks (). The 3D configuration of this precursor revealed multiple alpha helices possessing the typical HLH structure. It contained 3 and 9 amino acids in its N-terminal and C-terminal, respectively.
Clionin
A potential novel neuropeptide was identified from the transcriptome analysis of H. discus hannai. It amino acid sequences shared 94% identities with those of the clionin precursor of Tridachiella diomedea. Its open reading frame possessed a deduced peptide sequence of 104 amino acids with molecular weight and isoelectric point of 11.79 kDa and 5.89, respectively. An NH2-terminal signal peptide (24-residue) was predicted from this transcript (). Two cysteine residues at positions 46 and 64 are likely to form a disulfide bond. In contrast, three putative disulfide bonds were detected in Sepia officinalis (Zatylny-Gaudin et al. Citation2016). In silico analysis (Protcomp, http://linux1.softberry.com/berry.phtml) revealed that this neuropeptide precursor might be an extracellular secretory protein. For clionin, the 3D structure was characterized by multiple alpha helices separated by a loop.
Conopressin
Conopressin is a nonapeptide amide that causes contraction of the vas deferens in the pond snail, Lymnaea stagnalis (Van Kesteren et al. Citation1992). This neuropeptide was previously isolated from Conus geographus. It shared sequence identities with those of vasopressin-oxytocin peptides of vertebrates (Cruz et al. Citation1987). The conopressin gene of the Pacific abalone encodes a 25-residue signal peptide followed by one bioactive peptide (FIRNCPPGamide), and this is in agreement with the previous report (Ahn et al. Citation2017). An NCBI BLAST search indicated that this precursor protein shared 43% and 31% sequence identities with sequences of Octopus bimaculoides and L. stagnalis precursors, respectively. The HLH structure was formed by 7-aa residues of the N-terminal and 4-aa residues of the C-terminal.
Elevenin
The elevenin peptide was first reported in bag cells and abdominal ganglion neurons L11 in A. californica (Taussig et al. Citation1984). A 120-residue elevenin precursor was detected in H. discus hannai. It had a predicted N-terminal signal peptide (26-residue) and a mature peptide with two conserved cysteine residues. Its 3D predicted model showed the classical HLH structure. The long loop was formed by 17-aa adjacent to the C-terminal tail.
Enterin
Enterin is a nonapeptide and decapeptide composed of an FVamide sequence at the C terminus. Of 20 different enterin peptides, 35 copies of enterin peptides have been isolated from the gut and central nervous system of A. californica (Furukawa et al. Citation2001). The enterin precursor of H. discus hannai had a 247-aa region encoding a signal peptide and five copies of the mature peptide that shared HSFVamide sequences at the C-terminal end. Conversely, the structure of the enterin precursor deduced from cDNA cloning in L. gigantea exhibited eight copies of the peptide, seven of which possessed a HRFVamide at the C-terminal end (Veenstra Citation2010).
Feeding circuit-activating peptide (FCAP)
Feeding circuit-activating neuropeptide (FCAP) was first identified and characterized from the cerebral-buccal connective tissue of A. californica, encoding multiple copies of eight different FCAPs (Sweedler et al. Citation2002). In H. discus hannai, one full-length precursor was identified. It had 107-aa with a 24-residue signal peptide and 3 copies of the FCAP like peptide. Two FCAP precursors containing 7 putative FCAPs have been reported in D. reticulatum (Ahn et al. Citation2017). Phylogenetic analysis revealed that the FCAP precursor of H. discus hannai was closely grouped with FCAP precursor 2 of D. reticulatum ().
FFamide
FFamide has been reported in some mollusks, including gastropods. It was first identified in L. stagnalis (Li et al. Citation1995), L. gigantea (Veenstra Citation2010), Theba pisana (Adamson et al. Citation2015), and the cephalopod S. officinalis (Zatylny-Gaudin et al. Citation2016). This amide peptide is crucial for modulating the transfer rate of semen within the vas deferens. A single copy of C-terminally amidated FF amide precursor (GLRPGMNSLFFamide) was predicted in the H. discus hannai transcriptome. Sequence alignment of bioactive peptides revealed that H. discus hannai and D. reticulatum FFamide-1 shared the same residues (). The characteristic Leu/Met and Arg/Asn substitutions were observed in FFamide of M. yessoensis (Zhang et al. Citation2018).
FMRFamide
The tetrapeptide FMRFamide is widely distributed throughout the animal kingdom. The molluscan FMRFamide precursor participates in a variety of physiological mechanisms such as heart activity, amylase secretion, feeding, and reproduction (Jakobs & Schipp Citation1992; Favrel et al. Citation1994; Santama et al. Citation1994). It is also involved in regulating egg capsule secretion and oocyte transport in the oviduct (Henry et al. Citation1999). In this study, the FMRF amide precursor encoding a 28-residue signal peptide and 7 copies of FMRF tetrapeptides flanked by multiple cleavage sites was found. A BLAST search revealed that this precursor exhibited 73% and 66% sequence identities with sequences of H. asinina and C. gigas FMRF neuropeptide precursors, respectively.
Insulin-related peptide
Numerous insulin-like peptides have been identified in mollusks, especially in gastropods (Smit et al. Citation1988; Floyd et al. Citation1999; Veenstra Citation2010; Ahn et al. Citation2017) and bivalves (Cherif-Feildel et al. Citation2018). These neuropeptides are involved in the growth and glucose metabolism (Ebberink et al. Citation1989). They also play a role in mediating germinal cell proliferation and maturation (Monnier & Bride Citation1995). Three insulin-like precursors (Insulin-like 2A, 2B, and MIP-related peptide) were identified in H. discus hannai. Insulin-like 2A, 2B precursor, and MIP-related peptide contained 142, 124, 118 amino acid residues, respectively, with a predicted N-terminal signal peptide and two insulin-like domains A and B (). Alignment of multiple sequences illustrated that the characteristic cysteine residues of these identified insulin-like precursors were highly conserved with other molluscan insulin-like peptides.
Mytilus inhibitory peptides (MIPs)/PxFVamide
Mytilus inhibitory peptides (MIPs) have been identified from the pedal ganglia of Mytilus edulis. (Hirata et al. Citation1988). These peptides have a potent inhibitory effect on retractor muscle contractions in these animals (Tetsuya et al. Citation1992). A MIP transcript encoding a precursor of 371-aa, with a 21-aa signal peptide and 16 copies of mature peptide processed from multiple mono or dibasic cleavage sites, was identified in H. discus hannai.
Myomodulin
Myomodulin is considered to be an important neural cotransmitter in mollusks that plays a role in innervating the male reproductive system of L. stagnalis (De Lange et al. Citation1998; Koene Citation2010). This neuropeptide was initially identified in A. californica and L. stagnalis. It has been reported in many gastropod and bivalve mollusks. In the present study, two full-length myomodulin transcripts encoding multiple copies of myomodulin-like peptides possessing a consensus MLRLamide at the C-terminal end (Miller et al. Citation1993; Kellett et al. Citation1996) were found. The myomodulin precursor of H. discus hannai encodes 16 different myomodulin peptides with 7 copies of the PMNMLRLamide, while seven different peptides with 7 copies of the PMNMLRLamide in H. asinina have been reported (York et al. Citation2012).
Neuromacin-like protein
Neuromacin is a cysteine-rich antimicrobial peptide that is only expressed in the nervous system (Schikorski et al. Citation2008). It exhibits potent activity against bacteria. Molluscan neuromycin-like peptide has been observed in the central nervous system of A. californica (Moroz et al. Citation2006). In H. discus hannai, neuromacin-like protein is a 80-residue precursor protein comprising a predicted N-terminal signal peptide (19-residue), two copies of mature peptide, and six cysteines residues (residues 21, 28, 47, 57, 74, and 76) that are likely to form disulfide bridges. BLAST analysis results revealed that this precursor shared 59% sequence identities with sequences of a neuromycin-like protein isolated from Stylophora pistillata. Its predicted 3D structure exhibited HLH structure possessing long N- and C-termini ().
Neuroglian
Neuroglian is an extracellular cell adhesion molecule that plays an essential role in synaptic stability (Harris et al. Citation2016). In the present study, a 259-amino acid residue neuroglian precursor comprising a predicted N-terminal signal peptide (21-residue), two copies of the mature peptide, and four cysteines residues that might form intramolecular disulfide bridges was found. A potential N-linked glycosylation site was detected in this precursor.
PRQFVamide
PRQFVamide was identified from the CNS and gut of A. californica. This protein is crucial for regulating the feeding system (Furukawa et al. Citation2003). The structure of this precursor is composed of 33 copies of the PRQFVamide and four related pentapeptides with an N-terminal signal peptide. This precursor is highly expressed within the abdominal ganglion of A. californica (Furukawa et al. Citation2003). In H. discus hannai, a PRQFVamide comprised of a 25-residue signal peptide and 3 copies of the PRQFVamide separated by multiple dibasic cleavage sites was identified. This precursor shared 83% and 48% amino acid sequence identities with those of D. reticulatum and A. californica precursors, respectively.
Conclusion
In the present study, we sequenced and analyzed 26 neuropeptide precursors from the Pacific abalone. Of them, two (neuromacin and neuroglian) were potentially novel transcripts. These neuropeptide transcripts shared the highest sequence similarities with those of other bivalve and gastropod mollusks. This study will aid future physiological and behavioral studies of abalone species.
Acknowledgements
This research was a component of the project titled ‘ Development of technology for abalone aquaculture using sperm cryopreservation (Grant No. 2018-2129)’ funded by the Ministry of Oceans and Fisheries, Korea.
Disclosure statement
No potential conflict of interest was reported by the authors.
Additional information
Funding
References
- Adamson KJ, Wang T, Zhao M, Bell F, Kuballa AV, Storey KB, Cummins SF. 2015. Molecular insights into land snail neuropeptides through transcriptome and comparative gene analysis. BMC Genomics 16:1–15. DOI: 10.1186/s12864-015-1510-8.
- Ahn SJ, Martin R, Rao S, Choi MY. 2017. Neuropeptides predicted from the transcriptome analysis of the gray garden slug Deroceras reticulatum. Peptides 93:51–65. DOI: 10.1016/j.peptides.2017.05.005.
- Bose U, Suwansa-Ard S, Maikaeo L, Motti CA, Hall MR, Cummins SF. 2017. Neuropeptides encoded within a neural transcriptome of the giant triton snail Charonia tritonis, a crown-of-thorns starfish predator. Peptides 98:3–14. DOI: 10.1016/j.peptides.2017.01.004.
- Burbach JP. 2011. What are neuropeptides? Methods in Molecular Biology 789:1–36. DOI: 10.1007/978-1-61779-310-3_1.
- Cherif-Feildel M, Berthelin CH, Adeline B, Rivière G, Favrel P, Kellner K. 2018. Molecular evolution and functional characterization of insulin-related peptides in molluscs: Contributions of Crassostrea gigas genomic and transcriptomic-wide screening. General and Comparative Endocrinology 22:546–550. DOI: 10.1016/j.ygcen.2018.10.019.
- Chung JS, Katayama H, Dircksen H. 2012. New functions of arthropod bursicon: Inducing deposition and thickening of new cuticle and hemocyte granulation in the blue crab, Callinectes sapidus. PLoS ONE 7:e46299. DOI: 10.1371/journal.pone.0046299.
- Cruz LJ, De Santos V, Zafaralla GC, Ramilo CA, Zeikus R, Gray WR, Olivera BM. 1987. Invertebrate vasopressin/oxytocin homologs. Characterization of peptides from Conus geographus and Conus straitus venoms. The Journal of Biological Chemistry 262:5821–15824.
- De Lange RP, Joosse J, Van Minnen J. 1998. Multi-messenger innervation of the male sexual system of Lymnaea stagnalis. The Journal of Comparative Neurology 390:564–577. DOI: 10.1002/(ISSN)1096-9861.
- Du Vigneaud V, Ressler C, Trippett S. 1953. The sequence of amino acids in oxytocin, with a proposal for the structure of oxytocin. The Journal of Biological Chemistry 205:949–957.
- Dubos M-P, Bernay B, Favrel P. 2017. Molecular characterization of an adipokinetic hormone-related neuropeptide (AKH) from a mollusk. General and Comparative Endocrinology 243:15–21. DOI: 10.1016/j.ygcen.2016.11.002.
- Ebberink RHM, Smit AB, Van Minnen J. 1989. The insulin family: Evolution of structure and function in vertebrates and invertebrates. The Biological Bulletin 177:176–182. DOI: 10.2307/1541928.
- Fariselli P, Riccobelli P, Casadio R. 1999. Role of evolutionary information in predicting the disulfide bonding state of cysteines in proteins. Proteins 36:340–346. DOI: 10.1002/(SICI)1097-0134(19990815)36:3<340::AID-PROT8>3.0.CO;2-D.
- Favrel P, Giard W, Benlimane N, Boucaud-Camou E, Henry MA. 1994. A new biological activity for the neuropeptide FMRFamide: Experimental evidence for a secretagogue effect on amylase secretion in the scallop Pecten maximus. Experientia 50:1106−1110. DOI: 10.1007/BF01923468.
- Floyd PD, Li L, Rubakhin SS, Sweedler JV, Horn CC, Kupfermann I. 1999. Insulin prohormone processing, distribution, and relation to metabolism in Aplysia californica. The Journal of Neuroscience 19:7732–7741. DOI: 10.1523/JNEUROSCI.19-18-07732.1999.
- Furukawa Y, Nakamaru K, Sasaki K, Fujisawa Y, Minakata H, Ohta S, Morishita F, Matsushima O, Li L, Alexeeva V, Ellis TA, Dembrow NC, Jing J, Sweedler JV, Weiss KR, Vilim FS. 2003. PRQFVamide, a novel pentapeptide identified from the CNS and gut of Aplysia. Journal of Neurophysiology 89:3114–3127. DOI: 10.1152/jn.00014.2003.
- Furukawa Y, Nakamaru K, Wakayama H, Fujisawa Y, Minakata H, Ohta S, Morishita F, Matsushima O, Li L, Romanova E, Sweedler JV, Park JH, Romero A, Cropper EC, Dembrow NC, Jing J, Weiss KR, Vilim FS. 2001. The enterins: A novel family of neuropeptides isolated from the enteric nervous system and CNS of Aplysia. The Journal of Neuroscience 21:8247–8261. DOI: 10.1523/JNEUROSCI.21-20-08247.2001.
- Gäde G. 2009. Peptides of the adipokinetic hormone/red pigment-concentrating hormone family: A new take on biodiversity. Annals of the New York Academy of Sciences 1163:125–136. DOI: 10.1111/j.1749-6632.2008.03625.x.
- Harris KP, Zhang YV, Piccioli ZD, Perrimon N, Littleton JT. 2016. The postsynaptic t-SNARE Syntaxin 4 controls traffic of Neuroligin 1 and Synaptotagmin 4 to regulate retrograde signaling. Elife 5:e13881. DOI: 10.7554/eLife.13881.
- Hartenstein V. 2006. The neuroendocrine system of invertebrates: A developmental and evolutionary perspective. The Journal of Endocrinology 190:555–570. DOI: 10.1677/joe.1.06964.
- Henry J, Zatylny C, Boucaud-Camou E. 1999. Peptidergic control of egg-laying in the cephalopod Sepia officinalis: Involvement of FMRFamide and FMRFamide-related peptides. Peptides 20:1061−1070. DOI: 10.1016/S0196-9781(99)00102-3.
- Hirata T, Kubota I, Iwasawa N, Takabatake I, Ikeda T, Muneoka Y. 1988. Structures and actions of Mytilus inhibitory peptides. Biochemical and Biophysical Research Communication 152:1376–1382. DOI: 10.1016/S0006-291X(88)80437-6.
- Honegger H-W, Dewey EM, Ewer J. 2008. Bursicon, the tanning hormone of insects: Recent advances following the discovery of its molecular identity. The Journal of Comparative Physiology A, Neuroethology, Sensory Neural and Behavioral Physiology 194:989–1005. DOI: 10.1007/s00359-008-0386-3.
- Jakobs PM, Schipp R. 1992. The electrocardiogram of Sepia officinalis L. (Cephalopoda: Coleoida) and its modulation by neuropeptides of the FMRFamide group. Comparative Biochemistry and Physiology, C: Comparative Pharmacology 103:399−402. DOI: DOI.10.1016/0742-8413(92)90028-6.
- Jékely G. 2013. Global view of the evolution and diversity of metazoan neuropeptide signaling. Proceedings of the National Academy of Sciences of the United States of America 110:8702–8707. DOI: 10.1073/pnas.1221833110.
- Kataoka H, Toschi A, Li JP, Carney RL, Schooley DA, Kramer SJ. 1989. Identification of an allatotropin from adult Manduca sexta. Science 243:1481–1483. DOI: 10.1126/science.243.4897.1481.
- Kellett E, Perry SJ, Santama N, Worster BM, Benjamin PR, Burke JF. 1996. Myomodulin gene of Lymnaea: Structure, expression, and analysis of neuropeptides. The Journal of Neuroscience 16:4949−4957. DOI: 10.1523/JNEUROSCI.16-16-04949.1996.
- Kim MA, Markkandan K, Han N-Y, Park J-M, Lee JS, Lee H, Sohn YC. 2019. Neural ganglia transcriptome and peptidome associated with sexual maturation in female pacific abalone (Haliotis discus hannai). Genes 268:1–21. DOI: 10.3390/genes10040268.
- Kim MA, Rhee JS, Kim TH, Lee JS, Choi AY, Choi BS, Choi IY, Sohn YC. 2017. Alternative splicing profile and sex-preferential gene expression in the female and male Pacific Abalone Haliotis discus hannai. Genes 8:1–16. DOI: 10.3390/genes8030099.
- Koene JM. 2010. Neuro-endocrine control of reproduction in hermaphroditic freshwater snails: Mechanisms and evolution. Frontiers in Behavioral Neuroscience 4:167. DOI: 10.3389/fnbeh.2010.00167.
- Li KW, El Filali Z, Van Golen FA, Geraerts WP. 1995. Identification of a novel amide peptide, GLTPNMNSLFF-NH2, involved in the control of vas deferens motility in Lymnaea stagnalis. European Journal of Biochemistry 229:70–72. DOI: 10.1111/j.1432-1033.1995.0070l.x.
- Li L, Floyd P. 2001. Cerebrin prohormone processing, distribution and action in Aplysia californica. Journal of Neurochemistry 77:1569−1580. DOI: 10.1046/j.14714159.2001.00360.x.
- Mendoza-Porras O, Botwright NA, McWilliam SM, Cook MT, Harris JO, Wijels G, Colgrave ML. 2014. Exploiting genomic data to identify proteins involved in abalone reproduction. Journal of Proteomics 108:337–353. DOI: 10.1016/j.jprot.2014.06.001.
- Miller MW, Beushausen S, Vitek A, Stamm S, Kupfermann I, Brosius J, Weiss KR. 1993. The myomodulin-related neuropeptides: Characterization of a gene encoding a family of peptide cotransmitters in Aplysia. The Journal of Neuroscience 13:3358−3367.
- Monnier Z, Bride M. 1995. In vitro effects of methionine-enkephalin,somatostatin and insulin on cultured gonadal cells of the snail Helix aspersa. Experientia 51:824–830. DOI: 10.1007/bf01922437.
- Moroz LL, Edwards JR, Puthanveettil SV, Kohn AB, Ha T, Heyland A, Knudsen B, Sahni A, Yu F, Liu L, Jezzini S, Lovell P, Iannucculli W, Chen M, Nguyen T, Sheng H, Shaw R, Kalachikov S, Panchin YV, Farmerie W, Russo JJ, Ju J, Kandel ER. 2006. Neuronal transcriptome of Aplysia: Neuronal compartments and circuitry. Cell 127:1453–1467. DOI: 10.1016/j.cell.2006.09.052.
- Nässel DR, Larhammar D. 2013. Neuropeptides and peptide hormones. In: Galizia CG, Lledo PM, editors. Neurosciences - from molecule to behavior. Berlin, Heidelberg: Springer-Verlag. pp. 213–237.
- Popenoe EA, du Vigneaud V. 1954. A partial sequence of amino acids in performic acid-oxidized vasopressin. The Journal of Biological Chemistry 1:353–360.
- Santama N, Brierley M, Burke JF, Benjamin PR. 1994. Neural network controlling feeding in Lymnaea stagnalis: Immunocytochemical localization of myomodulin, small cardioactive peptide, buccalin, and FMRFamide-related peptides. The Journal of Comparative Neurology 342:352−365. DOI: 10.1002/cne.903420304.
- Schikorski D, Cuvillier-Hot V, Leippe M, Boidin-Wichlacz C, Slomianny C, Macagno E, Salzet M, Tasiemski A. 2008. Microbial challenge promotes the regenerative process of the injured central nervous system of the medicinal leech by inducing the synthesis of antimicrobial peptides in neurons and microglia. Journal of Immunology 181:1083–1095. DOI: 10.4049/jimmunol.181.2.1083.
- Sievers F, Wilm A, Dineen D, Gibson TJ, Karplus K, Li W, Lopez R, McWilliam H, Remmert M, Söding J. 2011. Fast, scalable generation of high-quality protein multiple sequence alignments using Clustal Omega. Molecular Systems Biology 7:1–6. DOI: 10.1038/msb.2011.75.
- Smit AB, Vreugdenhil E, Ebberink RHM, Geraerts WPM, Klootwijk J, Joosse J. 1988. Growth-controlling molluscan neurons produce the precursor of an insulin-related peptide. Nature 331:535–538. DOI: 10.1038/331535a0.
- Southey BR, Amare A, Zimmerman TA, Rodriguez-Zas SL, Sweedler JV. 2006. NeuroPred: A tool to predict cleavage sites in neuropeptide precursors and provide the masses of the resulting peptides. Nucleic Acids Research 34:267–272. DOI: 10.1093/nar/gkl161.
- Stewart MJ, Favrel P, Rotgans BA, Wang T, Zhao M, Sohail M, O’Connor WA, Elizur A, Henry J, Cummins SF. 2014. Neuropeptides encoded by the genomes of the Akoya pearl oyster Pinctata fucata and Pacific oyster Crassostrea gigas: A bioinformatic and peptidomic survey. BMC Genomics 15:840. DOI: 10.1186/1471-2164-15-840.
- Strand FL. 2003. Neuropeptides: General characteristics and neuropharmaceutical potential in treating CNS disorders. Progress in Drug Research 61:1–37. DOI: 10.1007/978-3-0348-8049-7_1.
- Sweedler JV, Li L, Rubakhin SS, Alexeeva V, Dembrow NC, Dowling O. 2002. Identification and characterization of the feeding circuit-activating peptides, a novel neuropeptide family of Aplysia. The Journal of Neuroscience 22:7797–7808. DOI: 10.1523/JNEUROSCI.22-17-07797.2002.
- Tamura K, Stecher G, Peterson D, Filipski A, Kumar S. 2013. MEGA6: Molecular evolutionary genetics analysis version 6.0. Molecular Biology and Evolution 30:2725–2729. DOI: 10.1093/molbev/mst197.
- Tanaka H, Yoshida T, Miyamoto N. 2003. Characterization of a family of endogenous neuropeptide ligands for the G protein-coupled receptors GPR7 and GPR8. Proceedings of the National Academy of Sciences of the United States of America 100:6251–6256. DOI: 10.1073/pnas.0837789100.
- Taussig R, Kaldany RR, Scheller RH. 1984. A cDNA clone encoding neuropeptides isolated from Aplysia neuron L11. Proceedings of the National Academy of Sciences of the United States of America 81:4988–4992. DOI: 10.1073/pnas.81.15.4988.
- Tetsuya I, Yoshimi Y-K, Hiroyuki M, Kenny PTM, Kyosuke N, Yojiro M. 1992. Mytilus-inhibitory peptide analogues isolated from the ganglia of a pulmonate mollusc, Achatina fulica. Comparative Biochemistry and Physiology Part C 101:245–249. DOI: 10.1016/0742-8413(92)90268-C.
- Trapnell C. 2009. TopHat: Discovering splice junctions with RNA-Seq. Bioinformatics 25:1105–1111. DOI: 10.1093/bioinformatics/btp120.
- Van Kesteren RE, Smit AB, Dirks RW, De With ND, Geraerts WPM, Joosse J. 1992. Evolution of the vasopressin/oxytocin superfamily: Characterization of a cDNA encoding a vasopressin-related precursor, preproconopressin, from the mollusc, Lymnaea stagnalis. Proceedings of the National Academy of Sciences of the United States of America 89:4593–4597. DOI: 10.1073/pnas.89.10.4593.
- Veenstra JA. 2009. Allatostatin C and its paralog allatostatin double C: The arthropod somatostatins. Insect Biochemistry and Molecular Biology 39:161–170. DOI: 10.1016/j.ibmb.2008.10.014.
- Veenstra JA. 2010. Neurohormones and neuropeptides encoded by the genome of Lottia gigantea, with reference to other mollusks and insects. General and Comparative Endocrinology 167:86–103. DOI: 10.1016/j.ygcen.2010.02.010.
- Wickham L, DesGroseillers L. 1991. A bradykinin-like neuropeptide precursor gene is expressed in neuron L5 of Aplysia californica. DNA and Cell Biology 10:249–258. DOI: 10.1089/dna.1991.10.249.
- Yang J, Yan R, Roy A, Xu D, Poisson J, Zhang Y. 2015. The I-TASSER suite: Protein structure and function prediction. Nature Methods 12:7–8. DOI: 10.1038/nmeth.3213.
- York PS, Cummins SF, Degnan SM, Woodcroft BJ, Degnan BM. 2012. Marked changes in neuropeptide expression accompany broadcast spawnings in the gastropod Haliotis asinina. Frontiers in Zoology 9:9. DOI: 10.1186/1742-9994-9-9.
- Zatylny-Gaudin C, Cornet V, Leduc A, Zanuttini B, Corre E, Le Corguille G. 2016. Neuropeptidome of the cephalopod Sepia officinalis: Identification, tissue mapping, and expression pattern of neuropeptides and neurohormones during egg laying. Journal of Proteome Research 15:48–67. DOI: 10.1021/acs.jproteome.5b00463.
- Zhang M, Wang Y, Li Y, Li W, Li R, Xie X, Wang S, Hu X, Zhang L, Bao Z. 2018. Identification and characterization of neuropeptides by transcriptome and proteome analyses in a bivalve mollusk Patinopecten yessoensis. Frontiers in Genetics 9:197. DOI: 10.3389/fgene.2018.00197.
- Zupanc GKH. 1996. Peptidergic transmission: From morphological correlates to functional implications. Micron 27:35–91. DOI: 10.1016/0968-4328(95)00028-3.