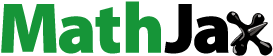
Abstract
Concentrations of trace elements (Cd, Pb, As, Cu and Zn) were determined in superficial sediments and in hepatopancreas, exoskeleton, and abdominal muscle of the red swamp crayfish Procambarus clarkii from several canals of the Po River Delta area. Sediments of the studied canals exhibited some metal pollution. The hepatopancreas of the crayfish showed a higher concentration of trace metals in comparison to the other tissues at all sites under scrutiny. Concentrations of As and Pb, either in sediment and P. clarkii tissues, were probably related to the use of fertilizers and pesticides. Moreover, the levels of certain metals accumulated in the abdominal muscle (Pb, Cu and Zn) exceeded the threshold values considered harmful to human health. The Toxic Contamination Index, depending on the bioaccumulation data of hepatopancreas and abdominal muscle, allowed us to assess the toxicity by heavy metals of sediments measuring the stress level of the detoxification organ, and resulted in >1 only at two sampling canals. We hypothesize that P. clarkii in the Po River Delta aquatic communities can act as a vector of pollutants, as crayfish can transfer their relatively high amount of heavy metals to higher trophic levels.
Introduction
Heavy metals of natural and anthropogenic sources are released into aquatic ecosystems, where they pose a serious threat because of their toxicity, long persistence, bioaccumulation and biomagnifications in the food chain (Zhou et al. Citation2008). Those most commonly found at contaminated sites are lead (Pb), chromium (Cr), arsenic (As), zinc (Zn), cadmium (Cd), copper (Cu), mercury (Hg), and nickel (Ni): contamination can originate from a wide variety of anthropogenic sources such as disposal of high metal wastes in improperly protected landfills, land application of fertilizer, animal manures, sewage sludge, compost, pesticides, coal combustion residues and atmospheric deposition (Wuana & Okieimen Citation2011). Heavy metals affect living organisms in various ways; some have essential functions (e.g. Cu, Mn and Zn) contributing to the maintenance of a good health in humans and animals (Rainbow Citation2007) and are toxic only at high levels, whereas others are xenobiotic: non-essential metals as Pb and Hg do not play any role in metabolism and are toxic even at low concentrations (Kouba et al. Citation2010).
Compared to chemo-physical analysis, biomonitoring can provide useful information to determine heavy metal pollution in the aquatic ecosystem over time. Indeed, whereas the former reflects existing conditions, biomonitoring gives an indication of past and current status (Zhou et al. Citation2008). Biomonitoring involves the use of organisms to assess environmental contamination. It can be done qualitatively by observing and noting changes in organisms or quantitatively by measuring the accumulation of chemicals in organism tissues. Aquatic invertebrates are sensitive to metals in their environment and are mostly used to assess aquatic ecosystem quality (Bonada et al. Citation2006). They can accumulate metals directly from the sediments or by food and, based on their life cycle, some species can accumulate more elements than the others (Pourang Citation1996). Molluscs, for example, as filter-feeding organisms are known to accumulate high concentrations of heavy metals (such as Cu, Cd, Zn and Hg) in their tissues. This is due to the fact that in the cells of these organisms heavy metal cations can be rapidly detoxified by different mechanisms, i.e. compartmentalization into lysosomes, accumulation into granules and membrane-bound vesicles or binding to specific soluble ligands such as metallothioneins (Viarengo & Nott Citation1993). Also, decapods accumulate metals in their tissues, and accumulation is dose- and time-dependent (Antòn et al. Citation2000). However, decapods are resistant organisms to environmental contamination, and even relatively high concentrations of metals are usually not responsible for their mortality (Kouba et al. Citation2010).
The model bioindicator species chosen in the present study is the red swamp crayfish Procambarus clarkii (Girard, 1852). P. clarkii stands as an important food in many parts of the world, being a rich source of protein. Native from northeastern Mexico and south-central USA (Hobbs et al. Citation1989), the red swamp crayfish was introduced for commercial and nutritional purposes in Europe in the seventies of the last century (Gherardi & Acquistapace Citation2007). P. clarkii is very diffuse and commercially exploited (Hobbs et al. Citation1989), also because of its invasiveness and tolerance to the environmental conditions. In recent decades, P. clarkii has invaded aquatic habitats and established stable populations in many water bodies across Europe, and particularly Spain and central Italy (Gherardi et al. Citation1999).
Red swamp crayfish are often an important component of the aquatic fauna, frequently being the largest invertebrate predator in their habitats. They are considered both key-species and ecosystem engineers (Statzner et al. Citation2003) and have been used as bioindicators to determine the bioavailability of heavy metals in the environment (Alcorlo et al. Citation2006; Bellante et al. Citation2015; Goretti et al. Citation2016). A number of characteristics make P. clarkii a good model species: they (i) are large enough to obtain samples from different organs, (ii) are easily recognizable and do not migrate, and finally (iii) have long life spans (2 years).
The aim of present work was to characterize heavy metal content in selected tissues of red swamp crayfish and surface sediment samples collected from different water bodies of the southern Po River Delta (northern Italy), in order to assess bioaccumulation mechanisms, evaluate whether these concentrations could be considered harmful for human health and, finally, to identify possible sources of contamination in the study area. We exploited the selective metal bioaccumulation in three different tissues of P. clarkii: hepatopancreas (the detoxification organ), exoskeleton, and abdominal muscle, which is the edible part. There is very scarce information on heavy metal distribution in the sediments and biota of this area, in spite of the presence of many industrial and agricultural activities.
Materials and methods
Crayfish and sediment sampling
Heavy metal content in organ tissue was assessed in crayfish from water bodies in Ferrara Province (Italy), a lowland area covering 2636 km2, located in the southern part of the Po River Delta. It is an intensively cultivated area, with more than 50% of land cultivated with winter cereals (32.11%) and maize (22.63%) (Comune di Ferrara Citation2004). Five sites in the hydrological network of the Province of Ferrara were chosen and sampled for Procambarus clarkii and sediments in June 2017: Cavo Bondesano (CB), Gramicia (GR), Val d’Albero (VA), Malpiglio (MA), and Bassa dei Frassini (BF) (). CB is surrounded by cropland cultivated with soy and maize, and low-density residential areas. The sampling site was located at 44°52ʹ1.48″N, 11°26ʹ53.67″E. GR flows alongside the Ferrara’s outer ring road, and it is susceptible to metal inputs from impervious surfaces and other urban inputs (sampling site: 44°50ʹ59.92″N, 11°37ʹ37.56″E). VA flows alongside a roadway, and it is surrounded by cropland (mainly orchards and wheat), with only slight urban influence (sampling site: 44°52ʹ30.76″N, 11°43ʹ5.76″E). MA runs through rice fields (sampling site: 44°53ʹ15.74″N, 11°59ʹ53.05″E), while BF flows inside the Nature Reserve of Bosco della Mesola, is surrounded with high levels of vegetation and organic debris, but receives waters from the Canal Bianco, a canal which drains cropland cultivated with vegetables (tomatoes, carrots, etc.); the sampling site was located at 44°51ʹ17.58″N, 12°14ʹ40.12″E.
Figure 1. Study sites in the Po River Delta area (Ferrara Province): CB Cavo Bondesano, GR Gramicia, VA Val d’Albero, MA Malpiglio, BF Bassa dei Frassini
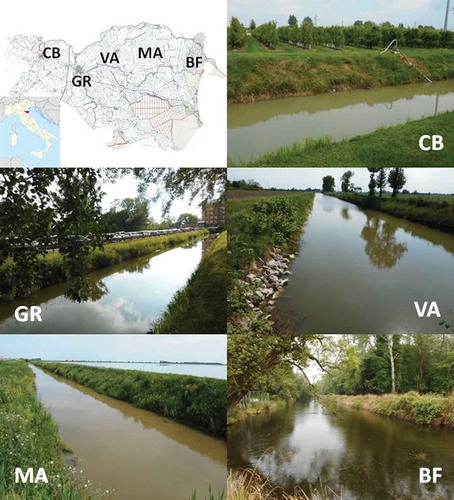
Adult crayfish were caught in cylindrical baited fish traps. For each site, 15 intermolt males were randomly selected from trapped crayfish. Each specimen was frozen and stored at −20°C. The frozen crayfish were thawed in the laboratory, and samples of hepatopancreas, exoskeleton, and abdominal muscle were removed. The tissue samples were oven-dried for 48 h at 40°C for weight determination. From each site, sediments were also collected using a Van Veen grab. At the laboratory, sediments were dried at 40°C for 3 days.
Heavy metal analysis
Sample preparation
The three dried parts dissected from the crayfish samples (hepatopancreas, exoskeleton and abdominal muscle) were ground using mortar and pestle before digestion with microwave technique for chemical analysis. An amount of 0.25 g of each sample was weighed and placed into TFM (tetrafluoromethoxyl) vessel, to which 8 mL of concentrated nitric acid (65% from Sigma Aldrich) and 2 mL of hydrogen peroxide (30% w/w from Honeywell-Fluka) were added. Vessels were sealed, placed in the rotor (SK15, Milestone) and then in the microwave oven (Ethos Easy Advanced Microwave Digestion System, Milestone). The digestion program reported in was employed. After cooling, samples were transferred into volumetric flasks (50 mL) and diluted to the mark with ultrapure water (Milli-Q water, Millipore corporation, USA). Sediment samples were grounded and 0.5 g of sample was placed in TFM vessels, with 9 mL of concentrated nitric acid (65%) and 3 ml of concentrated hydrochloric acid. reports also the microwave program employed for sediments. At the end of digestion, samples were filtered on ashless filters (Whatman 589/2) and diluted to the mark (50 mL) with ultrapure water. All samples were prepared and analysed in double.
Table I. Experimental conditions for sample digestion
Graphite Furnace Atomic Absorption Spectroscopy (GF-AAS)
As, Cd, Cu, Pb and Zn measurements in crayfish tissues and As in sediments were carried out with an Analyst 800 Atomic Absorption Spectrometer (Perkin-Elmer) equipped with Zeeman background correction system and electrothermal atomizer transversely heated graphite tube (THGA). Radiation sources for As, Cd and Pb were eletrodeless discarge lamps (EDLs, Perkin-Elmer) at wavelength 193.7, 282.3 and 283.3 nm, respectively (slit width 0.7 nm); for Cu and Zn cathode cave lamps (HCL, Perkin-Elmer) were employed at wavelength 324.8 nm for copper and 213.9 nm for zinc (slit width 0.7 nm). Twenty-microliter aliquots of the sample were injected into graphite tube, at room temperature, followed by a two-step drying, pyrolysis and atomization (one step each). Finally, the graphite tube was cleaned. As modifier for As and Cu 5 μL of a solution made of Pd and Mg(NO3)2 was used, while for Pb the solution (also 5 μL) was made of NH4H2PO4 and Mg(NO3)2.
Standard solutions for calibration curves were prepared by dilution of certified 1000 mg L−1 As, Cd, Pb, Cu and Zn monoelement standard solution (Trace Cert Fluka). All solutions were diluted with reagent blank. Detection limits of the method, evaluated from calibration curves of each element, were Cd, 0.19 mg kg−1; Pb, 0.51 mg kg−1; Cu, 0.48 mg kg−1; As, 0.25 mg kg−1; Zn, 3.76 mg kg−1.
Inductively Coupled Plasma-Optical Emission Spectrometer (ICP-OES)
Cd, Pb, Cu and Zn determination in sediments was carried out on a Perkin-Elmer Optima 3100 XL Inductively Coupled Plasma-Optical Emission Spectrometer equipped with an axial torch, segmented array charge-coupled device (SCD) detector and Babington-type nebulizer with cyclonic spray chamber for sample introduction. Plasma conditions were: RF power of 1.40 kW applied to the plasma; 15 L min−1 flow rate for plasma and 0.5 L min−1 for auxiliary gas. Nebulizer gas flow rate was 0.65 L min−1. Sample uptake was 1.5 mL min−1 for each of the three replicate scans.
Elemental standard solutions (1000 mg L−1 Cd, Pb, Cu and Zn from Trace Cert Fluka) were employed to prepare diluted standard solutions for calibration curves. Detection limits were obtained by the calibration curve. The following values were obtained: Cd, 0.35 mg kg−1; Pb 0.43 mg kg−1; Cu, 0.20 mg kg−1; Zn, 0.32 mg kg−1.
Method Quality Assessment
European Reference Material (ERM-CE278k Mussel Tissue) from IRMM (Institute for Reference Materials and Measurements) was used to assess method quality. An unweighted-means analysis was employed. Six replicate measurements of total concentrations of metals (Cd, Cu, Pb, As and Zn) were found to be not statistically different from the certified value at 95% of probability, thus attesting the accuracy of the method and the absence of bias. Additionally, method precision evaluated from the standard deviation for each analyte was comparable to that reported in certified material. summarizes the results of this study. Blank samples for crayfishes and sediments were prepared with analogous procedures.
Table II. Unweighted-mean analysis to assess method quality
Bioaccumulation and Toxic Contamination Index
We estimated the bioaccumulation in crayfish tissues at each site by using the Biota to Sediment Accumulation Factor, BSAF (Burkhard Citation2009), a ratio between metal concentration in the organism and sediment. Determination of the individual BSAF from appropriately paired observations enables the detection of differences (if they exist) in partitioning behavior among the paired observations for the site.
We also calculated the ratio between metal concentration in the hepatopancreas and in the abdominal muscle (HEP/ABD) of crayfish at each site.
The Toxic Contamination Index (TCI) was developed by Goretti et al. (Citation2016) to assess metal toxicity level in sediments. TCI for a specific metal (met) is expressed as
where PEC is the Probable Effect Concentration of the metal (Mac Donald et al. Citation2000); HEP and ABD are the mean concentrations of the metal in hepatopancreas and abdominal muscle, respectively.
Data analysis
One-way analysis of variance (ANOVA) was used to check for the difference in crayfish length among localities. For statistical analysis, metal concentrations below the detection limit were replaced with the mentioned detection limits. Differences in metal content of sediments among localities were evaluated using one-way ANOVA after log(x + 1) transformation of data to meet homoscedasticity. Metal concentration was analyzed through a two-way fixed-factor ANOVA, with sites (n = 5) and crayfish tissues (n = 3) as factors, to evaluate whether the measured metals showed greater accumulation in different tissues and localities. Significant terms were further analyzed through post-hoc, Tukey HSD tests. Data were checked for homoscedasticity and, whenever necessary, were log(x + 1)-transformed prior to analysis.
Results
Heavy metal concentrations in sediment samples
Sediment average concentration of heavy metals (As, Cd, Cu, Pb and Zn) is shown in . Following Mac Donald et al. (Citation2000), sediment toxicity by heavy metals was predicted using the Threshold Effect Concentration (TEC) and Probable Effect Concentration (PEC) reference values. The concentration of heavy metals in sediments showed significant differences between sampling sites (one-way ANOVA, all p < 0.01), and was higher at Cavo Bondesano (CB), followed by Bassa dei Frassini (BF) and Val d’Albero (VA), while Gramicia (GR) and Malpiglio (MA) exhibited a lower metal pollution scenario. Metal concentrations in sediment samples showed the following hierarchical order: Zn > Cu > Pb > As > Cd. The mean concentrations of Cd exceeded the TEC value at all study sites, Cu at CB, VA and BF, As only at BF. However, metal concentrations never exceeded the PEC thresholds.
Table III. Mean values of heavy metals (As, Cu, Cd, Pb and Zn) concentrations (mg kg−1) determined in sediments (standard deviation in parenthesis) from study sites (see text for acronyms)
Heavy metal concentrations in Procambarus clarkii tissues
From each sampling site, 15 intermolt male crayfish were analyzed. Average crayfish length was 7.38 (±1.26) cm at CB, 7.95 (±1.49) cm at GR, 7.65 (±1.65) cm at VA, 7.44 (±1.27) cm at MA, and finally 7.84 (±1.53) cm at BF (one-way ANOVA, all p > 0.05).
The concentration of metals in different tissues of crayfish is shown in . A wide range of concentrations of Cu (9.35–686.54 mg kg−1 d.w.) and Zn (15.39–197.80 mg kg−1 d.w.) were found in the analyzed tissues. A narrow range of concentrations was detected for each of the other heavy metals (<dl-4.48 mg kg−1 d.w. for As; <dl-1.26 mg kg−1 d.w. for Cd; <dl-2.43 mg kg−1 d.w. for Pb). In , the result of the two-way ANOVA is shown. The two main factors (tissue and site) plus the interaction term were significant for all metals, except for Pb, where only the interaction term resulted significant. Analysis across all sampling sites (Tukey HSD test: all p < 0.05) indicated that at all sites the crayfish hepatopancreas was significantly the primary accumulation tissue for Zn, Cu, Cd (except at CB), and As (except at PR), while Pb was significantly higher only in the abdominal muscle at MA.
Table IV. Mean values of heavy metals (As, Cu, Cd, Pb and Zn) concentrations (mg kg−1) determined in crayfish tissues (standard deviation in parenthesis) from study sites (see text for acronyms)
Table V. Results of two-way ANOVA (factors: crayfish tissue and site) on metal (As, Cu, Cd, Pb and Zn) concentration
Bioaccumulation in Procambarus clarkii tissues
The BSAF index resulted in>1 for Cu in hepatopancreas, abdominal muscle (except CB and BF) and, at two sites (GR and MA), even in an exoskeleton. Bioaccumulation was evident for Zn (mostly in hepatopancreas but also in abdominal muscle), and, at one site, for As. No bioaccumulation resulted for Cd and Pb. shows the BSAF index for crayfish tissues at each site.
Table VI. The Biota to Sediment Accumulation Factor (BSAF) for heavy metals (As, Cu, Cd, Pb and Zn) at each site (in bold BSAF > unit)
The HEP/ABD ratio was particularly high for Cu, especially at MA (14.99), and GR (9.75), and for As (particularly at MA and GR). This ratio decreased for Zn and Pb ().
Table VII. Bioaccumulation ratio (HEP/ABD) for heavy metals (As, Cu, Cd, Pb and Zn) at each site
In , the Toxic Contamination Index (TCI) for As, Cd, Cu, Pb and Zn is shown. TCI showed values >1, indicating quite a high level of contamination, for Cu at MA (TCI = 1.84), and GR (TCI = 1.57). It was also slightly positive, thus indicating a low level of contamination, for Cu at CB, VA, and BF, for Cd at MA, and for Zn at CB and MA.
Discussion
In contrast to waters, sediments integrate pollutant concentrations over the time so that concentrations of concern can be found. Sediments tend to absorb contaminants, being both sink and a potential source of pollutants to the water column (De Jonge et al. Citation2008). However, in the aquatic environment, sediments provide habitat, feeding, and breeding areas for many different species. Recently, the protection of sediments has become mandatory to preserve the ecological status in rivers, as requested by the EU Water Framework Directive 2000/60/EC. The Italian Act D.Lgs 152/2006 implements the Directive, and its Annex V establishes thresholds for metals contamination in aquatic sediments: 20 mg kg−1 d.w. for As, 120 for Cu, 2 for Cd, 100 for Pb, 150 for Zn. At our study sites, heavy metal concentrations below the legislative threshold were found in sediments, with the exception of Cd at Cavo Bondesano (CB: 2.08 mg kg−1 d.w.), Val d’Albero (VA: 2.6 mg kg−1 d.w.), and Bassa dei Frassini (BF: 3.6 mg kg−1 d.w.). In the Padanian Plain some heavy metals (e.g. Cr and Ni) can show high background concentration because of geochemical anomalies (Bianchini et al. Citation2012). This does not seem the case of Cd: it is a rare, non-nutritive element (according to Heinrichs et al. Citation1980, the average concentration of Cd in the lithosphere is 0.098 mg kg−1) and, in the present case, the relatively high amount of Cd in the canal sediments is probably derived from anthropogenic pollution. At our study sites, sediments may be contaminated with Cd most likely by agricultural runoff following application of fertilizers (e.g. phosphorous fertilizers) or pesticides (e.g. fungicides) which contain Cd (Wuana & Okieimen Citation2011), or by the discharge of Cd-containing waste materials from industrial or urban activities (Navratil & Minarik Citation2009).
Despite concentrations below the legislative threshold, suggesting slight metal contamination in our canal sediments, crayfish accumulated (even massively) certain heavy metals in their tissues. As shown in , high heavy metal concentrations were found in P. clarkii tissues after comparison with concentrations found previously in crayfish from other areas. Many studies on the distribution of contaminants in red swamp crayfish tissues report that the hepatopancreas is the most important storage organ for trace metals (). Results from this study also report a higher content of all considered metals in the hepatopancreas with respect to exoskeleton and abdominal muscle. Hepatopancreas is the organ where many physiological processes take place, as absorption and storage of digested food, secretion of digestive juices, and the detoxification and storage of heavy metals (Viarengo Citation1989). The exoskeleton is in direct contact with the environment: metals in exoskeleton are associated with passive adsorption from water rather than through bioaccumulation (Alcorlo et al. Citation2006). Muscle tissue is of the most concern because the abdominal muscle is the part consumed by humans.
Table VIII. Range of heavy metals in red swamp crayfish tissues from different areas (concentrations are mg kg−1 d.w.)
Previous studies (Alcorlo et al. Citation2006; Suárez-Serrano et al. Citation2010) have reported that As, which is a non-essential element for crayfish, tends to accumulate in crayfish tissues with increasing concentration in environment and exposure time. Results from our study showed that As accumulation distribution levels in crayfish tissues were highest in hepatopancreas, followed by muscle. The As concentrations found in our study were slightly lower than As concentrations found by other authors elsewhere (). European legislation (Commission Regulation 1881/2006) establishes no maximum threshold for As concentrations in seafood. China has legislation (SAPRC Citation2012) regulating the maximum level for this contaminant (5 mg kg−1 w.w., i.e. ≈1.5 mg kg−1 d.w.; Rigler & Downing Citation1984); thus, this threshold could be adopted as a model by other countries.
The maximum permissible limits for human consumption established by European legislation (Commission Regulation 1881/2006) set maximum levels for Cd and Pb to a threshold of 0.5 mg kg−1 w.w. (corresponding to 0.152 mg kg−1 d.w.; Rigler & Downing Citation1984) in muscle meat from appendages and abdomen of crustaceans. Cd and Pb are non-essential elements not involved in P. clarkii metabolism, tending to increase with increasing environmental concentrations. Our results show a higher concentration of Cd (range: 0.32–1.26 mg kg−1 d.w.) in the hepatopancreas, with respect to abdominal muscle and the exoskeleton, at almost all sites. Previous studies have reported bioaccumulation of Cd and Pb in tissues of P. clarkii. Bellante et al. (Citation2015) found that Pb accumulated in crayfish tissues in the order hepatopancreas (max 17.7 mg kg−1 d.w.) > muscle (max 0.65 mg kg−1 d.w.). Goretti et al. (Citation2016) evidenced a higher Cd and Pb accumulation in hepatopancreas than in muscles (). Conversely, at our study sites Pb resulted more concentrated in the abdominal muscle (range: <dl-2.43 mg kg−1 d.w.) than in hepatopancreas (range: <dl-1.11 mg kg−1 d.w.). Despite the BSAF index evidenced no bioaccumulation for Pb (), the concentration of this metal found in muscles exceeded the threshold limit established for human consumption at sites VA, MA, and BF.
Although toxic for aquatic organisms (Cu, for example, is used as a biocide in antifouling paints), Cu and Zn are essential micronutrients: Cu is a constituent of the crayfish respiratory pigment, and Zn is an active centre for metalloenzymes (Viarengo Citation1989). Due to the fact that Cu and Zn are essential, they occur in high concentrations in crayfish tissues regardless of their environmental concentrations. However, when their environmental concentration is high, the bioaccumulation of Cu and Zn greatly increases in crayfish tissues (Mac Donald et al. Citation2000). The biological response of P. clarkii shows a larger accumulation in the hepatopancreas with respect to abdominal muscle and exoskeleton of Cu and Zn. Although no EU threshold limit for human consumption of crayfish muscle is established for Cu and Zn, other national regulations set maximum limits, e.g. for Cu at 20 mg kg−1 w.w. in edible mass (Spain: Boletin Oficial del Estado 195, 15/8/1991), for Zn at 70 mg kg−1 w.w. (U.S. Food and Drugs Administration), as reported by Alcorlo et al. (Citation2006). Converting these values to dry weight (Rigler & Downing Citation1984), the maximum limit for Cu becomes 6.1 mg kg−1 d.w. and for Zn 21.2 mg kg−1 d.w. The concentration of Cu and Zn in abdominal muscle of crayfish from all our sampled localities resulted well above those maximum limits. It is known that at least 50–60% of the Cu in decapods is stored in the haemolymph (Depledge & Bjerregaard Citation1989). Haemocyanin is the major haemolymph constituent, exhibiting low oxygen affinity; a critical factor enabling haemocyanin to transport oxygen is the presence of Cu in its structure (Depledge & Bjerregaard Citation1989). A number of environmental factors are known to influence haemolymph proteins and Cu concentrations (Depledge & Bjerregaard Citation1989). A trend of seasonal variation in blood proteins which closely followed temperature changes was reported by Uglow (Citation1969). A compensatory increase in haemocyanin concentration is also known to occur with prolonged exposure to hypoxia: Senkbeil and Wriston (Citation1981) and Hagerman and Uglow (Citation1985) reported hypoxia-induced haemocyanin synthesis in different decapods. The most dramatic example of an environmental factor affecting haemolymph protein composition was observed in decapods exposed to hypo-osmotic seawater: haemocyanin concentration almost doubled within 48 h, increasing from 15–20 mg mL−1 to 35 mg mL−1, and this was associated with an increase in haemolymph Cu from 27 μg mL−1 to 62 μg mL−l (Depledge & Bjerregaard Citation1989). Environmental conditions in the sampled canals are very stressful, with differences in temperature of over 30 degrees between summer and winter, and hypoxic waters observed very often throughout the year (https://www.arpae.it/dettaglio_documento.asp?id=7464&idlivello=2020). Unfortunately, the quantification of haemolymph Cu was beyond the scope of this study, and consequently, we are unable to say whether the accumulation of Cu in the abdominal muscle (richly sprayed by haemolymph) was also due to a physiological response to external stress as found, for example, by Depledge and Bjerregaard (Citation1989).
The biological response to heavy metals of crayfish evidenced a higher accumulation (HEP/ABD ratio; ) in the hepatopancreas with respect to abdominal muscle of practically all metals at all sampled canals. According to Goretti et al. (Citation2016), these results show that the level of toxicity of a site can be given by the extent of the ratio between the tissue concentration values with high and low detoxifying activity, in our case, respectively, hepatopancreas and abdominal muscle of P. clarkii. Caution is however needed when a concentration is below the detection limit (e.g. Cd or Pb at some sites; ) since the HEP/ABD ratio may result in uncertain values for some metals. As suggested by Goretti et al. (Citation2016), and evidenced by our results (especially at Gramicia and Malpiglio for Cu), the HEP/ABD ratio may represent a robust biological response of crayfish to heavy metal contamination at sites where metal concentrations in hepatopancreas and abdominal muscle are clearly detectable, and where typically the BSAF>1.
The Toxic Contamination Index (TCI; Goretti et al. Citation2016) allowed us to assess the toxicity by heavy metals of sediments measuring the stress level of the detoxification organ of the crayfish. In fact, TCI formulation depends on metal bioaccumulation data of hepatopancreas and abdominal muscle of P. clarkii. According to Goretti et al. (Citation2016), when TCI > 1, the index highlights the toxicity level of a polluted site (e.g. Gramicia and Malpiglio for Cu), conversely when TCI < 1 the sediments are poorly contaminated, as in the case of the other metals at the various study sites.
Conclusions
First evidence of this study was that sediments of the studied canals in the Po River Delta exhibited low metal pollution. Crayfish accumulated higher levels of heavy metals in the hepatopancreas than in the other tissues, consistently with previous studies. The levels of certain metals accumulated in the abdominal muscle exceeded the threshold values established by the EU (as for Pb) or some National (as for Cu and Zn) legislation. Even though our results should be treated with caution because of the relatively small sample size and the sampled canals, from an ecosystem perspective, attention should be paid to the role of the red swamp crayfish P. clarkii in the Po River Delta aquatic communities as a vector of pollutants, as crayfish can transfer their relatively high amount of heavy metals to higher trophic levels (e.g. eels, pikes, herons, otters, etc.). To the legislators, we propose P. clarkii for use as a bioindicator of heavy metals due to its ability to accumulate these environmental pollutants. Finally, our results also evidence that red swamp crayfish P. clarkii living in the Po River Delta are not suitable for human consumption.
Acknowledgements
We thank Michela Argentieri and Filippo Sarti (University of Ferrara) for fieldwork. We are grateful to two anonymous reviewers for their constructive criticism.
Disclosure statement
No potential conflict of interest was reported by the authors.
References
- Alcorlo P, Otero M, Crehuet M, Baltanás A, Montes C. 2006. The use of the red swamp crayfish (Procambarus clarkii, Girard) as indicator of the bioavailability of heavy metals in environmental monitoring in the River Guadiamar (SW, Spain). Science of the Total Environment 366:380–390. DOI: 10.1016/j.scitotenv.2006.02.023.
- Antòn A, Serrano T, Angulo E, Ferrero G, Rallo A. 2000. The use of two species of crayfish as environmental quality sentinels: The relationship between heavy metal content, cell and tissue biomarkers and physico-chemical characteristics of the environment. Science of the Total Environment 247:239–251. DOI: 10.1016/S0048-9697(99)00493-3.
- Bellante A, Maccarone V, Buscaino G, Buffa G, Filiciotto F, Traina A, Del Core M, Mazzola S, Sprovieri M. 2015. Trace element concentrations in red swamp crayfish (Procambarus clarkii) and surface sediments in Lake Preola and Gorghi Tondi natural reserve, SW Sicily. Environmental Monitoring and Assessment 187:1–18. DOI: 10.1007/s10661-015-4613-4.
- Bianchini G, Natali C, Di Giuseppe D, Beccaluva L. 2012. Heavy metals in soils and sedimentary deposits of the Padanian Plain (Ferrara, Northern Italy): Characterisation and biomonitoring. Journal of Soils and Sediments 12:1145–1153. DOI: 10.1007/s11368-012-0538-5.
- Bonada N, Prat N, Resh VH, Statzner B. 2006. Developments in aquatic insect biomonitoring: A comparative analysis of recent approaches. Annual Review of Entomology 51:495–523. DOI: 10.1146/annurev.ento.51.110104.151124.
- Burkhard L. 2009. Estimation of Biota Sediment Accumulation Factor (BSAF) from Paired Observations of Chemical Concentrations in Biota and Sediment (Final Report). Cincinnati, OH: U.S. Environmental Protection Agency, Ecological Risk Assessment Support Center. EPA/600/R-06/047.
- Comune di Ferrara. 2004. L’agricoltura a Ferrara: Il censimento 2000 e le ultime annate agrarie. Ferrara: Ufficio Stampa del Comune di Ferrara. 40 pp (in Italian).
- De Jonge M, Van de Vijver B, Blust R, Bervoets L. 2008. Responses of aquatic organisms to metal pollution in a lowland river in Flanders: A comparison of diatoms and macroinvertebrates. Science of the Total Environment 407:615–629. DOI: 10.1016/j.scitotenv.2008.07.020.
- Depledge M-H, Bjerregaard P. 1989. Haemolymph protein composition and copper levels in decapod crustaceans. Helgoländer Meeresuntersuchungen 43:207–223. DOI: 10.1007/BF02367900.
- Devesa V, Súñer MA, Lai VM, Granchinho SCR, Martínez JM, Vélez D, Cullen WR, Montoro R. 2002. Determination of arsenic species in a freshwater crustacean Procambarus clarkii. Applied Organometallic Chemistry 16:123–132. DOI: 10.1002/aoc.v16:3.
- El Qoraychy I, Fekhaoui M, El Abidi A, Benakame R, Bellaouchou A, Yahyaoui A. 2015. Accumulation of copper, lead, chrome, cadmium in some tissues of Procambarus clarkii in Rharb Region in Morocco. Journal of Geoscience and Environment Protection 3:74–81.
- Faria M, Huertas D, Soto DX, Grimalt JO, Catalan J, Riva MC, Barata C. 2010. Contaminant accumulation and multi-biomarker responses in field collected zebra mussels (Dreissena polymorpha) and crayfish (Procambarus clarkii), to evaluate toxicological effects of industrial hazardous dumps in the Ebro river (NE Spain). Chemosphere 78:232–240. DOI: 10.1016/j.chemosphere.2009.11.003.
- Gedik K, Kongchum M, DeLaune RD, Sonnier JJ. 2017. Distribution of arsenic and other metals in crayfish tissues (Procambarus clarkii) under different production practices. Science of the Total Environment 574:322–331. DOI: 10.1016/j.scitotenv.2016.09.060.
- Gherardi F, Acquistapace P. 2007. Invasive crayfish in Europe: The impact of Procambarus clarkii on the littoral community of a Mediterranean lake. Freshwater Biology 52:1249–1259. DOI: 10.1111/fwb.2007.52.issue-7.
- Gherardi F, Baldaccini GN, Barbaresi S, Ercolini P, De Luise G, Mazzoni D, Mori M. 1999. Alien crayfish in Europe: The situation in Italy. In: Gherardi F, Holdich DM, editors. Crayfish in Europe as alien species. How to make the best of a bad situation? Rotterdam: A.A. Balkema. pp. 107–128.
- Goretti E, Pallottini M, Ricciarini MI, Selvaggi R, Cappelletti D. 2016. Heavy metals bioaccumulation in selected tissues of red swamp crayfish: An easy tool for monitoring environmental contamination levels. Science of the Total Environment 559:339–346. DOI: 10.1016/j.scitotenv.2016.03.169.
- Hagerman L, Uglow RF. 1985. Effects of hypoxia on the respiratory and circulatory regulation of Nephrops norvegicus. Marine Biology 87:273–278. DOI: 10.1007/BF00397805.
- Heinrichs H, Schulz-Dobrick B, Wedepohl KH. 1980. Terrestrial geochemistry of Cd, Bi. Ti, Pb, Zn and Rb. Geochimica et Cosmochimica Acta 44:1519–1532. DOI: 10.1016/0016-7037(80)90116-7.
- Hobbs HH, Jass JP, Huner JV. 1989. A review of global crayfish introductions with particular emphasis on two north American species (Decapoda, Cambaridae). Crustaceana 56:299–316. DOI: 10.1163/156854089X00275.
- Hothem RL, Bergen DR, Bauer ML, Crayon JJ, Meckstroth AM. 2007. Mercury and trace elements in crayfish from Northern California. Bulletin of Environmental Contamination and Toxicology 79:628–632. DOI: 10.1007/s00128-007-9304-6.
- Kouba A, Buřič M, Kozák P. 2010. Bioaccumulation and effects of heavy metals in crayfish: A review. Water, Air, & Soil Pollution 211:5–16. DOI: 10.1007/s11270-009-0273-8.
- Mac Donald DD, Ingersoll CG, Berger TA. 2000. Development and evaluation of consensus-based sediment quality guidelines for freshwater ecosystems. Archives of Environmental Contamination and Toxicology 39:20–31. DOI: 10.1007/s002440010075.
- Madden JD, Grodner RM, Feagley SE, Finerty MW, Andrews LS. 1991. Minerals and xenobiotic residues in the edible tissues of wild and pond-raised Louisiana crayfish. Journal of Food Safety 12:1–15. DOI: 10.1111/jfs.1991.12.issue-1.
- Mancinelli G, Papadia P, Ludovisi A, Migoni D, Bardelli R, Fanizzi FP, Vizzini S. 2018. Beyond the mean: A comparison of trace- and macroelement correlation profiles of two lacustrine populations of the crayfish Procambarus clarkii. Science of the Total Environment 624:1455–1466. DOI: 10.1016/j.scitotenv.2017.12.106.
- Navratil T, Minarik L. 2009. Trace elements and contaminants. In: Cilek V, editor. Earth system: History and natural variability. Vol. IV. Singapore: EOLSS Publishers/UNESCO. pp. 250–279.
- Peng Q, Nunes LM, Greenfield BK, Dang F, Zhong H. 2016. Are Chinese consumers at risk due to exposure to metals in crayfish? A bioaccessibility-adjusted probabilistic risk assessment. Environment International 88:261–268. DOI: 10.1016/j.envint.2015.12.035.
- Pourang N. 1996. Heavy metal concentrations in surficial sediments and benthic macroinvertebrates from Anzali wetland, Iran. Hydrobiologia 331:53–61. DOI: 10.1007/BF00025407.
- Rainbow PS. 2007. Trace metal bioaccumulation: Models, metabolic availability and toxicity. Environment International 33:576–582. DOI: 10.1016/j.envint.2006.05.007.
- Rigler FH, Downing JA. 1984. The calculation of secondary production. In: Downing JA, Rigler FH, editors. A manual on methods for the assessment of secondary productivity in freshwaters. IBP Handbook. Vol. 17. Oxford: Blackwell. pp. 19–58.
- Sánchez-López FJ, Gil Garcia MD, Martínez JL, Aguilera PA, Garrido PA. 2004. Assessment of metal contamination in Doñana National Park (Spain) using Crayfish (Procamburus Clarkii). Environmental Monitoring and Assessment 93:17–29. DOI: 10.1023/B:EMAS.0000016789.13603.e5.
- SAPRC. 2012. Maximum levels of contaminants in foods. GB2762-2012. Beijing, China: Standardization Administration of the People’s Republic of China.
- Senkbeil EG, Wriston JC. 1981. Hemocyanin synthesis in the american lobster, Homarus americanus. Comparative Biochemistry and Physiology 68B:163–171.
- Statzner B, Peltret O, Tomanova S. 2003. Crayfish as geomorphic agents and ecosystem engineers: Effect of a biomass gradient on baseflow and flood-induced transport of gravel and sand in experimental streams. Freshwater Biology 48:147–163. DOI: 10.1046/j.1365-2427.2003.00984.x.
- Suárez-Serrano A, Alcaraz C, Ibáñez C, Trobajo R, Barata C. 2010. Procambarus clarkii as a bioindicator of heavy metal pollution sources in the lower Ebro River and Delta. Ecotoxicology and Environmental Safety 73:280–286. DOI: 10.1016/j.ecoenv.2009.11.001.
- Uglow RF. 1969. Haemolymph protein concentrations in portunid crabs—I. Studies on adult Carcinus maenas. Comparative Biochemistry and Physiology 30:1083–1090. DOI: 10.1016/0010-406X(69)91046-9.
- Viarengo A. 1989. Heavy metals in marine invertebrates: Mechanisms of regulation and toxicity at the cellular level. Aquatic Sciences 1:295–317.
- Viarengo A, Nott JA. 1993. Mechanisms of heavy metal cation homeostasis in marine invertebrates. Comparative Biochemistry and Physiology Part C 104:355–372. DOI: 10.1016/0742-8413(93)90001-2.
- Wuana RA, Okieimen FE. 2011. Heavy metals in contaminated soils: A review of sources, chemistry, risks and best available strategies for remediation. ISRN Ecology 2011:1–20. DOI: 10.5402/2011/402647.
- Zhou Q, Zhang J, Fu J, Shi J, Jiang G. 2008. Biomonitoring: An appealing tool for assessment of metal pollution in the aquatic ecosystem. Analytica Chimica Acta 606:135–150. DOI: 10.1016/j.aca.2007.11.018.