Abstract
Morphological changes and prolonged sperm storage and loss of female reproductive tract per year and site as well as reproductive cycle were investigated by using an optical microscope, scanning electron microscope and transmission electron microscope in Rhinolophus ferrumequinum korai. The size and morphological characteristics of the uterus were found to be highly relevant to mating and the delivery period. In particular, some of the sperm ejaculated to the female reproductive tract became extinct due to phagocytosis by leukocytes starting from the end of August, which continued until the end of February. Accordingly, this period may be called the cleansing period. The sperm became extinct mainly in the uterine lumen, and an extremely small amount of sperm became extinct by secretion from the uterine gland and the phagocytosis by leukocytes. Such phagocytosis seemed to result in maintaining the clean intrauterine environment to facilitate the implantation of the fertilized egg. Also, phagocytosis by leukocytes was considered as a physiological mechanism to kill the sperm breaking into the female reproductive tract by recognizing them as alien materials, to maintain an appropriate number of sperm at the fertilization site of the fallopian tubes, so as to improve fertility. Only the sperm reaching the caudal isthmus of oviduct were found to be involved in fertilization in the next year. Ovulation and fertilization occurred approximately at the end of March. Embryogenesis appeared consecutively with implantation. The pregnancy period was approximately 70 days; delivery occurred approximately early- and middle June; and the nurturing period was approximately 80 days.
Introduction
In terms of animal reproductive pattern, a number of mammal species subject to seasonal reproduction react to annual climate change (Deshmukh & Dhamani Citation2011). In some bats, foodstuffs and ambient temperature impact the embryonic development and gestation period (Racey Citation1973), and major factors impacting the reproductive cycle include hormone (Kawamoto Citation2003) as well as a number of environmental changes such as diet, water, habitat space, climate condition and availability of climate (Vasantha Citation2016).
The male reproductive cycle of hibernating bats is classified as three types, “Pipistrellus pattern”, “Myotis pattern” and “Miniopterus pattern”, in consideration of sperm differentiation process and change in adnexal organs (Gustafson Citation1979). In particular, studies of serial events in testicles and sperm fate and loss in epididymis during the male reproductive cycle, conducted on temperate hibernating bats, are worthy of notice (Lee Citation2018a, Citation2018b).
In terms of reproduction in female bats, mating, ovulation, fertilization, egg development and delivery occur unremitted in the tropical non-hibernating bats as same to the general mammalian types. The tropical non-hibernating bat species include Desmodus rotundus mutinus (Wimsatt & Trapido Citation1952), Pteropus giganteus, Cynopterus sphinx, C. gangeticus, Rhinopoma kinneari, Megaderma lyra, Rhinolophus rouxi, Hipposideros bicolor pallidus, H. speoris, Taphozous longimanus and Scotophilus wroughtoni (Gopalakrishna & Moghe Citation1960; Gopalakrishna et al. Citation1974).
As a strategy to adapt to environmental changes, the temperate female bats have evolved by unique reproduction of their own, including “prolonged sperm storage type” and “delayed implantation type”.
First, the prolonged sperm storage type refers to that the sperms stored in the female reproductive tract have long-term hibernation after mating in autumn, followed by ovulation, fertilization and embryonic development in consecutive order in spring of next year, and includes vespertilionine from North America (Guthrie Citation1933; Reeder Citation1939; Christian Citation1956; Krutzsch Citation1975), and temperate vespertilionids and rhinolophids (Matthews Citation1937; Wimsatt Citation1942; Hiraiwa & Uchida Citation1955, Citation1956; Wimsatt et al. Citation1966; Racey & Potts Citation1970; Gopalakrishna & Madhavan Citation1971; Medway Citation1972; Mōri & Uchida Citation1974; Krishna & Dominic Citation1978; Racey Citation1979; Uchida et al. Citation1984, Citation1988; Son et al. Citation1987, Citation1988; Mōri et al. Citation1989; Lee & Son Citation2000; Sharifi et al. Citation2004).
Second, the delayed implantation type refers to that ovulation and fertilization occur after mating in autumn and the fertilized egg is stored in the female reproductive tract while embryogenesis occurs consecutively in the spring of the next year. This type includes Miniopterus schreibersii and Eidolon helvum (Mutere Citation1965) and Rhinolophus rouxi (Ramakrishna & Rao Citation1977) of vespertilionid, as well as Miniopterus schreibersii fuliginosus (Uchida & Mōri Citation1974a; Mōri & Uchida Citation1980, Citation1981a, Citation1981b, Citation1982; Kimura & Uchida Citation1983, Citation1984).
Fragmentary studies of reproductive phenomenon in female bats were reported (Uchida Citation1950, Citation1953; Hiraiwa & Uchida Citation1955, Citation1956; Wimsatt & Parks Citation1966; Wimsatt et al. Citation1966; Racey & Potts Citation1970; Mōri & Uchida Citation1974, Citation1980, Citation1981a, Citation1981b, Citation1982; Uchida & Mōri Citation1974a, Citation1974b; Racey Citation1979; Oh et al. Citation1985; Son et al. Citation1987, Citation1988; Uchida et al. Citation1988; Mōri et al. Citation1989; Kim & Oh Citation1991; Lee & Son Citation2000), while an annual reproductive cycle in the female Rhinolophus ferrumequinum korai was not conducted. Accordingly, this study was conducted to examine serial reproductive cycles including morphological changes and characteristics in female reproductive tract as well as sperm storage and loss in reproductive tract per month and site.
Materials and methods
Experimental animals were collected and examined under the guidelines of the Kyungnam University Institutional Animal Care and Use Committee (KUIAC).
In this study, 58 female Rhinolophus ferrumequinum korai entities collected at the same date every month as possible January 2017-December 2018 in the abandoned mines over Gyeongnam and Jeonnam regions were used as study materials (), transferred to the laboratory immediately after collection and inhalation-anesthetized with ether before use. The tissues collected for monthly observation of morphological characteristics and changes in reproductive tract were washed immediately with Milloning’s buffer (4°C, pH 7.4) solution. After completion of washing, the tissues were soaked in a 3%-Glutaraldehyde solution (4°C, pH 7.4, Milloning’s buffer) for 24 h and photographed (). For observation with scanning electron microscope, the reproductive tract of each month was transected with the razor and fixed in 3% Glutaraldehyde solution for 24 h. Post-fixation tissues were washed with Milloning’s buffer solution twice in 20-min intervals and fixed in 1.33% OsO4 solution after 2 h. Post-fixation tissues were washed with Milloning’s buffer solution twice in 20-min intervals. Post-washing tissues were dehydrated twice in 30-min intervals in alcohol concentration-ascending order (70%, 80%, 85%, 90%, 95% and 100%, respectively). Post-dehydration tissues were transposed into HMDS (hexamethyl-disilazane, Sigma) solution twice for 24 h, respectively. Post-transposition tissues were evaporated to OsO4 steam within the Osmium coater (HPC-1 S, Japan) at 10–13 PS (10 x 0.1 Torr) vacuum and 3 mA for 15 s. Post-evaporation tissues were observed by the scanning electron microscope (TM-1000, Hitachi, Japan). Hematoxylin & Eosin staining and Trichrome staining were conducted by using the conventional paraffin sectioning method in each tissue, respectively, to understand the histological characteristics in the reproductive tract per year. For the transmission electron microscopic observation, tissues by the site of the female reproductive tract were sectioned in 1.5–2 mm3 size, pre-fixed in 3% Glutaraldehyde solution for 24 h, washed with the same buffer (Milloning’s buffer) twice in 20-min intervals, and immediately post-fixed in 1.33% OsO4 solution for 2 h. Post-fixation tissues were washed with the same buffer twice in 20-min intervals. Post-washing tissues were dehydrated twice in 30-min intervals in alcohol concentration-ascending order (70%, 80%, 85%, 90%, 95% and 100%, respectively). The tissues dehydrated were transposed to propylene oxide solution and embedded with Epon 812 synthetic resins. The tissues embedded were cut in 400 nm thickness by ultramicrotome (MT-1; Sorvall, Dupont), stained with 0.5% toluidine blue and observed, from which consecutive fragments were obtained in 60–70 nm thickness to be double-stained with Uranyl acetated and lead citrate solutions and observed by transmission electron microscope (TEM, H-600, Hitachi).
Table I. Sperm storage and disappearance per month and the site in the female reproductive tract in Korean greater horseshoe bats (Rhinolophus ferrumequinum korai)
Figure 1. Morphological change in the female reproductive tract in Korean greater horseshoe bats (Rhinolophus ferrumequinum korai). The size of the uterus was maintained consistently from the end of August to the end of March of the next year, increased gradually since the end of March when ovulation and fertilization occurred and reached a maximum in May. Meanwhile, it decreased remarkably during the delivery and nurturing period. F, fetus; O, ovary; Ov, oviduct; U, uterus; V, vagina; (a), late-August; (b), late-September; (c), late-October; (d), late-November; (e), late-December; (f), late-January; (g), late-February; (h), late-March; (i), late-April; (j), late-May; (k), early-June; (l), late-July
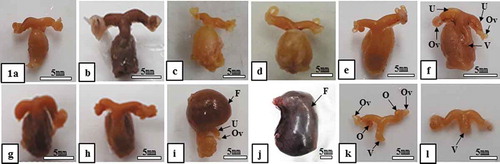
Results
To understand female the reproductive cycle of the Korean hibernating horseshoe bats (R. ferrumequinum korai), morphological changes in the reproductive tract per month and prolonged sperm storage and sperm loss per site (uterine lumen, uterine gland, vagina, uterotubal junction and caudal isthmus of the oviduct) were investigated and the results are below ( and ; –).
Table II. Comparison of characteristics of female annual reproductive cycle by step and sperm survival, storage, and disappearance by site in 14 species of Vespertilionidae and 2 species of Rhinolophidae
Figure 2. Optical, scanning electron microscopic and transmission electron microscopic images of virginal lumen, cervix, uterus and uterine lumen (August sample). Attention should be focused on the figure of sperm mixed by secretion from vaginal epithelium in the vaginal lumen (Figure 2(a)) and phagocytosed by leukocytes in the uterine lumen (Figure 2(b, c)). L, leucocyte; S, sperm; Sh, sperm head; Uc, uterine cervix; Ucl, uterine cervix lumen; Ug, uterine gland; Ul, uterine lumen; Uw, uterine wall; V, vagina. (a), SEM; (b), Hematoxylin & Eosin stain; (c), TEM
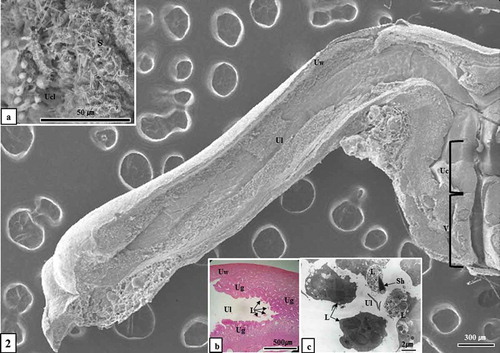
Figure 3. Optical microscopic, scanning electron microscopic and transmission electron microscopic images of sperm killing in the uterine lumen and uterine gland (December sample). Sperm were phagocytosed by leukocytes in the uterine lumen (Figure 3(a-c)) and uterine gland (Figure 3(d)). Ec, endometrial epithelial cell; L, leucocyte; S, sperm; Sh, sperm head; Ug, uterine gland; Ul, uterine lumen; Uw, uterine wall; (a), Hematoxylin & Eosin stain; (b), SEM; (c), TEM; (d), toluidine blue stain
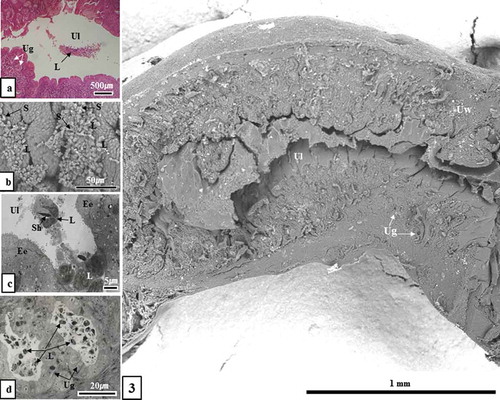
Figure 4. Scanning electron microscopic and transmission electron microscopic images of sperm kill in uterine lumen and uterine gland. In the experimental group around the last 10 days of January, a large number of sperm was phagocytosed by leukocytes in the uterine lumen (Figure 4(a, b)) and uterine gland (Figure 4(c)). In the experimental group around the end of February, an extremely small number of sperm were phagocytosed by leukocytes in the uterine lumen (Figure 4(d, e)). In particular, in the experimental group in February, no sperm was observed in the uterine gland as a result of phagocytosis by leukocytes (Figure 4(f)). Ec, endometrial epithelial cell; L, leucocyte; Ly, lysosome; Mv, microvilli; N, nucleus; S, sperm; Sh, sperm head; Ug, uterine gland; Uge, uterine gland epithelium; Ul, uterine lumen. (a, c, d and f), SEM; (b, e), TEM
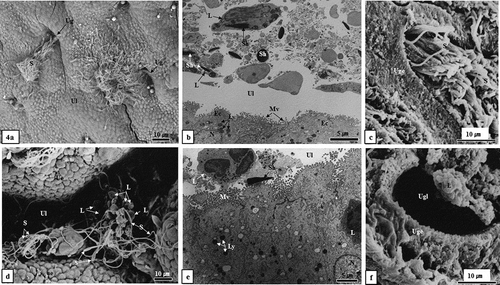
Figure 5. Images of the uterus before ovulation and ovulated ovum, and post-delivery litters and the uterus during the nurturing period. Endometrium was wrinkled approximately at the end of March before ovulation (Figure 5(a)). Note the ovulated ovum (Figure 5(b)) and the appearance of the cub after giving birth (Figure 5(c)). While the uterus approximately the end of July was not wrinkled but even (Figure 5(d)). In particular, it should be noted that no sperm were observed in the uterine lumen before ovulation (the early of March) (Figure 5(a)) and during the nurturing period (the end of July) (Figure 5(d)). Cc, cumulus cell; N, nucleus; Ul, uterine lumen; z, zona pellucida. (a), early-March; (b), late-March; (c), mid-June; (d), late-July
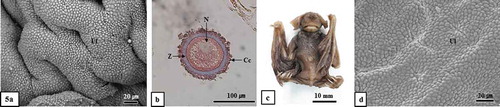
Figure 6. Optical and scanning electron microscopic images of the caudal isthmus of oviduct and sperms stored Therein. It should be noted that no sperm was observed in the caudal isthmus of the oviduct (Figure 6(a, b)). The epithelium of the caudal isthmus of the oviduct consists of ciliated epithelial cells and non-ciliated epithelial cells (Figure 6(c)). It should be noted that sperm were stored between the ciliated epithelial cells of the caudal isthmus of the oviduct (Figure 6(d)). Ce, ciliated epithelial cell; Cil, caudal isthmus lemen; Ne, non-ciliated epithelial cell; Oe, oviductal epithelium; S, sperm; (a), hematoxylin & Eosin stain; (b-d), SEM; (a and b), mid-August; (c), mid-September; (d), late-December
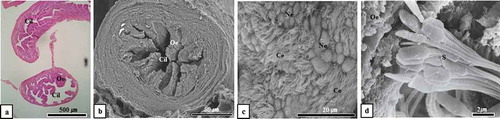
Figure 7. General mimetic diagram of relationship between sperm storage and loss in reproductive tract during female annual reproductive cycle of Korean hibernating greater horseshoe bats (R. ferrumequinum korai). The reproductive cycle is divided into six stages. The first stage is the mating period (the end of August). The second stage is the cleansing period (from the end of August to the end of February of the next year). During the cleansing period, some of sperm ejaculated after mating are phagocytosed and killed by leukocytes and this process proceeds gradually until February of the next year. From ovulation to the gestation and nurturing period, no sperm exist in the uterine lumen and uterine gland (, ). Sperm survival and storage occur in the caudal isthmus of the oviduct. The third stage is from the end of August to the end of September. When sperm in the uterine lumen move towards the uterotubal junction and are stored in the caudal isthmus of the oviduct. Only the sperm reaching the caudal isthmus of oviduct were found to be engaged in fertilization in the next year, and the duration of sperm storage was approximately 170 days. The fourth stage is the ovulation and fertilization period (the end of March). The fifth stage is the gestation period (from the end of March to the end of May) (approximately 70 days). The sixth stage is delivery (early and mid-June) and the nurturing period (from the end of June to mid-August) (nurturing period: approximately 80 days). †When sperm in uterine lumen move towards the uterotubal junction
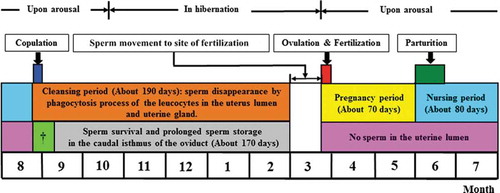
Morphological changes and characteristics of female reproductive tract per month
Change in the size of the uterus
There was no change in size of the uterus observed from the mating period (the end of August) to ovulation and implantation period (approximately the end of March) (-)). Meanwhile, the size of the uterus expanded during the gestation period, April (from the end of March to the end of May) ()), reaching a maximum in May ()). The size of uterus was reduced remarkably during the delivery period (early and middle of June) and the nurturing period in June ()) and July ()).
Prolonged sperm storage and sperm fate in female reproductive tract per month
Sperm fate in the uterine lumen, uterine gland and vagina
Approximately at the end of August, the mating period, some of the sperm ejaculated immediately after mating were phagocytosed by secretion of vaginal epithelial cells ()). In particular, an extremely small number of sperm was phagocytosed by leucocytes (), while penetration and satiating activity by leucocytes were not observed from the uterine gland (). A greater number of sperm were phagocytosed by leucocytes in the uterine lumen approximately at the end of September, compared to the experimental group in August, while an extremely small number of sperm was phagocytosed by leucocytes in the uterine gland (). Phagocytosis by leucocytes was observed from the uterine lumen and uterine gland approximately at the end of October, compared to that at the end of September (). In November and December, phagocytosis by leucocytes reached a peak in the uterine lumen and uterine gland (; ). During January of the next year, a number of phagocytosis by leucocytes was observed from the uterine lumen and uterine gland (; ). Meanwhile, during February, phagocytosis by leucocytes caused an extremely small number of sperm to be killed by leucocytes in the uterine lumen (; ). Differing from the experimental group of January, only the leucocytes existed in the uterine gland (, ). Such phagocytosis by leucocytes in the uterine lumen was observed from the end of August to the end of February in the next year (). Sperm were not observed from the uterine lumen until ovulation (early and middle of March) (); ). Ovulation occurred approximately at the end of March (); ), and delivery occurred approximately at the early and middle of June (); ). During the nurturing period (from the early of June to middle August), sperm were not observed from the uterine lumen (); ).
Sperm fate in the uterotubal junction
The uterotubal junction, the entry to the oviduct from the uterus, divided into two parts, colliculus tubaricus and intramural part of the uterotubal junction was observed, and the result thereof is below.
Situation in the colliculus tubaricus
The colliculus tubaricus is the first gate where sperm ejaculated after mating pass the uterus and moving to the oviduct, referring to the well-developed site in which the oviduct is stretched to the uterine lumen. According to the result of this study, sperm failed to reach the colliculus tubaricus approximately the last 10 days of August, the starting point of mating (). A number of sperm reached the colliculus tubaricus September–February of the next year and no phagocytosis by leucocytes was observed (). Also, sperm were not observed from the colliculus tubaricus approximately at the end of March when ovulation and implantation occurred, as well as penetration by leucocytes and sperm were not observed from the colliculus tubaricus during the gestation (from the end of March to the end of May) and nurturing (June and July) periods and until August ().
Situation in the intramural part of the uterotubal junction
The situation in the intramural part of the uterotubal junction led to almost same result as the situation in the colliculus tubaricus described above (). The only difference was that an extremely small number of sperm was observed from the colliculus tubaricus and intramural part of the uterotubal junction during January and February, while no sperm was observed from the intramural part of the uterotubal junction from March to the end of August ().
Sperm storage in the caudal isthmus of the oviduct
Some of the sperm that ejaculated during the mating-starting period (the end of August) was captured by the secretion of vaginal epithelial cells ()), among which some flowing into the uterus were killed by leukocytes (). Most of sperm ejaculated into the uterus approximately in August failed to reach the caudal isthmus of the oviduct (; ). In the experimental group from March to August, sperm were not observed from the caudal isthmus of the oviduct ().
However, sperm alive in the uterus (in the experimental group September-mid-February) passed through the uterotubal junction and reached the caudal isthmus of the oviduct in which it was observed as alive for long-term hibernation (; )), with no presence of leukocytes ().
Discussion
Morphological changes and characteristics of female reproductive tract per month
According to the results of this study, there was no major change in the size of the uterus from the end of August (mating-starting period) ()) to March of the next year ()). Meanwhile, remarkable change appeared approximately at the end of April ()). In other words, the appearance of the uterus largely expanded in April, resulting from fertilization by sperm and egg ovulating approximately the end of March already and consecutive embryogenesis. The pregnancy period was approximately 70 days (from the end of March to the end of May (-j)), and delivery occurred approximately early and mid-June (), ) and ; ). Approximately the early of June, immediately after delivery, the size of the uterus remarkably reduced ()). It suggests that this period is for nurturing (approximately 80 days) when much energy is consumed to nurture litters.
Sperm storage and fate in the female reproductive tract
As shown in , during hibernation, sperm survived in the uterus for a number of months and fertilized with the egg, ovulated in spring of the next year while attaining fertility capacity in Pipistrellus tralatitius abramus (Uchida Citation1950, Citation1953), P. abramus abramus (Hiraiwa & Uchida Citation1955, Citation1956), P. abramus (Mōri & Uchida Citation1974; Uchida & Mōri Citation1974b; Uchida et al. Citation1988), P. pipistrellus (Racey & Potts Citation1970) belonging to Vespertilionidae. This phenomenon also appears in Myotis lucifugus, Epitesicus fuscus (Wimsatt Citation1942, Citation1944). Accordingly, Uchida and Mōri (Citation1974b) have reported that infiltration by leukocytes does not occur in the uterus where sperms are stored during the winter and that the intrauterine accumulation of sperm plays a crucial role in the survival of an appropriate number of sperm by limiting contact between sperm and leukocytes penetrating into the uterine lumen and as the site where acrosome reaction is induced. Mōri and Uchida (Citation1974) describe that sperm are digested as a part of the phagocytosis by epithelial cells of fallopian tube in Japanese house bats, Pipistrellus abramus.
Meanwhile, survival and storage of sperm in the uterus and caudal isthmus of the oviduct have been reported in Indian P. ceylonicus chrysothrix (Gopalakrishna & Madhavan Citation1971) and Tylonycteris pachypus, T. Robustula (Medway Citation1972) as well as Scotophilus heathi (Krishna & Dominic Citation1978). Also, almost no leukocyte were reported in P. pipistrellus, Nyctalus noctula, Myotis nattereri and M. Daubentoni as well as Rhinolophus ferrumequinum, R. Hipposideros (Racey Citation1975, Citation1979). In South African Pipistrellus rusticus species, no leukocyte was found in the oviduct and uterine lumen and some of the sperm may be phagocytized by uterine epithelial cells while a number of sperm may be rapidly lost, suggesting the possibility of exposure from the vagina after fertilization (Van der Merwe & Rautenbach Citation1990). According to Mattner (Citation1968, Citation1969a), the uterus where leucocytes penetrate and sperm storage are spatially separated from each other per animal species, which has effects in that sperm are protected from phagocytosis by leucocytes and a sufficient number of sperm necessary for fertilization is secured. Meanwhile, the caudal isthmus of the oviduct is the only space for sperm storage in Myotis formosus tsuensis species (Son et al. Citation1987), suggesting that the caudal isthmus of the oviduct is closely related to sperm survival when considering that sperm exist between non-ciliated epithelial cells in the caudal isthmus of the oviduct ()).
Meanwhile, sperm storage occurs in the UTJ (uterotubal junction) and the caudal isthmus of the oviduct, respectively, in P. savii velox and P. endoi (Son et al. Citation1988), in both of which sperm death are caused in the uterus. As influential factors on prolonged sperm survival and sperm storage, fructose detected from endometrial cells and a great volume of glycogen from non-ciliated epithelial cells in the caudal isthmus of the oviduct (Pipeistrellus: Racey Citation1975; Myotis lucifugus, M. velifer: Crichton et al. Citation1981; Rhinolophus ferrumequinum korai: Lee & Son Citation2000) serve as a nutrient source to prolong sperm storage as well as influence the ovary and prolonged survival of Graafian follicles (Wimsatt & Kallen Citation1957; Wimsatt & Parks Citation1966; Son et al. Citation1987). Additionally, Son et al. (Citation1988) imply that characteristics and amount of secretory granules in epithelial cells are closely related to the amount of sugar molecules covering the epithelial microvilli. For the Murina leucogaster species, the major site of sperm storage is the uterotubal junction; the sperm that head towards the non-ciliated epithelial cells are closely related to the well-developed microfibril the amount of sugar molecules after ovulation are swallowed by non-ciliated epithelial cells covered by a large amount of glycocalyx (Mōri et al. Citation1989).
Meanwhile, for R. ferrumequinum nippon (Mōri et al. Citation1982; Oh et al. Citation1985) R. ferrumequinum korai (Kim & Oh Citation1991; Lee & Son Citation2000) which belong to Rhinolophus, sperm are killed in the uterus and an extremely small number of sperm survive and are stored in the intramural part of the uterotubal junction (R. ferrumequinum insulanus: Racey Citation1975, Citation1979; R. ferrumequinum korai: Lee & Son Citation2000). In this study, an extremely small number of sperm were observed in the colliculus tubaricus and intramural part of the uterotubal junction (). However, most of the sperm were reported surviving in the caudal isthmus of the oviduct (Gopalakrishna & Madhavan Citation1971; Medway Citation1972; Krishna & Dominic Citation1978; Mōri & Uchida Citation1980, Citation1981a, Citation1981b, Citation1982; Oh et al. Citation1985; Lee & Son Citation2000). A similar result was obtained in this study ().
Meanwhile, for Miniopterus schreibersii fuliginosis of delayed implantation type, leukocytes were noted to penetrate the uterus before mating (Uchida & Mōri Citation1974a); however, it was found to penetrate the uterus immediately after mating in this study (; inset b, c)). For M. schreibersii fuliginosis, intrauterine sperms were all killed and the uterotubal junction and caudal isthmus of the oviduct were determined as the space for sperm survival and storage (Uchida & Mōri Citation1974a; Mōri & Uchida Citation1980, Citation1981a, Citation1981b, Citation1982). Additionally, ovulation and fertilization occurred for the first time a number of days after mating (Mōri & Uchida Citation1980, Citation1981a, Citation1981b, Citation1982). A similar result was obtained from this study () and ).
For the Pipistrellus abramus (Uchida & Mōri Citation1974b), Myotis lucifugus, and M. velifer (Krutzsch et al. Citation1982), phagocytosis by sperm is caused by endometrial epithelial cells and phagocytes during the hibernation period. Based on this fact, Krutzsch et al. (Citation1982) highlight that sperm survival should be based on the capacity of sperm in the female reproductive system. However, according to this study, sperm were gradually killed by leukocytes in the uterine lumen, secretion in the uterine gland, and phagocytosis by leukocytes (, and ). Such phagocytosis is to keep the intrauterine environment clean and facilitate implantation of the fertilized egg. This period may be considered a cleansing period ().
There are three barriers against sperm transfer, including the uterine cervix, uterotubal junction and caudal isthmus of the oviduct in the female reproductive system (Hafez Citation1974), in which unnecessary matters as well as the destroyed sperms were removed from the fertilization site and female reproductive system. A number of study results showed that sperm were removed by enzyme lysis and phagocytosis caused by polymorphonuclear neutrophilic leucocytes in the vagina (Beaver Citation1960; Howe & Black Citation1963; Phillips & Mahler Citation1977a, Citation1977b) and uterus (Austin Citation1957, Citation1960), phagocytosis by leukocytes penetrated the uterine cervix (Mattner Citation1969a, Citation1969b), oviduct (Howe Citation1967), etc., and absorptive action by the oviductal epithetial cells (Zamboni Citation1971) and cervical epithelium (Rasweiler Citation1987). Meanwhile, for Japanese greater horseshoe bats (R. ferrumequinum nippon), sperm are phagocytized and digested by fibroblasts. Thereafter, sperm are deposited in the uterine lumen in addition to a large amount of secretion from the endometrial connective tissues infiltrated by polymorphonuclear neutrophilic leucocytes (Uchida et al. Citation1984). Sperm not involved in fertilization were removed by phagocytosis by macrophage (Oxberry Citation1979; Koyanagi & Nishiyama Citation1981). As mentioned earlier, there is a difference in sperm killing sites by species, considered to contribute to reproductive cycling by species.
Thus, the breeding cycle of a species should be explained on the basis of the breeding phenomenon in females and males. In other words, for the reproductive cycle of hibernating Korean greater horseshoe bats (R. ferrumequinum korai), mating is initiated August–September when spermatogenesis is active in males (Lee Citation2018a). During long-term hibernation after mating, immature sperm cells left in the male seminiferous tubule are completely removed by phagocytosis by Sertoli cells, which is called the cleansing period. Such phagocytosis suggests the preparation period to produce new sperm from testicles (Lee Citation2018a). Additionally, sperm left in the epididymis after mating and ejaculation are also removed by phagocytosis by leukocytes as well as secretion from testicular epithelium, also called the cleansing period to prepare to accept new sperm the next year by removing extra sperm left in the epididymis (April–June) when the first removal process occurs (Lee Citation2018b). As the second sperm removal process (July–August), purification occurs to be ready for maintaining mature sperm only by removing the deformed sperms introduced from the testicles and other residues (Lee Citation2018b). It is considered as a breeding strategy to improve fertility.
In the serial process of change occurring in the female reproductive tract, some of the sperm ejaculated to the uterus during the mating period (the end of August) are removed by phagocytosis of leucocytes. Such a process occurs until the end of February of the next year and is considered as to maintain the cleanliness of the intrauterine environment to facilitate implantation of the fertilized egg. Accordingly, this period may be called the cleansing period (approximately 190 days). Consecutive embryogenesis () proceeds, as survived sperm among them are stored in the caudal isthmus of the oviduct during long-term hibernation, moved to the fertilization site the next year, and infuse with the egg for fertilization (; ). At this point, sperm storage, survival, and transfer in the female reproductive tract have a major impact on fertilization (Son et al. Citation1987). Also, according to this study, sperm initially infiltrated to the inside of the female reproductive tract are recognized as foreign materials and killed by leukocytes to maintain an appropriate number of sperm, considered as a physiological mechanism to prevent polyspermy and improve fertility. Fertilization may be favorable for the mid or late sperm group compared to the initial sperm group ejaculated inside of the female reproductive tract to infuse with the egg.
Accordingly, the female annual reproductive cycle in the Korean greater horseshoe bat (R. ferrumequinum korai) was classified as: (1) Mating period (the end of August), (2) Cleansing period (from the end of August to the end of February of the next year), (3) Sperm transfer period (when the intrauterine sperm move towards the uterotubal junction to be stored in the caudal isthmus of the oviduct), (4) Ovulation and fertilization period (the end of March), (5) Pregnancy period (from the end of March to the end of May) and (6) delivery (early and mid-June) and nurturing period (June-mid August). In particular, only the sperm reaching the caudal isthmus of the oviduct as the space for sperm storage engages in fertilization. For the annual female reproductive cycle in R. ferrumequinum korai, mating and ovulation as well as fertilization, implantation, delivery and the nurturing period occur approximately 1 month earlier than Rhinolophidae bats including most of the species belonging to Vespertilionidae (), suggesting that such change and difference in reproductive cycle may contribute to photoperiod and temperature change as insisted by Lee (Citation2018a, Citation2018b).
In conclusion, change in the size of the uterus during the female reproductive cycle is strongly related to the mating and delivery periods in hibernating Korean greater horseshoe bats (R. ferrumequinum korai). In particular, initial sperm that had been ejaculated into the female reproductive tract since the end of August were phagocytosed and killed by phagocytosis and leukocytes that had infiltrated into the uterus until the end of February of the next year. Such phagocytosis seemed to result in maintaining a clean intrauterine environment to facilitate of implantation of the fertilized egg. Also, phagocytosis by leukocytes was considered a physiological mechanism to kill the sperm penetrating the female reproductive tract by recognizing them as alien materials, to maintain the appropriate number of sperm at the fertilization site of the fallopian tubes, thus improving fertility. Accordingly, this period may be called the cleansing period (approximately 190 days from the end of August to the end of February of the next year). The sperm became extinct mainly in the uterine lumen, and sperm became extinct by secretion from the uterine gland and the phagocytosis by leukocytes.
Only the sperm reaching the caudal isthmus of the oviduct were found to be involved in fertilization in the next year. The sperm were stored at the caudal isthmus of oviduct for approximately 170 days. Ovulation and fertilization occurred at the end of March while embryogenesis appeared consecutively with implantation. The pregnancy period was approximately 70 days; delivery occurred around early and mid-June; and the nurturing period was approximately 80 days.
Geolocation information
Country: Korea, Republic of Region: Gyeongsangnam-do City: Changwon-si
Latitude: N35°11′ (Masan-si)
Longitude: E128°34′ (Masan-si)
Disclosure statement
No potential conflict of interest was reported by the author.
Additional information
Funding
References
- Austin CR. 1957. Fate of spermatozoa in the uterus of the mouse and rat. Journal of Endocrinology 14:335–342. DOI: 10.1677/joe.0.0140335.
- Austin CR. 1960. Fate of spermatozoa in the female genital tract. Journal of Reproduction and Fertility 1:151–156. DOI: 10.1530/jrf.0.0010151.
- Beaver DL. 1960. The hormonal induction of a vaginal leukocytic exudate in the germ-free mouse. The American Journal of Pathology 37:769–773.
- Christian JJ. 1956. The natural history of a summer aggregation of the big brown bat, Eptesicus fuscus fuscus. The American Midland Naturalist 55:66–95. DOI: 10.2307/2422322.
- Crichton EG, Krutzsch PH, Wimsatt WA. 1981. Studies on prolonged spermatozoa survival in Chiroptera—I. The role of uterine free fructose in the spermatozoa storage phenomenon. Comparative Biochemistry and Physiology Part A: Physiology 70:387–395. DOI: 10.1016/0300-9629(81)90195-x.
- Deshmukh GD, Dhamani AA. 2011. Seasonal testicular changes during the annual reproductive cycle of Indian flying fox Pteropus giganteus giganteus (Brunnich). Journal of Cell & Tissue Research 11:2943–2948.
- Gopalakrishna A, Madhavan A. 1971. Survival of spermatozoa in the female genital tract of the Indian vespertilionid bat Pipistrellus ceylonicus chrysothrix (Wroughton). Proceedings of Indian Academy of Sciences Section B 73:43–49.
- Gopalakrishna A, Madhavan A, Thakur RS, Rajgopal G. 1974. The Graafian follicle in some Indian bats. Current Science 43:400–402.
- Gopalakrishna A, Moghe MA. 1960. Observations on the ovaries of some Indian bats. Proceedings of National Institute of the Science of India 26:11–19.
- Gustafson AW. 1979. Male reproductive patterns in hibernating bats. Reproduction 56:317–331. DOI: 10.1530/jrf.0.0560317.
- Guthrie MJ. 1933. The reproductive cycles of some cave bats. Journal of Mammalogy 14:199–216. DOI: 10.2307/1373819.
- Hafez ESE. 1974. Gamete transport. In: Hafez ESE, Hafez B, editors. Reproduction in farm animals. 3rd ed. Philadelphia: Lea and Febiger. pp. 123.
- Hiraiwa YK, Uchida TA. 1955. Fertilization in the bat, Pipistrellus abramus abramus (Temminck): II. On the properties of semen stored in the uterus. Faculty of Agriculture, Kyushu University 15:255–266. DOI: 10.15017/21367.
- Hiraiwa YK, Uchida TA. 1956. Fertilization in the bat, Pipistrellus abramus abramus (Temminck): III. Fertilizing capacity of spermatozoa stored in the uterus after the copulation in the fall. Faculty of Agriculture, Kyushu University 15:565–574. DOI: 10.15017/21410.
- Howe GR. 1967. Leucocytic response to spermatozoa in ligated segments of the rabbit vagina, uterus and oviduct. Reproduction 13:563–566. DOI: 10.1530/jrf.0.0130563.
- Howe GR, Black DL. 1963. Spermatozoan transport and leucocytic responses in the reproductive tract of calves. Reproduction 6:305–311. DOI: 10.1530/jrf.0.0060305.
- Kawamoto K. 2003. Endocrine control of the reproductive activity in hibernating bats. Zoological Science 20:1057–1069. DOI: 10.2108/zsj.20.1057.
- Kim EJ, Oh YK. 1991. A study on the spermatogenesis and spermatozoa fate in the female reproductive tract of the Korean greater horseshoe bat (Rhinolophus ferrumequinum korai). Korean Journal of Electron Microscopy 21:14–28.
- Kimura K, Uchida TA. 1983. Ultrastructural observations of delayed implantation in the Japanese long-fingered bat, Miniopterus schreibersii fuliginosus. Reproduction 69:187–193. DOI: 10.1530/jrf.0.0690187.
- Kimura K, Uchida TA. 1984. Development of the main and accessory placentae in the Japanese long-fingered bat, Miniopterus schreibersii fuliginosuse. Journals of Reproduction and Fertility 71:119–126. DOI: 10.1530/jrf.0.0710119.
- Koyanagi F, Nishiyama H. 1981. Fate of spermatozoa that do not participate in fertilization in the domestic fowl. Cell Tissue Research 214. DOI: 10.1007/bf00235147.
- Krishna A, Dominic CJ. 1978. Storage of spermatozoa in the female genital tract of the vespertilionid bat, Scotophilus heathi. Reproduction 54:319–321. DOI: 10.1530/jrf.0.0540319.
- Krutzsch PH. 1975. Reproduction of the canyon bat, Pipistrellus hesperus, in Southwestern United States. American Journal of Anatomy 143:163–200. DOI: 10.1002/aja.1001430203.
- Krutzsch PH, Crichton EG, Nagle RB. 1982. Studies on prolonged spermatozoa survival in Chiroptera: A morphological examination of storage and clearance of intrauterine and cauda epididymal spermatozoa in the bats Myotis lucifugus and M. velifer. American Journal of Anatomy 165:421–434. DOI: 10.1002/aja.1001650406.
- Lee JH. 2018a. Male reproductive cycle of hibernating Korean greater horseshoe bat, Rhinolophus ferrumequinum korai (Chiroptera: Rhinolophidae): Annual cycle of the seminiferous epithelium and morphological changes of the testes. European Zoological Journal 85:104–117. DOI: 10.1080/24750263.2018.1447029.
- Lee JH. 2018b. Morphological changes of cauda epididymis, sperm infiltration into cauda epididymis, sperm storage and sperm disappearance of cauda epididymis in Rhinolophus ferrumequinum korai (Chiroptera: Rhinolophidae). European Zoological Journal 85:119–128. DOI: 10.1080/24750263.2018.1445786.
- Lee JH, Son SW. 2000. Sperm storage and disappearance in the reproductive tract of the female Korean greater horseshoe bat, Rhinolophus ferrumequinum korai, during the hibernation. Korean Journal of Electron Microscopy 30:21–44.
- Matthews LH. 1937. The female sexual cycle in the British house-shoe bats, Rhinolophus ferrum-equinum insulanvs Barrett-Hamilton and R. hipposidergs minutus Montagu. Transactions of the Zoological Society of London 23:224–266. DOI: 10.1111/j.1096-3642.1937.tb00339.x.
- Mattner PE. 1968. The distribution of spermatozoa and leucocytes in the female genital tract in goats and cattle. Reproduction 17:253–261. DOI: 10.1530/jrf.0.0170253.
- Mattner PE. 1969a. Differential leucocytic responses to spermatozoa in the cervix and the uterus in ewes. Reproduction 18:297–303. DOI: 10.1530/jrf.0.0180297.
- Mattner PE. 1969b. Phagocytosis of spermatozoa by leucocytes in bovine cervical mucus in vitro. Reproduction 20:133–134. DOI: 10.1530/jrf.0.0200133.
- Medway L. 1972. Reproductive cycles of the flat-headed bats Tylonycteris pachypus and T. robustula (Chiroptera: Vespertilioninae) in a humid equatorial environment. Zoological Journal Linnean Society of London 51:33–61. DOI: 10.1111/j.1096-3642.1972.tb00772.x.
- Mōri T, Oh YK, Uchida TA. 1982. Sperm storage in the oviduct of the Japanese greater horseshoe bat, Rhinilophus ferrumequinum nippon. Zoological Laboratory, Journal of Faculty Agriculture, Kyushu University 27:47–53.
- Mōri T, Son SW, Yoon MH, Uchida TA. 1989. Prolonged survival of the Graafian follicle accompanied with sperm storage and the subsequent early development in the female greater tube-nosed bat, Murina leucogaster. Journal of Faculty Agriculture, Kyushu University 34:1–22. Available: http://hdl.handle.net/2324/23883.
- Mōri T, Uchida TA. 1974. Electron microscopic analysis of the mechanism of fertilization in Chiroptera. II. Engulfment of spermatozoa by epithelial cells of the fallopian tube in the Japanese house bat, Pipistrellus abramus. Zoological Magazine 83:163–170.
- Mōri T, Uchida TA. 1980. Sperm storage in the reproductive tract of the female Japanese long-fingered bat, Miniopterus schreibersii fuliginosus. Reproduction 58:429–433. DOI: 10.1530/jrf.0.0580429.
- Mōri T, Uchida TA. 1981a. Ultrastructural observations of fertilization in the Japanese long-fingered bat, Miniopterus schreibersii fuliginosus. Reproduction 63:231–235. DOI: 10.1530/jrf.0.0630231.
- Mōri T, Uchida TA. 1981b. Ultrastructural observations of ovulation in the Japanese long-fingered bat, Miniopterus schreibersii fuliginosus. Journal of Reproduction Fertility 63:391–395. DOI: 10.1530/jrf.0.0630391.
- Mōri T, Uchida TA. 1982. Changes in the morphology and behaviour of spermatozoa between copulation and fertilization in the Japanese long-fingered bat, Miniopterus schreibersii fuliginosus. Journal of Reproduction and Fertility 65:23–28. DOI: 10.1530/jrf.0.0650023.
- Mutere FA. 1965. Delayed implantation in an equatorial fruit bat. Nature 207:780–780. DOI: 10.1038/207780a0.
- Oh YK, Mōri T, Uchida TA. 1985. Prolonged survival of the Graafian follicle and fertilization in the Japanese greater horseshoe bat, Rhinolophus ferrumequinum nippon. Journal of Reproduction and Fertility 73:121–126. DOI: 10.1530/jrf.0.0730121.
- Oxberry BA. 1979. Female reproductive patterns in hibernating bats. Journal of Reproduction and Fertility 56:359–367. DOI: 10.1530/jrf.0.0560359.
- Phillips DM, Mahler S. 1977a. Leukocyte emigration and migration in the vagina following mating in the rabbit. The Anatomical Record 189:45–59. DOI: 10.1002/ar.1091890104.
- Phillips DM, Mahler S. 1977b. Phagocytosis of spermatozoa by the rabbit vagina. The Anatomical Record 189:61–71. DOI: 10.1002/ar.1091890105.
- Racey PA. 1973. Environmental factors affecting the length of gestation in heterothermic bats. Journal of Reproduction and Fertility Supplement 19:175–189. [PMID: 4522371].
- Racey PA. 1975. The prolonged survival of spermatozoa in bats. In: Duckett JG, Racey PA, editors. The biology of the male gamete. London: Academic Press. pp. 385–416.
- Racey PA. 1979. The prolonged storage and survival of spermatozoa in Chiroptera. Reproduction 56:391–402. DOI: 10.1530/jrf.0.0560391.
- Racey PA, Potts DM. 1970. Relationship between stored spermatozoa and the uterine epithelium in the pipistrelle bat (Pipistrellus pipistrellus). Reproduction 22:57–63. DOI: 10.1530/jrf.0.0220057.
- Ramakrishna PA, Rao KVB. 1977. Reproductive adaptations in the Indian rhinolophid bat, Rhinolophus rouxi (Temminck). Current Science 46:270–271. Available: https://www.jstor.org/stable/24080102.
- Rasweiler JJ. 1987. Prolonged receptivity to the male and the fate of spermatozoa in the female black mastiff bat, Molossus ater. Reproduction 79:643–654. DOI: 10.1530/jrf.0.0790643.
- Reeder EM. 1939. Cytology of the reproductive tract of the female bat Myotis lucifugus lucifugus. Journal of Morphology 64:431–453. DOI: 10.1002/jmor.1050640304.
- Richardson EG. 1977. The biology and evolution of the reproductive cycle of Miniopterus schreibersii and M. australis (Chiroptera: Vespertilionidae). Journal of Zoology 183:353–375. DOI: 10.1111/j.1469-7998.1977.tb04193.x.
- Sharifi M, Ghorbani R, Fazeli A, Holt W. 2004. Evidence of sperm storage in Pipistrellus kuhlii (Chiroptera: Vespertilioniodae) in western Iran. Folia Zoologica 53:1–6.
- Son SW, Mōri T, Yoon MH, Uchida TA. 1988. Reproduction of two rare Pipistrellus species, with special attention to the fate of spermatozoa in their female genital tracts. Journal of Mammalogical Society of Japan 13:77–91. DOI: 10.11238/jmammsocjapan1987.13.77.
- Son SW, Yoon MH, Mōri T, Uchida TA. 1987. Sperm storage in the reproductive tract and prolonged survival of the Graafian follicle in the female orange whiskered bat, Myotis formosus tsuensis, of Korea. Journal of Mammalogical Society of Japan 12:1–14. DOI: 10.11238/jmammsocjapan1987.12.1.
- Uchida T. 1950. Studies on the embryology of the Japanese house bat, Pipistrellus tralatitius abramus (Temminck). Ⅰ. On the period of gestation and the number of litter. Science Bulletin of the Faculty of Agriculture, Kyushu University 12:11–14. DOI: 10.15017/21137.
- Uchida TA. 1953. Studies on the embryology of the Japanese house bat, Pipistrellus tralatitius abramus (Temminck). Ⅱ. From the maturation of the ova to the fertilization, especially on the behaviour of the follicle cells at the period of fertilization. Science Bulletin of the Faculty of Agriculture, Kyushu University 14:153–168. DOI: 10.15017/21284.
- Uchida TA, Mōri T. 1974a. Electron microscopic analysis of the mechanism of fertilization in Chiroptera. Ⅰ. Acrosomal reaction and consequence of death of the sperm in the Japanese long-fingered bat, Miniopterus schreibersi. Science Bulletin of the Faculty of Agriculture, Kyushu University 83:177–184.
- Uchida TA, Mōri T. 1974b. Electron microscopic analysis of the mechanism of fertilization in Chiroptera. Ⅱ. Engulfment of spermatozoa by epithelial cells of the fallopian tube in the Japanese house bat, Pipistrellus abramus. Zoological Magazine 83:163–170.
- Uchida TA, Mōri T, Oh YK. 1984. Sperm invasion of the oviducal mucosa, fibroblastic phagocytosis and endometrial sloughing in the Japanese greater horseshoe bat, Rhinilophus ferrumequinum nippon. Cell and Tissue Research 236:327–331. DOI: 10.1007/bf00214234.
- Uchida TA, Mōri T, Son SW. 1988. Delayed capacitation of sperm in the Japanese house bat, Pipistrellus abramus. Journal of the Mammalogical Society Japan 13:1–10. DOI: 10.11238/jmammsocjapan1987.13.1.
- Van der Merwe M, Rautenbach IL. 1990. Reproduction in the rusty bat, Pipistrellus rusticus, in the northern Transvaal bushveld, South Africa. Journal of Reproduction & Infertility 89:537–542. DOI: 10.1530/jrf.0.0890537.
- Vasantha I. 2016. Physiology of seasonal breeding: A review. Journal of Veterinary Science and Technology 7:331. DOI: 10.4172/2157-7579.1000331.
- Wallace GI. 1978. A histological study of the early stages of pregnancy in the bent-winged bat (Miniopterus schreibersii) in north-eastern New South Wales, Australia (30°27’S). Journal of Zoology 185:519–537. DOI: 10.1111/j.1469-7998.1978.tb03350.x.
- Wang Z, Liang B, Racey PA, Wang YL, Zhang SY. 2008. Sperm storage, delayed ovulation, and menstruation of the female rickett,s big-footed Bat (Myotis ricketti). Zoological Studies 47:215–221.
- Wimsatt WA. 1942. Survival of spermatozoa in the female reproductive tract of the bat. The Anatomical Record 83:299–307. DOI: 10.1002/ar.1090830208.
- Wimsatt WA. 1944. Further studies on the survival of spermatozoa in the female reproductive tract of the bat. The Anatomical Record 88:193–204. DOI: 10.1002/ar.1090880206.
- Wimsatt WA, Kallen FC. 1957. The unique maturation response of the Gaafian follicles of hibernating vespertilionid bats and the question of its significance. The Anatomical Record 129:115–132. DOI: 10.1002/ar.1091290110.
- Wimsatt WA, Krutzsch PH, Napolitano L. 1966. Studies on sperm survival mechanisms in the female reproductive tract of hibernating bats. I. Cytology and ultra- structure of intra-uterine spermatozoa in Myotis lucifugus. The American Journal of Anatomy 119:25–60. DOI: 10.1002/aja.1001190104.
- Wimsatt WA, Parks HF. 1966. Ultrastructure of the surviving follicle of hibernation and of the ovum-follicle cell relationship in the vespertilionid bat Myotis lucifugus. Symposia Zoological Society of London 15:419–454.
- Wimsatt WA, Trapido H. 1952. Reproduction and the female reproductive cycle in the tropical American vampire bat, Desmodus rotundus murinus. The American Journal of Anatomy 91:415–446. DOI: 10.1002/aja.1000910304.
- Zamboni L. 1971. Fine morphology of mammalian fertilization. New York, NY: Harper & Row.