Abstract
A huge progress has been achieved in mammalian in vitro technique. Instead, of the many trials to develop marine invertebrate cell cultures, only a few have obtained them and only from few tissues. Since in vitro cell culture of invertebrates could be very useful for many aspects of basic and applied science, in this work we investigate and describe the development of a technique for the establishment of cell cultures from gill, mantle and gonadic tissue of the Manila clam (Ruditapes philippinarum). We maintained viable cultures for up to 25 days. Culture viability and proliferation were tested with 3-(4,5-Dimethylthiazol-2-yl)-2,5-Diphenyltetrazolium Bromide (MTT) and with trypan blue, while an antibody against the ATP-dependent RNA helicase VASA, a protein expressed in the germline, and in multipotent stem cells of some animals, was used to verify the presence of these cell types. Following the described protocol: 1) explant resulted the better source to obtain cell cultures, when compared to enzymatic dissociation; 2) cultures of suspended cells were viable for longer period than adherent cells; 3) cell cultures obtained from tissues sampled in September-October performed better compared to other periods of the year, regarding maintenance and growth; 4) the tissue from which we obtained longer-lived cell cultures was gonadic tissue, especially form samples that show more undifferentiated germ cells and more VASA-stained cells. This study describes the challenges concerning the development of in vitro culture techniques for aquatic invertebrates.
Introduction
The aquatic environment is the final recipient of contaminants that result from industrial, agricultural and urban wastes. The complex mixture of these contaminants affects the environment and ecosystems, living organisms, and a wide range of processes, such as organ function and the reproductive status, thus influencing species survival, population size and ultimately biodiversity (Bickham et al. Citation2000; Dixon et al. Citation2002). Among the most problematic contaminants are carcinogenic and mutagenic compounds, since their effects may extend far from individual lifespan, acting across generations. The chemical analysis of water and sediment is one of the direct methods for the environmental evaluation of toxicity of complex mixtures which is limited by the availability of detection methods. Xenobiotics lead to variations in cellular or biochemical components, processes, structures or functions (“biomarkers”) in sentinel organisms (“bioindicators”) (Shugart et al. Citation2003). Different strategies have been applied using a number of biomarkers in different bioindicators to evaluate the pollutant-induced stress syndrome in aquatic environments (Viarengo et al. Citation2007; Cappello et al. Citation2018, Citation2019; Gornati et al. Citation2019; Caricato et al. Citation2019).
The genotoxic effect of the polluted environment in fishes (e.g., Lazzari et al. Citation2017, Citation2019) and bivalves was investigated applying different programs. In bivalve, for example, although a lot of genotoxicity biomarkers were examined in marine mussels, only a limited number were verified on a broad range of laboratory and field conditions (Dixon et al. Citation2002). The Manila clam Ruditapes philippinarum is another example from bivalves. It was utilized in biomonitoring environmental contaminants in the Lagoon of Venice (Da Ros & Nesto Citation2005; Matozzo et al. Citation2010, Citation2013; Matozzo & Marin Citation2010), and recent DNA microarray studies allowed to set up reliable gene-expression markers associated to chemical contamination (Moschino et al. Citation2012; Papo et al. Citation2014). R. philippinarum is used as marine bioindicator for heavy metals in marine ecotoxicology being it a sentinel animal with wide distribution, ease to collect, with a long-life cycle, and high tolerance to harsh environment for salinity and temperature.
One example of biomarker is the micronucleus (MN) test. This biomarker shows the accumulation of genetic damage during cell life, and it is one of the most commonly applied biomarkers for genotoxicity in aquatic animals. In bivalves, the MN test has been applied under various conditions. Applying the MN assay in the field is useful in discrimination and identification of genotoxicity events along a pollution gradient (e.g. polycyclic aromatic hydrocarbons, heavy metals, organochlorinated compounds) (Bolognesi & Hayashi Citation2011). This and similar types of assessments are perfect for biomonitoring certain substances directly in the field, however, to test the effects of particular substances/conditions, without the interference of other variables also present in the field, the animals must be transferred to the lab and kept for the duration of the experiment. Moreover, biofiltering animals, such as bivalves, need specific feeding and tanks connected to the sea or supplied with big filters to renew the water along the time of the experiment in the laboratory.
Applying in vitro methods is highly recommended but rarely considered (Laville et al. Citation2004). A number of efforts have been done in improving and applying in vitro techniques in aquatic ecotoxicology for economic and scientific considerations. Firstly, the application of in vitro models reduces the requirement of organisms, consequently reducing the need for complex apparatuses to recreate their original habitat. Secondly, in vitro techniques can be employed as useful, rapid, low cost and reliable screening (Olabarrieta et al. Citation2001). Furthermore, in vitro techniques allow to classify the toxic potential of various xenobiotics and to compare their impacts on distinct species at the cellular level under comparable toxicant exposure environments. Lastly, a major advantage of these methods is their importance in understanding the mechanisms of action in response to environmental pollutants at the cellular and molecular level (Gagnaire et al. Citation2004). Cell culture can therefore help in guiding and justifying in vivo studies with whole organisms or mesocosms (Blaauboer Citation2008; Gura Citation2008).
There are two approaches to develop primary cell cultures: enzymatic dissociation or migration of cells from tissue parts (explants) into culture. Cells may proliferate in vitro, although the number of cell division for differentiated cells is usually limited, at least this is what has been known in mammals (Cristofalo et al. Citation2004; Lorenzini et al. Citation2005). On rare occasions, primary cells replicate indefinitely likely deriving from single cellular lineages, and are referred to as cell lines (Yoshino et al. Citation2013). Against the successful establishment of cell cultures, frequent problems are cellular damages occurring during tissue isolation and contamination with microorganisms. Overcoming these constraints is a hard task and has resulted in the study of a relatively small subset of tissues and cell types.
A lot of difficulties in mollusc cell cultivation explain the relatively low number of published reports involving primary-cell cultures from their tissues (Rinkevich Citation1999, Citation2005). Few mollusc species have been used for cell-culture studies, those with specific characteristics, for example: commercial value (e.g., oysters, clams, mussels, abalones), medical importance (snails hosts of human disease, e.g., Biomphalaria), or considered as model organisms for specific aspect (e.g., the gastropods Aplysia, Lymnaea and Helisoma in neurobiology). The sources for cell isolation have been representatives of the classes Bivalvia (Odintsova & Khomenko Citation1991; Renault et al. Citation1995) and Gastropoda (van der Merwe et al. Citation2010; Latire et al. Citation2012). Cell-culture establishment has been attempted from both larval (Odintsova & Khomenko Citation1991; van der Merwe et al. Citation2010) and adult tissues: gills (Domart-Coulon et al. Citation2000), heart (Pennec et al. Citation2002), hepatopancreas (Le Pennec & Le Pennec Citation2001), hemocytes (Latire et al. Citation2012), and mantle (Awaji & Suzuki Citation1998; Barik et al. Citation2004; Suja & Dharmaraj Citation2005; Endoh & Hasegawa Citation2006). There are a few studies in which the mussel Mytilus edulis was employed to make cell culture of mantle cells (Daugavet & Blinova Citation2015), gills and digestive glands (Faucet et al. Citation2004).
The physiology of marine bivalves and their response to environmental stimuli have attracted much interest from scientists (Venier et al. Citation2006; Chapman et al. Citation2009, Citation2011; Milan et al. Citation2011). With the observation of the exceptional longevity of the ocean quahog Arctica islandica (Ridgway & Richardson Citation2011), bivalves are becoming also an attractive model for ageing studies (Abele et al. Citation2009). Comparative biology of longevity determinants is an informative field that is progressively expanding its observations at the cellular level (Stuart et al. Citation2013; Croco et al. Citation2016, Citation2017b).
The aim of the present work is to develop in vitro cell culture of the Manila clam R. philippinarum, an important economic source, as it is largely consumed as seafood, and a model to study germline development (Milani et al. Citation2011, Citation2015a, Citation2015b, Citation2017, Citation2018), as well as a member of the same order, Veneroida, as A. islandica. The Manila clam is a filter-feeding organism with a worldwide distribution, that, differently from the sessile Mytilus, inhabits sandy-mud bottoms. We used gills, mantle, and gonadic tissue for the isolation of cells by explant or enzymatic dissociation. This protocol allowed us to obtain cell cultures with a good viability up to 25 days, in which the presence of putative, multipotent stem cells was documented by the immunological detection of VASA protein, a protein expressed in germline cells and in multipotent stem cells, with a broad function in developmental regulation (Yajima & Wessel Citation2011, Citation2015). Interestingly, the existence of putative multipotent stem cells was documented in all the produced cell cultures. In summary, we addressed the following issues: (1) optimize the best method of cell isolation, (2) determine cell activity during cultivation (ability of adhesion to the surface of culture vessels and MTT crystal formation), (3) use a specific anti-VASA antibody for R. philippinarum VASA homolog (anti-VASPH) (Milani et al. Citation2015a, Citation2015b) to detect of putative stem cells (Gustafson & Wessel Citation2010; Milani et al. Citation2018).
The described protocol can be used to obtain cell cultures useful for investigations in different fields of science: e.g. longevity determinants (Rabinowitz Citation2010) or germline cell differentiation and reproductive biology (Reunov et al. Citation2019).
Materials and methods
Sample collection and maintenance
Adult Manila clams were collected in 2017 (Goro Lagoon, Po River Delta, Ferrara, Northern Italy). Clams were transported to the laboratory where they were kept at constant temperature (19 ± 1°C) in 4-liter tanks (one clam per liter), with water of 25.00 ppt salinity (Reef salt, Aqua Medic GmbH, Bissendorf, Germany, used as marine salt mix) and aeration, for 1 day before making the tissue culture.
Tissues used in cell culture
Mantle
The mantle edge consists of three folds. The inner fold contains muscles (Richardson et al. Citation1981). The middle mantle fold bears tentacles and, presumably, fulfills sensory functions (Paillard & Le Pennec Citation1993). Cells in the groove between the middle and exterior folds secrete an organic material of the outermost shell layer-periostracum (Saleuddin Citation1974; Richardson et al. Citation1981). The epithelium of the exterior fold that faces toward the shell is involved in generation of the prismatic layer composed of an organic matrix and crystals of calcium carbonate (Bubel Citation1973; Richardson et al. Citation1981). Mantle has one physiological feature advantageous for maintenance in axenic culture (pure culture): the mantle edge is in permanent contact with the ambient medium and may be a place of parasite invasion (Allam et al. Citation2000), but it is also the site of synthesis of several antimicrobial peptides, as evidenced by some studies in Crassostrea gigas (Gonzalez et al. Citation2007; Schmitt et al. Citation2012) and in the larvae of Mytilus galloprovincialis, in which the expression of immunity-related genes takes place precisely in the cells of the mantle edge (Balseiro et al. Citation2013).
Gills
Gills are the filter feeding apparatus and the respiratory organ. Proliferating gill cells are more affected by genotoxic agents if compared with haemocytes due to the direct contact to pollutants (Bolognesi & Hayashi Citation2011). Gill cells have been analyzed in several species: freshwater mussels, Pyganodon cataracta, Utterbackia imbecillis and Ligumia substrata (Unionid mussels) (Kays et al. Citation1990; Schwartz & Dimock Citation2001); in vivo and in vitro analyses were performed using gill epithelium of the seawater mussel M. galloprovincialis (Gomez-Mendikute et al. Citation2005). Gills hold a considerable interest for the development of in vitro models due to the direct effect, in the paleal cavity of aquatic pollutants, on cell membranes of the outer epithelial layer of gills (Bigas et al. Citation2001; Le Pennec & Le Pennec Citation2001; Lopes et al. Citation2011a, Citation2011b).
Gonadic tissue
In mussels (e.g., Mytilus), the gonadic tissue develops inside the mantle, while in clams (e.g., R. philippinarum) it develops inside the visceral mass. In fact, in the Manila clam, during the reproductive season, there is a strict association between the intestine and the gonad, that develops as a soft, incoherent mass located inside the connective tissue intermingled to intestinal loops at the base of the foot (Devauchelle Citation1990; Milani et al. Citation2014). This species, as other gonochoric bivalves, exhibits seasonal gonad rebuilding: the gonad is absorbed after the spawning season, leaving no clue about the sex of the specimen, and is regenerated at the beginning of the following spawning season (Milani et al. Citation2015a, Citation2018). Organ-culture methods were established for gonads of the scallop Patinopecten yessoensis (Osada et al. Citation2003; Nakamura et al. Citation2007). In addition, Otani et al. (Citation2017) studied the role of sex steroids in scallops and Manila clam gonadal development, establishing a gonad culture system that was maintained for 14 days.
Procedure for cell culture
Animals were washed in fresh calcium- and magnesium-free artificial seawater solution (CMFSS): prepared by dissolving salts in deionized Super-Q water: 25.5 g/L NaCl, 0.8 g/L Na2SO4, 2.86 g/L HEPES (Daugavet & Blinova Citation2015). Shells were wiped with alcohol and were opened under sterile conditions. For other details see Supplementary Material.
Culture medium
The culture medium that gave the better results was L 15 medium (MP Biomedicals, United States) with 2% fetal bovine serum (FBS) and 0.6 mg/mL glutamine. Osmolarity was adjusted to 25 ppt by addition of salts: 18.05 g/L NaCl, 0.29 g/L KCl, 5.48 g/L MgCl2 6H2O, 4.28 g/L MgSO4 7H2O, 2.86 g/L HEPES (Sigma, United States), and 1.2 g/L CaCl2 2H2O. The pH was adjusted to 7.4–7.5 with 2 N NaOH. The medium was then filtered under sterile conditions through a membrane filter with 0.22 μm pore size. The culture medium and CMFSS were supplemented with 100 units/mL penicillin, 100 μg/mL streptomycin, 2.5 μg/mL amphotericin and 80 μg/mL gentamicin (based on Daugavet & Blinova Citation2015).
During preliminary trials, the following complex media were also tested. However, the medium described above gave the better results.
Tunicate Medium (cell culture medium composed as following):
Basic Medium (Modified Seawater):
Natural seawater 180 mL, MilliQ H2O 60 mL, 1 M HEPES 2.4 mL, Antibiotics (200×) 1.2 mL
[Antibiotics stock solution 200× (Penicillin G 200,000 U, Streptomycin 200 mg, 10 mL H20)]
RPMI 1640 with additives (RPMT 1640 (Gibco), 7.5% NaHCO3 50 mM 2-mercaptoethanol (MeEtOH).
Growth medium (RPMI 1640 with additives 85 mL + FBS 15 mL)
Growth medium mixture (immediately before the use, we mixed the Basic medium with the Growth medium in the 5:1 ratio).
Enzymatic dissociation
To obtain gill and mantle cells by enzymatic dissociation we have attempted different approaches: trypsin 0.1% (as in Daugavet & Blinova Citation2015) in PBS, down to a concentration 40 times lower, and collagenase 0.06 U/mL (as in Venier et al. Citation1997) in 2× Hank’s Balanced Salt Solution (HBSS), up to a concentration 200 times higher. The best result was obtained when single tissue fragments were placed in 1 mL of collagenase solution at the lowest concentration (0.06 U/mL) per tube. Samples were incubated for 5 min at RT in a shaker. The cell suspension was filtered through a nylon filters (180 µm and 80 µm) in sequence. Cells were collected by centrifugation at 300 g for 5 min. Supernatant was discarded, and the cell pellet was resuspended in the culture medium. Cell number and viability were determined by trypan blue (see below) and cells were seeded in 12-multiwell plates at 10,000 viable cells/cm2 according to the results of the viability determination. Cells were maintained in the growth medium (see above).
Explant
The mantle edge was excised from the posterior end of the mollusc with a sterile scalpel. Both mantle edge and isolated excised gills were washed sequentially in 3 wells containing sterile Alsever’s saline solution (NaCl 4.2 g/L, Citric Acid•3Na•2H2O 8.0 g/L, Citric Acid•H2O 0.55 g/L, D-Glucose 20.5 g/L, pH 7.5) (Venier et al. Citation1997), then transferred in 3 wells containing sterile CMFSS, and next cut into fragments (1–2 mm). To obtain cells from the explant culture, mantle and gill fragments were placed individually in 6-multiwell plates. Seven hundred microliters of culture medium (based on Daugavet & Blinova Citation2015, see above) were then added to each well. The gonadic tissue is located in the visceral mass above the foot and wrapped around the intestine. Whitish, gonadic tissue and small part of intestine, which may contain some putative primordial stem cells (Milani et al. Citation2017, Citation2018), were taken and positioned in 6-multiwell plates with 500 μl of media per well. After one week, we removed the tissues, seeded the cells at 10,000 viable cells/cm2 (gill, mantle, and gonadic cells) after evaluating viability with trypan blue.
Procedure for cell viability and proliferation
The suspended gill, mantle, and gonadic cells in culture were obtained by both explant cultures and enzymatic dissociation. However, due to the poor results obtained from enzymatic dissociation, we decided to proceed further only with cells derived from explants. Also, adherent cells were initially obtained from all the three tissues, but only for gonadic cells it was possible to keep them up to two weeks. For these reasons, we analyzed only suspended cells for viability and proliferation.
MTT viability test
This colorimetric assay is based on the reduction of the yellow tetrazolium salt to blue formazan by mitochondrial enzymes. The 3-(4,5-Dimethylthiazol-2-yl)-2,5-Diphenyltetrazolium Bromide (MTT) cell viability assay was developed by Mosmann for screening lymphocyte stimulation (Mosmann Citation1983) and was also adapted to measure the viability of invertebrate cells (Downs et al. Citation2010), including cultures of bivalve cells (Domart-Coulon et al. Citation2000). A stock solution was prepared by dissolving MTT in PBS at a 5 mg mL−1 final concentration. This solution was sterilized by filtration through a 0.22 µm filter, and stored at 4°C. MTT stock solution, 50 µL, was added directly to 500 µL of cell suspensions previously distributed into 24-multiwell plates and incubated for (24, 48, 72 h) at 10°C. At the end of the incubation, the precipitated formazan produced by the cells was dissolved by adding to each well 500 µL of SDS and left overnight at RT. Then, the amount of formazan was measured at λ = 570 nm (with a reference wavelength = 690 nm) with a spectrophotometer. All experiments were repeated three times.
Statistical analysis
All results are expressed as mean ± standard error of the mean (SEM). For the analysis of the vitality with MTT test among tissue, we used the one-way analysis of variance (ANOVA) for unpaired data, followed by Tukey test for multiple post comparison. All the statistical analyses were performed using Prism Software 7.
Trypan blue exclusion
Trypan blue exclusion was used to detect the viability of haemocytes in cell culture of bivalves and other invertebrates (Cao et al. Citation2007; Quinn et al. Citation2009; Downs et al. Citation2010). In a sample of cells suspension, trypan blue (TB) dye (0.4%) was added in a 1:1 ratio (1 of TB and 1 of the media containing suspended cells). The number of viable cells (excluding the dye) were counted with a Bürker chamber on an inverted microscope. Viability was measured when the cells were initially placed in suspension and up to 21 days. All experiments were repeated three times.
Protocol for VASA immunodetection in gill, mantle and gonad samples
Procedure for anti-VASA staining
The suspension was centrifuged at 200 g for 5 min. Then, the pellet was resuspended in 100 μL of L 15 medium, and the cytospin (32 g for 10 min) was used to allow the cells adhere to the slides.
Adherent gonadic cells
The medium with suspended cells was removed. Adherent cells were washed with new medium to verify they were actually adherent cells. For every well of 6–well plate, 200 µl collagenase (0.06 U/mL) was added and after 2 min 1 mL of L 15 medium was added to resuspend and pick up the cells. The suspension was centrifuged at 200 g for 5 min. Then, the pellet was resuspended in 100 μ of L 15 medium, and the cytospin (32 g for 10 min) was used to allow the cells adhere to the slides.
3-day immunocytochemistry procedure
DAY 1
Slides with attached cells (as prepared above) were fixed in 4% paraformaldehyde for 10 min at RT. Next, samples were washed in PBS (pH 7.4; Sigma-Aldrich) for 20 min with 3 changes. Antigenic sites were unmasked using 0.01% Pronase E in PBS, pH 7.2, for 4 min at RT. Samples were washed for 20 min, firstly with PBS, then with Tris-Buffered Saline (TBS) (pH 7.4; 10 mM Tris–HCl, 155 mM NaCl) with 0.05% Triton X-100 (TBS-0.05%T), and then permeabilized by leaving samples overnight at 4°C in TBS-0.1%T, pH 7.4.
DAY 2
Samples were placed in TBS-0.05% T. Non-specific protein-binding sites were blocked with 10% Normal Goat Serum (NGS) and 1% Bovine Serum Albumin (BSA) in TBS-0.1% T, pH 7.4, for 30 min at RT. Then, samples were incubated with an anti-VASA antibody specific for R. philippinarum VASA homologue (anti-VASPH-KFG; for antibody production, specificity and applications see (Milani et al. Citation2015a, Citation2015b, Citation2017, Citation2018), diluted 1:30,000 with TBS-0.05%T containing 3% BSA, pH 7.4. The incubation lasted 1 h 30 min at RT, followed by washes with TBS-0.05%T for 3 h. After washing, samples were incubated in the dark for 1 h at RT with the secondary antibody [1:400 polyclonal goat anti-rabbit Alexa Fluor 488 in TBS-0.1% T containing 1% NGS and 1% BSA, pH 7.4]. Samples were then washed for 1 h in TBS-0.05%T pH 7.4, left overnight at 4°C and washed again the day after.
DAY 3
The nuclear staining was performed with 0.5 μM TO-PRO-3 nuclear dye in PBS, pH 7.2, for 2 min at RT, in the dark, then the dye was washed for 4 h, firstly with PBS and then with TBS-0.05% T pH 7.4. Then, samples were mounted in anti-fade medium (2.5% DABCO, 50 mM Tris base pH 8, 90% glycerol). Slides were stored horizontally at 4°C in the dark and observed the day after. Images were recorded with a confocal laser scanning microscope (Leica confocal SP2 microscope), using Leica software.
Results
Cell-culture establishment from explant resulted the best performing method, with cell culture from gonadic explant being the most viable and metabolically active cells
Enzymatic dissociation was not as successful as the explant culture. We used trypsin and collagenase independently and at different concentrations. The viability of dissociated cells with all trypsin concentrations and with the higher collagenase concentration was lower than 50% for all the three tissues. Among the enzymatic dissociation approaches, the lowest collagenase concentration used (0.06 U/mL) seemed the gentler approach since it effected only slightly cell viability; this except for the gonadic tissue, for which the viability was less than 50% (data not shown). However, following enzymatic dissociation, cells took more time for growth when compared to explants and we did not see any adherent cells (),)).
Figure 1. Gill cells. (a) Explant of gills from the Manila clam after 4 days in culture showing migration of fibroblast-like (black arrow) and oval-shaped cells cells (red arrow) (Quinn et al. Citation2009). (b) Formazan crystals formed with gill cells after 3 weeks in culture. These crystals formed after 72 h of incubation with MTT and dissolved with SDS. (c) Explant of gills after 4 days in culture showing migration of explant cell (white arrow) and oval-shaped cells (red arrow). These cells were visible in culture around the tissue in the first days, and after the explants were removed they increased in number. (d) Example of dissociated gill cells after 4 days in culture; oval-shaped cells (red arrow) and suspended cells (green arrow). (e) Cells from gill explant after 2 weeks in culture; (f) after 4 weeks in culture. Clusters of cells suggesting proliferation (white arrow), oval-shaped cells (red arrow), and suspended cells (green arrow)
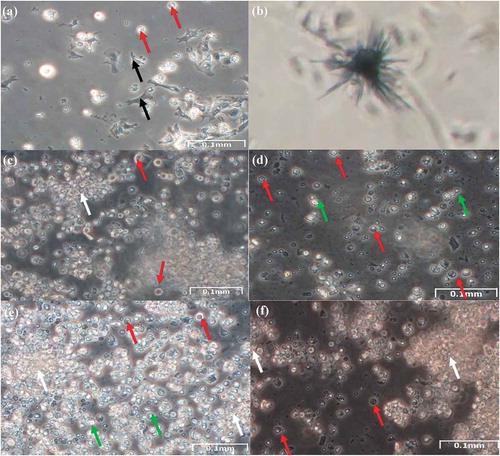
Figure 2. Mantle cells. (a) Explant of mantle from the Manila clam after 4 days in culture showing migration of explant cells (white arrow), and fibroblast-like (black arrow). (b) Formazan crystals formed with mantle cells after 3 weeks in culture. These crystals formed after incubation 72 h with MTT. (c) Example of dissociated mantle cells after 4 days in culture; oval-shaped cells (red arrow), and suspended cells (green arrow). (d) Cells from mantle explant after 2 weeks in culture; (e) after 4 weeks in culture. Clusters of cells suggesting proliferation (white arrow), oval-shaped cells (red arrow), and suspended cells (green arrow)
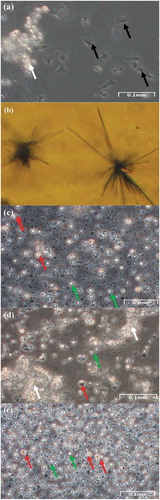
The culture became highly contaminated when using tunicate cell medium. Instead, L 15 medium with additives resulted in a better cell-culture maintenance, especially in September-October, for all tissues and especially for gonadic tissue.
To obtain cells from the gonad, the colorless crystals that contain hydrolytic enzymes were discarded since these may damage the cells.
We found large differences in viability depending on the sampling period, especially for samples obtained from gonadic tissues. September-October resulted to be the better period for cell-culture maintenance and growth, and, in this period, between 3 and 7 days after making explant culture of gills, mantle, and gonad, the cells began to migrate around the explant ().
Figure 3. Gonadic cells. (a) Growth curve of gonadic cells from the Manila clam from three different animals for three weeks. (b) Viability of gonadic cells in suspension 4 weeks in culture measured using the trypan blue exclusion test. Percentage of viable cells per square on a 1 mm3 graticule. Average counts from two different animals. (c) Suspended gonadic cells. Distribution of cells on a well surface after 4 days in culture; oocytes (green arrow); oval-shaped cells (red arrow). (d) Formazan crystals formed with gonadic cells after 3 weeks in culture. These crystals formed after incubation 72 h with MTT. (e) Epithelial or fibroblast-like cells (black arrow) from gonadic explant after 7 days. (f) Cells from female gonad explant after 2 weeks in culture; oocytes (green arrow). (g) Cells from male gonad explant after 2 weeks in culture; spermatozoa (green arrows). (h) Cells from female gonad explant after 4 weeks in culture, showing cluster suggesting proliferation (white arrow)
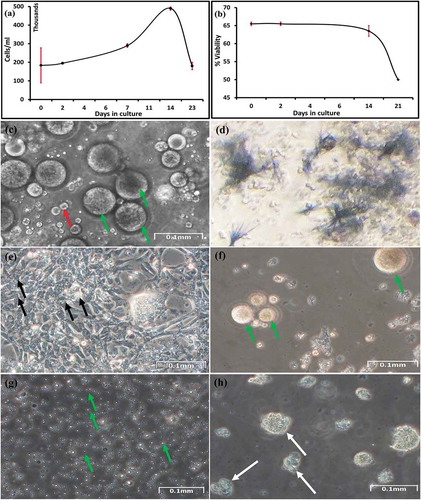
We observed different types of cells (). We examined cell morphology directly with an inverted microscope. In all cell cultures derived by the three different tissues, cells were found to adhere to the dishes (). Indeed, in the first days from the explant, adherent cells were epithelial or fibroblast-like cells (),),)). In the case of gonad explants, adherent cells are present for a longer period when compared to somatic cells (), and some of these fibroblast-like cells also appear to interact with growing cellular masses of oval-shaped cells, with which they make contact with structure like pseudopodia (-)). After 2 weeks from the gonad explant, the disintegration of such masses is accompanied by the disappearance of connected adherent cells. Thus, adherent gonadic cells were maintained in culture up to two weeks, while the period between appearance and disappearance of adherent cells in gill and mantle samples was very short.
Table I. Cell types obtained
Figure 4. Adherent cells. (a) Phase contrast image of adherent cells in mantle cell culture. (b) Phase contrast image of adherent cells in germ cell culture. (c-d) Phase contrast images of adherent cells in germ cell culture: explants are surrounded by a plenty of fibroblast-like cells. (c) Some smaller masses of cells appear to detach from the explant. (d) Fibroblast-like cell pseudopodia appear to be connected with the cell masses. (e) The same sample of (d), but a slightly different field, at phase-contrast
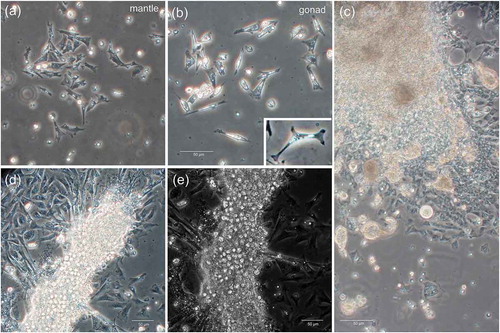
Besides the adherent cells, other cells were in suspension, floating, forming clusters. Some cells were specific to each tissue: for example, rounded gill cells with cilia, and oocytes and spermatozoa among gonadic cells ()), distinguishable for shape and dimensions.
In the cell cultures of all the three tissues, we observed clusters of cells suggesting proliferation (); ); )).
The growth of mantle and gill suspended cells reached the maximum after 2 weeks from the beginning of the explant culture (data not shown), then began to decrease. Also, the growth of gonadic suspended cells reached the maximum growth after 3 2 weeks from the beginning of the explant culture ()). The rate of growth was higher in gonadic cells ()) than in mantle and gill cells.
Gill, mantle and gonadic cells were viable for 25 days (); ), according to the appearance of blue formazan crystals which were abundant after 24 to 72 h of incubations with yellow tetrazolium salt (),),)). We had to use a long incubation time since incubation of few hours, as routinely done for mammalian cells, did not yield formazan crystals. The crystals increased with increasing incubation time (48–72 h). Cells of gonadic origin resulted significantly more viable than those of somatic lineages (gills and mantle) (p-value: gills vs gonads 0.0061; mantle vs gonad 0.0173); gills and mantle, instead, did not show significant difference between each other (p-value: mantle vs gills 0.8987). We also used trypan blue exclusion to detect the viability (e.g., )); however, this test was complicated by the presence of a high number of naturally coloured cells.
Figure 5. MTT viability test. Results are expressed as mean ± standard error of the mean (SEM). The results of MTT test between tissues were performed with the one-way analysis of variance (ANOVA) for unpaired data, followed by Tukey test for multiple post comparison. Statistically different values were obtained between gonadic cells and mantle cells (*) and between gonadic cells and gill cells (**). *P < 0.05, **P < 0.01, ***P < 0.001
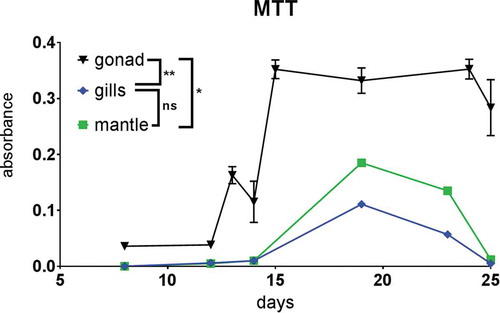
In April-August and November-December, we did not see any adherent cells for gills and mantle, and for gonadic cells the growth was scarce. Instead, in September-October, it was possible to obtain adherent cells for all three tissues, especially in gonadic samples in which adherent cells were present in culture for 2 weeks.
Immunodetection with anti-VASA antibody of putative multipotent stem cells
We used anti-VASPH (Milani et al. Citation2015b) to document the presence of VASA-expressing multipotent cells in the Manila clam R. philippinarum cultures. For such analysis we used samples from September–October, given the best results obtained in cell isolation and maintenance in that period. We tested three samples for each tissue. We found cells stained with anti-VASA in all the three tested cultures: gill, mantle, and gonadic cells. The VASA-staining was usually localized at one side of the nucleus, as previously reported for VASA-positive cells (Milani et al. Citation2015a, Citation2015b, Citation2017, Citation2018). We tested VASA immunostaining in suspended cells from the three tissues, and in gonadic adherent cells, since somatic adherent cells survived for a very short time.
In more detail, in gill samples at 2 weeks from the beginning of the explant culture, sparse cells are present ()), while, after 4 weeks, clusters of cells were detected ()). Some cells were VASA-positive ()) some forming tight groups ()). At the culture set up utilized, gills appeared to contain abundant VASA-stained cells compared to mantle.
Figure 6. VASA-immunostaining in somatic cells: gill (a-d) and mantle (e-h) cell cultures. (a,b) Phase contrast images of suspended cells obtained from gill explants: (a) 2 weeks, (b) 4 weeks. (c,d) Gill cells at confocal microscope: nuclear dye in green, VASA-staining in red. (d) A magnification of a cluster of VASA-stained cells from (c). (e,f) Phase contrast images of suspended cells obtained from mantle explant: (e) 2 weeks, (f) 4 weeks. (g,h) Mantle cells at confocal microscope: nuclear dye in green, VASA-staining in red. (g) At 2 weeks, sparse VASA-positive cells are visible (one cell magnified in the inset). (h) At 4 weeks, clusters of VASA-stained cells, with a faint labelling, are present in the culture (one cluster magnified in the inset)
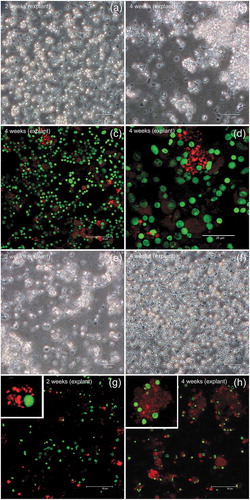
In mantle samples after 2 weeks from the beginning of the explant culture ()), some cells were VASA-positive ()) similar to what was observed for gill samples. After 4 weeks ()), masses of cells slightly labelled with anti-VASA are present, with a few cells showing a bright staining ()).
In samples from September–October, the best period to obtain cell culture, we got better results for the female gonadic samples than the male samples. Indeed, male germ cell culture ()) generally showed fewer VASA-stained cells and survived in culture for about 2 weeks, and then became contaminated, especially with fungi. Female specimens of the same sampling showed oocytes of 70–80 µm of diameter ()), dimension compatible with mature oocytes (Milani et al. Citation2018), but samples also included several VASA-stained cells (-i)). Oocytes did not show evident VASA labelling (,e)). Nonetheless, several VASA-positive cells were found (-i)). Indeed, during the first two weeks in culture, female gonadic cells appear to have proliferated ()) and clusters of VASA-positive cells were found (-i)). In addition, groups of VASA-positive adherent cells, some with a strong labelling, were also detected ().
Figure 7. VASA-immunostaining in gonadic cell cultures. (a-h) 10 days in culture. (a) Phase contrast image of male germ cells (mainly spermatozoa). (b) Male germ cells at confocal microscope: nuclear dye in green, VASA-staining in red. In the inset, a magnification of a VASA-stained cell. (c) Phase contrast image of female germ cells (many large, round cells, i.e. oocytes, are visible). (d,e) Oocytes at confocal microscope (meiotic chromosomes compatible with n = 19, 2 n = 38, submetacentric chromosomes, as previously described for R. philippinarum) (nucleus perimeter = dotted oval). Oocytes did not show a clear VASA-staining in their cytoplasm, but adjacent cells, with characteristic rounded nuclei with compact chromatin, show the VASA labelling (magnification of one of these cells in the inset in (d). (f-h) In germ cell cultures, both cells with VASA-staining and cells with no VASA-staining are visible. The VASA-staining is present as granules localized in the cytoplasm, at one side of the nucleus. (g) a cluster of VASA-stained cells (magnification of the dotted square in (f). (h) A VASA-stained cell adjacent to a putative early oocyte (both circled in dotted line). The VASA-staining at one side of the nucleus is well evident in the two cells in the inset. (i) After 3 weeks in culture, a majority of VASA-stained cells are present in the samples
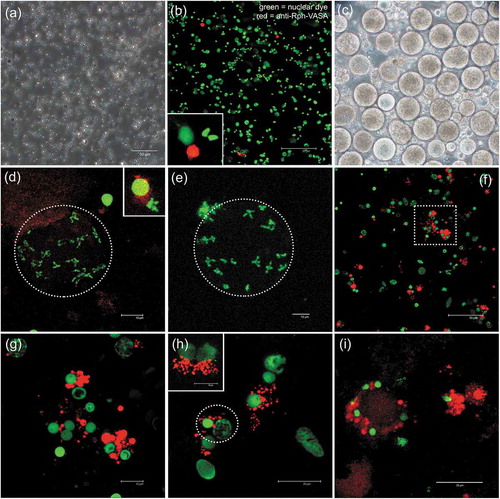
Figure 8. VASA-immunostaining in gonadic adherent cells. (a,b) Adherent cells of germ cell culture at confocal microscope: nuclear dye in green, VASA-staining in red. Both cells with VASA-staining and cells with no VASA-staining are visible. However, only few of them show VASA labelling. The inset in (b) shows a magnification of the VASA-stained cell in the dotted oval: the VASA-staining is present as granules localized in the cytoplasm. (c) The same cell is circled in a transmitted-light image of the same field, highlighting the oval shape of the cell. (d,e) Another example of adherent cells in germ cell culture at confocal microscope, description as in (a-c)
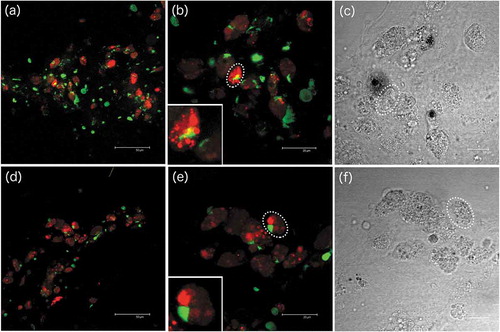
Discussion
To optimize the in vitro culture protocol, we worked on the culture media, on different cell-culture establishment techniques, and on different samplings over a period of 9 months (April–December 2017) in order to select the best conditions to obtain cell culture from three selected tissues: mantle, gills and gonadic tissue. The use of trypsin and of collagenase to dissociate cells was not as successful as letting the cells migrate from the explants. Following enzymatic dissociation, even at the lower collagenase concentration, which seemed to be the least harsh procedure, cells took more time for growth when compared with the cells migrating from the explants, and we did not observe adherent cells (,), suggesting that for enzymatic dissociation other less aggressive enzymes or enzyme cocktails should be tested.
In the first days after placing the explants in culture, we obtained adherent epithelial or fibroblast-like cells from all tissues tested. Migration from explant culture resulted in the best method for cell-culture production and the more consistent results were obtained with gonads, whose adherent cells were maintained in culture for 2 weeks. Although this approach to cell culture has generally a lower initial yield compared with enzymatic dissociation, in this case, explant culture seems the best approach to obtain viable and metabolically active cells from the three tissues of Manila clam.
The growth of somatic cells (from mantle and gills) and gonadic cells reached the maximum after 20 days and 2 weeks, respectively, then began to decrease ()). The rate of growth was higher in gonadic cells than in somatic cells. We can hypothesize that the gonadic tissue contains a greater amount of undifferentiated cells and/or stem cells, with higher capability of proliferating, when compared to somatic cell lineages, such as mantle and gill cells.
Gill, mantle and gonadic cells were alive for about 25 days, according to the formation of blue formazan crystals (),),)). To obtain formazan crystals, we had to use longer incubation times (48–72 h) than what is usually done for mammalian cells (few hours) (Croco et al. Citation2017a); this suggests a considerably lower metabolic activity in clam cells, compared to mammalian cells.
The selection of the suitable season for making culture of aquatic marine animals resulted to be extremely important, since huge differences in cell-culture parameters were documented during the tested period (e.g., growth of cells, type of cells recognized, and general condition of the culture). For example, in April–August and November–December, we did not see any adherent cells for gills and mantle, and for gonadic cells the growth was scarce. In April–August, the culture condition was possibly affected by the presence of easily recognizable, differentiated gametes that underwent degradation in the culture. In November–December, the animals were entering the sexual rest and it was difficult, almost impossible, to find and sample gonadic tissue. Instead, in September–October, it was possible to obtain adherent cells for all the three tissues, with adherent cells being present in the culture for few days in case of somatic samples and up to 2 weeks for gonadic samples, and suspended cells viable to about one month from all the three tested tissues (); ).
We documented the presence of putative, VASA-expressing multipotent cells in the Manila clam cell cultures. Interestingly, we found cells stained with anti-VASA in all the three tested cultures–gill, mantle and germ cells–using samples in September–October. In previous studies, VASA-positive cells, detected at the basal portion of the intestinal epithelium of R. philippinarum and of other bivalve species, that appear to proliferate during the reproductive season, were proposed to be multipotent cells able to migrate and differentiate into diverse cell types (Milani et al. Citation2015a, Citation2015b, Citation2017, Citation2018). The obtained finding appears to support a broader action of VASA (Yajima & Wessel Citation2011, Citation2015), as other studies have recently pointed out for other animals, suggesting that, in these cases, multipotent stem cells are maintained during the animal life span by expressing germline multipotency program genes, such as vasa, nanos and piwi (Juliano et al. Citation2010).
With the culture set up utilized, gills appeared to contain abundant VASA-stained cells compared to mantle, the other somatic tissue tested. This can be in some way related to the presence of particularly active niche of stem cells in such tissue, as documented in oysters (Jemaà et al. Citation2014).
In gill samples at 2 weeks, sparse cells are present while, after 4 weeks, clusters of cells were detected (compare and )). Interestingly, some cells were VASA-positive ()) and formed tight groups suggesting ongoing cell proliferation ()). In mantle samples after 4 weeks ()), cells appeared to have proliferated as masses of cells slightly labelled with anti-VASA, with a few cells showing a bright staining ()). This observation further supports the proliferation of VASA-stained cells also in mantle culture.
Cell culture from gonadic explant resulted in the most viable and metabolically active cells. Differences in the stage of gonad differentiation, depending on the sampling period, appear to have influenced the large differences in the viability of cells from gonadic tissues. We sampled from April to December because in this species no clear gonadic tissue is usually detectable from January to March that, in the north Adriatic Sea, is the period of sexual rest (the precise range of time can change year by year due to, for example, temperature oscillation and consequent food availability). Instead, the period of gametogenesis in the Goro Lagoon usually ranges from May to September, with spawning that happens normally in June–August. The prevalence of differentiated germ cells during the spawning season could have prevented cell-culture maintenance. Of course, when there is an abundance of differentiated cells, the proliferation activity should be at least slowed down by the presence of other cells, some of which, in the meanwhile, begin to degrade. This would actually affect the viability, maintenance and proliferation of still undifferentiated cells. For this reason, our expectation was a higher chance of culture establishment from proliferation of undifferentiated cells at the beginning of the reproductive season. Instead, the best result was obtained from sampling in September–October. This could be due to a particular responsiveness of undifferentiated germ cells at the end of the reproductive season. In fact, the presence of fewer undifferentiated germ cells in male samples ()) could have reduced the viability of male germ cell culture when compared to female germ cell culture of the same period (-)): male samples showed few VASA-stained cells and survived in culture for only about 2 weeks; instead, female samples included several VASA-stained cells (-)). Thus, this difference observed between the two sexes might be due to differences in the proportion of differentiated/undifferentiated cells in the analyzed specimens, being gametogenesis not synchronous between males and females (spermatogenesis completion usually preempts oogenesis; Devauchelle Citation1990). All these variables should be considered when starting a cell culture of germ cells.
Among gonadic adherent cells, groups of VASA-positive cells, some with a very strong labelling, were detected ()). For their shape, these VASA-positive adherent cells were similar to the oval-shaped cells that initially formed growing cellular masses and then dispersed ()). We can hypothesize that the VASA-stained cells were actually multiplying cells. Also, the morphology of the nuclei is compatible with cells that have recently divided (“telophase-like” stage; see inset in )). Overall, these immunological observations appear to support the multiplication of multipotent, VASA-stained cells. Additionally, in female germ cell culture, oocytes with meiotic-like chromosome set (as suggested by the chromosome number and appearance) were still detectable after 10 days in culture ()), supporting the possibility of maintaining oocytes in culture. Oocytes, normally blocked in prophase I (Yi et al. Citation2002), are described to dye shortly after spawning; in our experimental conditions, oocytes appeared with a normal morphology and chromosome structure up to 10 days. Their actual viability and fertilization performance should be tested in future studies on bivalve gamete and embryo preservation.
Conclusions
In summary, based on the present study, the explant culture using supplemented L15 medium resulted the most efficient approach to establish Manila calm culture. Among mantle, gills and gonads, the tissue more suitable to obtain cell culture, showing cells metabolically active for longer periods, is the gonadic tissue. Suspended cells thrive for longer time than adherent cells. September–October (at the end of the gametogenic period) resulted to be the best period for cell-culture establishment, maintenance and growth. The presence of VASA-stained cells in culture may indicate the presence of stem-like cells, and this surely deserves additional investigation. In the future, bivalve cell cultures obtained following the technique here reported could be used for genotoxicity test, for application in reproductive biology, or for the study of longevity determinants, thus allowing to have a more precise control of all experimental conditions.
Author contributions
A.A., S.M., A.L. and L.M. designed the research; A.A. performed cell-culture experiments, L.M. carried out immunological preparations; A.A., S.M., A.L. and L.M. analyzed the data; A.A., A.L. and L.M. wrote Introduction, Results, and Discussion. A.A. wrote Materials and methods; all authors discussed and commented on the manuscript.
Supplemental Material
Download PDF (221.8 KB)Acknowledgements
We would like to thank Emanuela Leoncini for help with the MTT assay and Maria Gabriella Maurizii for suggestions in immunological preparation.
Disclosure statement
The authors declare no competing interests.
Supplementary material
Supplemental data for this article can be accessed here.
Additional information
Funding
References
- Abele D, Brey T, Philipp E. 2009. Bivalve models of aging and the determination of molluscan lifespans. Experimental Gerontology 44:307–315. DOI: 10.1016/j.exger.2009.02.012.
- Allam B, Paillard C, Howard A, Le Pennec M. 2000. Isolation of the pathogen Vibrio tapetis and defense parameters in brown ring diseased Manila clams Ruditapes philippinarum cultivated in England. Diseases of Aquatic Organisms 41:105–113. DOI: 10.3354/dao041105.
- Awaji M, Suzuki T. 1998. Monolayer formation and DNA synthesis of the outer epithelial cells from pearl oyster mantle in coculture with amebocytes. Vitro Cellular & Developmental Biology-Animal 34:486–491. DOI: 10.1007/s11626-998-0083-0.
- Balseiro P, Moreira R, Chamorro R, Figueras A, Novoa B. 2013. Immune responses during the larval stages of Mytilus galloprovincialis: Metamorphosis alters immunocompetence, body shape and behavior. Fish & Shellfish Immunology 35:438–447. DOI: 10.1016/j.fsi.2013.04.044.
- Barik S, Jena J, Ram KJ. 2004. CaCO 3 crystallization in primary culture of mantle epithelial cells of freshwater pearl mussel. Current Science 86:730–734.
- Bickham JW, Sandhu S, Hebert PD, Chikhi L, Athwal R. 2000. Effects of chemical contaminants on genetic diversity in natural populations: Implications for biomonitoring and ecotoxicology. Mutation research/Reviews in Mutation Research 463:33–51. DOI:10.1016/S1383-5742(00)00004-1.
- Bigas M, Durfort H, Poquet M. 2001. Cytological effects of experimental exposure to Hg on the gill epithelium of the European flat oyster Ostrea edulis: Ultrastructural and quantitative changes related to bioaccumulation. Tissue & Cell 33:178–188. DOI: 10.1054/tice.2000.0169.
- Blaauboer BJ. 2008. The contribution of in vitro toxicity data in hazard and risk assessment: Current limitations and future perspectives. Toxicology Letters 180:81–84. DOI: 10.1016/j.toxlet.2008.05.008.
- Bolognesi C, Hayashi M. 2011. Micronucleus assay in aquatic animals. Mutagenesis 26:205–213. DOI: 10.1093/mutage/geq073.
- Bubel A. 1973. An electron-microscope study of periostracum formation in some marine bivalves. I. The origin of the periostracum. Marine Biology 20:213–221.
- Cao A, Novas A, Ramos-Martinez JI, Barcia R. 2007. Seasonal variations in haemocyte response in the mussel Mytilus galloprovincialis Lmk. Aquaculture 263:310–319. DOI: 10.1016/j.aquaculture.2006.11.004.
- Cappello T, Giannetto A, Parrino V, De Marco G, Mauceri A, Maisano M. 2018. Food safety using NMR-based metabolomics: Assessment of the Atlantic bluefin tuna, Thunnus thynnus, from the Mediterranean Sea. Food and Chemical Toxicology 115:391–397. DOI: 10.1016/j.fct.2018.03.038.
- Cappello T, Maisano M, Giannetto A, Natalotto A, Parrino V, Mauceri A, Spanò N. 2019. Pen shell Pinna nobilis L. (Mollusca: Bivalvia) from different peculiar environments: Adaptive mechanisms of osmoregulation and neurotransmission. The European Zoological Journal 86:333–342. DOI: 10.1080/24750263.2019.1673492.
- Caricato R, Giordano ME, Schettino T, Maisano M, Mauceri A, Giannetto A, Cappello T, Parrino V, Ancora S, Caliani I, Bianchi N, Leonzio C, Mancini G, Cappello S, Fasulo S, Lionetto MG. 2019. Carbonic anhydrase integrated into a multimarker approach for the detection of the stress status induced by pollution exposure in Mytilus galloprovincialis: A field case study. Science of the Total Environment 690:140–150. DOI: 10.1016/j.scitotenv.2019.06.446.
- Chapman RW, Mancia A, Beal M, Veloso A, Rathburn C, Blair A, Holland AF, Warr GW, Didinato G, Sokolova IM, Wirth EF, Duffy E, Sanger D. 2011. The transcriptomic responses of the eastern oyster, Crassostrea virginica, to environmental conditions. Molecular Ecology 20:1431–1449. DOI: 10.1111/j.1365-294X.2011.05018.x.
- Chapman RW, Mancia A, Beal M, Veloso A, Rathburn C, Blair A, Sanger D, Holland AF, Warr GW, Didonato G. 2009. A transcriptomic analysis of land-use impacts on the oyster, Crassostrea virginica, in the South Atlantic bight. Molecular Ecology 18:2415–2425. DOI: 10.1111/j.1365-294X.2009.04194.x.
- Cristofalo VJ, Lorenzini A, Allen RG, Torres C, Tresini M. 2004. Replicative senescence: A critical review. Mechanisms of Ageing and Development 125:827–848. DOI: 10.1016/j.mad.2004.07.010.
- Croco E, Marchionni S, Bocchini M, Angeloni C, Stamato T, Stefanelli C, Hrelia S, Sell C, Lorenzini A. 2017a. DNA damage detection by 53BP1: Relationship to species longevity. The Journals of Gerontology: Series A 72:763–770. DOI: 10.1093/gerona/glw170.
- Croco E, Marchionni S, Lorenzini A. 2016. Genetic instability and aging under the scrutiny of comparative biology: A meta-analysis of spontaneous micronuclei frequency. Mechanisms of Ageing and Development 156:34–41. DOI: 10.1016/j.mad.2016.04.004.
- Croco E, Marchionni S, Storci G, Bonafe M, Franceschi C, Stamato TD, Sell C, Lorenzini A. 2017b. Convergent adaptation of cellular machineries in the evolution of large body masses and long life spans. Biogerontology 18:485–497. DOI: 10.1007/s10522-017-9713-9.
- Da Ros L, Nesto N. 2005. Cellular alterations in Mytilus galloprovincialis (LMK) and Tapes philippinarum (Adams and Reeve, 1850) as biomarkers of environmental stress: Field studies in the Lagoon of Venice (Italy). Environment International 31:1078–1088. DOI:10.1016/j.envint.2005.05.018.
- Daugavet MA, Blinova MI. 2015. Culture of mussel (Mytilus edulis L.) mantle cells. Cell and Tissue Biology 9:233–243. DOI:10.1134/S1990519X15030037.
- Devauchelle N. 1990. Sexual development and maturity of Tapes philippinarum. In: Agostini D, Alessandra G, editors. Tapes philippinarum: Biologia e sperimentazione. 1st ed. Venice: Ente Sviluppo Agricolo Veneto. pp. 48–62.
- Dixon DR, Pruski AM, Dixon LRJ, Jha AN. 2002. Marine invertebrate eco-genotoxicology: A methodological overview. Mutagenesis 17:495–507. DOI: 10.1093/mutage/17.6.495.
- Domart-Coulon I, Auzoux-Bordenave S, Doumenc D, Khalanski M. 2000. Cytotoxicity assessment of antibiofouling compounds and by-products in marine bivalve cell cultures. Toxicology in Vitro 14:245–251. DOI: 10.1016/s0887-2333(00)00011-4.
- Downs CA, Fauth JE, Downs VD, Ostrander GK. 2010. In vitro cell-toxicity screening as an alternative animal model for coral toxicology: Effects of heat stress, sulfide, rotenone, cyanide, and cuprous oxide on cell viability and mitochondrial function. Ecotoxicology 19:171–184. DOI: 10.1007/s10646-009-0403-5.
- Endoh M, Hasegawa Y. 2006. Culture of mantle epithelial cells expressing shell matrix proteins from scallop Patinopecten yessoensis. Fisheries Science 72:1277–1285. DOI: 10.1111/j.1444-2906.2006.01286.x.
- Faucet J, Maurice M, Gagnaire B, Renault T, Burgeot T. 2004. Isolation and primary culture of gill and digestive gland cells from the common mussel Mytilus edulis. Methods in Cell Science 25:177–184. DOI:10.1007/s11022-004-8227-4.
- Gagnaire B, Thomas-Guyon H, Renault T. 2004. In vitro effects of cadmium and mercury on Pacific oyster, Crassostrea gigas (Thunberg), haemocytes. Fish & Shellfish Immunology 16:501–512. DOI: 10.1016/j.fsi.2003.08.007.
- Gomez-Mendikute A, Elizondo M, Venier P, Cajaraville M. 2005. Characterization of mussel gill cells in vivo and in vitro. Cell and Tissue Research 321:131–140. DOI: 10.1007/s00441-005-1093-9.
- Gonzalez M, Gueguen Y, Destoumieux-Garzon D, Romestand B, Fievet J, Pugniere M, Roquet F, Escoubas JM, Vandenbulcke F, Levy O, Saune L, Bulet P, Bachere E. 2007. Evidence of a bactericidal permeability increasing protein in an invertebrate, the Crassostrea gigas Cg-BPI. Proceedings of the National Academy of Sciences of the United States of America 104:17759–17764. DOI: 10.1073/pnas.0702281104.
- Gornati R, Maisano M, Pirrone C, Cappello T, Rossi F, Borgese M, Giannetto A, Cappello S, Mancini G, Bernardini G, Fasulo S. 2019. Mesocosm system to evaluate BF-MBR efficacy in mitigating oily wastewater discharges: An integrated study on Mytilus galloprovincialis. Mar Biotechnol 21:773–790. DOI: 10.1007/s10126-019-09923-9.
- Gura T. 2008. Toxicity testing moves from the legislature to the Petri dish—and back. Cell 134:557–559. DOI:10.1016/j.cell.2008.08.011.
- Gustafson EA, Wessel GM. 2010. Vasa genes: Emerging roles in the germ line and in multipotent cells. Bioessays 32:626–637. DOI: 10.1002/bies.201000001.
- Jemaà M, Morin N, Cavelier P, Cau J, Strub JM, Delsert C. 2014. Adult somatic progenitor cells and hematopoiesis in oysters. Journal of Experimental Biology 217:3067–3077. DOI: 10.1242/jeb.106575.
- Juliano CE, Swartz SZ, Wessel GM. 2010. A conserved germline multipotency program. Development 137:4113–4126. DOI: 10.1242/dev.047969.
- Kays W, Silverman H, Dietz T. 1990. Water channels and water canals in the gill of the freshwater mussel, Ligumia subrostrata: Ultrastructure and histochemistry. Journal of Experimental Zoology Part A: Ecological Genetics and Physiology 254:256–269. DOI:10.1002/jez.1402540304.
- Latire T, Le Pabic C, Mottin E, Mottier A, Costil K, Koueta N, Lebel JM, Serpentini A. 2012. Responses of primary cultured haemocytes from the marine gastropod Haliotis tuberculata under 10-day exposure to cadmium chloride. Aquatic Toxicology 109:213–221. DOI: 10.1016/j.aquatox.2011.09.017.
- Laville N, Ait-Aissa S, Gomez E, Casellas C, Porcher JM. 2004. Effects of human pharmaceuticals on cytotoxicity, EROD activity and ROS production in fish hepatocytes. Toxicology 196:41–55. DOI: 10.1016/j.tox.2003.11.002.
- Lazzari M, Bettini S, Milani L, Maurizii MG, Franceschini V. 2017. Differential response of olfactory sensory neuron populations to copper ion exposure in zebrafish. Aquatic Toxicology 183:54–62. DOI: 10.1016/j.aquatox.2016.12.012.
- Lazzari M, Bettini S, Milani L, Maurizii MG, Franceschini V. 2019. Differential nickel-induced responses of olfactory sensory neuron populations in zebrafish. Aquatic Toxicology 206:14–23. DOI: 10.1016/j.aquatox.2018.10.011.
- Le Pennec G, Le Pennec M. 2001. Acinar primary cell culture from the digestive gland of Pecten maximus (L.): An original model for ecotoxicological purposes. Journal of Experimental Marine Biology and Ecology 259:171–187. DOI: 10.1016/s0022-0981(01)00232-5.
- Lopes TM, Barcarolli IF, de Oliveira CB, de Souza MM, Bianchini A. 2011a. Effect of copper on ion content in isolated mantle cells of the marine clam Mesodesma mactroides. Environmental Toxicology and Chemistry 30:1582–1585. DOI: 10.1002/etc.528.
- Lopes TM, Barcarolli IF, de Oliveira CB, de Souza MM, Bianchini A. 2011b. Mechanisms of copper accumulation in isolated mantle cells of the marine clam Mesodesma mactroides. Environmental Toxicology and Chemistry 30:1586–1592. DOI: 10.1002/etc.527.
- Lorenzini A, Tresini M, Austad SN, Cristofalo VJ. 2005. Cellular replicative capacity correlates primarily with species body mass not longevity. Mechanisms of Ageing and Development 126:1130–1133. DOI:10.1016/j.mad.2005.05.004.
- Matozzo V, Binelli A, Parolini M, Locatello L, Marin MG. 2010. Biomarker responses and contamination levels in the clam Ruditapes philippinarum for biomonitoring the Lagoon of Venice (Italy). Journal of Environmental Monitoring 12:776–786. DOI: 10.1039/b920536e.
- Matozzo V, Giacomazzo M, Finos L, Marin MG, Bargelloni L, Milan M. 2013. Can ecological history influence immunomarker responses and antioxidant enzyme activities in bivalves that have been experimentally exposed to contaminants? A new subject for discussion in “eco-immunology” studies. Fish & Shellfish Immunology 35:126–135. DOI:10.1016/j.fsi.2013.04.013.
- Matozzo V, Marin MG. 2010. First evidence of gender-related differences in immune parameters of the clam Ruditapes philippinarum (Mollusca, Bivalvia). Marine Biology 157:1181–1189. DOI: 10.1007/s00227-010-1398-4.
- Milan M, Coppe A, Reinhardt R, Cancela LM, Leite RB, Saavedra C, Ciofi C, Chelazzi G, Patarnello T, Bortoluzzi S, Bargelloni L. 2011. Transcriptome sequencing and microarray development for the Manila clam, Ruditapes philippinarum: Genomic tools for environmental monitoring. BMC Genomics 12:234. DOI: 10.1186/1471-2164-12-234.
- Milani L, Ghiselli F, Maurizii MG, Nuzhdin SV, Passamonti M. 2014. Paternally transmitted mitochondria express a new gene of potential viral origin. Genome Biology and Evolution 6:391–405. DOI: 10.1093/gbe/evu021.
- Milani L, Ghiselli F, Maurizii MG, Passamonti M. 2011. Doubly uniparental inheritance of mitochondria as a model system for studying germ line formation. PLoS ONE 6:e28194. DOI: 10.1371/journal.pone.0028194.
- Milani L, Ghiselli F, Pecci A, Maurizii MG, Passamonti M. 2015a. The expression of a novel mitochondrially-encoded gene in gonadic precursors may drive paternal inheritance of mitochondria. PLoS One 10:e0137468. DOI: 10.1371/journal.pone.0137468.
- Milani L, Maurizii MG, Pecci A, Ghiselli F, Passamonti M. 2015b. Localization and dynamics of primordial germ cells in the bivalve species Ruditapes philippinarum. Molecular Reproduction and Development 82:406–407. DOI: 10.1002/mrd.22502.
- Milani L, Pecci A, Ghiselli F, Passamonti M, Bettini S, Franceschini V, Maurizii MG. 2017. VASA expression suggests shared germ line dynamics in bivalve molluscs. Histochemistry and Cell Biology 148:157–171. DOI: 10.1007/s00418-017-1560-x.
- Milani L, Pecci A, Ghiselli F, Passamonti M, Lazzari M, Franceschini V, Maurizii MG. 2018. Germ cell line during the seasonal sexual rest of clams: Finding niches of cells for gonad renewal. Histochemistry and Cell Biology 149:105–110. DOI: 10.1007/s00418-017-1607-z.
- Moschino V, Delaney E, Da Ros L. 2012. Assessing the significance of Ruditapes philippinarum as a sentinel for sediment pollution: Bioaccumulation and biomarker responses. Environmental Pollution 171:52–60. DOI: 10.1016/j.envpol.2012.07.024.
- Mosmann T. 1983. Rapid colorimetric assay for cellular growth and survival: Application to proliferation and cytotoxicity assays. Journal of Immunological Methods 65:55–63. DOI: 10.1016/0022-1759(83)90303-4.
- Nakamura S, Osada M, Kijima A. 2007. Involvement of GnRH neuron in the spermatogonial proliferation of the scallop, Patinopecten yessoensiss. Molecular Reproduction and Development 74:108–115. DOI: 10.1002/mrd.20544.
- Odintsova NA, Khomenko AV. 1991. Primary cell culture from embryos of the Japanese scallop Mizuchopecten yessoensis (Bivalvia). Cytotechnology 6:49–54. DOI: 10.1007/bf00353702.
- Olabarrieta I, L’Azou B, Yuric S, Cambar J, Cajaraville MP. 2001. In vitro effects of cadmium on two different animal cell models. Toxicology in Vitro 15:511–517. DOI: 10.1016/s0887-2333(01)00056-x.
- Osada M, Takamura T, Sato H, Mori K. 2003. Vitellogenin synthesis in the ovary of scallop, Patinopecten yessoensis: Control by estradiol-17 beta and the central nervous system. Journal of Experimental Zoology Part A Comparative Experimental Biology 299:172–179. DOI: 10.1002/jez.a.10276.
- Otani A, Nakajima T, Okumura T, Fujii S, Tomooka Y. 2017. Sex reversal and analyses of possible involvement of sex steroids in scallop gonadal development in newly established organ-culture systems. Zoological Science 34:86–92. DOI: 10.2108/zs160070.
- Paillard C, Le Pennec M. 1993. Ultrastructural studies of the mantle and the periostracal lamina in the manila clam, Ruditapes philippinarum. Tissue and Cell 25:183–194. DOI: 10.1016/0040-8166(93)90018-g.
- Papo MB, Bertotto D, Quaglio F, Vascellari M, Pascoli F, Negrato E, Binato G, Radaelli G. 2014. Histopathology and stress biomarkers in the clam Venerupis philippinarum from the Venice Lagoon (Italy). Fish & Shellfish Immunology 39:42–50. DOI: 10.1016/j.fsi.2014.04.016.
- Pennec J-P, Gallet M, Gioux M, Dorange G. 2002. Cell culture of bivalves: Tool for the study of the effects of environmental stressors. Cellular and Molecular Biology (Noisy-le-grand, France) 48:351–358.
- Quinn B, Costello MJ, Dorange G, Wilson JG, Mothersill C. 2009. Development of an in vitro culture method for cells and tissues from the zebra mussel (Dreissena polymorpha). Cytotechnology 59:121–134. DOI: 10.1007/s10616-009-9202-3.
- Rabinowitz JS. 2010. Centrosome-mediated RNA segregation: A novel patterning mechanism in Ilyanassa. Doctoral dissertation, University of Rochester, New York, NY.
- Renault T, Flaujac G, Le Deuff R-M. 1995. Isolation and culture of heart cells from the European flat oyster, Ostrea edulis. Methods in Cell Science 17:199–205. DOI:10.1007/BF00996127.
- Reunov A, Alexandrova Y, Reunova Y, Komkova A, Milani L. 2019. Germ plasm provides clues on meiosis: The concerted action of germ plasm granules and mitochondria in gametogenesis of the clam Ruditapes philippinarum. Zygote 27:25–35. DOI: 10.1017/S0967199418000588.
- Richardson CA, Runham NW, Crisp DJ. 1981. A histological and ultrastructural study of the cells of the mantle edge of a marine bivalve, Cerastoderma edule. Tissue and Cell 13:715–730. DOI: 10.1016/s0040-8166(81)80008-0.
- Ridgway ID, Richardson CA. 2011. Arctica islandica: The longest lived non colonial animal known to science. Reviews in Fish Biology and Fisheries 21:297–310. DOI: 10.1007/s11160-010-9171-9.
- Rinkevich B. 1999. Cell cultures from marine invertebrates: Obstacles, new approaches and recent improvements. Journal of Biotechnology 70:133–153. DOI: 10.1016/S0168-1656(99)00067-X.
- Rinkevich B. 2005. Marine invertebrate cell cultures: New millennium trends. Marine Biotechnology 7:429–439. DOI: 10.1007/s10126-004-0108-y.
- Saleuddin A. 1974. An electron microscopic study of the formation and structure of the periostracum in Astarte (Bivalvia). Canadian Journal of Zoology 52:1463–1471. DOI:10.1139/z74-188.
- Schmitt P, de Lorgeril J, Gueguen Y, Destoumieux-Garzon D, Bachere E. 2012. Expression, tissue localization and synergy of antimicrobial peptides and proteins in the immune response of the oyster Crassostrea gigas. Developmental & Comparative Immunology 37:363–370. DOI: 10.1016/j.dci.2012.01.004.
- Schwartz ML, Dimock RV. 2001. Ultrastructural evidence for nutritional exchange between brooding unionid mussels and their glochidia larvae. Invertebrate Biology 120:227–236. DOI: 10.1111/j.1744-7410.2001.tb00033.x.
- Shugart L, Theodorakis CW, Bickham AM, Bickham JW. 2003. Genetic effects of contaminant exposure and potential impacts on animal populations. Handbook of Ecotoxocology, Lewis Publisher, Boca Raton, 1129–1147.
- Stuart JA, Liang P, Luo X, Page MM, Gallagher EJ, Christoff CA, Robb EL. 2013. A comparative cellular and molecular biology of longevity database. Age 35:1937–1947. DOI: 10.1007/s11357-012-9458-y.
- Suja CP, Dharmaraj S. 2005. In vitro culture of mantle tissue of the abalone Haliotis varia Linnaeus. Tissue and Cell 37:1–10. DOI: 10.1016/j.tice.2004.08.002.
- van der Merwe M, Auzoux-Bordenave S, Niesler C, Roodt-Wilding R. 2010. Investigating the establishment of primary cell culture from different abalone (Haliotis midae) tissues. Cytotechnology 62:265–277. DOI: 10.1007/s10616-010-9293-x.
- Venier P, De Pitta C, Pallavicini A, Marsano F, Varotto L, Romualdi C, Dondero F, Viarengo A, Lanfranchi G. 2006. Development of mussel mRNA profiling: Can gene expression trends reveal coastal water pollution? Mutation Research/Fundamental and Molecular Mechanisms of Mutagenesis 602:121–134. DOI:10.1016/j.mrfmmm.2006.08.007.
- Venier P, Maron S, Canova S. 1997. Detection of micronuclei in gill cells and haemocytes of mussels exposed to benzo[a]pyrene. Mutation Research/Genetic Toxicology and Environmental Mutagenesis 390:33–44. DOI: 10.1016/s0165-1218(96)00162-0.
- Viarengo A, Lowe D, Bolognesi C, Fabbri E, Koehler A. 2007. The use of biomarkers in biomonitoring: A 2-tier approach assessing the level of pollutant-induced stress syndrome in sentinel organisms. Comparative Biochemistry and Physiology Part C: Toxicology & Pharmacology 146:281–300. DOI: 10.1016/j.cbpc.2007.04.011.
- Yajima M, Wessel GM. 2011. The multiple hats of Vasa: Its functions in the germline and in cell cycle progression. Molecular Reproduction and Development 78:861–867. DOI: 10.1002/mrd.21363.
- Yajima M, Wessel GM. 2015. Essential elements for translation: The germline factor Vasa functions broadly in somatic cells. Development 142:1960–1970. DOI: 10.1242/dev.118448.
- Yi J-H, Lefievre L, Gagnon C, Anctil M, Dube F. 2002. Increase of cAMP upon release from prophase arrest in surf clam oocytes. Journal of Cell Science 115:311–320.
- Yoshino TP, Bickham U, Bayne CJ. 2013. Molluscan cells in culture: Primary cell cultures and cell lines. Canadian Journal of Zoology 91:391–404. DOI: 10.1139/cjz-2012-0258.