Abstract
Myrmecophilous insects depend on their ant hosts during at least part of their life cycle. Lasius ants appear to be primarily involved as host in myrmecophilous interactions, especially with aphids and beetles, e.g. staphylinid. Pella laticollis is a rove beetle having a close ecological association with Lasius fuliginosus, which is correlated to its behavior adapted to avoid attack. Microorganisms can also play an important role in the maintenance of such relationships. We used 16S rRNA sequencing on the Illumina MiSeq platform to identify the bacterial communities associated with larvae and adults of both L. fuliginosus and P. laticollis. In addition, we determined the microbiota profiles of the nest-carton, an organic material lining the nest chambers. We obtained more than two million good quality reads. Taxonomic annotation showed that the profiles consisted of a total of 20 phyla, among which Proteobacteria was the most abundant. The samples were grouped according to the host’s developmental stage or the nest material, and the differences between those groups were significant. Only the microbiota of L. fuliginosus larvae and adults did not differ significantly. Analyses at the genus level indicated a heightened abundance of Pseudomonas in the insects’ profiles. The bacterial communities associated with the nest-carton included bacterial genera recorded from soil and dead wood. The profiles showed the presence of two well-known endosymbiotic bacteria, namely, Rickettsia and Wolbachia. According to our findings, the bacterial communities associated with larvae and adults of both L. fuliginosus and P. laticollis formed clusters according to the host’s identity and its developmental stage. The profiles determined for the nest-carton formed a separate group. Our study is in line with a new research trend that is focusing on microbiota associated not only with ants, but also with myrmecophiles and ant nests inhabited by those species.
Introduction
Insects have evolved non-pathogenic, persistent associations with various microorganisms. These microbes, particularly gut microbiota, have been shown to contribute to the host’s digestion, pathogen resistance, and physiology (Douglas Citation1998, Citation2009, Citation2015; Van Borm et al. Citation2002; Pinto-Tomas et al. Citation2009; Koch & Schmid-Hempel Citation2011; Raychoudhury et al. Citation2013; Engel & Moran Citation2013; Michalkova et al. Citation2014; Jing et al. Citation2020). The evolutionary success of insects has depended in part on these myriad relationships with beneficial microorganisms. Particular attention has been paid to the microbiota associated with social insects. Close interactions among host individuals, e.g. maternal care, food sharing, and grooming, facilitate the transmission of gut-associated bacteria, resulting in persistent associations, coevolution with hosts and the emergence of specialized symbiont lineages, as well as the competitive exclusion of unspecific microbes (Hongoh et al. Citation2005; Russell et al. Citation2009; Martinson et al. Citation2011; Anderson et al. Citation2012; Engel & Moran Citation2013; Kwong et al. Citation2017; Powell et al. Citation2018). Honeybees are important models in studies to determine the range of influences of microbial communities on their hosts and in understanding of the pathogenic and mutualistic components of the microbiota associated with these ecologically and economically important pollinators (Zheng et al. Citation2017; Jones et al. Citation2018; Wang et al. Citation2018; Raymann & Moran Citation2018; Kešnerová et al. Citation2020). The complex host-microbe relationships revealed by studies of honeybee microbiota have fuelled growing interest in the microbial communities associated with other social insects, such as ants.
The family Formicidae (Insecta, Hymenoptera) is an ecologically dominant group of invertebrates divided into 17 subfamilies and 333 genera. More than 13 000 known ant species occur worldwide (CitationBolton). The great diversity of ants is probably due to their ecological diversity, for example, as regards nesting, feeding preferences, social behaviour and the division of labor among castes (Wilson Citation1987; Hölldobler & Wilson Citation1990; Ramalho et al. Citation2017a). Analyses of the bacterial communities associated with ants have shown important aspects of host biology in structuring the diversity and abundance of these communities. Anderson et al. (Citation2012) found similarity of the microbiota among ants species from the same trophic level, and identified differences between the microbiome profiles of herbivorous and predatory species. In the case of herbivorous ants, e.g. Cephalotes turtle ants, gut bacteria take part in nitrogen recycling and the synthesis of essential amino acids that are acquired by the host in substantial quantities (Hu et al. Citation2018). A nutritional role of associated bacteria has been identified for Camponotini ants (Sameshima et al. Citation1999; Wernegreen et al. Citation2009; Ramalho et al. Citation2017a). Blochmannia, a Proteobacteria specific to this tribe, not only assists in supplying its hosts with essential amino acids, but also harbours genes necessary for the metabolism of nitrogen, sulphur and lipids (Gil et al. Citation2003; Degnan et al. Citation2005; Feldhaar et al. Citation2007; Williams & Wernegreen Citation2010). It has also been shown that the number of Blochmannia increases strongly during host’s metamorphosis and that aposymbiotic larvae have much-reduced capacity to complete metamorphosis (Feldhaar et al. Citation2007; Stoll et al. Citation2010). This trend implies an important function of Blochmannia in the developmental phase during which the hosts are metabolically very active. Moreover, the bacteria associated with various ants species can produce antibiotics against a range of bacterial and fungal pathogens. One example is the genus Pseudonocardia, associated with ants from the Attini tribe, which produces antibiotics to suppress Escovopsis, a genus of virulent microfungal parasites that attacks the basidiomycetous fungi cultivated as the main food source by these ants (Currie et al. Citation1999, Citation2003; Cafaro & Currie Citation2005; Sen et al. Citation2009; Oh et al. Citation2009; Mueller et al. Citation2010; Zucchi et al. Citation2010). However, the associated bacteria do not only directly affect the fitness of their hosts. Microorganisms can also shape or interrupt close relationships among ants and other animals (e.g. myrmecophiles), plants or fungi (for ant interactions see (North et al. Citation1997; Pierce et al. Citation2002; Heil & McKey Citation2003; Chomicki & Renner Citation2017; Nelsen et al. Citation2018; von Beeren et al. Citation2018; Casacci et al. Citation2019; Tartally et al. Citation2019)). In a recent study, Di Salvo et al. (Citation2019) identified two bacteria, Serratia mercescens and S. entomophila, which could be involved in the chemical cross-talk among microbiota, Maculinea larvae and Myrmica ants (Di Salvo et al. Citation2019). In turn, Fischer et al. (Citation2017) have shown that bacteria may contribute to the recognition of distant aphid species by Lasius niger ants (Fischer et al. Citation2017). These results strengthen interest in studying the role of microorganisms in shaping relationships among closely associated species (Szenteczki et al. Citation2019; Kaczmarczyk-Ziemba et al. Citation2020).
Lasius ants appear to be primarily involved as host in myrmecophilous interactions with other insects, especially aphids (Fischer et al. Citation2017), but also beetles, such as staphylinids (Akino Citation2002; Lapeva-Gjonova Citation2013). The genus Pella is a rove beetle that has a close ecological association with Lasius ants (Hölldolber et al. Citation1981). For example, Lasius fuliginosus (Latreille, 1798) has been reported as the host of six Pella species (P. humeralis, P. funesta, P. cognata, P. similis, P. lugens, and P. laticollis) (Hölldolber et al. Citation1981). These myrmecophiles are found inside the carton-nests built by their hosts in tree hollows. The nests are divided into chambers by thin walls. The material used, usually macerated wood but occasionally soil particles, is intermixed and overgrown by fungus mycelium (Cladosporium myrmecophilum). Collected honeydews as well as supplied sugar-water are used as adhesives for sticking the wood and soil particles together, while at the same time providing a culture medium for the fungus (Fresenius Citation1852; Lagerheim Citation1900; Elliott Citation1915; Maschwitz & Hölldobler Citation1970). Further analyses of fungal communities associated with L. fuliginosus nests based on both morphological and molecular data have revealed the presence of a further three species of fungi cultured by ants. However, their identification using both standard keys and DNA sequence comparisons with GenBank entries has not been successful (Schlick-Steiner et al. Citation2008). More recently, Brinker et al. (Citation2019) analyzed microbial communities associated with L. fuliginosus nests and found that the fungal communities were stable over years and distinct from surrounding soil (Brinker et al. Citation2019).
Larvae of Pella are observed in waste dumps inside those carton-nests, while adults are found in shelters during the day and in close proximity of hosts’ nests during the night. More detailed descriptions of Pella behaviour have been made by Hölldolber et al. (Citation1981). These authors frequently observed the Pella larvae feeding on dead ants. In turn, when ants encountered the larvae, they usually attacked them. The attacks resulted in a typical defense behaviour with Pella larvae raising abdominal tip towards the head of the ants. This behavior apparently protected larvae against ant attacks although several such interactions ended with the death of the Pella larvae. On the other hand, when the temperature is low, the Pella larvae are able to come into close contact to L. fuliginosus workers without being attacked. Hölldobler et al. observed even the beetle larvae licking the cuticles of live ants, including the mandibles and mouthparts. However, experiments described by authors clearly showed that the Pella larvae do not solicit regurgitation in ants and dead ants are their main food source. In the case of P. laticollis adults, observations revealed that these insects predominantly live as scavengers feeding on dead or disabled ants, but are also able to act as very effective predators on ant adults and larvae, when starved (Hölldolber et al. Citation1981; Staniec et al. Citation2009).
The staphylinid, Pella laticollis (Maerkel, 1845) (Staphylinidae, Aleocharinae) is associated only with L. fuliginosus. Thus, the association between P. laticollis and its host seems to be species-specific on the part of Pella. Moreover, previous studies have suggested that P. laticollis exhibits behavioural pre-adaptation towards a closer relationship with its host better than other Pella representatives (Hölldolber et al. Citation1981; Stoeffler et al. Citation2011; Staniec et al. Citation2018). Evidence for this statement is provided by the defensive behaviour observed in P. laticollis larvae and adults. On encountering an ant, a beetle discharges a secretion from its tergal glands, while simultaneously flexing its abdomen (Staniec et al. Citation2009). This “duck down” appeasing behaviour and presenting the abdominal tip, observed only in P. laticollis, appears to mollify the hosts’ aggressive reaction: the ants focus on the tip of the beetle’s abdomen, which allows the latter to escape (Stoeffler et al. Citation2011). These results can bring us nearer to understanding of the mechanisms that govern the close relationships between P. laticollis and its host L. fuliginosus.
Undoubtedly, one cannot gainsay the possible role of microbiota in shaping the interaction between these two species. On the other hand, bacterial communities associated with both P. laticollis larvae and adults may be similar to those associated to their host’s adults. Both developmental stages of this staphylinid feed on dead and disabled L. fuliginosus ants (and ants’ larvae, if available) and thus, they may acquire fractions of microbiota associated with their host. To test this hypothesis, we investigated the bacterial communities associated with the larvae and adults of both P. laticollis and its host L. fuliginosus. We used 16S rRNA sequencing to define whether the bacterial communities varied among two closely associated species. Lastly, we analyzed the microbiome profile of the nest-carton – the material which lines the inside of L. fuliginosus nests and may mediate the horizontal transfer of bacteria.
Materials and methods
Sample collection
Samples were collected during the spring of 2018. Permission for the field studies was granted by the Polish Ministry of the Environment (Permit No. DOP-WPN.286.214.2018.MŚ). Larvae and adults of both L. fuliginosus and P. laticollis were obtained from a single, large carton-nest found inside an old birch tree trunk (Betula sp.) near Lake Długie (Polesie National Park; N 51º27ʹ04.12” E 23º10ʹ14.17”). We also sampled the nest-carton. The number of collected samples was limited (5 samples of L. fuliginosus (2 for larvae and 3 for adults), 6 for P. laticollis (3 for larvae and 3 for adults) and 3 for nest material). All larvae and adults were starved prior to the subsequent analyses, then placed separately into Eppendorf or Falcon tubes and stored at −80°C until DNA extraction.
DNA extraction and Illumina Miseq sequencing
The protocol for the sterilization of the ants cuticles was invoked to eliminate surface microbes and exogenous DNA prior to the investigation of internal microbe diversity. Individuals were processed separately with 70% ethanol for 30 s and then rinsed for 1 min in three successive baths of DNA-free water. The DNA was then extracted from whole specimens using a Sherlock AX Purification Kit (A&A Biotechnology, Poland). The DNA from the nest material (carton) was in turn extracted using a DNeasy PowerSoil Kit (Qiagen, UK) preceded by a homogenization step with garnet beads. All the extractions were performed in accordance with the manufacturer’s protocols. Negative controls were not included in the present study, but all the procedures were performed in such a way as to prevent DNA contamination.
After extraction, the quantity and quality of the DNA were evaluated with a Nano Drop ND-1000 spectrophotometer (Nano Drop Technologies, USA). The extracted DNA was sent to the Genoplast Laboratory (Poland) for library preparation and 16S Miseq Illumina sequencing as described in (Kaczmarczyk-Ziemba et al. Citation2019). Sequences of the V3-V4 region of the 16S rRNA gene were amplified using 341 F and 785 R primers. Amplification was performed using NEBNext® Hotstart DNA polymerase with high fidelity using the following protocol: 1 cycle at 98°C for 30 s, 25 cycles at 98°C for 10 s, at 55°C for 30 s and at 72°C for 20 s, and finally 1 cycle at 72°C for 2 min.
The DNA extracted from 14 samples was sequenced separately: 5 samples of L. fuliginosus (2 of larvae and 3 of adults), 6 samples of P. laticollis (3 of larvae and 3 of adults) and 3 samples of nest material. For the subsequent analyses, the samples were designated as follows: Lf-L and Lf-A – L. fuliginosus larvae and adults, Pl-L and Pl-A – P. laticollis larvae and adults, and K – carton. The raw sequential reads were submitted to the Sequence Read Archive (SRA) database under accession number PRJNA612990.
Data analysis
The samples were analysed using the QIIME 1.9.1 pipeline (Caporaso et al. Citation2010b). Paired-end reads from MiSeq sequencing were quality trimmed and joined with PANDAseq version 2.8 (Masella et al. Citation2012) with a quality threshold of 0.9. The sequences that did not meet the quality criteria were removed from further analysis (mean quality >20). Chimeric reads detection was performed with VSEARCH, version 1.7.0, an open-source replacement of USEARCH software. The clustering of operational taxonomic units (OTUs) at 97% similarity was performed using the uclust method, version 1.2.22q (Edgar Citation2010). Sample reads were rarefied to 52 233 reads. Taxonomic assignment was performed at 97% against the SILVA v.132 database (Quast et al. Citation2013), with the taxonomy assignment tool PyNAST (Caporaso et al. Citation2010a). OTU saturation was evaluated with rarefaction curves using the Chao1 richness estimate. The BIOM table (The Biological Observation Matrix) was used as the core data for downstream analyses (McDonald et al. Citation2012). Any sequences that were classified as Mitochondria or Chloroplast, as well as the singletons, were filtered out of the dataset. The diversity indices, including Shannon and observed OTUs were estimated for each sample. Further statistical analyses were preceded by data distribution analysis using the Shapiro-Wilk test. Differences in alpha diversity indices among the sample groups were statistically assessed with the Kruskal-Wallis test. The bacterial community structure was compared using UniFrac (Lozupone & Knight Citation2005) and Emperor (Vázquez-Baeza et al. Citation2013). A two-dimensional principal coordinate analysis (PCoA) was conducted from weighted UniFrac distances obtained from the core diversity analyses.
A permutation-based multivariate analysis of variance (PERMANOVA) was performed to assess whether there were differences among the predefined groups of samples, i.e. Pella larvae, Pella adults, Lasius larvae, Lasius adults, and nest material. Similarity percentage (SIMPER) analysis was performed to calculate the average dissimilarities in the bacterial community structures between samples and to ascertain which family was responsible for the observed differences. The relative abundances of those bacterial families that were primarily responsible for the differences between samples were then used for a proximity analysis. To illustrate the most abundant bacterial families and microbiome relationships across the tested samples, a heatmap and dendrogram were generated with the Bray-Curtis dissimilarity index. A similarity profile (SIMPROF) test was used to identify well-defined groups of samples (Clarke et al. Citation2008). All the statistical analyses were performed using Primer version 7 software (Clarke & Gorley Citation2015).
Results and discussion
To date, studies using high throughput sequencing techniques for microbiota profiling have focused primarily on identifying and analysing the bacterial communities associated with different ant species (Kautz et al. Citation2013b; Vieira et al. Citation2017; Lucas et al. Citation2017; Ramalho et al. Citation2017b, Citation2017c, Citation2019; Hu et al. Citation2018; Chua et al. Citation2018; Segers et al. Citation2019). Although myrmecophiles interact closely with ants and are often found inside ants’ nests, no exhaustive analyses of the bacterial communities associated with those species have been carried out (but see (Liberti et al. Citation2015; Fischer et al. Citation2017; Ivens et al. Citation2018; Di Salvo et al. Citation2019; Szenteczki et al. Citation2019; Kaczmarczyk-Ziemba et al. Citation2020)). In the present study, we have extended the state of the art regarding the microbiota of ants and their associates by investigating the potential similarities among the bacterial communities associated with two closely interacting species, L. fuliginosus and P. laticollis (both larvae and adults). We also determined the microbiota profile for the nest-carton, an organic material present inside the nest chambers. We collected only a limited number of samples, performing the analyses on 5 samples of L. fuliginosus (2 of larvae and 3 of adults), 6 of P. laticollis (3 of larvae and 3 of adults) and 3 of nest material. Thus, presented results and statistics should be recognized rather as the first attempt to identify trend characteristics in L. fuliginosus – P. laticollis interaction and more comprehensive analyses involving additional biological replicates should be performed in the future to test the stability of the described patterns.
Following demultiplexing, quality filtering and chimera removal, 2 006 036 reads were retained for the 5 samples of L. fuliginosus (2 of larvae and 3 of adults), the 6 samples of P. laticollis (3 of larvae and 3 of adults) and the 3 samples of nest material (52 233–169 028 reads per sample). The calculated alpha diversity metrics differed significantly among the samples grouped according to the source (Kruskal-Wallis test (H): 12.13, p < 0.05 for OTU and 11.32, p < 0.05 for the Shannon indices).
Taxonomic classification yielded 20 phyla present in the bacterial communities analysed (, Table S1). All the samples contained high abundances of Proteobacteria (up to 99.84% for the Pl-L1 profile), Actinobacteria (up to 29.19% for Lf-L3) and Firmicutes (up to 23.58% for Lf-L3) (Figure S1). These three phyla have frequently been listed among the most abundant groups of bacteria present in the microbiota of various insect species (Jones et al. Citation2013; Yun et al. Citation2014), including ants (Kellner et al. Citation2015; Sapountzis et al. Citation2015; Lanan et al. Citation2016; Meirelles et al. Citation2016; Vieira et al. Citation2017; Łukasik et al. Citation2017; Ramalho et al. Citation2017a) and their associates (Liberti et al. Citation2015; Ivens et al. Citation2018; Szenteczki et al. Citation2019; Kaczmarczyk-Ziemba et al. Citation2020).
Figure 1. Bacterial phyla present in nest material and in profiles of Pella laticollis and Lasius fuliginosus individuals
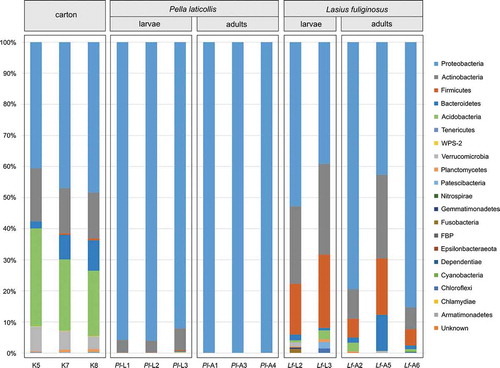
The average similarities of the profiles calculated on the basis of the Bray-Curtis index at the bacterial family level varied among the predefined groups. The bacterial communities associated with P. laticollis adults resembled each other the most (99.84% of the average similarity within the group). The profiles of the other samples showed the following average similarities at the bacterial family level: 59.71% for P. laticollis larvae, 55.90% for L. fuliginosus larvae, 47.77% for L. fuliginosus adults and 82.61% for the nest-carton. SIMPER analysis indicated that 23 families were primarily responsible for the differences between the samples. The relative abundances of these families were used to generate a heatmap for all the samples (). The bacterial communities were grouped according to their origin, this approach being supported by the results of the SIMPROF and PERMANOVA (Pseudo-F = 13.4, p = 0.001) analyses. Only sample Lf-A5 was grouped with the L. fuliginosus larvae samples. This pattern was also discernible in the bar plot at the phylum level and in the PCoA plot ( and ). The relationships among the microbiome profiles on the PCoA plot and the heatmap were congruent. The microbiota associated with the carton and L. fuliginosus ants (both larvae and adults) displayed the greatest similarity, whereas the bacterial communities associated with P. laticollis adults were the most highly differentiated from the other communities (). In turn, PERMANOVA calculated for all pairs of samples showed that only the microbiota associated with Lasius larvae and adults were not significantly different (). However, some of these significant differences may have resulted from our use of two different methods of extracting DNA from insects and the nest-carton (e.g. (Ketchum et al. Citation2018; Mallott et al. Citation2019)).
Table I. Results of PERMANOVA analysis after 999 permutations. Values above diagonal indicate p values. Groups were determined according to the sample origin
Figure 2. The heatmap showing relationships among tested profiles of bacterial communities and the Principal Coordinate Analysis (PCoA) plot. (a) – The heatmap showing bacterial families distributed across tested samples. Only those families which were primarily responsible for the observed differences among samples were considered. Both dendrograms were estimated with the Bray-Curtis dissimilarity index; (b) – PCoA of bacterial communities associated with tested specimens based on weighted UniFrac distances
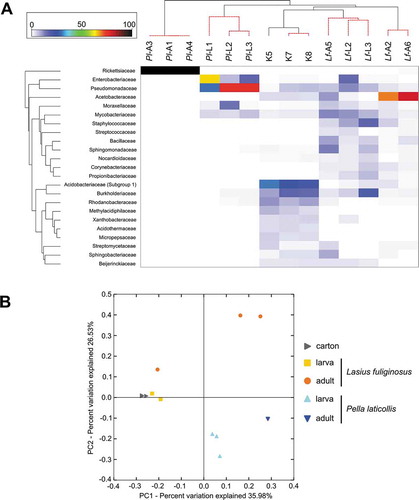
More detailed examination of the L. fuliginosus microbiota showed that Acetobacteraceae (Proteobacteria) was the most abundant in the bacterial communities associated with adults. Previous studies had found that members of this family were characteristic of the microbiota of ants consuming sugar-rich substances like honeydew, which is the principal dietary component of L. fuliginosus adults (Hu et al., Citation2017; Russell et al. Citation2009; Brown & Wernegreen Citation2016; Ivens et al. Citation2018). At the genus level, all the ant microbiota profiles were characterized by a relatively high percentage of certain bacterial genera: Pseudomonas and Acinetobacter (Proteobacteria), Mycobacterium, Cutibacterium and Nocardioides (Actinobacteria), Bacillus, Staphylococcus and Streptococcus (Firmicutes). Moreover, a relatively high abundance of Turicella (Actinobacteria) was identified in the microbiota of adults (Table S1). These bacteria may play key roles in different digestive and defensive processes. For example, Pseudomonas species engage in cellulolytic, lipolytic, esterase, amylolytic and xylanolytic activities (Briones-Roblero et al. Citation2017b), which appear to be necessary for honeydew digestion and wood maceration during nest building. In turn, Mycobacterium may impair the development of fungal infections in insects (Kabaluk et al. Citation2017), an aspect especially crucial in the case of L. fuliginosus, as living in a social group may increase the risk of an epidemic outbreak. Entomopathogenic fungi, as well as their toxins, are natural threats to social insect colonies. To combat them, social insects have evolved a series of unique disease defences at the colony level, which consist of behavioural and physiological adaptations, i.e. social immunity (Liu et al. Citation2019). We cannot rule out the possibility that the Mycobacterium found in bacterial communities associated with ants is involved in these defensive mechanisms. Bacteria-derived antifungal compounds have recently been described from the head and body of L. fuliginosus (Liu et al. Citation2016a, Citation2016b; Ye et al. Citation2017; Shen et al. Citation2017; Brinker et al. Citation2019). The higher abundances of the genera listed above are in accordance with the profiling of ant microbiota performed to date. Pseudomonas and Mycobacterium, as well as other genera listed above, have been found in communities associated with ant species belonging to the genera Acromyrmex (Zhukova et al. Citation2017), Allomerus and Tetraponera (Seipke et al. Citation2013), Camponotus and Colobopsis (Ramalho et al. Citation2017b), Cephalotes (Kautz et al. Citation2013a; Lanan et al. Citation2016), Formica (Kaczmarczyk-Ziemba et al. Citation2020), Oecophylla (Chua et al. Citation2018), Solenopsis (Li et al. Citation2005; Ishak et al. Citation2011), Brachymyrmex and other Lasius species (Ivens et al. Citation2018).
The bacterial communities associated with P. laticollis larvae and adults exhibited lower alpha diversity indices than those calculated for the ant profiles. At the phylum level, all these communities were dominated by Proteobacteria, but Actinobacteria were also present in the larval profiles. These differences were reflected in the profile clustering (congruent to the host’s developmental stage) and the significant PERMANOVA value. Analyses of lower taxonomic ranks indicated relatively high abundances of the families Pseudomonadaceae in P. laticollis larvae and Rickettsiaceae in adults; this was directly related to higher percentages of two genera, i.e. Pseudomonas and Rickettsia, respectively. As mentioned above, members of Pseudomonas participate in many different enzymatic activities. Moreover, members of Pseudomonas associated with females from the genus Paederus (staphylinids like Pella laticollis) can synthesize the polyketide pederin, the most complex non-proteinaceous insect defensive secretion known (Kellner & Dettner Citation1996; Kador et al. Citation2011; Krinsky Citation2019). In rove beetles, pederin acts as a substance for chemical defence against potential predators (Kellner & Dettner Citation1996). Furthermore, both Pseudomonas and Serratia found in the larval profiles possess antimicrobial activity and regulate mechanisms within bacterial populations (quorum sensing) (Venturi Citation2006; Liu et al. Citation2007; Charyulu et al. Citation2009). Briones-Roblero et al. (Citation2017a) hypothesized that those bacteria, along with an insect’s immune system, could regulate the gut bacterial communities associated with the bark beetle Dendroctonus rhizophagus (Briones-Roblero et al. Citation2017a). Apart from host-species, Serratia has also been found in the microbiota of other curculionid beetles, as well as scarabaeid and staphylinid species (Kolasa et al. Citation2019). Here, we found a heightened abundance of S. proteamaculans (3.34% for Pl-L2 and 5.90% for Pl-L3), which is responsible for amber disease in the New Zealand beetles Costelytra zealandica, Pyronota festiva and P. setosa (Sanchez-Contreras & Vlisidou Citation2008; Hurst et al. Citation2018). Its pathogenicity is directly correlated with the presence of the pADAP plasmid, so not all strains of S. proteamaculans are pathogenic (Koppenhöfer et al. Citation2012). Moreover, the pADAP plasmid has only been found in New Zealand bacterial isolates (Jurat-Fuentes & Jackson Citation2012). Members of our team have been taking part in long-term studies of the biology of myrmecophiles, P. laticollis in particular (Staniec et al. Citation2009), and their investigations have not revealed any potential symptoms of amber disease in P. laticollis larvae. Although S. proteamaculans does not appear to affect the biology of P. laticollis, endosymbiotic Rickettsia (over 99.00% in adult profiles) can induce diverse effects on the host, from influencing host fitness to manipulating reproduction. Rickettsia strains have been reported to affect host fitness negatively, for example, by reducing in body weight, fecundity and longevity, and by increasing susceptibility to insecticides (Chen et al. Citation2000; Sakurai et al. Citation2005; Simon et al. Citation2007; Kontsedalov et al. Citation2008). There is also evidence that this endosymbiont has positive effects on host fitness, for example, via a possible role in oogenesis and the promotion of more offspring (Zchori-Fein et al. Citation2006; Himler et al. Citation2011). Finally, Rickettsia may manipulate the host’s reproductive processes in such a way as to bias the host sex ratio towards females, thus favoring the spread of this transovarially inherited endosymbiont in the infected population (Perlman et al. Citation2006; Hurst & Frost Citation2015). Infections with Rickettsia have been identified in various groups of insects (Weinert et al. Citation2009), including ants (Hu et al., Citation2017; Lester et al. Citation2017), and also myrmecophilous species like the caterpillars of the Large Blue butterfly Maculinea alcon butterfly (Di Salvo et al. Citation2019) and adults of the beetles Thiasophila angulata, Monotoma angusticollis, Dendrophilus pygmaeus, Leptacinus formicetorum, Myrmechixenus subterraneus and Ptenidium formicetorum (Kaczmarczyk-Ziemba et al. Citation2020). Nevertheless, the potential effects of such an infection with Rickettsia have not been detected in those hosts. Similarly, long-term studies of the biology and morphology of P. laticollis have not revealed any female-biased sex ratio in its natural populations (authors’ observations). It is highly advisable, however, that the prevalence of Rickettsia in P. laticollis populations be analysed in further studies. Here, the sample size was limited and only three profiles of adult individuals collected from a single nest were determined. Thus, we cannot state definitely that all P. laticollis adults are heavily infected with Rickettsia. Neither can we rule out the possibility that immature individuals, as well as their host L. fuliginosus, are also infected with these endosymbiotic bacteria. In the present study, Rickettsia was found in microbiota of a single P. laticollis larva (0.05% for Pl-L3) and a single L. fuliginosus adult (0.55% for Lf-A6).
In addition to the microbiota profiles determined for L. fuliginosus and P. laticollis, we analysed the bacterial communities associated with the nest-carton. Ant colonies build extensive nests to live in, produce waste, and interact in ways that produce distinct microbial communities (Hölldobler & Wilson Citation1990; Hölldobler & Wilson Citation2009). Interest in analyses of the microbiota associated with the nest material has been increasing in recent years, and one such analysis has been conducted for L. fuliginosus (Brinker et al. Citation2019). In that study, however, the composition of the bacterial community was characterized by automated ribosomal intergenic spacer analysis (ARISA), an approach enabling the rapid generation of whole-community “fingerprints” of microbiota assemblages (Fisher & Triplett Citation1999; Ranjard et al. Citation2001). Although ARISA-based approaches are suitable for investigating microbiota patterns, they prevent direct comparison with available data because comparable taxonomic information is missing (Brinker et al. Citation2019). In the context of those results, therefore, we cannot evaluate the structure of the nest-carton bacterial community identified here. Nevertheless, reports based on 16S sequencing have been published for nests of the Neotropical ants Azteca trigona and A. alfari, and the Palearctic species Formica exsecta (Lucas et al. Citation2017, Citation2019; Lindström et al. Citation2019). Three phyla (Proteobacteria, Actinobacteria and Acidobacteria) were the most abundant in the microbiota profiles determined in those studies; the same pattern emerged in the present study. Nine genera within these phyla (Acidipila, Granulicella, Candidatus Koribacter, Mycobacterium, Acidothermus, Roseiarcus, Afipia, Burkholderia-Caballeronia-Paraburkholderia and Rhodanobacter) were more abundant than in the other samples tested and accounted jointly for 32.21–39.93% of those communities. These bacteria are frequently listed as members of microbiota present in soil (Rawat et al. Citation2012; Ritpitakphong et al. Citation2016; Siles & Margesin Citation2017; Haas et al. Citation2018; Karimi et al. Citation2018; Jiao et al. Citation2019; Lee et al. Citation2019; Domeignoz-Horta et al. Citation2019; Bartelme et al. Citation2020) and dead wood (Tláskal et al. Citation2017; Bani et al. Citation2018). Inside L. fuliginosus nests, however, soil particles as well as shredded wood are bound into a cardboard-like building material (carton) (Maschwitz & Hölldobler Citation1970; Brinker et al. Citation2019). Thus, the presence of bacteria characteristic of soil and decomposing dead wood in microbiota associated with the nest-carton is perfectly possible. In addition, some genera identified in carton-associated communities may give an indication of the acidity of the soil surrounding the nest. The genera Acidipila and Roseiarcus, found in these profiles, were previously more frequently identified in bacterial communities associated with acidic soil taken from a coniferous forest (Siles & Margesin Citation2017). The L. fuliginosus nest examined in the present study was located inside a birch trunk growing between oak-hornbeam and alder carr forests, in close proximity to a dystrophic lake with a littoral zone of floating sphagnum mats. Although the pH of the soil surrounding the nest in question was not measured, the soil incorporated into the nest is likely to have been of higher acidity.
Wolbachia was also found in the nest-carton profiles, but its relative abundance was low, not exceeding 1% (Table S1). Like Rickettsia, this well-known endosymbiotic bacteria, can induce mechanisms manipulating its host’s reproductive processes, causing cytoplasmic incompatibility, parthenogenesis, male killing, or male feminization (Stouthamer et al. Citation1999; Charlat et al. Citation2003; Correa & Ballard Citation2016; Pietri et al. Citation2016). Wolbachia potentially infects more than 65% of insect species (Hilgenboecker et al. Citation2008; Lewis & Lizé Citation2015), including a wide range of ant genera, such as Atta and Acromyrmex (Van Borm et al. Citation2003; Frost et al. Citation2010, Citation2014), Camponotus (Ramalho et al., Citation2018; Ramalho et al. Citation2017), Cephalotes (Kelly et al. Citation2019; Reeves et al. Citation2020), Colobopsis (Ramalho et al. Citation2017b), Formica (Keller et al. Citation2001; Viljakainen et al. Citation2008; Kaczmarczyk-Ziemba et al. Citation2020), Polyrhachis (Ramalho et al. Citation2017a), Solenopsis (Ishak et al. Citation2011; Martins et al. Citation2012; de Souza et al. Citation2014), and others (Wenseleers & Ito Citation1998; Russell et al. Citation2012; Pontieri et al. Citation2017). This endosymbiont has also been identified with a high relative abundance in bacterial communities associated with myrmecophilous insects, such as caterpillars or adults of Maculinea butterfly (Patricelli et al. Citation2013; Di Salvo et al. Citation2019) and adults of the beetles Dendrophilus pygmaeus, Leptacinus formicetorum, Monotoma angusticollis, Myrmechixenus subterraneus, Ptenidium formicetorum and Thiasophila angulata (Kaczmarczyk-Ziemba et al. Citation2020). Here, we confirmed the presence of Wolbachia only in the microbiota from the nest material, which consists of dead wood particles chewed by ants. Thus, in view of the limited number of samples analysed, we cannot unreservedly rule out the possible horizontal transmission of Wolbachia and the potential infection of L. fuliginosus ants. This phenomenon has recently been described in insects sharing the same habitats and/or host plants. Li et al. (Citation2017) described plant-mediated horizontal transmission of Wolbachia between infected and uninfected Bamisia tabaci whiteflies. When uninfected individuals fed on the infected cotton leaves (after infected whiteflies fed on leaves, Wolbachia was visualized in the phloem vessels), the majority of them became infected with the symbiont. Moreover, Wolbachia persisted in cotton leaves for at least 50 days. The role of host plants in endosymbiont spread has been also described for two species of Crioceris leaf beetles (Kolasa et al. Citation2017) and revised by Chrostek et al. (Citation2017). Moreover, there are known groups of insects that inhabit the same environments and share the same or very similar Wolbachia strains (Kawasaki et al. Citation2016; Kajtoch & Kotásková Citation2018). For example, bacteria horizontal transfer was observed when infected and uninfected larvae of parasitoid Trichogramma wasps shared the same host egg (Huigens et al. Citation2004). In turn, Stahlhut et al. (Citation2010) found that ecological associations can facilitate horizontal of Wolbachia within mycophagous Diptera species. On the other hand, not all Wolbachia strains have the same genetic potential for horizontal transmission (Tolley et al. Citation2019). Our analyses were based on 16S rDNA sequencing, which cannot distinguish closely-related Wolbachia strains (Andersen et al. Citation2012). For further strain typing, the diversity of the wsp gene, as well as five genes described in the MLST protocol, will need to be analysed (Baldo et al. Citation2005, Citation2006; Bordenstein et al. Citation2009).
Conclusion
This paper presents the results of a study of the bacterial communities associated with the larvae and adults of both L. fuliginosus and the myrmecophilous beetle P. laticollis, as well as the nest-carton used by L. fuliginosus to build chambers inside its nests. The close relationships between these insects, as well as the shared habitat may have been seen as potential factors shaping the similarities in their microbiota. However, our findings show that the bacterial communities associated with both the larvae and adults of both L. fuliginosus and P. laticollis were clustered according to the host’s identity and its developmental stage. The profiles determined for the nest-carton comprised a separate group. The differences between all pairs of groups but one were significant. Only the bacterial communities associated with L. fuliginosus larvae and adults were not significantly different. In these profiles, we identified the presence of two well-known endosymbiotic bacteria – Rickettsia and Wolbachia although the latter was found only in the nest-carton profiles. Our study is in line with a new research trend that is focusing on the microbiota associated not only with ants but also with myrmecophiles and the nests inhabited by those species. There is still a great deal to discover about these systems. Some important questions that need to be addressed are: What factors shape the myrmecophiles’ microbiota profiles? What functional benefits are derived from the associated bacteria? Do close interactions among myrmecophiles and their hosts permit horizontal transmission of microbiota? Can microorganisms associated with nests protect the ant colony and its inhabitants against pathogens? The broad nature of these questions means that multidisciplinary collaborations will be required to address them in the future.
Acknowledgements
The raw sequential reads generated during the current study are available in the Sequence Read Archive (SRA) repository under accession number PRJNA612990 (https://www.ncbi.nlm.nih.gov/sra/). The authors declare that they have no competing interests.
We are very grateful to the staff of the OMICRON Centre for Medical Genomics for their advices.
Disclosure statement
No potential conflict of interest was reported by the authors.
Additional information
Funding
References
- Akino T. 2002. Chemical camouflage by myrmecophilous beetles Zyras comes (Coleoptera: Staphylinidae) and Diaritiger fossulatus (Coleoptera: Pselaphidae) to be integrated into the nest of Lasius fuliginosus (Hymenoptera: Formicidae). Chemoecology 12:83–89. DOI: 10.1007/s00049-002-8330-4.
- Andersen SB, Boye M, Nash DR, Boomsma JJ. 2012. Dynamic Wolbachia prevalence in Acromyrmex leaf-cutting ants: Potential for a nutritional symbiosis. Journal of Evolutionary Biology 25:1340–1350. DOI: 10.1111/j.1420-9101.2012.02521.x.
- Anderson KE, Russell JA, Moreau CS, Kautz S, Sullam KE, Hu Y, et al. 2012. Highly similar microbial communities are shared among related and trophically similar ant species. Molecular Ecology 21:2282–2296.
- Baldo L, Dunning Hotopp JC, Jolley KA, Bordenstein SR, Biber SA, Choudhury RR, et al. 2006. Multilocus sequence typing system for the endosymbiont Wolbachia pipientis. Applied and Environmental Microbiology 72:7098–7110. DOI: 10.1128/AEM.00731-06.
- Baldo L, Lo N, Werren JH. 2005. Mosaic nature of the Wolbachia surface protein. Journal of Bacteriology 187:5406–5418. DOI: 10.1128/JB.187.15.5406-5418.2005.
- Bani A, Pioli S, Ventura M, Panzacchi P, Borruso L, Tognetti R, et al. 2018. The role of microbial community in the decomposition of leaf litter and deadwood. Applied Soil Ecology 126:75–84. DOI: 10.1016/j.apsoil.2018.02.017.
- Bartelme RP, Custer JM, Dupont CL, Espinoza JL, Torralba M, Khalili B, et al. 2020. Influence of substrate concentration on the culturability of heterotrophic soil microbes isolated by high-throughput dilution-to-extinction cultivation. mSphere 5:1–15. DOI: 10.1128/mSphere.00024-20.
- Bolton B. An online catalog of the ants of the wolrd. Available: http://antcat.org.
- Bordenstein SR, Paraskevopoulos C, Dunning Hotopp JC, Sapountzis P, Lo N, Bandi C, et al. 2009. Parasitism and mutualism in Wolbachia: What the phylogenomic trees can and cannot say. Molecular Biology and Evolution 26:231–241. DOI: 10.1093/molbev/msn243.
- Brinker P, Weig A, Rambold G, Feldhaar H, Tragust S. 2019. Microbial community composition of nest-carton and adjoining soil of the ant Lasius fuliginosus and the role of host secretions in structuring microbial communities. Fungal Ecology 38:44–53. DOI: 10.1016/j.funeco.2018.08.007.
- Briones-Roblero CI, Hernández-García JA, Gonzalez-Escobedo R, Soto-Robles LV, Rivera-Orduña FN, Zúñiga G. 2017a. Structure and dynamics of the gut bacterial microbiota of the bark beetle, Dendroctonus rhizophagus (Curculionidae: Scolytinae) across their life stages. PLoS One 12:e0175470.
- Briones-Roblero CI, Rodríguez-Díaz R, Santiago-Cruz JA, Zúñiga G, Rivera-Orduña FN. 2017b. Degradation capacities of bacteria and yeasts isolated from the gut of Dendroctonus rhizophagus (Curculionidae: Scolytinae). Folia Microbiologica 62:1–9. DOI: 10.1007/s12223-016-0469-4.
- Brown BP, Wernegreen JJ. 2016. Deep divergence and rapid evolutionary rates in gut-associated Acetobacteraceae of ants. BMC Microbiology 16:1–17. DOI: 10.1186/s12866-016-0721-8.
- Cafaro MJ, Currie CR. 2005. Phylogenetic analysis of mutualistic filamentous bacteria associated with fungus-growing ants. Canadian Journal of Microbiology 51:441–446. DOI: 10.1139/w05-023.
- Caporaso JG, Bittinger K, Bushman F, DeSantis T, Andersen G, Knight R. 2010a. PyNAST: A flexible tool for aligning sequences to a template alignment. Bioinformatics 26:266–267. DOI: 10.1093/bioinformatics/btp636.
- Caporaso JG, Kuczynski J, Stombaugh J, Bittinger K, Bushman FD, Costello EK, et al. 2010b. QIIME allows analysis of high-throughput community sequencing data. Nature Methods 7:335–336. DOI: 10.1038/nmeth.f.303.
- Casacci LP, Bonelli S, Balletto E, Barbero F. 2019. Multimodal signaling in myrmecophilous butterflies. Frontiers in Ecology and Evolution 7:454. DOI: 10.3389/fevo.2019.00454.
- Charlat S, Hurst GDD, Merçot H. 2003. Evolutionary consequences of Wolbachia infections. Trends in Genetics 19:217–223. DOI: 10.1016/S0168-9525(03)00024-6.
- Charyulu EM, Sekaran G, Rajakumar GS, Gnanamani A. 2009. Antimicrobial activity of secondary metabolite from marine isolate, Pseudomonas sp. against Gram positive and negative bacteria including MRSA. Indian Journal of Experimental Biology 47:964–968.
- Chen D-Q, Montllor CB, Purcell AH. 2000. Fitness effects of two facultative endosymbiotic bacteria on the pea aphid, Acyrthosiphon pisum, and the blue alfalfa aphid, A. kondoi. Entomologia Experimentalis Et Applicata 95:315–323. DOI: 10.1046/j.1570-7458.2000.00670.x.
- Chomicki G, Renner SS. 2017. The interactions of ants with their biotic environment. Proceedings of the Royal Society B: Biological Sciences 284:20170013. DOI: 10.1098/rspb.2017.0013.
- Chrostek E, Pelz-Stelinski K, Hurst GDD, Hughes GL. 2017. Horizontal transmission of intracellular insect symbionts via plants. Frontiers in Microbiology 8:2237. DOI: 10.3389/fmicb.2017.02237.
- Chua KO, Song SL, Yong HS, See-Too WS, Yin WF, Chan KG. 2018. Microbial community composition reveals spatial variation and distinctive core microbiome of the weaver ant Oecophylla smaragdina in Malaysia. Scientific Reports 8:1–11. DOI: 10.1038/s41598-018-29159-2.
- Clarke KR, Gorley R. 2015. PRIMER version 7: User manual/tutorial. Plymouth: Prim Ltd.
- Clarke KR, Somerfield PJ, Gorley RN. 2008. Testing of null hypotheses in exploratory community analyses: Similarity profiles and biota-environment linkage. Journal of Experimental Marine Biology and Ecology 366:56–69. DOI: 10.1016/j.jembe.2008.07.009.
- Correa CC, Ballard JWO. 2016. Wolbachia associations with insects: Winning or losing against a master manipulator. Frontiers in Ecology and Evolution 3:1–18.
- Currie CR, Scott JA, Summerbell RC, Malloch D. 1999. Fungus-growing ants use antibiotic-producing bacteria to control garden parasites. Nature 398:701–704. DOI: 10.1038/19519.
- Currie CR, Wong B, Stuart AE, Schultz TR, Rehner SA, Mueller UG, et al. 2003. Ancient tripartite coevolution in the attine ant-microbe symbiosis. Science 299:386–388. DOI: 10.1126/science.1078155.
- de Souza RF, Martins C, Pereira RM, Bueno OC. 2014. Analysis of the Hypervariable Regions (HVRs) of the wsp Gene of Wolbachia from Solenopsis invicta Ants in Southeastern Brazil. Advances in Entomology 2:135–143. DOI: 10.4236/ae.2014.23021.
- Degnan PH, Lazarus AB, Wernegreen JJ. 2005. Genome sequence of Blochmannia pennsylvanicus indicates parallel evolutionary trends among bacterial mutualists of insects. Genome Research 15:1023–1033. DOI: 10.1101/gr.3771305.
- Di Salvo M, Calcagnile M, Talà A, Tredici SM, Maffei ME, Schönrogge K, et al. 2019. The microbiome of the Maculinea-Myrmica host-parasite interaction. Scientific Reports 9:1–10. DOI: 10.1038/s41598-019-44514-7.
- Domeignoz-Horta LA, DeAngelis KM, Pold G. 2019. Draft genome sequence of acidobacteria group 1 Acidipila sp. Strain EB88, Isolated from Forest Soil. Microbiology Resource Announcements 8:1–2. DOI: 10.1128/MRA.01464-18.
- Douglas AE. 1998. Nutritional interactions in insect-microbial symbioses: Aphids and their symbiotic bacteria Buchnera. Annual Review of Entomology 43:17–37. DOI: 10.1146/annurev.ento.43.1.17.
- Douglas AE. 2009. The microbial dimension in insect nutritional ecology. Functional Ecology 23:38–47. DOI: 10.1111/j.1365-2435.2008.01442.x.
- Douglas AE. 2015. Multiorganismal insects: Diversity and function of resident microorganisms. Annual Review of Entomology 60:17–34. DOI: 10.1146/annurev-ento-010814-020822.
- Edgar RC. 2010. Search and clustering orders of magnitude faster than BLAST. Bioinformatics 26:2460–2461. DOI: 10.1093/bioinformatics/btq461.
- Elliott JSB. 1915. Fungi in the nests of ants: Plate II. Transactions of the British Mycological Society 5:138–142. DOI: 10.1016/S0007-1536(14)80012-0.
- Engel P, Moran NA. 2013. The gut microbiota of insects – Diversity in structure and function. FEMS Microbiology Reviews 37:699–735. DOI: 10.1111/1574-6976.12025.
- Feldhaar H, Straka J, Krischke M, Berthold K, Stoll S, Mueller MJ, et al. 2007. Nutritional upgrading for omnivorous carpenter ants by the endosymbiont Blochmannia. BMC Biology 5:1–11. DOI: 10.1186/1741-7007-5-48.
- Fischer CY, Detrain C, Thonart P, Haubruge E, Francis F, Verheggen FJ, et al. 2017. Bacteria may contribute to distant species recognition in ant-aphid mutualistic relationships. Insect Science 24:278–284. DOI: 10.1111/1744-7917.12302.
- Fisher MM, Triplett EW. 1999. Automated approach for ribosomal intergenic spacer analysis of microbial diversity and its application to freshwater bacterial communities. Applied and Environmental Microbiology 65:4630–4636. DOI: 10.1128/AEM.65.10.4630-4636.1999.
- Fresenius G. 1852. Beiträge zur Mykologie. 1–2:1–80.
- Frost CL, FernÁndez-MarÍn H, Smith JE, Hughes WOH. 2010. Multiple gains and losses of Wolbachia symbionts across a tribe of fungus-growing ants. Molecular Ecology 19:4077–4085. DOI: 10.1111/j.1365-294X.2010.04764.x.
- Frost CL, Pollock SW, Smith JE, Hughes WOH. 2014. Wolbachia in the flesh: Symbiont intensities in germ-line and somatic tissues challenge the conventional view of Wolbachia transmission routes. PLoS One 9:e95122. DOI: 10.1371/journal.pone.0095122.
- Gil R, Silva FJ, Zientz E, Delmotte F, Gonzalez-Candelas F, Latorre A, et al. 2003. The genome sequence of Blochmannia floridanus: Comparative analysis of reduced genomes. Proceedings of the National Academy of Sciences 100:9388–9393. DOI: 10.1073/pnas.1533499100.
- Haas JC, Street NR, Sjödin A, Lee NM, Högberg MN, Näsholm T, et al. 2018. Microbial community response to growing season and plant nutrient optimisation in a boreal Norway spruce forest. Soil Biology and Biochemistry 125:197–209. DOI: 10.1016/j.soilbio.2018.07.005.
- Heil M, McKey D. 2003. Protective ant-plant interactions as model systems in ecological and evolutionary research. Annual Review of Ecology, Evolution, and Systematics 34:425–453. DOI: 10.1146/annurev.ecolsys.34.011802.132410.
- Hilgenboecker K, Hammerstein P, Schlattmann P, Telschow A, Werren JH. 2008. How many species are infected with Wolbachia? A statistical analysis of current data. FEMS Microbiology Letters 281:215–220.
- Himler AG, Adachi-Hagimori T, Bergen JE, Kozuch A, Kelly SE, Tabashnik BE, et al. 2011. Rapid spread of a bacterial symbiont in an invasive whitefly is driven by fitness benefits and female bias. Science (80-) 332:254–256. DOI: 10.1126/science.1199410.
- Hölldobler B, Wilson EO. 1990. The ants. Springer, Berlin. pp. 1–732.
- Hölldobler B, Wilson EO. 2009. The superorganism: The beauty, elegance, and strangeness of insect societies. W. W. Norton & Company, New York. pp. 1–522.
- Hölldolber B, Möglich M, Maschwitz U. 1981. Myrmecophilic relationship of Pella (Coleoptera: Staphylinidae) to Lasius fuliginosus (Hymenoptera: Formicidae). Psyche: A Journal of Entomology 88:347–374. DOI: 10.1155/1981/75317.
- Hongoh Y, Deevong P, Inoue T, Moriya S, Trakulnaleamsai S, Ohkuma M, et al. 2005. Intra-and interspecific comparisons of bacterial diversity community structure support co-evolution of gut microbiota and termite host. Applied and Environmental Microbiology 71:6590–6599.
- Huigens ME, de Almeida RP, Boons PAH, Luck RF, Stouthamer R. 2004. Natural interspecific and intraspecific horizontal transfer of parthenogenesis-inducing Wolbachia in Trichogramma wasps. Proceedings of the Royal Society B: Biological Sciences 271:509–515. DOI: 10.1098/rspb.2003.2640.
- Hu Y, Holway DA, Łukasik P, Chau L, Kay AD, LeBrun EG, et al. 2017. By their own devices: Invasive Argentine ants have shifted diet without clear aid from symbiotic microbes. Molecular Ecology 26:1608–1630. DOI: 10.1111/mec.13991.
- Hu Y, Sanders JG, Łukasik P, D’Amelio CL, Millar JS, Vann DR, et al. 2018. Herbivorous turtle ants obtain essential nutrients from a conserved nitrogen-recycling gut microbiome. Nature Communications 9:964. DOI: 10.1038/s41467-018-03357-y.
- Hurst GDD, Frost CL. 2015. Reproductive parasitism: Maternally inherited symbionts in a biparental world. Cold Spring Harbor Perspectives in Biology 7:1–21. DOI: 10.1101/cshperspect.a017699.
- Hurst MRH, Beattle A, Jones SA, Laugraud A, van Koten C, Harper L. 2018. Serratia proteamaculans Strain AGR96X Encodes an Antifeeding Prophage (Tailocin) with Activity against Grass Grub (Costelytra giveni) and Manuka Beetle (Pyronota Species) Larvae. Applied and Environmental Microbiology 84:e02739–17. DOI: 10.1128/AEM.02739-17.
- Ishak HD, Plowes R, Sen R, Kellner K, Meyer E, Estrada DA, et al. 2011. Bacterial diversity in Solenopsis invicta and Solenopsis geminata Ant Colonies Characterized by 16S amplicon 454 Pyrosequencing. Microbial Ecology 61:821–831. DOI: 10.1007/s00248-010-9793-4.
- Ivens ABF, Gadau A, Kiers ET, Kronauer DJC. 2018. Can social partnerships influence the microbiome? Insights from ant farmers and their trophobiont mutualists. Molecular Ecology 27:1898–1914. DOI: 10.1111/mec.14506.
- Jiao S, Xu Y, Zhang J, Hao X, Lu Y. 2019. Core microbiota in agricultural soils and their potential associations with nutrient cycling. mSystems 4:1–16. DOI: 10.1128/mSystems.00313-18.
- Jing TZ, Qi FH, Wang ZY. 2020. Most dominant roles of insect gut bacteria: Digestion, detoxification, or essential nutrient provision? Microbiome 8:1–20. DOI: 10.1186/s40168-020-00823-y.
- Jones JC, Fruciano C, Hildebrand F, Al Toufalilia H, Balfour NJ, Bork P, et al. 2018. Gut microbiota composition is associated with environmental landscape in honey bees. Ecology and Evolution 8:441–451. DOI: 10.1002/ece3.3597.
- Jones RT, Sanchez LG, Fierer N. 2013. A cross-taxon analysis of insect-associated bacterial diversity. PLoS One 8:e61218. DOI: 10.1371/journal.pone.0061218.
- Jurat-Fuentes JL, Jackson TA. 2012. Bacterial entomopathogens. In: Vega FE, Kaya HK (Eds.) Insect pathology. Academic Press, San Diego. pp. 265–349.
- Kabaluk T, Li-Leger E, Nam S. 2017. Metarhizium brunneum – An enzootic wireworm disease and evidence for its suppression by bacterial symbionts. Journal of Invertebrate Pathology 150:82–87. DOI: 10.1016/j.jip.2017.09.012.
- Kaczmarczyk-Ziemba A, Wagner GK, Grzywnowicz K, Kucharczyk M, Zielińska S. 2019. The microbiome profiling of fungivorous black tinder fungus beetle Bolitophagus reticulatus reveals the insight into bacterial communities associated with larvae and adults. PeerJ 7:e6852. DOI: 10.7717/peerj.6852.
- Kaczmarczyk-Ziemba A, Zagaja M, Wagner GK, Pietrykowska-Tudruj E, Staniec B. 2020. First insight into microbiome profiles of myrmecophilous beetles and their host, red wood ant Formica polyctena (Hymenoptera: Formicidae)— A case study. Insects 11:134. DOI: 10.3390/insects11020134.
- Kador M, Horn MA, Dettner K. 2011. Novel oligonucleotide probes for in situ detection of pederin-producing endosymbionts of Paederus riparius rove beetles (Coleoptera: Staphylinidae). FEMS Microbiology Letters 319:73–81. DOI: 10.1111/j.1574-6968.2011.02270.x.
- Kajtoch Ł, Kotásková N. 2018. Current state of knowledge on Wolbachia infection among Coleoptera: A systematic review. PeerJ 6:e4471. DOI: 10.7717/peerj.4471.
- Karimi B, Terrat S, Dequiedt S, Saby NPA, Horrigue W, Lelièvre M, et al. 2018. Biogeography of soil bacteria and archaea across France. Science Advances 4:eaat1808. DOI: 10.1126/sciadv.aat1808.
- Kautz S, Rubin BER, Moreau CS. 2013a. Bacterial infections across the ants: Frequency and prevalence of Wolbachia, Spiroplasma, and Asaia. Psyche: A Journal of Entomology 2013:1–11. DOI: 10.1155/2013/936341.
- Kautz S, Rubin BER, Russell JA, Moreaua CS. 2013b. Surveying the microbiome of ants: Comparing 454 pyrosequencing with traditional methods to uncover bacterial diversity. Applied and Environmental Microbiology 79:525–534.
- Kawasaki Y, Schuler H, Stauffer C, Lakatos F, Kajimura H. 2016. Wolbachia endosymbionts in haplodiploid and diploid scolytine beetles (Coleoptera: Curculionidae: Scolytinae). Environmental Microbiology Reports 8:680–688. DOI: 10.1111/1758-2229.12425.
- Keller L, Liautard C, Reuter M, Brown WD, Sundström L, Chapuisat M. 2001. Sex ratio and Wolbachia infection in the ant Formica exsecta. Heredity 87:227–233. DOI: 10.1046/j.1365-2540.2001.00918.x.
- Kellner K, Ishak HD, Linksvayer TA, Mueller UG. 2015. Bacterial community composition and diversity in an ancestral ant fungus symbiosis. FEMS Microbiology Ecology 91:1–10. DOI: 10.1093/femsec/fiv073.
- Kellner RLL, Dettner K. 1996. Differential efficacy of toxic pederin in deterring potential arthropod predators of Paederus (Coleoptera: Staphylinidae) offspring. Oecologia 107:293–300. DOI: 10.1007/BF00328445.
- Kelly M, Price SL, de Oliveira Ramalho M, Moreau CS. 2019. Diversity of Wolbachia associated with the giant turtle ant, Cephalotes atratus. Current Microbiology 76:1330–1337. DOI: 10.1007/s00284-019-01722-8.
- Kešnerová L, Emery O, Troilo M, Liberti J, Erkosar B, Engel P. 2020. Gut microbiota structure differs between honeybees in winter and summer. The ISME Journal 14:801–814. DOI: 10.1038/s41396-019-0568-8.
- Ketchum RN, Smith EG, Vaughan GO, Phippen BL, McParland D, Al-Mansoori N, et al. 2018. DNA extraction method plays a significant role when defining bacterial community composition in the marine invertebrate Echinometra mathaei. Frontiers in Marine Science 5:255. DOI: 10.3389/fmars.2018.00255.
- Koch H, Schmid-Hempel P. 2011. Socially transmitted gut microbiota protect bumble bees against an intestinal parasite. Proceedings of the National Academy of Sciences 108:19288–19292. DOI: 10.1073/pnas.1110474108.
- Kolasa M, Montagna M, Mereghetti V, Kubisz D, Mazur MA, Kajtoch Ł. 2017. Preliminary evidence of the horizontal transmission of Wolbachia between Crioceris leaf beetles (Coleoptera: Chrysomelidae) and their Asparagus host plants. European Journal of Entomology 114:446–454.
- Kolasa M, Ścibior R, Mazur MA, Kubisz D, Dudek K, Kajtoch Ł. 2019. How hosts taxonomy, trophy, and endosymbionts shape microbiome diversity in beetles. Microbial Ecology 78:995–1013. DOI: 10.1007/s00248-019-01358-y.
- Kontsedalov S, Zchori-Fein E, Chiel E, Gottlieb Y, Inbar M, Ghanim M. 2008. The presence of Rickettsia is associated with increased susceptibility of Bemisia tabaci (Homoptera: Aleyrodidae) to insecticides. Pest Management Science 64:789–792. DOI: 10.1002/ps.1595.
- Koppenhöfer AM, Jackson TA, Klein MG. 2012. Bacteria for use against soil-inhabiting insects. In: Lacey LA (Ed.) Manual of techniques in invertebrate pathology. Elsevier Academic Press, San Diego. pp. 129–149.
- Krinsky WL. 2019. Beetles (Coleoptera). In: Mullen Gr, Durden LA (Eds.) Medical and veterinary entomology. Academic Press, San Diego. pp. 129–143.
- Kwong WK, Medina LA, Koch H, Sing K-W, Soh EJY, Ascher JS, et al. 2017. Dynamic microbiome evolution in social bees. Science Advances 3:e1600513. DOI: 10.1126/sciadv.1600513.
- Lagerheim G. 1900. Über Lasius fuliginosus (Latr.) und seine Pilzzucht. The Entomologisk Tidskrift 21:17–29.
- Lanan MC, Rodrigues PAP, Agellon A, Jansma P, Wheeler DE. 2016. A bacterial filter protects and structures the gut microbiome of an insect. The ISME Journal 10:1866–1876. DOI: 10.1038/ismej.2015.264.
- Lapeva-Gjonova A. 2013. Ant-associated beetle fauna in Bulgaria: A review and new data. Psyche 2013:14–16.
- Lee SA, Kim Y, Kim JM, Chu B, Joa JH, Sang MK, et al. 2019. A preliminary examination of bacterial, archaeal, and fungal communities inhabiting different rhizocompartments of tomato plants under real-world environments. Scientific Reports 9:1–15.
- Lester PJ, Sébastien A, Suarez AV, Barbieri RF, Gruber MAM. 2017. Symbiotic bacterial communities in ants are modified by invasion pathway bottlenecks and alter host behavior. Ecology 98:861–874. DOI: 10.1002/ecy.1714.
- Lewis Z, Lizé A. 2015. Insect behaviour and the microbiome. Current Opinion in Insect Science 9:86–90. DOI: 10.1016/j.cois.2015.03.003.
- Li H, Medina F, Vinson SB, Coates CJ. 2005. Isolation, characterization, and molecular identification of bacteria from the red imported fire ant (Solenopsis invicta) midgut. Journal of Invertebrate Pathology 89:203–209. DOI: 10.1016/j.jip.2005.05.008.
- Li S-J, Ahmed MZ, Lv N, Shi P-Q, Wang X-M, Huang J-L, et al. 2017. Plant-mediated horizontal transmission of Wolbachia between whiteflies. The ISME Journal 11:1019–1028. DOI: 10.1038/ismej.2016.164.
- Liberti J, Sapountzis P, Hansen LH, Sørensen SJ, Adams RMM, Boomsma JJ. 2015. Bacterial symbiont sharing in Megalomyrmex social parasites and their fungus-growing ant hosts. Molecular Ecology 24:3151–3169.
- Lindström S, Timonen S, Sundström L, Johansson H. 2019. Ants reign over a distinct microbiome in forest soil. Soil Biology and Biochemistry 139:107529. DOI: 10.1016/j.soilbio.2019.107529.
- Liu C, Bai L, Zhao J, Yan K, Xiang W, Wang X. 2016a. Nocardia lasii sp. nov., a novel actinomycete isolated from the cuticle of an ant (Lasius fuliginosus L). Anntonie Van Leeuwenhoek 109:1513–1520. DOI: 10.1007/s10482-016-0753-y.
- Liu C, Li Y, Ye L, Zhao J, Piao C, Li Z, et al. 2016b. Actinocorallia lasiicapitis sp. nov., an actinomycete isolated from the head of an ant (Lasius fuliginosus L.). International Journal of Systematic and Evolutionary Microbiology 66:2172–2177. DOI: 10.1099/ijsem.0.001005.
- Liu L, Zhao XY, Tang QB, Lei CL, Huang QY. 2019. The mechanisms of social immunity against fungal infections in eusocial insects. Toxins (Basel) 11:1–21. DOI: 10.3390/toxins11050244.
- Liu X, Bimerew M, Ma Y, Müller H, Ovadis M, Eberl L, et al. 2007. Quorum-sensing signaling is required for production of the antibiotic pyrrolnitrin in a rhizospheric biocontrol strain of Serratia plymuthica. FEMS Microbiology Letters 270:299–305. DOI: 10.1111/j.1574-6968.2007.00681.x.
- Lozupone C, Knight R. 2005. UniFrac: A new phylogenetic method for comparing microbial communities. Applied and Environmental Microbiology 71:8228–8235. DOI: 10.1128/AEM.71.12.8228-8235.2005.
- Lucas J, Bill B, Stevenson B, Kaspari M. 2017. The microbiome of the ant-built home: The microbial communities of a tropical arboreal ant and its nest. Ecosphere 8:1–14. DOI: 10.1002/ecs2.1639.
- Lucas JM, Madden AA, Penick CA, Epps MJ, Marting PR, Stevens JL, et al. 2019. Azteca ants maintain unique microbiomes across functionally distinct nest chambers. Proceedings of the Royal Society B: Biological Sciences 286:20191026.
- Łukasik P, Newton JA, Sanders JG, Hu Y, Moreau CS, Kronauer DJC, et al. 2017. The structured diversity of specialized gut symbionts of the New World army ants. Molecular Ecology 26:3808–3825.
- Mallott EK, Malhi RS, Amato KR. 2019. Assessing the comparability of different DNA extraction and amplification methods in gut microbial community profiling. Access Microbiology 1:1–15. DOI: 10.1099/acmi.0.000060.
- Martins C, Souza RF, Bueno OC. 2012. Presence and distribution of the endosymbiont Wolbachia among Solenopsis spp. (Hymenoptera: Formicidae) from Brazil and its evolutionary history. Journal of Invertebrate Pathology 109:287–296. DOI: 10.1016/j.jip.2012.01.001.
- Martinson VG, Danforth BN, Minckley RL, Rueppell O, Tingek S, Moran NA. 2011. A simple and distinctive microbiota associated with honey bees and bumble bees. Molecular Ecology 20:619–628. DOI: 10.1111/j.1365-294X.2010.04959.x.
- Maschwitz U, Hölldobler B. 1970. Der Kartonnestbau bei Lasius fuliginosus Latr. (Hym. Formicidae). Zeitschrift für Vergleichende Physiologie 66:176–189. DOI: 10.1007/BF00297777.
- Masella AP, Bartram AK, Truszkowski JM, Brown DG, Naufeld JD. 2012. PANDAseq: Paired-end assembler for Illumina sequences. BMC Bioinformatics 13:31. DOI: 10.1186/1471-2105-13-31.
- McDonald D, Clemente JC, Kuczynski J, Rideout JR, Stombaugh J, Wendel D, et al. 2012. The Biological Observation Matrix (BIOM) format or: How I learned to stop worrying and love the ome-ome. Gigascience 1:1–6. DOI: 10.1186/2047-217X-1-7.
- Meirelles LA, McFrederick QS, Rodrigues A, Mantovani JD, de Melo Rodovalho C, Ferreira H, et al. 2016. Bacterial microbiomes from vertically transmitted fungal inocula of the leaf-cutting ant Atta texana. Environmental Microbiology Reports 8:630–640. DOI: 10.1111/1758-2229.12415.
- Michalkova V, Benoit JB, Weiss BL, Attardo GM, Aksoy S. 2014. Vitamin B6 generated by obligate symbionts is critical for maintaining proline homeostasis and fecundity in tsetse flies. Applied and Environmental Microbiology 80:5844–5853. DOI: 10.1128/AEM.01150-14.
- Mueller UG, Scott JJ, Ishak HD, Cooper M, Rodrigues A. 2010. Monoculture of leafcutter ant gardens. PLoS One 5:1–7. DOI: 10.1371/journal.pone.0012668.
- Nelsen MP, Ree RH, Moreau CS. 2018. Ant–plant interactions evolved through increasing interdependence. Proceedings of the National Academy of Sciences of the United States of America 115:12253–12258.
- North RD, Jackson CW, Howse PE. 1997. Evolutionary aspects of ant-fungus interactions in leaf-cutting ants. Trends in Ecology & Evolution 12:386–389. DOI: 10.1016/S0169-5347(97)87381-8.
- Oh DC, Poulsen M, Currie CR, Clardy J. 2009. Dentigerumycin: A bacterial mediator of an ant-fungus symbiosis. Nature Chemical Biology 5:391–393. DOI: 10.1038/nchembio.159.
- Patricelli D, Sielezniew M, Ponikwicka-Tyszko D, Ratkiewicz M, Bonelli S, Barbero F, et al. 2013. Contrasting genetic structure of rear edge and continuous range populations of a parasitic butterfly infected by Wolbachia. BMC Evolutionary Biology 13:14. DOI: 10.1186/1471-2148-13-14.
- Perlman SJ, Hunter MS, Zchori-Fein E. 2006. The emerging diversity of Rickettsia. Proceedings of the Royal Society B: Biological Sciences 273:2097–2106. DOI: 10.1098/rspb.2006.3541.
- Pierce NE, Braby MF, Health A, Lohman DJ, Mathew J, Rand DB, et al. 2002. The Ecology and Evolution of Ant Association in the Lycaenidae (Lepidoptera). Annual Review of Entomology 47:733–771. DOI: 10.1146/annurev.ento.47.091201.145257.
- Pietri JE, DeBruhl H, Sullivan W. 2016. The rich somatic life of Wolbachia. MicrobiologyOpen 5:923–936. DOI: 10.1002/mbo3.390.
- Pinto-Tomas AA, Anderson MA, Suen G, Stevenson DM, Chu FST, Cleland WW, et al. 2009. Symbiotic nitrogen fixation in the fungus gardens of leaf-cutter ants. Science 326:1120–1123. DOI: 10.1126/science.1173036.
- Pontieri L, Schmidt AM, Singh R, Pedersen JS, Linksvayer TA. 2017. Artificial selection on ant female caste ratio uncovers a link between female-biased sex ratios and infection by Wolbachia endosymbionts. Journal of Evolutionary Biology 30:225–234. DOI: 10.1111/jeb.13012.
- Powell EJ, Eiri D, Moran NA, Rangel J. 2018. Modulation of the honey bee queen microbiota: Effects of early social contact. PLoS One 13:e0200527. DOI: 10.1371/journal.pone.0200527.
- Quast C, Pruesse E, Yilmaz P, Gerken J, Schweer T, Yarza P, et al. 2013. The SILVA ribosomal RNA gene database project: Improved data processing and web-based tools. Nucleic Acids Research 41:D590–D596. DOI: 10.1093/nar/gks1219.
- Ramalho MO, Bueno OC, Moreau CS. 2017a. Microbial composition of spiny ants (Hymenoptera: Formicidae: Polyrhachis) across their geographic range. BMC Evolutionary Biology 17:96. DOI: 10.1186/s12862-017-0945-8.
- Ramalho MO, Bueno OC, Moreau CS. 2017b. Species-specific signatures of the microbiome from Camponotus and Colobopsis ants across developmental stages. PLoS One 12:e0187461. DOI: 10.1371/journal.pone.0187461.
- Ramalho MO, Bueno OC, Moreau CS. 2017c. Microbial composition of spiny ants (Hymenoptera: Formicidae: Polyrhachis) across their geographic range. BMC Evolutionary Biology 17:93.
- Ramalho MO, Martins C, Silva LMR, Martins VG, Bueno OC. 2017. Intracellular symbiotic bacteria of Camponotus textor, Forel (Hymenoptera, Formicidae). Current Microbiology 74:589–597. DOI: 10.1007/s00284-017-1201-6.
- Ramalho MO, Moreau CS, Bueno OC. 2019. The potential role of environment in structuring the microbiota of Camponotus across parts of the body. Advances in Entomology 7:47–70. DOI: 10.4236/ae.2019.73005.
- Ramalho MO, Vieira AS, Pereira MC, Moreau CS, Bueno OC. 2018. Transovarian Transmission of Blochmannia and Wolbachia Endosymbionts in the Neotropical Weaver Ant Camponotus textor (Hymenoptera, Formicidae). Current Microbiology 75:866–873. DOI: 10.1007/s00284-018-1459-3.
- Ranjard L, Poly F, Lata JC, Mougel C, Thioulouse J, Nazaret S. 2001. Characterization of bacterial and fungal soil communities by automated ribosomal intergenic spacer analysis fingerprints: Biological and methodological variability. Applied and Environmental Microbiology 67:4479–4487. DOI: 10.1128/AEM.67.10.4479-4487.2001.
- Rawat SR, Männistö MK, Bromberg Y, Häggblom MM. 2012. Comparative genomic and physiological analysis provides insights into the role of Acidobacteria in organic carbon utilization in Arctic tundra soils. FEMS Microbiology Ecology 82:341–355. DOI: 10.1111/j.1574-6941.2012.01381.x.
- Raychoudhury R, Sen R, Cai Y, Sun Y, Lietze VU, Boucias DG, et al. 2013. Comparative metatranscriptomic signatures of wood and paper feeding in the gut of the termite Reticulitermes flavipes (Isoptera: Rhinotermitidae). Insect Molecular Biology 22:155–171.
- Raymann K, Moran NA. 2018. The role of the gut microbiome in health and disease of adult honey bee workers. Current Opinion in Insect Science 26:97–104. DOI: 10.1016/j.cois.2018.02.012.
- Reeves DD, Price SL, Ramalho MO, Moreau CS. 2020. The diversity and distribution of Wolbachia, Rhizobiales, and Ophiocordyceps within the widespread neotropical turtle ant, Cephalotes atratus (Hymenoptera: Formicidae). Neotropical Entomology 49:52–60. DOI: 10.1007/s13744-019-00735-z.
- Ritpitakphong U, Falquet L, Vimoltust A, Berger A, Métraux JP, L’Haridon F. 2016. The microbiome of the leaf surface of Arabidopsis protects against a fungal pathogen. New Phytologist 210:1033–1043. DOI: 10.1111/nph.13808.
- Russell JA, Funaro CF, Giraldo YM, Goldman-Huertas B, Suh D, Kronauer DJC, et al. 2012. A veritable menagerie of heritable bacteria from ants, butterflies, and beyond: Broad molecular surveys and a systematic review. PLoS One 7:e51027. DOI: 10.1371/journal.pone.0051027.
- Russell JA, Moreau CS, Goldman-Huertas B, Fujiwara M, Lohman DJ, Pierce NE. 2009. Bacterial gut symbionts are tightly linked with the evolution of herbivory in ants. Proceedings of the National Academy of Sciences 106:21236–21241. DOI: 10.1073/pnas.0907926106.
- Sakurai M, Koga R, Tsuchida T, Meng X-Y, Fukatsu T. 2005. Rickettsia Symbiont in the Pea Aphid Acyrthosiphon pisum: Novel Cellular Tropism, Effect on Host Fitness, and Interaction with the Essential Symbiont Buchnera. Applied and Environmental Microbiology 71:4069–4075. DOI: 10.1128/AEM.71.7.4069-4075.2005.
- Sameshima S, Hasegawa E, Kitade O, Minaka N, Matsumoto T. 1999. Phylogenetic comparison of endosymbionts with their host ants based on molecular evidence. Zoological Science 16:993–1000. DOI: 10.2108/zsj.16.993.
- Sanchez-Contreras M, Vlisidou I. 2008. The diversity of insect-bacteria interactions and its applications for disease control. Biotechnology and Genetic Engineering Reviews 25:203–244. DOI: 10.5661/bger-25-203.
- Sapountzis P, Zhukova M, Hansen LH, Sørensen SJ, Schiøtt M, Boomsma JJ. 2015. Acromyrmex leaf-cutting ants have simple gut microbiota with nitrogen-fixing potential. Applied and Environmental Microbiology 81:5527–5537. DOI: 10.1128/AEM.00961-15.
- Schlick-Steiner BC, Steiner FM, Konrad H, Seifert B, Christian E, Moder K, et al. 2008. Specificity and transmission mosaic of ant nest-wall fungi. Proceedings of the National Academy of Sciences 105:940–943. DOI: 10.1073/pnas.0708320105.
- Segers FHID, Kaltenpoth M, Foitzik S. 2019. Abdominal microbial communities in ants depend on colony membership rather than caste and are linked to colony productivity. Ecology and Evolution 9:13450–13467. DOI: 10.1002/ece3.5801.
- Seipke RF, Org Barke J, Heavens D, Yu DW, Hutchings MI. 2013. Analysis of the bacterial communities associated with two ant–plant symbioses. MicrobiologyOpen 2:276–283. DOI: 10.1002/mbo3.73.
- Sen R, Ishak HD, Estrada D, Dowd SE, Hong E, Mueller UG. 2009. Generalized antifungal activity and 454-screening of Pseudonocardia and Amycolatopsis bacteria in nests of fungus-growing ants. Proceedings of the National Academy of Sciences 106:17805–17810. DOI: 10.1073/pnas.0904827106.
- Shen Y, Fu Y, Yu Y, Zhao J, Li J, Li Y, et al. 2017. Psychrobacillus lasiicapitis sp. nov., isolated from the head of an ant (Lasius fuliginosus). International Journal of Systematic and Evolutionary Microbiology 67:4462–4467. DOI: 10.1099/ijsem.0.002315.
- Siles JA, Margesin R. 2017. Seasonal soil microbial responses are limited to changes in functionality at two Alpine forest sites differing in altitude and vegetation. Scientific Reports 7:1–16.
- Simon JC, Sakurai M, Bonhomme J, Tsuchida T, Koga R, Fukatsu T. 2007. Elimination of a specialised facultative symbiont does not affect the reproductive mode of its aphid host. Ecological Entomology 32:296–301. DOI: 10.1111/j.1365-2311.2007.00868.x.
- Stahlhut JK, Desjardins CA, Clark ME, Baldo L, Russell JA, Werren JH, et al. 2010. The mushroom habitat as an ecological arena for global exchange of Wolbachia. Molecular Ecology 19:1940–1952. DOI: 10.1111/j.1365-294X.2010.04572.x.
- Staniec B, Pietrykowska-Tudruj E, Pilipczuk J. 2009. Morphology of the developmental stages of Pella (=Zyras) laticollis (Märkell, 1844) with remarks on its biology (Coleoptera: Staphylinidae). Genus 20:225–242.
- Staniec B, Zagaja M, Pietrykowska-Tudruj E, Wagner GK. 2018. Comparative larval ultramorphology of some myrmecophilous Aleocharinae (Coleoptera, Staphylinidae), with a first description of the larvae of Amidobia talpa (Heer O, 1841) and Oxypoda haemorrhoa (Mannerheim C.G., 1830), associated with the Formica rufa sp. Zookeys 2018:93–114.
- Stoeffler M, Tolasch T, Steidle JLM. 2011. Three beetles-three concepts. Different defensive strategies of congeneric myrmecophilous beetles. Behavioral Ecology and Sociobiology 65:1605–1613. DOI: 10.1007/s00265-011-1171-9.
- Stoll S, Feldhaar H, Fraunholz MJ, Gross R. 2010. Bacteriocyte dynamics during development of a holometabolous insect, the carpenter ant Camponotus floridanus. BMC Microbiology 10:308. DOI: 10.1186/1471-2180-10-308.
- Stouthamer R, Breeuwer JAJ, Hurst GDD. 1999. Wolbachia pipientis: Microbial manipulator of arthropod reproduction. Annual Review of Microbiology 53:71–102. DOI: 10.1146/annurev.micro.53.1.71.
- Szenteczki MA, Pitteloud C, Casacci LP, Kešnerová L, Whitaker MRL, Engel P, et al. 2019. Bacterial communities within Phengaris (Maculinea) alcon caterpillars are shifted following transition from solitary living to social parasitism of Myrmica ant colonies. Ecology and Evolution 9:4452–4464. DOI: 10.1002/ece3.5010.
- Tartally A, Thomas JA, Anton C, Balletto E, Barbero F, Bonelli S, et al. 2019. Patterns of host use by brood parasitic Maculinea butterflies across Europe. Philosophical Transactions of the Royal Society B: Biological Sciences 374:20180202. DOI: 10.1098/rstb.2018.0202.
- Tláskal V, Zrustová P, Vrška T, Baldrian P. 2017. Bacteria associated with decomposing dead wood in a natural temperate forest. FEMS Microbiology Ecology 93:1–13.
- Tolley SJA, Nonacs P, Sapountzis P. 2019. Wolbachia horizontal transmission events in ants: What do we know and what can we learn? Frontiers in Microbiology 10:1–9. DOI: 10.3389/fmicb.2019.00296.
- Van Borm S, Buschinger A, Boomsma JJ, Billen J. 2002. Tetraponera ants have gut symbionts related to nitrogen-fixing root-nodule bacteria. Proceedings of the Royal Society of London. Series B: Biological Sciences 269:2023–2027. DOI: 10.1098/rspb.2002.2101.
- Van Borm S, Wenseleers T, Billen J, Boomsma JJ. 2003. Cloning and sequencing of wsp encoding gene fragments reveals a diversity of co-infecting Wolbachia strains in Acromyrmex leafcutter ants. Molecular Phylogenetics and Evolution 26:102–109. DOI: 10.1016/S1055-7903(02)00298-1.
- Vázquez-Baeza Y, Pirrung M, Gonzalez A, Knight R. 2013. EMPeror: A tool for visualizing high-throughput microbial community data. Gigascience 26:2–16.
- Venturi V. 2006. Regulation of quorum sensing in Pseudomonas. FEMS Microbiology Reviews 30:274–291.
- Vieira AS, Ramalho MO, Martins C, Martins VG, Bueno OC. 2017. Microbial communities in different tissues of Atta sexdens rubropilosa leaf-cutting ants. Current Microbiology 74:1216–1225. DOI: 10.1007/s00284-017-1307-x.
- Viljakainen L, Reuter M, Pamilo P. 2008. Wolbachia transmission dynamics in Formica wood ants. BMC Evolutionary Biology 8:55.
- von Beeren C, Brückner A, Maruyama M, Burke G, Wieschollek J, Kronauer DJC. 2018. Chemical and behavioral integration of army ant-associated rove beetles - a comparison between specialists and generalists. Frontiers in Zoology 15:1–15.
- Wang X, Zhang X, Zhang Z, Lang H, Zheng H. 2018. Honey bee as a model organism to study gut microbiota and diseases. Drug Discovery Today: Disease Models 28:35–42.
- Weinert LA, Werren JH, Aebi A, Stone GN, Jiggins FM. 2009. Evolution and diversity of Rickettsiabacteria. BMC Biology 7:1–15. DOI: 10.1186/1741-7007-7-6.
- Wenseleers T, Ito F, van Borm S, Huybrechts R, Volckaert F, Billen J. 1998. Widespread occurrence of the microorganism Wolbachia in ants. Proceedings Biological Sciences / The Royal Society 265:1447–1452.
- Wernegreen JJ, Kauppinen SN, Brady SG, Ward PS. 2009. One nutritional symbiosis begat another: Phylogenetic evidence that the ant tribe Camponotini acquired Blochmannia by tending sap-feeding insects. BMC Evolutionary Biology 9:1–17. DOI: 10.1186/1471-2148-9-292.
- Williams LE, Wernegreen JJ. 2010. Unprecedented loss of ammonia assimilation capability in a urease-encoding bacterial mutualist. BMC Genomics 11:1–14. DOI: 10.1186/1471-2164-11-687.
- Wilson EO. 1987. Causes of ecological success: The case of the ants. The Journal of Animal Ecology 56:1–9. DOI: 10.2307/4795.
- Ye L, Zhao S, Li Y, Jiang S, Zhao Y, Li J, et al. 2017. Streptomyces lasiicapitis sp. nov., an actinomycete that produces kanchanamycin, isolated from the head of an ant (Lasius fuliginosus L.). International Journal of Systematic and Evolutionary Microbiology 67:1529–1534. DOI: 10.1099/ijsem.0.001756.
- Yun J-H, Roh SW, Whon TW, Jung M-J, Kim M-S, Park D-S, et al. 2014. Insect gut bacterial diversity determined by environmental habitat, diet, developmental stage, and phylogeny of host. Applied and Environmental Microbiology 80:5254–5264. DOI: 10.1128/AEM.01226-14.
- Zchori-Fein E, Borad C, Harari AR. 2006. Oogenesis in the date stone beetle, Coccotrypes dactyliperda, depends on symbiotic bacteria. Physiological Entomology 31:164–169. DOI: 10.1111/j.1365-3032.2006.00504.x.
- Zheng H, Powell JE, Steele MI, Dietrich C, Moran NA. 2017. Honeybee gut microbiota promotes host weight gain via bacterial metabolism and hormonal signaling. Proceedings of the National Academy of Sciences 114:4775–4780. DOI: 10.1073/pnas.1701819114.
- Zhukova M, Sapountzis P, Schiøtt M, Boomsma JJ. 2017. Diversity and transmission of gut bacteria in Atta and Acromyrmex leaf-cutting ants during development. Frontiers in Microbiology 8:1–14. DOI: 10.3389/fmicb.2017.01942.
- Zucchi TD, Guidolin AS, Consoli FL. 2010. Isolation and characterization of actinobacteria ectosymbionts from Acromyrmex subterraneus brunneus (Hymenoptera, Formicidae). Microbiological Research 166:68–76. DOI: 10.1016/j.micres.2010.01.009.