Abstract
Activities of nineteen hydrolases were measured in the digestive systems of predatory and blood-feeding true leeches (Hirudinida) and their closest relatives, Branchiobdellida and Acanthobdellida. Hydrolase activities were analyzed in different parts of the digestive systems: the species-specific anterior part, i.e. jaws, pharynx or proboscis, crop and intestine. The results obtained suggest that food digestion and possible absorption predominate in the intestine of most of the studied Hirudinida and A. peledina, whereas in B. astaci these processes take place in the anterior part of the digestive system and crop. In Erpobdellidae and Piscicola respirans, the activity of acid and alkaline phosphatases, N-acetyl-β-glucosaminidase, leucine and valine arylamidases, and α-fucosidase was also detected in the anterior part of the digestive system. We also detected differences in enzyme occurrence between the studied species, which are probably connected with their different food preferences. Moreover, the presence of the whole spectrum of enzymes in predatory leeches and the absence of trypsin and α-chymotrypsin activity in the crop of all the leeches support the hypothesis that the leech ancestor was a blood-feeder. Our study showed that “Rhynchobdellida” constitute a paraphyletic group which confirms the previous results based on molecular phylogenetics, while Arhynchobdellida appears to be a non-monophyletic group which is not consistent with previous molecular results.
Introduction
Research on enzymes can have alternative character; either enzymes are studied to understand the physiological abilities of an organism, or it can be a perspective challenge for taxonomists as a method of description of new species (Ayala & Powell Citation1972).
The digestive system of ectoparasitic Clitellata, i.e. Branchiobdellida, Acanthobdellida and Hirudinida, similarly to other invertebrates is composed of three distinct regions: the foregut, midgut and hindgut (Fernández et al. Citation1992). The proboscis, jaws and pharynx are ectodermal regions of the foregut, while the midgut is formed by four regions: the esophagus, crop, posterior crop caecum and intestine. The hindgut is a short tube-shaped structure composed of the rectum and anus. They are lined with simple epithelium (Jennings & Gelder Citation1979; Rost-Roszkowska et al. Citation2012). The precise ultrastructure of the digestive cells in the midgut of Hirudinida has been analyzed only in five species: Hirudo medicinalis (Hirudinidae), Haementeria depressa, Helobdella triserialis and Theromyzon rude (Glossiphoniidae) as well as Piscicola geometra (Piscicolidae) (Hammersen & Pokahr Citation1972a, Citation1972b; Fernández et al. Citation1992; Rost-Roszkowska et al. Citation2012, Citation2015). Apart from general morphological and functional descriptions (summarized by Gelder & Williams Citation2015) more detailed studies devoted to the digestive system structure and digestion in Branchiobdellida come from Cambarincola macrodonta (Jennings & Gelder Citation1979). Similarly, studies on the Acanthobdellida digestive system were mainly focused on its general morphology (Epshtein Citation1966; summarized by Bielecki et al. Citation2014b).
Numerous morphological and molecular analyses have confirmed that ectoparasitic and predatory clitellates, i.e. true leeches (Hirudinida), Branchiobdellida and Acanthobdellida, form a monophyletic taxon among Clitellata with Lumbriculida as their sister group (Purschke et al. Citation1993; Martin Citation2001; Siddall et al. Citation2001; Erséus & Källersjö Citation2004; Marotta et al. Citation2008; Rousset et al. Citation2008; Martinez-Ansemil et al. Citation2012; Tessler et al. Citation2018). However, the sister relationships between these three leech-like taxa are still unresolved (Siddall et al. Citation2001; Erséus & Källersjö Citation2004; Marotta et al. Citation2008; Urbisz & Świątek Citation2013; Phillips et al. Citation2019 for more details see Discussion).
Leeches (Hirudinida), a taxon grouping approximately 1000 species (Govedich & Moser Citation2015), seem to be one of the most derived groups of annelids (Sawyer Citation1986; Bielecki Citation1997; Erséus & Källersjö Citation2004; Sket & Trontelj Citation2008; Bielecki et al. Citation2011a, Citation2011b, Citation2012a, Citation2012b, Citation2013; Cichocka et al. Citation2015; Elliott & Dobson Citation2015). Generally, leeches are known for their blood-feeding habits. However, different feeding strategies have evolved among these animals, i.e. ectocommensalism, predation, scavengery and sanguivory (Sawyer Citation1986; Apakupakul et al. Citation1999; Sket & Trontelj Citation2008). Leeches can attack and swallow small animals, such as earthworms, but generally feed on soft animal tissues and body fluids including blood (Pawłowski Citation1936, Citation1968; Lukin Citation1976; Sawyer Citation1986; Bielecki Citation1988a, Citation1988b). A wide variety of animals can be prey for leeches, e.g. earthworms (Pawłowski Citation1936, Citation1968), molluscs (Daniels & Sawyer Citation1975; Klemm Citation1975; Sawyer Citation1986), fish (Bielecki Citation1997; Williams & Burreson Citation2006; Utevsky Citation2007; Kovalenko & Utevsky Citation2015; Adamiak-Brud et al. Citation2016), amphibians (Van der Lande & Tinsley Citation1976), reptiles, water birds (Davies & Wilkialis Citation1981; Bielecki et al. Citation2009; Buczyński et al. Citation2014), and mammals (Hong et al. Citation1999). There are also reports showing opportunistic feeding of leeches on amphibian and fish eggs, but such habits seem to be occasional and therefore they are usually overlooked (Davies & Govedich Citation2001; Light et al. Citation2005).
Branchiobdellidans are obligate ectosymbionts of crayfish. In fact, they are omnivorous animals feeding on different organisms occurring on their host exoskeleton. Their guts may contain algae, diatoms, ciliates, nematodes and oligochaetes, as well as branchiobdellidans, insect larvae and host haemolymph; some of them parasitize crayfish gills (Gelder & Williams Citation2015). Free living branchiobdellidans have not been observed (Sawyer Citation1986; Gelder & Williams Citation2015).
Two described acanthobdellidan species (Acanthobdella peledina and Paracanthobdella livanowi) are regarded as temporary ectoparasites of salmonid fish, feeding on fish blood and soft tissues from the fish body wall. After feeding, acanthobdellidans detach from the host and live freely; especially P. livanowi specimens were frequently found unattached to the fish in the local environment (Livanow Citation1906; Epshtein Citation1966; Sawyer Citation1986; Erséus & Källersjö Citation2004; Kaygorodova et al. Citation2012; Utevsky & Shedko Citation2013a; Utevsky et al. Citation2013b; Bielecki et al. Citation2014b).
To enrich our knowledge about the functioning of a digestive tract in ectoparasitic and predatory clitellates we decided to analyze the occurrence and activity of hydrolases, an important group of digestive enzymes, and finally to compare the obtained data among species. In the present study, hydrolase activity was assessed in eight leech species of different food preference (both sanguivorous and carnivorous) as well as in Branchiobdella astaci (Branchiobdellida) and Acanthobdella peledina (Acanthobdellida), which have not been studied until now, because collecting these unique species is highly problematic due to their occurrence in hardly accessible habitats. For our purposes we analyzed hydrolases within three parts of the digestive system: the foregut (alternatively proboscis, jaws or pharynx – depending on the foregut structure in a given species) and two parts of the midgut – the crop and intestine. Therefore, here we present the first report on types of enzymes synthesized not only in Hirudinida, but also in their relatives: Branchiobdellida and Acanthobdellida.
Materials and methods
Collection of specimens and crude extract preparation
The species studied, their feeding habits and place of collection are listed in . They originated from natural and unpolluted environments. For each species, three specimens were analyzed. All the specimens collected were in a good condition, actively moving and feeding. We assume they were in comparable physiological condition. Predatory species feed in short intervals (every 1–2 days) and had access to large invertebrate prey assemblages at the collection sites. Sanguivores collected directly from their hosts also had direct access to their food sources. Though some sanguivores specializing in fish hosts were collected outside their hosts, we can assume they had free access to their food sources (due to an abundant fish assemblage at the collection sites). It should also be noted that sanguivores (particularly those feeding on tetrapods) are adapted to take food at long time intervals (a few times a year) without any effect on their condition, so it was unlikely that they were captured hungry, taking into account the stage of their life cycle. To collect the material no specific permissions were required for locations/activities, except for H. medicinalis (permission WPN.6401.239.2017.MS).
Table I. The list of studied species, their feeding habits and place of collection
To eliminate bacteria and fungi, each collected specimen was kept for two days in an aqueous solution of antibiotics and fungicides (penicillin 100 U ml−1, streptomycin 100 μl ml−1, nystatin 100 U ml−1) (Dziekońska et al. Citation2009). Then, specimens were frozen at −20°C in vials with physiological saline. After unfreezing, each specimen was dissected and the three parts of the digestive system were carefully isolated: the proboscis, pharynx or jaws depending on the species (see ), the crop, and the intestine. After isolation, fragments of the digestive system were cut lengthwise, and their contents were washed out with 1 ml of physiological saline. Then the samples were crushed in a glass Potter homogenizer with the addition of 1 ml of physiological saline (0.9% NaCl) and sand until a homogeneous suspension was obtained. The homogenates were centrifuged for 10 min at 3 000 g and supernatants were used for the determination of protein content and for enzymatic activity tests.
Table II. Activity of hydrolases in the digestive system of Branchiobdella astaci and Hirudo medicinalis.
Table III. Activity of hydrolases in the digestive system of predatory leeches of the genus Erpobdella and blood feeding Acanthobdella peledina.
Table IV. Activity of hydrolases in the digestive system of rhynchobdellid leeches
It should be noted that field conditions during sampling as well as freezing and unfreezing procedure may affect enzyme activity. For this reason, we treated all specimens in the same way, keeping them under unified laboratory conditions before freezing to standardize samples collected in different conditions and time.
API ZYM analyses
To determine the activity of hydrolases along the digestive system of the analyzed species, we used commercial API ZYM tests (bioMérieux, Lyon, France), which is a laboratory kit for semi-quantitative analysis of production of hydrolytic enzymes. Each strip is composed of 20 microcupules containing dehydrated chromogenic substrates for 19 enzymatic reactions and a control without a substrate. Microcupules contained a buffer with a specific optimum pH value for each enzyme activity as shown in the . Up to now, this type of kit has been used in order to analyze unpurified samples of the digestive systems in invertebrates (Boetius & Felbeck Citation1995; Martin et al. Citation2011; Collin & Starr Citation2013). Nineteen enzymes were identified: peptide hydrolases (leucine, valine, and cystine arylamidases, trypsin, α-chymotrypsin), phosphohydrolases (alkaline and acid phosphatases, naphthol-AS-BI-phosphohydrolase), ester hydrolases (esterase C 4, esterase lipase C 8, lipase C 14), glycosidases (α-galactosidase, β-galactosidase, β-glucuronidase, α-glucosidase, β-glucosidase, N-acetyl-β-glucosaminidase, α-mannosidase and α-fucosidase). Prior to application to the strip, protein content of the crude extracts was measured according to Bradford (Citation1976) with bovine serum albumin (BSA, Sigma-Aldrich Co., St. Louis, USA) as a standard. Next, the crude extracts were diluted with 0.9% NaCl solution to an average protein content of 1.5 mg ml−1. Following the manufacturer’s instructions, 65 µl of the examined solution containing ca 100 µg of protein was added to wells in the provided trays and incubated at 37°C for 4 h. After incubation, the reagents ZymA and ZymB were added to stop the reaction, then exposed to intense fiber-optic light for 5 minutes. The results were expressed using the five-step enzyme activity scale according to the colour chart provided by the manufacturer: 0 – negative reaction; 1 (5 nmol), 2 (10 nmol), 3 (20 nmol), 4 (30 nmol) and 5 (≥ 40 nmol) of suitable colorimetric substrate hydrolyzed during 4 h. The results reported are means of 3 assays.
Data analysis
Principal component analysis and general linear models
Principal component analysis (PCA) (data log-transformed and standardized) was applied to reduce the number of variables for further analyses and to find groups of enzymes with activities correlated with one another. The samples obtained from specific parts of the digestive system of particular species were ordinated along the distinguished principal components to check their similarity with respect to the activities of particular sets of enzymes. Furthermore, species scores for particular principal components were analyzed using general linear models (GLM) with Species as a between-subject factor, Part of the digestive system as a within-subject factor (as three parts were sampled from the same individual), and their interaction. Significant effects of these analyses were further examined using post-hoc Fisher LSD tests with a Dunn-Šidák correction for multiple comparisons.
The PCA was carried out using the Vegan 2.5–5 package for R (Oksanen et al. Citation2019), whereas GLM were run using SPSS 25.0 (IBM Inc.).
Phylogenetic analysis
The values of enzyme activity were coded with the gap-weighting method (Thiele Citation1993). A new character state (xnew) was calculated according to the following formula:
xnew = n*[(x − min)/(max − min)],
where “max” and “min” are the maximum and minimum mean values of the character across all operational taxonomic units (OTUs), “x” is the mean value of the current taxon, and “n” is the number of allowed character states (in the present study n = 10). Codes for all taxa are shown in .
Table V. Matrix of coded enzyme activities in the digestive system in 8 leech species and Acanthobdella peledina and Branchiobdella astaci.
The analyses based on codes for enzyme activities were carried using PAUP* 4.0b (Swofford Citation2002) under the branch-and-bound option. Statistical support for clades was assessed using a bootstrap analysis (BS) for 1000 replicates in PAUP. Additional statistics used here were total tree length (L), consistency index (CI), retention index (RI), rescaled consistency index (RC) and homoplasy index (HI) (). Obtained cladograms were explored in FigTree version 1.4.2 (Rambaut Citation2014).
Table VI. Statistics of obtained phylogenetic trees
Results
Activity of hydrolases
Hydrolytic enzyme activities in different parts of the digestive system in the species examined here (means and standard deviations) are presented in .
In the anterior region of the digestive system and in the crop of B. astaci, similar activities of 5 enzymes were detected (valine and cystine arylamidases, trypsin, lipase C 14 and α-galactosidase). In the intestine of this species only valine and cystine arylamidases were active ().
In H. medicinalis, 12 enzymes were detected (10 of them in the anterior region of the digestive system, 11 in the crop and 12 in the intestine). The highest activity in the digestive system of this leech was exhibited by both analyzed phosphatases and N-acetyl-β-glucosamidase. Two glycosidases (β-glucuronidase and α-fucosidase) had residual activity, especially in the anterior region ().
In E. monostriata, the activity of all the analyzed enzymes was observed. This is especially true for the anterior region where 19 hydrolases were active. Two glycosidases (β-glucuronidase and α-mannosidase) were inactive in the crop and intestine, and esterase C 4 was absent from the intestine. Leucine arylamidase activity was the highest of the tested hydrolases in this species ().
All the tested enzymes were active in E. nigricollis except β-glucuronidase (). α-Galactosidase, β-glucosidase, N-acetyl-β-glucosaminidase and α-fucosidase were not detected in the anterior region of this species, whereas trypsin was missing in the crop and intestine ().
All the examined hydrolases were detected in E. testacea. Sixteen enzymes were active in the anterior region of its digestive system, with trypsin, acid phosphatase and α-mannosidase absent from this part. In the crop, the activity of 18 enzymes (all except alkaline phosphatase) was detected. In the intestine of E. testacea, all the examined hydrolases were active ().
In A. peledina, only 13 enzymes were detected. The activity of trypsin, α-chymotrypsin, lipase C 14, α-galactosidase, α- and β-glucosidases was not identified. Only 6 enzymes were active in the anterior region, 12 in the crop and 11 in the intestine of A. peledina. In this species, the highest activity was exhibited by leucine arylamidase and alkaline phosphatase ().
In Piscicola sp. n., 18 hydrolases were active (all except esterase C4). There was no α -chymotrypsin, α -galactosidase, β-glucuronidase and β-glucosidase activity in the proboscis of this species ().
In the digestive system of P. respirans, all the analyzed enzymes were active. Esterase C 4 was missing in the anterior part of the digestive system, and α-galactosidase did not appear in the crop of this species, whereas its intestine contained all the examined enzymes. In particular, distinctly high esterase lipase C 8 activity was noted in the final section of the digestive system of P. respirans ().
Twelve hydrolases were active in P. costata. All of them were active in the crop and in the intestine. 10 enzymes occurred in the anterior region of the digestive system of this leech (without valine arylamidase and β-galactosidase). Particularly high activity of N-acetyl-β-glucosaminidase was noted in the crop and intestine, whereas alkaline phosphatase was highly active in the intestine of this species. In the anterior part of the digestive system of P. costata, cysteine arylamidase, esterases C4 and C8 and α -fucosidase had very low activities ().
In T. maculosum, the presence of 15 enzymes was confirmed, with lipase C 14, α-galactosidase, β-glucuronidase and β-glucosidase missing. In the anterior region of its digestive system, 11 active hydrolases were observed. Cystine arylamidase, trypsin, α-chymotrypsin, lipase C 14, α-galactosidase, β-glucuronidase, β-glucosidase, and α-mannosidase were not detected in this part. In the crop, activity of α-chymotrypsin was additionally detected. Fourteen enzymes were found in the intestine of T. maculosum, and their activity was relatively high compared to other parts of its digestive system ().
Comparisons among species and parts of the digestive system
The two first principal components (PC1 and PC2) resulting from the PCA analysis explained 92% of the variability in enzyme activities ()). Moreover, the GLM conducted on PC1 and PC2 species scores revealed significant species × part of the digestive system interactions (F2, 18 = 63.0, P < 0.001 and F2, 18 = 45.0, P < 0.001, for PC1 and PC2 respectively), indicating that differences among species varied with specific parts of the digestive system.
Figure 1. Principal component analysis (PCA) of log-transformed and standardized enzyme activities. (a) Correlations of particular enzyme activities with principal components 1 and 2 determined by the PCA. (b) Ordination of leech species along Principal Components 1 and 2. (c, d). Species scores assigned by the PCA for principal component 1 (the horizontal axis in panel b) principal component 2 (the vertical axis in panel b). Enzyme names in panel a: leuc.aryl – leucine arylamidase, val.aryl – valine arylamidase, cyst.aryl – cystine arylamidase, tryp – trypsin, α-chym – α- chymotrypsin, alk.phos – alkaline phosphatase, acid.phos – acid phosphatase, naphphos – naphthol-AS-BI-phosphohydrolase, est.C4 – esterase (C 4), est.lip.C8 – esterase lipase (C 8), lip.C14 – lipase (C 14), α-galact – α-galactosidase, β-galact - β-galactosidase, α-glucos – α-glucosidase, β-glucos – β-glucosidase, N-acet β-glucos – N-acetyl-β-glucosaminidase, α-mannos – α-mannosidase, α-fucos – α-fucosidase, β -glucur – β-glucuronidase. Values for the anterior part of the digestive system, crop and intestine are shown in white, grey and black, respectively. The same lowercase letters labeling the bars in panels c and d denote the lack of significant differences between particular species for a given part of the digestive system. Arrows with asterisks indicate significant differences between particular parts of the digestive system for a given species. All significances are Dunn-Šidák corrected for multiple comparisons
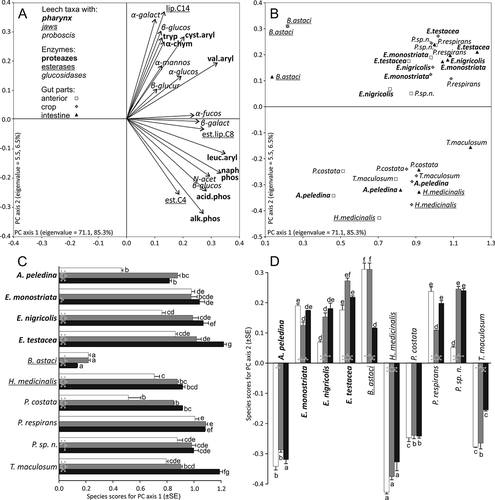
PC1 was positively correlated with the activities of all enzymes, with the highest positive correlations shown for the proteases: leucine arylamidase, valine arylamidase, phosphatases and naphthol AS-BI-phosphohydrolase, as well as with esterase lipase (C8), β-galactosidase and N-acetyl-β-glucosaminidase ()). Thus, PC1 discriminated between the species with generally low and high enzyme numbers and activities. Activities of enzymes associated with PC1 were the lowest in B. astaci ()). The PC1 score for this species differed significantly from those for all other species, irrespective of the part of the digestive system (see Table S1 for the full results of post-hoc analyses). Moreover, enzyme activities in the anterior parts of the digestive system of P. costata and A. peledina, as well as in the crop of P. costata and in the intestine of A. peledina, were significantly lower than in the remaining species ()). The highest enzyme activities were observed in the intestine of E. testacea and T. maculosum ()). They were significantly different from those from the other parts of the digestive system and from the other species. In the anterior part of the digestive system of all species except B. astaci, E. monostriata and P. respirans, enzyme activity was lower than in the subsequent parts of the digestive system. Also, enzyme activity in the crop was lower than in the intestine of all species but A. peledina and B. astaci (where the opposite pattern was found), as well as P. respirans and Piscicola sp. n. (where no differences between these parts were found).
PC2 was correlated with high activities of valine arylamidase, cystine arylamidase, trypsin, α-chymotrypsin and lipase C14 as well as with low activities of phosphatases, esterase C4 and N-acetyl-β-glucosaminidase ()), (see Table S2 for the full results of post-hoc analyses). In general, this component distinguished between two distinct leech species groups, differing from each other in the activities of the above-mentioned enzyme sets. One group included B. astaci, Erpobdellidae, Piscicola sp. n. and P. respirans, which had high PC2 scores ()). Among them, B. astaci had the highest scores for the anterior digestive system part and for the crop, whereas Piscicola sp. n., P. respirans and E. testacea had the highest scores for the intestine. The other group contained T. maculosum, P. costata, A. peledina and H. medicinalis, having low PC2 scores ()). The two latter species had the lowest scores for PC2 and differed significantly with this respect from the other leeches from the second group ()).
Phylogenetic analysis
The phylogenetic analysis based on enzyme activities in particular parts of the digestive system resulted in trees presenting a lack of monophyly both in the traditionally considered groups of rhynchobdellid and arhynchobdellid leeches and at the family level (). The representatives of the genera Piscicola and Erpobdella formed a clade, but within the clade none of the genera was monophyletic. Branchiobdella astaci appeared to be sister to the clade of Piscicola + Erpobdella. The representatives of the family Glossiphoniidae (P. costata and T. maculosum) formed a clade only when enzymes in the intestine were considered ()). In other cases, P. costata formed a sister branch to the clade of Piscicola + Erpobdella + B. astaci ()). Theromyzon maculosum appeared to be sister to H. medicinalis in the cladogram based on the enzyme activities in the entire digestive system and its anterior part ()). The leech-like annelid A. peledina, was placed in the outer position to Hirudinida and B. astaci ()). The highest value of the consistency index (CI = 0.6552) and the lowest tree length (TL = 290) were obtained for the cladogram generated using data of enzyme activities in the anterior part of the digestive system (). Additionally, the analyses setting B. astaci in the outgroup were performed (Fig. S1). The statistics for the cladograms were very similar to these obtained for the cladograms with A. peledina as an outgroup (TL = 959, CI = 0.5944, HI = 0.4056, RI = 0.6125, compare with the ). However, the topology of the cladograms appeared to by slightly different. There are two clades distinguished: one containing sanguivory leeches and A. peledina sister to T. maculosum and H. medicinalis, and the second including mainly sanguivory piscolids and predatory erpobdellids (Fig. S1).
Figure 2. The most parsimonious trees for 8 leech species, as well as Acanthobdella peledina and Branchiobdella astaci based on hydrolytic enzyme activities coded with gap-weighting method in particular parts of the digestive system: (a) – anterior part, (b) – crop, (c) – intestine, (d) – entire digestive system. ▪ – species feeding on tissues, ♦ – species feeding on body fluids. Numbers at nodes indicate bootstrap values > 50
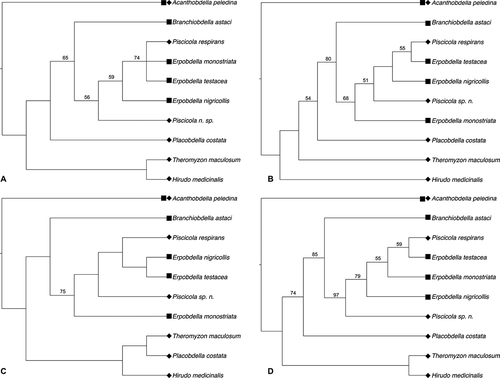
Discussion
Differences in activity of hydrolases in the digestive systems of the studied taxa
Most likely, bloodsucking in leeches (Hirudinida) derived from predatory Annelida, which can be seen in the feeding strategy of leech relatives, the acanthobdellidans A. peledina and Paracanthobdella livanowi (Siddall et al. Citation2001; Erséus & Källersjö Citation2004; Cichocka & Bielecki Citation2015). It should be pointed out here that A. peledina feeds, to some extent, as predatory leeches do, as its pharynx is constructed similarly to that of these annelids (Epshtein Citation1987; Bielecki et al. Citation2014b). Bigger specimens drill holes beneath a dorsal fin to suck blood and often consume large parts of tissue (Andersson Citation1988). In our study, the level of enzyme activity in A. peledina appeared to be similar to bloodsucking leeches (), but the outer position of A. peledina in the cladograms ()) might result from this mixed feeding strategy, consisting in bloodsucking and taking “bites” of solid tissues. Additionally, A. peledina, in contrast to Piscicolidae and similarly to the typical bloodsucking leeches, takes blood and lymph from the deeper layers of the skin. This may explain the result of the PCA and GLM analyses that grouped A. peledina together with H. medicinalis, T. maculosum and P. costata ().
One of the most striking results of the current study was the low number (five) of active enzymes in the digestive system of Branchiobdella astaci. It suggests the high individuality of the digestive physiology of Branchiobdellida in comparison with Acanthobdellida and Hirudinida. It may be connected with the uniqueness of arthropods (crayfish), which are branchiobdellidan hosts (Sawyer Citation1986). The differences may be caused by chemical properties of their food, i.e. arthropod haemolymph (the basic food type), which has already been suggested by other authors (Small et al. Citation2007). Arthropods are prey for predatory erpobdellids, glossiphoniids and a few species of fish leeches (Bielecki et al. Citation2014b). The results of the current study strongly suggest that, contrary to acanthobdellids and true leeches, B. astaci digests food mainly in its foregut and crop (anterior and mid parts of the digestive system), because only two peptide hydrolases (valine arylamidase and cystine arylamidase) were found in the intestine (). Notwithstanding all these differences, in our study B. astaci was placed as a sister branch to the clade of Piscicolidae and Erpobdellidae ()). However, the monophyly of the clade comprising true leeches (Hirudinida), leech-like acanthobdellidans (Acanthobdellida) and crayfish worms (Branchiobdellida) seems to be evident, as has been shown in numerous morphological and molecular analyses (e.g. Purschke et al. Citation1993; Martin Citation2001; Siddall et al. Citation2001; Erséus & Källersjö Citation2004; Marotta et al. Citation2008; Rousset et al. Citation2008; Martinez-Ansemil et al. Citation2012; Tessler et al. Citation2018). The phylogenetic relationships between those taxa are still unresolved (the history of this debate has been summarized by e.g. Bielecki et al. Citation2014a; Tessler et al. Citation2018; Phillips et al. Citation2019). Most morphological analyses have shown that acanthobdellids are sister to true leeches (e.g. Purschke et al. Citation1993; Świątek et al. Citation2012; Urbisz & Świątek Citation2013) and from the early morphological descriptions A. peledina was regarded as an intermediate form between oligochaetous annelids and true leeches (an ancient leech with chaetae) (Livanow Citation1906,; Sawyer Citation1986). On the other hand, the use of such molecular markers as COI and 18S DNA sequences revealed that branchiobdellidans are sister to true leeches with A. peledina as an outgroup (Apakupakul et al. Citation1999; Erséus & Källersjö Citation2004; Siddall et al. Citation2001; Rousset et al. Citation2008). To complicate matters, combined morpho-molecular studies (18S sequences and sperm characters) performed by Marotta et al. (Citation2008) suggested that Acanthobdellida was sister to true leeches.
The latest molecular investigations using new sequences obtained from the freshly collected A. peledina specimens (the formerly used sequences were found to be contaminated) also recovered A. peledina as sister to Hirudinida with Branchiobdellida as an outgroup (Tessler et al. Citation2018; Phillips et al. Citation2019). Phylogenomic analysis performed by Phillips et al. (Citation2019) showed Branchiobdellida as sister to a clade including Hirudinida and Acanthobdella and questioned the hypothesis about Acanthobdella as a “missing link” between true leeches and other clitellates. Being suggested by the results of Tessler et al. (Citation2018) and Phillips et al. (Citation2019) additional analyses using B. astaci as an outgroup were performed in our study (Fig. S1). The outcome, however, did confirm neither the hypothesis about piscicolid/erpobdellid-like ancestor of leeches nor the hypothesis about Acanthobdellida being sister to true leeches. Nevertheless, the branch support values in our cladograms are not satisfactorily high and this probably suggests that enzymatic data used in the analysis are not suitable to be included in the phylogenetic inference alone.
The occurrence of hydrolases and their activity show strong physiological specializations of B. astaci when compared to leeches. The branchiobdellid is clearly separated from other studied taxa considering both number of active enzymes and the place of the highest enzymatic activity which is pictured in the PCA and GLM analysis in this study (). The outcome is also coherent with the latest phylogenomic studies by Phillips et al. (Citation2019). To receive wider picture of relations based on hydrolase activity, enzymatic spectra of other Oligochaeta, such as megadriles, lumbriculids, naidids, etc., should be examined. It would be especially valuable to obtain data about hydrolase activity in lumbriculids, considered as close relatives of leech-like annelids (Martin Citation2001; Siddall et al. Citation2001; Erséus & Källersjö Citation2004; Marotta et al. Citation2008; Rousset et al. Citation2008; Tessler et al. Citation2018). It would also be useful to extend the spectrum of analyzed ectoparasitic clitellates to further species of predatory and sanguivory leeches as well as branchiobdellidans.
Deliberating the evolutionary relationships between B. astaci and leeches, it should be mentioned that a similar picture of the species placed in a sister grouping with Piscicolidae was obtained in the phylogenetic analysis based on morphometric data (Cichocka & Bielecki Citation2015; Thorp et al. Citation2019). In their study, Cichocka and Bielecki (Citation2015) presented some suggestions to explain the similarity and affinity of branchiobdellidans to Piscicolidae fish leeches, e.g. variety in body forms and feeding on crustacean body fluids. All in all, our results suggest some physiological specialization and adaptations of the branchiobdellid species studied, and this seems to be an interesting issue in and of itself. However, as it was mentioned above, the methodological aspect of using enzyme activity levels as data for phylogenetic inference needs to be reconsidered and improved to give more reliable outcome.
The phylogenetic analysis based on the enzymatic activity did not confirm the monophyly of either “Rhynchobdellida” (here considered as Piscicolidae and Glossiphoniidae) or Arhynchobdellida (Erpobdelliformes and Hirudiniformes) (). In our study, Erpobdellidae and Piscicolidae formed one group or clade (). These results seem to be consistent with the hypothesis of the origin of Hirudinida and their ancestor, which most likely was sanguivorous and erpobdellid- or piscicolid-like (Borda & Siddall Citation2004a, Citation2004b; Trontelj et al. Citation1999). Moreover, molecular analyses have shown a close evolutionary relationship between Erpobdellidae and Piscicolidae (Bielecki & Polok Citation2012). Admittedly, Erpobdellidae are considered as predators and Piscicolidae as bloodsuckers, but if we take a closer look at the type of substance they actually feed on, some similarities can be observed. Erpobdellids consume arthropod haemolymph, whereas piscicolids, although defined as bloodsuckers, actually suck lymph, as the amount of blood in the fish body is small. They were usually collected from fins where numerous lymphatic vessels occur, and the digestive system of these leeches was filled with yellowish or amberish fluid. Blood is mainly taken by piscicolids just before the time of reproduction (Bielecki et al. Citation1997, Citation2011b; Cichocka et al. Citation2018). As fish and mammalian lymphatic systems share many molecular and morphological features (Hedrick et al. Citation2013), it can be assumed that the products of fat digestion are transported via lymphatic vessels (Dixon Citation2010). Piscicolids may suck lymph to get these nutrients. Furthermore, as mentioned above, some piscicolids feed on crustaceans by sucking their haemolymph, e.g. Mysidobdella borealis on Mysidae, as well as Baicalobdella torquata and Codonobdella truncata on Gammaridae (Sawyer Citation1986). On the other hand, there are some reports on erpobdellid leeches, e.g. E. octoculata and E. vilnensis found on injured fish, aggregated next to their wounds and probably feeding on the surrounding tissues (Bielecki Citation1977, Citation1997; Cichocka et al. Citation2015; Jabłońska-Barna et al. Citation2017). This may suggest that Erpobdellidae, similar to fish leeches, can also include lymph in their diet.
The last group of taxa considered here shows enzymatic activity of typical bloodsucking leeches, H. medicinalis, T. maculosum and P. costata, grouped along the PC2 axis () whereas phylogenetic analysis placed them in different arrangements depending on the part of the digestive system (). Hirudo medicinalis can suck blood from a wide variety of vertebrates, mainly mammals, but it can also feed on fish and amphibians (Elliot & Dobson Citation2015). Recently it has been suggested that this medicinal leech also attacks birds (Buczyński et al. Citation2014). Placobdella costata drinks blood from turtles, but it was recently shown to attack birds (Bielecki et al. Citation2012a) and humans (Wilkialis Citation1973) as well. Theromyzon maculosum, similar to other species of the genus, is monozoic and stenotrophic, preferring bird blood. The similarity in enzyme activity between T. maculosum, H. medicinalis and P. costata may be explained by their feeding on vertebrate blood.
However, it should be noted that Theromyzon leeches bear some primeval characters (e.g. penis and vagina in the reproductive system, structures of the digestive system divided into several diverticuli) and were formerly named Protoclepsis (Lukin Citation1976; Sawyer Citation1986; Bielecki et al. Citation2009). It is possible that a blood feeding leech ancestor had similar segmentation in the digestive system, as it is known from other polymerized systems in other animals. It should be noted here that Glossiphoniidae, as the most specious group of leeches (Bielecki et al. Citation1999), have the highest number of prey and hosts, including both invertebrates and vertebrates. It seems that a wide variety of feeding habits and unique parental care were the basis for the evolutionary success of these leeches.
Differences in enzyme activity among various regions of the digestive system of the studied taxa
The comparison of enzyme activity among different regions of the digestive system suggests that the digestion and potentially absorption in some species take place in the crop, while in others they are carried out in the intestine (Jenings & Van der Lande Citation1967). Leeches feeding on blood synthesize many substances which are secreted into the host’s body, where they inhibit blood coagulation, reduce blood viscosity or dilate blood vessels (Sawyer Citation1986; Whitaker et al. Citation2005). Moreover, blood-feeding leeches have their midgut (crop and intestine) differentiated into numerous caeca, enlarging its absorbent surface (Sawyer Citation1986; Rost-Roszkowska et al. Citation2012). In the salivary glands of P. geometra, the high activity of esterase, as well as acid and alkaline phosphatases, is combined with the fact that they participate in dissolving the mucus covering the host skin and tissues (Jennings & van der Lande Citation1967; Van der Lande Citation1968). Van der Lande (Citation1968) and Hovingh and Linker (Citation1999) have also described the presence of esterases in the anterior region of the digestive system of P. geometra. Our study has revealed the activity of esterases and peptide hydrolases in the majority of the species examined here. It confirms that the salivary glands participate in the digestion of host tissues, but the differences in the level of enzyme activity result from the different structure of the host’s skin. In many invertebrate parasites, hydrolases can be secreted into the lumen of the digestive system or to the external environment (Hinck & Ivey Citation1976; Matthews Citation1984; Knox & Kennedy Citation1988; Moczoń & Wranicz Citation1999; Jefferies et al. Citation2001; Irwin et al. Citation2004). These enzymes (e.g. phosphatases, aminopeptidases, esterases, glycosidases, etc.) fulfill many functions: the digestion of connective tissues, inhibition of blood coagulation, or protecting the parasite against the response of the host immune system. Moreover, they enable the parasites to penetrate the host body. Such a relationship was observed by Żółtowska et al. (Citation2007), showing a significant role of α -fucosidase of the nematode during penetration of the host tissues.
Generally, in our study the enzyme activities in the anterior part of the digestive system in parasitic leeches appeared to be lower than in predators (). According to Dziekońska et al. (Citation2009), enzymes synthesized in the anterior region of the digestive system in predatory leeches feeding on large invertebrates are responsible for the beginning of digestion. Our study confirmed this statement, because as many as 16 to 19 enzymes have been found to be active in the pharynx of predatory leeches. They possess the whole spectrum of enzymes including those which take part in blood digestion. It reinforces the hypothesis about a blood-feeding ancestor of leeches. However, it should be mentioned that predatory leeches feed on invertebrates which also contain blood or haemolymph in their body.
According to Jenings and Van der Lande (Citation1967), digestion takes part in the crop of P. geometra, where ingested erythrocytes are digested by enzymes, e.g. esterase, for 10 days. Additionally, water and all water-soluble compounds (e.g. glucose) are absorbed in the crop of P. geometra. Moreover, enzymatic (Dziekońska et al. Citation2009) and ultrastructural studies (Rost-Roszkowska et al. Citation2012) showed that digestion and absorption occur in the crop of these leeches. This statement was confirmed in our study as in the crop of the analyzed species (except B. astaci) the activity of acid and alkaline phosphatases was detected. These enzymes are involved in the digestion of numerous nourishments. Our study revealed that the number of active enzymes in the crop of leeches that parasitize fish and predatory leeches is similar (17 to 18 enzymes), as reported by Dziekońska et al. (Citation2009). Moreover, we observed a very low level or absence of trypsin and α-chymotrypsin activity in the crop of all the leeches analyzed during our study. According to Roters and Zebe (Citation1992a, Citation1992b) and Baskova and Zavalova (Citation2001), low concentration or lack of these hydrolases in the crop of H. medicinalis can be caused by the presence of their inhibitors. They inhibit the activity of proteases which are present in leukocytes, protecting the accumulated blood against its digestion. Inhibitors belong to two types: bdellins, which inhibit trypsin, plasmin and acrosine; and eglins, which inhabit chymotrypsin, elastin, cathepsin D and subtilisin (Baskova & Zavalova Citation2001). It has been suggested that, although not all leeches feed on blood, they all have the same pattern of enzymatic activity (Borda & Siddall Citation2004a, Citation2004b). According to Borda and Siddall (Citation2004a), the ancestor of leeches had a similar body to that of the representatives of the family Erpobdellidae or Piscicolidae and was a blood feeder. This hypothesis was also confirmed by further analyses of morphometric characters (Cichocka & Bielecki Citation2015). It can be justified by our current study, as the numbers of active enzymes in Piscicolidae and Erpobdellidae were similar to each other (17–18) and rather different from the number found in H. medicinalis (11) and even A. peledina (12) and B. astaci (5) ().
In Piscicolidae leeches, alkaline phosphatase, acid phosphatase and naphthol-AS-BI-phosphohydrolase had stable activity levels in the entire digestive system. These enzymes are responsible for absorption of food masses. Our results suggest that the digestion takes place along the entire digestive system at a similar level. In the crop and intestine of Piscicolidae, high activity of esterases has been detected, confirming the results of Jennings and van der Lande (Citation1967) and Dziekońska et al. (Citation2009). Similar to other invertebrates (e.g., Fasciola hepatica) these enzymes are associated with intramembranous transport (Humiczewska Citation2002).
In our study, the activity of lipase C14 was not found in the intestine of H. medicinalis, A. peledina, B. astaci, T. maculosum and P. costata. Lipase, as the enzyme responsible for hydrolysis of esters of glycerol and fatty acids, has not been described in the T. tessulatum (Jennings & van der Lande Citation1967), Haemopis sanguisuga, E. octoculata, G. complanata, P. geometra (Dziekońska et al. Citation2009). It has been suggested that endosymbionts participate in the digestion of lipids, so lipase does not have to be synthesized (Jennings & van der Lande Citation1967). In leeches from the genus Theromyzon, endosymbionts (e.g., Aeromonas, Klebsiella, Proteobacteria, Pseudomonas including P. hirudinis, Xanthomonas) accumulate in the cytoplasm of intestinal cells (Büsing et al. Citation1953; Graf Citation1998; Kikuchi & Fukatsu Citation2002; Kunicki–Goldfinger Citation2008). However, the lack of lipase may also be due to the small amount of lipids in their diet.
In the majority of leeches analyzed here, except Piscicolidae, high enzyme activity was detected in the intestine. The high activity of N-acetyl-β-glucosaminidase in the intestine and crop in all of the leeches examined here (with the exception of B. astaci) is connected with the digestion of bacterial cell walls and chitin (Boetius & Felbeck Citation1995; Dziekońska et al. Citation2009) as well as with the hydrolysis of products of digestion of hyaluronic acid, which is a component of connective tissues.
Conclusions
Our studies showed that: (1) the intestine is the main region of the digestive system which is responsible for digestion and possible absorption in Hirudinida and Acanthobdellida, while in Branchiobdellida these processes start in the foregut and crop and only five enzymes was active; (2) the level of enzyme activity and their composition in the anterior region of the digestive system (proboscis, jaws or pharynx) is connected with different modes of feeding; (3) the types of active enzymes are associated with the type of food (the different structure of epidermis/dermis covering the host body) and its chemical character (blood, haemolymph, tissue fluid); (4) the lack or low activity level of trypsin and chymotrypsin in all Hirudinida supports the hypothesis about a blood-feeding ancestor of the leeches; (5) using the activity of enzymes for evolutionary inference, our outcome confirms the previous results, based on molecular phylogenetics, showing that “Rhynchobdellida” constitute a paraphyletic group; (6) Arhynchobdellida appear not to be a monophyletic group in contrast to the earlier studies based on molecular and morphological data; (7) Using enzyme activities levels as data for phylogenetic analysis seems to be highly problematic and requires further considerations for optimization of methodology
Future studies should focus on the precise enzymatic activity in all regions of the digestive system in the remaining taxa of Clitellata, first of all in Lumbriculida, which should enable us to draw conclusions about the evolutionary history of food preferences among ectoparasitic and predatory clitellates. The results presented in this paper form the basis for further research on the relationship between phylogeny and enzymology within the Clitellata.
Supplemental Material
Download TIFF Image (10.2 MB)Supplemental Material
Download MS Word (32.7 KB)Acknowledgements
Publication financed by the funds granted under the Research Excellence Initiative of the University of Silesia in Katowice.
Disclosure statement
No potential conflict of interest was reported by the authors.
Supplementary material
Supplemental data for this article can be accessed here.
References
- Adamiak-Brud Ż, Bielecki A, Kobak J, Jabłońska-Barna I. 2016. Rate of short-term colonization and distribution of leeches (Clitellata: Hirudinida) on artificial substrates. Journal of Zoology 299(3):191–201. DOI:10.1111/jzo.12341.
- Andersson E. 1988. The biology of the fish leech Acanthobdella peledina Grube. Zoologische Beiträge 32:31–50.
- Apakupakul K, Siddall ME, Burreson EM. 1999. Higher level relationships of leeches (Annelida: Clitellata: Euhirudinea) based on morphology and gene sequences. Molecular Phylogenetics and Evolution 12(3):350–359. DOI:10.1006/mpev.1999.0639.
- Ayala FJ, Powell JR. 1972. Allozymes as diagnostic characters of sibling species of Drosophila. Proceedings of the National Academy of Sciences of the USA 69(5):1094–1096. DOI:10.1073/pnas.69.5.1094.
- Baskova IP, Zavalova LL. 2001. Proteinase inhibitors from medicinal leech Hirudo medicinalis. Biochemistry 66:703–714. DOI: 10.1023/a:1010223325313.
- Bielecki A. 1977. Pijawki (Hirudinea) ryb żyjących w rzekach i potokach Kotliny Kłodzkiej. Przegląd Zoologiczny 21:141–145.
- Bielecki A. 1988a. Cystobranchus respirans Troschel, 1850 (Hirudinea, Piscicolidae) w rzece Mąkolnicy. [Cystobranchus respirans Troschel, 1850 (Hirudinea, Piscicolidae) in Mąkolnica river]. Przegląd Zoologiczny 32:53–57.
- Bielecki A. 1988b. Pijawki (Hirudinea) pasożyty ryb. [Leeches (Hirudinea) the fish parasites]. Wiadomości Parazytologiczne 41:2–7.
- Bielecki A. 1997. Fish leeches of Poland in relation to the Palearctic piscicolines (Hirudinea: Piscicolidae: Piscicolinae). Genus 8:223–378.
- Bielecki A, Cichocka JM, Jabłoński A, Jeleń I, Ropelewska E, Biedunkiewicz A, Szlachciak J. 2012a. Coexistence of Placobdella costata (Fr. Müller, 1846) (Hirudinida: Glossiphoniidae) and mud turtle Emys orbicularis. Biologia 67(4):731–738. DOI:10.2478/s11756-012-0069-y.
- Bielecki A, Cichocka JM, Jeleń I, Świątek P, Adamiak-Brud Ż. 2011a. A checklist of leech species from Poland. Wiadomości Parazytologiczne 57:11–20.
- Bielecki A, Cichocka JM, Jeleń I, Świątek P, Płachno BJ, Pikuła D. 2014b. New data about the functional morphology of the chaetiferous leech-like annelids Acanthobdella peledina (Grube, 1851) and Paracanthobdella livanowi (Epshtein, 1966) (Clitellata, Acanthobdellida). Journal of Morphology 275:528–539. DOI: 10.1002/jmor.20235.
- Bielecki A, Cichocka JM, Świątek P, Gorzel M. 2013. A new leech species from the river Łyna near Olsztyn, in Poland (Clitellata: Hirudinida: Piscicolidae). Journal of Parasitology 99(3):467–474. DOI:10.1645/GE-3154.1.
- Bielecki A, Cichocka JM, Terlecki J, Witkowski A. 2011b. Invasion of the leech Piscicola respirans Troschel, 1850 (Hirudinea, Piscicolidae) on fins of European grayling Thymallus thymallus (L.). Biologia 66(2):294–298. DOI:10.2478/s11756-011-0019-0.
- Bielecki A, Cios S, Cichocka JM, Pakulnicka J. 2012b. Piscicola siddalli n. sp., a leech species from the United Kingdom (Clitellata: Hirudinida: Piscicolidae). Comparative Parasitology 79(2):219–230. DOI:10.1654/4511.1.
- Bielecki A, Palińska K, Cichocka JM. 2009. New data about rare leech species – Theromyzon maculosum (Rathke, 1862) (Hirudinida: Glossiphoniidae). Teka Komitetu Ochrony Kształtowania Środowiska Przyrodniczego - OL PAN 6:13–20.
- Bielecki A, Polok K. 2012. Genetic variation and species identification among selected leeches (Hirudinea) revealed by RAPD markers. Biologia 67(4):721–730. DOI:10.2478/s11756-012-0063-4.
- Bielecki A, Rybak J, Lukowiak-Bielecka M. 1999. Glossiphoniidae Vaillant, 1850 (Hirudinea) of Poland – Systematics and perspectives of studies. Wiadomości Parazytologiczne 45:29–61.
- Bielecki A, Świątek P, Cichocka JM, Siddall ME, Urbisz AZ, Płachno BJ. 2014a. Diversity of features of the female reproductive system and other morphological characters in leeches (Citellata, Hirudinida) in phylogenetic conception. Cladistics 30(5):540–554. DOI:10.1111/cla.12058.
- Boetius A, Felbeck H. 1995. Digestive enzymes in marine invertebrates from hydrothermal vents and other reducing environments. Marine Biology 122(1):105–113. DOI:10.1007/BF00349283.
- Borda E, Siddall ME. 2004a. Archynchobdellida (Annelida: Oligochaeta: Hirudinida): Phylogenetic relationships and evolution. Molecular Phylogenetics and Evolution 30(1):213–225. DOI:10.1016/j.ympev.2003.09.002.
- Borda E, Siddall ME. 2004b. Review of the evolution of life history strategies and phylogeny of the Hirudinida (Annelida: Oligochaeta). Lauterbornia 52:5–25.
- Bradford MM. 1976. A rapid and sensitive method for the quantitation of microgram quantities of protein utilizing the principle of protein–dye binding. Analytical Biochemistry 72(1–2):248–254. DOI:10.1016/0003-2697(76)90527-3.
- Buczyński P, Tończyk G, Bielecki A, Cichocka JM, Kitowski I, Grzywaczewski G, Buczyńska E. 2014. Occurrence of the medicinal leech (Hirudo medicinalis) in birds’ nests. Biologia 69(4):484–488. DOI:10.2478/s11756-014-0329-0.
- Büsing KH, Dőli W, Freytag K. 1953. Die Bakterienflora der medizinischen Blutegel. Archiv für Mikrobiologie 19(1):52–86. DOI:10.1007/BF00412315.
- Cichocka JM, Bielecki A. 2015. Phylogenetic utility of the geometric model of the body form in leeches (Clitellata: Hirudinida). Biologia 70(8):1078–1092. DOI:10.1515/biolog-2015-0121.
- Cichocka JM, Bielecki A, Kulikowski M, Jabłońska-Barna I, Najda K. 2018. New record of the fish leech Piscicola pojmanskae (Annelida: Hirudinida: Piscicolidae) - DNA barcoding and phylogeny. Biologia 73(7):693–701. DOI:10.2478/s11756-018-0081-y.
- Cichocka JM, Bielecki A, Kur J, Pikuła D, Kilikowska A, Biernacka B. 2015. A new leech species (Hirudinida: Erpobdellidae: Erpobdella) from a cave in the West Azerbaijan province of Iran. Zootaxa 4013:413–427. DOI: 10.11646/zootaxa.4013.3.5.
- Collin R, Starr MJ. 2013. Comparative ontogenetic changes in enzyme activity during embryonic development of Calyptraeid Gastropods. Biology Bulletin 225:8–17. DOI: 10.1086/BBLv225n1p8.
- Daniels BA, Sawyer RT. 1975. THE BIOLOGY OF THE LEECH Myzobdella lugubris INFESTING BLUE CRABS AND CATFISH. Biology Bulletin 148(2):193–198. DOI:10.2307/1540542.
- Davies RW, Govedich FR. 2001. Annelida: Euhirudinea and Acanthobdellidea. In: Thorp JH, Covich AP, editors. Ecology and classification of north american freshwater invertebrates. San Diego: Academic Press. pp. 465–504.
- Davies RW, Wilkialis J. 1981. A preliminary investigation on the effects of parasitism of domestic ducklings by Theromyzon rude (Hirudinoidea: Glossiphoniidae). Canadian Journal of Zoology 59(6):1196–1199. DOI:10.1139/z81-170.
- Dixon JB. 2010. Lymphatic lipid transport: sewer or subway? Trends Endocrinology and Metabolism 21(8):480–487. DOI:10.1016/j.tem.2010.04.003.
- Dziekońska J, Bielecki A, Palińska K. 2009. Activity of selected hydrolytic enzymes from leeches (Clitellata: Hirudinida) with different feeding strategies. Biologia 64:370–376. DOI: 10.2478/s11756-009-0048-0.
- Elliott JM, Dobson M. 2015. Freshwater leeches of Britain and Ireland. Keys to the Hirudinea and a review of their ecology. In: Crowden A, editor. Scientific publications of freshwater biological association 69. Ambleside: Freshwater Biological Association. pp. 1–108
- Epshtein VM. 1966. Acanthobdella livanowi sp. n., a new species of the ancient leeches (Archihirudinea) from Kamchatka. Doklady Akademii Nauk SSSR 168:955–958.
- Epshtein VM. 1987. Pijavki. [Leeches]. In: Bauer ON, editor. Opredelitel parazitov presnovodnych rib fauni SSSR. Akad. Nauk SSSR. Nauka, Leningrad: Zoologitseskii Institut, Izdat. pp. 340–372.
- Erséus C, Källersjö M. 2004. 18S rDNA phylogeny of Clitellata (Annelida). Zoologica Scripta 33(2):187–196. DOI:10.1111/j.1463-6409.2004.00146.x.
- Fernández J, Tellez V, Olea N. 1992. Hirudinea. In: Harrison FW, Gardiner SL, editors. Microscopic Anatomy of Invertebrates. Annelida. Vol. 7. New York: Wiley-Liss. pp. 323–394.
- Gelder SR, Williams BW. 2015. Clitellata: Branchiobdellida. In: Thorp JH, Rogers DC, editors. Thorp and covich’s freshwater invertebrates. Vol. i. ecology and general biology. Cambridge: Academic Press. pp. 551–563.
- Govedich FR, Moser WE. 2015. Clitellata: Hirudinida and Acanthobdellida. In: Thorp JH, Rogers DC, editors. Thorp and Covich’s freshwater invertebrates. Vol. I. Ecology and general biology. Cambridge: Academic Press. pp. 565–588.
- Graf J. 1998. Symbiosis of Aeromonas veronii biovar sobria and H. medicinalis. Infection and Immunity 67(1):1–7. DOI:10.1128/IAI.67.1.1-7.1999.
- Hammersen F, Pokahr A. 1972a. Epithelial ultrastructure of the alimentary tract of Hirudo medicinalis L.. Zeitschrift für Zellforschung und mikroskopische Anatomie 125(3):378–403. DOI:10.1007/BF00306633.
- Hammersen F, Pokahr A. 1972b. Epithelial ultrastructure of the alimentary tract of Hirudo medicinalis L.. Zeitschrift für Zellforschung und mikroskopische Anatomie 125(4):532–552. DOI:10.1007/BF00306658.
- Hedrick MS, Hillman SS, Drewes RC, Withers PC. 2013. Lymphatic regulation in nonmammalian vertebrates. Journal of Applied Physiology 115(3):297–308. DOI:10.1152/japplphysiol.00201.2013.
- Hinck LW, Ivey MH. 1976. Proteinase activity in Ascaris suum eggs, hatching fluid, and excretions-secretions. Journal of Parasitology 62(5):771–774. DOI:10.2307/3278959.
- Hong SJ, Sawyer RT, Kang KW. 1999. Prolonged bleeding from the bite of the Asian medicinal leechHirudo nipponia. Comparative Haematology International 9(3):125–131. DOI:10.1007/BF02600370.
- Hovingh P, Linker A. 1999. Hialuronidase activity in leeches (Hirudinea). Comparative Biochemistry and Physiology B 124(3):319–326. DOI:10.1016/S0305-0491(99)00128-5.
- Humiczewska M. 2002. Some specific and non-specific phosphatases of the sporocyst of Fasciola hepatica. II. Enzymes associated with the membrane transport. Folia Parasitologica 49(3):221–226. DOI:10.14411/fp.2002.040.
- Irwin JA, Morrisey PEW, Ryan JP, Walsche A, O’Neill SM, Carrington SD, Dalton JP. 2004. Glycosidase activity in the excretory-secretory products of the liver fluke, Fasciola hepatica. Parasitology 129(4):465–472. DOI:10.1017/S0031182004005803.
- Jabłońska–Barna I, Bielecki A, Cichocka JM, Buczyńska E, Buczyński P, Walerzak A, Szlauer–Łukaszewska A. 2017. Environmental factors determining the distribution pattern of leeches (Clitellata: Hirudinida) in large river systems: A case study of the Oder river system. Biologia 72(4):407–416. DOI:10.1515/biolog-2017-0043.
- Jefferies JR, Campbell AM, van Rossum AJ, Barrett J, Brophy PM. 2001. Proteinase analysis of Fasciola hepatica excretory-secretory products. Proteomics 1:1128–1132. DOI: 10.1002/1615-9861(200109)1:9<1128::AID-PROT1128>3.0.CO;2-0.
- Jennings JB, Gelder SR. 1979. Gut structure, feeding and digestion in the Branchiobdellid Oligochaete Cambarincola Macrodonta Ellis 1912, an ectosymbiote of the freshwater crayfish Procambarus CLARKII. The Biological Bulletin 156(3):300–314. DOI:10.2307/1540919.
- Jennings JB, van der Lande V. 1967. Histochemical and bacteriological studies on digestion in nine species of leech. The Biological Bulletin 33(1):166–183. DOI:10.2307/1539801.
- Kaygorodova IA, Dzyuba EV, Pronin NM. 2012. Leech-like parasites (Clitellata, Acanthobdellida) infecting native and endemic eastern Siberian salmon fishes. The Scientific World Journal 2012:1–8. DOI: 10.1100/2012/652827.
- Kikuchi Y, Fukatsu T. 2002. Endosymbiotic Bacteria in the Esophageal Organ of Glossiphoniid Leeches. Applied and Environmental Microbiology 68(9):4637–4641. DOI:10.1128/aem.68.9.4637-4641.2002.
- Klemm DJ. 1975. Studies on the feeding relationships of leeches (Annelida: Hirudinea) as natural associates of mollusks. Sterkiana 58:1–50.
- Knox P, Kennedy MW. 1988. Proteinases released by the parasitic larval stages of Ascaris suum, and their inhibition by antibody. Molecular and Biochemical Parasitology 28(3):207–216. DOI:10.1016/0166-6851(88)90005-9.
- Kovalenko MV, Utevsky SY. 2015. Comparative structural analysis of jaws of selected blood-feeding and predacious arhynchobdellid leeches (Annelida: Clitellata: Hirudinida). Zoomorphology 134(1):33–43. DOI:10.1007/s00435-014-0245-4.
- Kunicki–Goldfinger WJH. 2008. Życie bakterii [The life of bacteria]. Warszawa: Wydawnictwo Naukowe PWN.
- Light JE, Fiumera AC, Porter BA. 2005. Egg-feeding in the freshwater piscicolid leech Cystobranchus virginicus (Annelida, Hirudinea). Invertebrate Biology 124(1):50–56. DOI:10.1111/j.1744-7410.2005.1241-06.x.
- Livanow N. 1906. Acanthobdella peledina Grube, 1851. Zoologische Jahrbücher. Abteilung für Anatomie und Ontogenie der Tiere 22:637–866.
- Lukin EJ. 1976. Leeches. Fauna SSSR. Leningrad: Academy of Science of the SSSR.
- Marotta R, Ferraguti M, Erseus C, Gustavsson LM. 2008. Combined-date phylogenetics and character evolution of Clitellata (Annelida) using 18S rDNA and morphology. Zoological Journal of the Linnean Society 154(1):1–26. DOI:10.1111/j.1096-3642.2008.00408.x.
- Martin GG, Martin A, Tsai W, Hafner JC. 2011. Production of digestive enzymes along the gut of the giant keyhole limpet Megathura crenulata (Mollusca: Vetigastropoda). Comparative Biochemistry and Physiology – Part A: Molecular and Integrative Physiology 160(3):365–373. DOI:10.1016/j.cbpa.2011.07.003.
- Martin P. 2001. On the origin of the Hirudinea and the demise of the Oligochaeta. Proceedings of the Royal Society of London 268:1089–1098. DOI: 10.1098/rspb.2001.1616.
- Martinez-Ansemil E, Creuze Des Chatelliers M, Martin P, Sambugar B. 2012. The Parvidrilidae – A diversified groundwater family: Description of six new species from southern Europe and clues for its phylogenetic position within Clitellata (Annelida). Zoological Journal of the Linnean Society 166:530–558. DOI: 10.1111/j.1096-3642.2012.00857.x.
- Matthews B. 1984. The source, release and specificity of proteolytic enzyme activity produced by Anisakis simplex larvae (Nematoda: Ascaridida) in vitro. Journal of Helminthology 58(3):175–185. DOI:10.1017/S0022149X00026924.
- Moczoń T, Wranicz M. 1999. Trichinella spiralis: Proteinases in the larvae. Parasitology Research 85:47–58.
- Oksanen J, Blanchet FG, Friendly M, Kindt R, Legendre P, McGlinn D, McGlinn D, Minchin PR, O’Hara RB, Simpson GL, Solymos P, Stevens MHH, Szoecs E, Wagner H 2019. Vegan: community ecology package. R Package Version 2.5-5. Available: https://cran.r-project.org/web/packages/vegan/vegan.pdf
- Pawłowski LK. 1936. Pijawki (Hirudinea) [Leeches]. Fauna Słodkowodna Polski 26:1–176.
- Pawłowski LK. 1968. Pijawki (Hirudinea) [Leeches]. Katalog Fauny Polski 11:l–94.
- Phillips AJ, Dornburg A, Zapfe KL, Anderson FE, James SW, Erseus C, Moriarty Lemmon E, Lemmon AR, Williams BW. 2019. Phylogenomic Analysis of a Putative Missing Link Sparks Reinterpretation of Leech Evolution. Genome Biology and Evolution 11(11):3082–3093. DOI:10.1093/gbe/evz247.
- Purschke G, Westheide W, Rohde D, Brinkhurst RO. 1993. Morphological reinvestigation and phylogenetic relationship of Acanthobdella peledina (Annelida, Clitellata). Zoomorphology 113(2):91–101. DOI:10.1007/BF00403087.
- Rambaut A 2014. FigTree v1.4.2: Tree figure drawing tool. Available: http://tree.bio.ed.ac.uk/software/figtree accessed March 2019 15.
- Rost-Roszkowska MM, Świątek P, Kszuk M, Główczyk K, Bielecki A. 2012. Morphology and ultrastructure of the midgut in Piscicola geometra (Annelida, Hirudinea). Protoplasma 249:1037–1047. DOI: 10.1007/s00709-011-0337-7.
- Rost-Roszkowska MM, Świątek P, Poprawa I, Rupik W, Swadźba E, Kszuk-Jendrysik M. 2015. Ultrastructural analysis of apoptosis and autophagy in the midgut epithelium of Piscicola geometra (Annelida, Hirudinida) after blood feeding. Protoplasma 252(5):1387–1396.
- Roters FJ, Zebe E. 1992a. Protease inhibitors in the alimentary tract of the medicinal leech Hirudo medicinalis: In vivo and in vitro studies. Journal of Comparative Physiology B 162(1):85–92. DOI:10.1007/BF00257940.
- Roters FJ, Zebe E. 1992b. Proteinases of the medicinal leech Hirudo medicinalis: Purification and partial characterisation of three enzymes from the digestive tract. Comparative Biochemistry and Physiology 102:627–634.
- Rousset V, Plaisance L, Erséus C, Siddall ME, Rouse GW. 2008. Evolution of habitat preference in Clitellata (Annelida). Biological Journal of the Linnean Society 95(3):447–464. DOI:10.1111/j.1095-8312.2008.01072.x.
- Sawyer RT. 1986. Leech Biology and Behaviour. Vols. I, II, III. Oxford: Clarendon Press.
- Siddall ME, Apakupakul K, Burreson EM, Coates KA, Erséus C, Gelder SR, Trapido-Rosenthal H. 2001. Validating livanow: molecular data agree that leeches, branchiobdellidans, and Acanthobdella peledina form a monophyletic group of Oligochaetes. Molecular Phylogenetics and Evolution 21(3):346–351. DOI:10.1006/mpev.2001.1021.
- Sket B, Trontelj P. 2008. Global diversity of leeches (Hirudinea) in freshwater. Hydrobiologia 595:129–137. DOI: 10.1007/s10750-007-9010-8.
- Świątek P, Urbisz AZ, Strużyński W, Płachno BJ, Bielecki A, Cios S, Salonen E, Klag J. 2012. Ovary architecture of two branchiobdellid species and Acanthobdella peledina (Annelida, Clitellata). Zoologischer Anzeiger 251(1):71–82. DOI:10.1016/j.jcz.2011.08.001.
- Small H, Shields J, Neil D, Taylor A, Coombs G. 2007. Differences in enzyme activities between two species of Hematodinium, parasitic dinoflagellates of crustaceans. Journal of Invertebrate Pathology 94(3):175–183. DOI:10.1016/j.jip.2006.10.004.
- Swofford DL. 2002. PAUP*. Phylogenetic Analysis Using Parsimony (*and Other Methods). Version 4. Sunderland, Massachusetts: Sinauer Associates. http://www.sinauer.com/paup-phylogenetic-analysis-using-parsimony-and-other-methods-4-0-beta.html accessed 12 March 2019
- Tessler M, de Carle D, Voiklis ML, Gresham OA, Neumann JS, Cios S, Siddall ME. 2018. Worms that suck: Phylogenetic analysis of Hirudinea solidifies the position of Acanthobdellida and necessitates the dissolution of Rhynchobdellida. Molecular Phylogenetics and Evolution 127:129–134. DOI: 10.1016/j.ympev.2018.05.001.
- Thiele K. 1993. The holy grail of the perfect character: The cladistic treatment of morphometric data. Cladistics 9:295–304. DOI: 10.1111/j.1096-0031.1993.tb00226.x.
- Thorp JH, Lovell LL, Timm T, Martin P, Gelder SR, Govedich FR, Moser WE, Nakano T, Bielecki A, Bain BA, Utevsky S, Gil J, Glasby CJ, Martin D. 2019. Phylum Annelida. In: Rogers D, Thorp JH, editors. Keys to Palaearctic Fauna: Thorp and Covich’s Freshwater Invertebrates Vol. IV. Elsevier: Academic Press. pp. 357–518. DOI:10.1016/B978-0-12-385028-7.00012-3.
- Trontelj P, Sket B, Steinbrück G. 1999. Molecular phylogeny of leeches: Congruence of nuclear and mitochondrial rDNA data sets and the origin of bloodsucking. Journal of Zoological Systematics and Evolutionary Research 37:141–147. DOI: 10.1111/j.1439-0469.1999.00114.x.
- Urbisz AZ, Świątek P. 2013. Ovary organization and oogenesis in two species of Lumbriculida (Annelida, Clitellata). Zoology 116(2):118–128. DOI:10.1016/j.zool.2012.10.003.
- Utevsky AY. 2007. Antarctic Piscicolid Leeches. Bonner Zoologischer Monographien 54:1–80.
- Utevsky SY, Shedko MB. 2013a. Order Hirudinida. Check-list of species of free-living invertebrates of the Russian Far Eastern seas. Vol. 78. St. Petersburg: Russian Academy of Sciences Zoological Institute.
- Utevsky SY, Sokolov SG, Shedko MB. 2013b. New records of the chaetiferous leech-like annelid Paracanthobdella livanowi (Epshtein, 1966) (Annelida: Clitellata: Acanthobdellida) from Kamchatka, Russia. Systematic Parasitology 84(1):71–79. DOI:10.1007/s11230-012-9390-7.
- Van der Lande V. 1968. Esteras activity in certain glands of leeches. Comparative Biochemistry and Physiology 25:447–456.
- Van der Lande VM, Tinsley RC. 1976. Studies on the anatomy, life history and behaviour of Marsupiobdella africana (Hirudinea: Glossiphoniidae). Journal of Zoology 180(4):537–563. DOI:10.1111/j.1469-7998.1976.tb04703.x.
- Whitaker IS, Cheung CK, Chahal CAA, Karoo ROS, Gulati A, Foo ITH. 2005. By what mechanism do leeches help to salvage ischaemic tissues? British Journal of Oral Maxillofacial Surgery 43:155–160. DOI: 10.1016/j.bjoms.2004.09.012.
- Wilkialis J. 1973. The biology of nutrition in Haementeria costata (Fr. Müller, 1846). Zoologica Poloniae 23(4):213–225.
- Żółtowska K, Dmitryjuk M, Rokicki J, Łopieńska-Biernat E. 2007. Hydrolases of Hysterothylacium aduncum (Nematoda). Annals of Parasitology 53:91–95.
- Williams JI, Burreson EM. 2006. Phylogeny of the fish leeches (Oligochaeta, Hirudinida, Piscicolidae) based on nuclear and mitochondrial genes and morphology. Zoologica Scripta 35(6):627–639. DOI:10.1111/j.1463-6409.2006.00246.x.