Abstract
Biofortification of plants with selenium (Se) is one of the current trends in agriculture. This treatment enriches plants with this essential element for humans and animals, and also increases plant resistance to stress factors. The current knowledge of the interactions between Se and plants is well documented but between the plants biofortificated with Se and phytophages is insufficient and this problem should be further investigated. The research has focused on the effect of the pre-treatment of pea with Se on the pea aphid feeding behaviour and demographic parameters of the aphid population, and also has shown the activity of antioxidant (superoxide dismutase and catalase), detoxifying (S-glutathione transferase and β-glucosidase) and redox (peroxidase and polyphenol oxidase) enzymes in tissues of Acyrthosiphon pisum. The analysis of feeding models recorded using the Electronic Penetration Graph technique has shown that the pre-treatment of peas with Se affects the activity of aphids during the feeding. The use of 10 and 20 µM sodium selenite and sodium selenate caused changes in A. pisum probing and feeding behavior. What is more, Se reduced the activity of aphids associated with the phloem phase and caused an extension of non-probing time and non-phloem penetration. The effect of Se on the demographic population parameters of the aphid was also observed in the significant reduction of female fertility and the reproductive potential of the insects. Selenium enrichment of plants colonized by aphids increased the activity of antioxidant, detoxifying and redox enzymes in the tissues of A. pisum. It was also found that both Se taken up by plants, as well as, the feeding of aphids resulted in greater synthesis of flavonols and carotenoids in pea leaves.
Introduction
Pea (Pisum sativum L.) is the species of great economic importance, the most widely grown legume in Europe and the second-most in the world. Numerous pea varieties are used as human food, forage for animals or green manures (Pavek Citation2012; FAOSTAT Citation2016).
Phloem-feeding aphids (Aphidoidea) are major agricultural pests worldwide, depriving plants of photosynthates and vectoring plant pathogenic viruses. Aphids are important phytophages among a variety of pests feeding on legumes. The pea aphid [Acyrthosiphon pisum (Harris) (Hemiptera: Aphididae)] is a monoecious, oligophagous species infesting all plant species of the Fabaceae family (Holman Citation2009). The feeding phase (i.e. reaching the phloem) is proceeded by the probing, which includes the penetration of other tissues (Goławska Citation2007).
Selenium (Se) is an essential component of human and animal cells, but it is not considered to be an essential element for higher plants. Soils of some areas of North America, New Zealand, Australia, Asia, Europe, and Africa are Se-deficient. It is reported that 15% of human population suffer from Se deficiency (Combs Citation2001; White & Broadley Citation2009). Since much of Se in human diet derives directly or indirectly from plant food, numerous studies are focused on the biofortification of edible crops with this element. Inorganic fertilization is the most common practice to enhance Se level in crop plants (Ros et al. Citation2016). Pea belongs to the group of crop plants which are subject to biofortification with Se (Pablociones et al. Citation2013). Although Se biofortification has been introduced into agricultural practice, it should be used with great caution, since in contrast to most trace elements, the gap between deficiency and toxic dose is very small (Hartikainen et al. Citation2000). Moreover, it is difficult to predict the effects of Se fertilization on other organisms, i.e. on insect pests.
Selenium exerts dual effects on living organisms – at low concentrations it is beneficial, however, at high concentrations, it is toxic (Hartikainen et al. Citation2000; Combs Citation2001). It is difficult to appoint the common ranges of beneficial and toxic concentrations of Se, because the plant response to this element depends on plant species, developmental stage, form of Se and conditions of root environment (Terry et al. Citation2000). Numerous reports have been published regarding the beneficial antioxidant effects of Se in the reduction of stress caused by various factors (Gupta & Gupta Citation2017). However, its role under stress induced by aphid attack is not yet well documented. The toxicity of Se is attributed to its pro-oxidative activity, as well as, its incorporation into cysteine and methionine, and the non-specific selenoproteins formation (Terry et al. Citation2000; Kolbert et al. Citation2016; White Citation2016).
Studies on the interactions of unfavourable factors affecting plants have proven that there is a so-called phenomenon of cross-interactions. It covers increasing resistance or susceptibility to a stress factor, as a result of an earlier action of another factor (Bandurska et al. Citation2013; Durak et al. Citation2020). In our preliminary research, we found that the treatment of pea seedlings with Se affected negatively the feeding behaviour and demographic parameters of the pea aphid colonizing the seedlings. The main goal of the research was to check whether the stress triggered by the treatment of pea seedlings with Se (earlier stress factor) is the cause of a negative effect on the pea aphid feeding (later stress factor).
The aim of the study was also to investigate the effect of Se in pea seedlings on pea aphid feeding behaviour and on the demographic parameters of aphid population, as well as to determine the activities of antioxidant (superoxide dismutase and catalase), detoxifying (glutathione S-transferase and β-glucosidase) and redox (peroxidase and polyphenol oxidase) enzymes in A. pisum tissues.
Material and methods
Material
Plants
Pea (Pisum sativum L. cultivar Akord) seeds used in the experiments were obtained from the Plant Breeding Company in Tulce near the city of Poznań, Poland. Pea seedlings were grown in Hoagland nutrient solution in a controlled environment of a growth chamber with the following conditions: under luminescent light with photon flux density 135 μmol m−2 s−1 (Philips lamps), a 14/10 h day/night photoperiod, at 27/23°C day/night temperatures and relative humidity of about 60%. Nutrient solution was aerated with an aeration system commonly used for fishkeeping in aquariums. Selenium was supplied to nutrient solution in two forms: sodium selenate or sodium selenite, at concentrations of 10 and 20 µM, when plants were in the stage of the first pair of leaves. On the fourth day, after Se introduction into nutrient solution, Se content in pea seedlings was determined. Method of Se content determination and results were published in our earlier work (Łukaszewicz et al. Citation2018). A part of results necessary for this research is cited in .
Table I. Selenium content in shoots of pea seedlings pre-treated with Se in forms of sodium selenite and selenate (Łukaszewicz et al. Citation2018)
Aphids
The pea aphids (Acyrthosiphon pisum Harris) were obtained from the aphid stock culture kept at the Department of Plant Physiology, Poznań University of Life Sciences. The aphids colony was maintained in climatic chamber on pea plants with following conditions: under luminescent light with photon flux density 130–150 μmol m−2 s−1 (Philips lamps), a 14/10 h light/dark photoperiod, at 22–23°C and 65–70% relative humidity. Adult apterous females were applied on pea seedlings (20 insects per plant), pre-treated for 4 d with sodium selenite or sodium selenate. Control consisted of aphids uncolonized plants untreated with Se compounds.
Methods
Aphids probing behaviour
Acyrthosiphon pisum probing behaviour was observed using the Electrical Penetration Graph technique (EPG) (Tjallingii Citation1985, Citation1988) that is commonly applied in Hemiptera-plant relationship studies (Tjallingii Citation1995; Dancewicz et al. Citation2008, Citation2016, Citation2018, Citation2020; Gabryś et al. Citation2015). In this experimental setup, aphid and plant are made parts of an electric circuit, which is completed when the aphid inserts its stylets into the plant. Weak voltage is supplied in the circuit, and all changing electric properties are recorded as EPG waveforms that can be correlated with aphid activities and stylet position in plant tissues (Tjallingii Citation1994, Citation2001). In our study, probing behaviour of pea aphid was monitored 4 d after the administration of Se. Apterous adults of A. pisum were collected from the stock colony and dorsally tethered on the abdomen with a gold wire electrode (1.5 − 2.0 cm long, 20 μm diameter) and water-based conductive silver paint (EPG-Systems; Dillenburg 12 6703 CJ Wageningen, The Nederlands; www.epgsystems.eu). After attachment of the wire electrode, aphids were starved for 1 h prior to the experiment. The plant electrode (copper wire) was inserted in the test tube containing the plant not treated Se (control) or pre-treated with Se in form of sodium selenite or sodium selenate, at concentrations of 10 and 20 µM. Probing behaviour of 16 apterous females per studied plant/aphid combination was monitored for 8 h continuously with two the eight-channel DC EPG recording equipment at the same time. All experiments were started at 10˗11 a.m. During EPG recordings, aphids were in a Faraday cage in the laboratory (21 ± 1°C, 65% R.H., and 16:8 (L/D) photoperiod). The electrical signals were saved and analysed using the PROBE 3.1 software provided by W.F. Tjallingii (EPG-Systems; Dillenburg 12 6703 CJ Wageningen, The Nederlands). The incomplete (i.e., shorter than 8 h) recordings were eliminated from analysis. Various behavioural phases were labelled manually using the Stylet + software. The following EPG patterns were distinguished: np – non-probing (aphid stylets outside the plant), C − pathway (probing in non-vascular tissues) G – xylem phase (xylem sap ingestion), E – phloem phase (divided into E1 – watery salivation into sieve elements and E2 − ingestion of phloem sap) (Tjallingii Citation1985, Citation1994; Prado & Tjallingii Citation1994). The duration and frequency of individual patterns could be associated with various aspects of plant suitability for aphid feeding (Mayoral et al. Citation1996). A number of sequential (i.e., describing the sequence of events during the recording) and non-sequential (i.e., referring to frequency, total, and average duration of patterns) parameters were calculated (van Helden & Tjallingii Citation1993; Woźniak et al. Citation2019). All EPG parameters describing aphid probing and feeding behaviors were calculated manually and individually for every aphid using the EPG analysis Excel worksheet created for this study. Afterwards, the mean and standard errors were determined. Aphid behavior on pea seedlings pre-treated with sodium selenite or sodium selenate at concentrations of 10 and 20 µM were separately compared to aphid behavior on control plants.
Demographic parameters
One hundred, 1-d-old nymphs were used for the experiment. Then apterous females 1 d after reaching maturity were placed separately and free on pea seedlings (25 insects per plants). The duration of the period of pre-reproduction, reproduction, post-reproduction, total life span, and fecundity of aphids were recorded. Observations were made daily. In order to calculate the total fertility of females, newly born nymphs were counted and removed. For each variant, the percentage of mortality of larvae was determined.
Demographic data was determined according to the methods described by Birch (Citation1948). The influence of Se on the demographic parameters of the aphid population, i.e. the net reproduction rates (Ro), mean generation time (T), intrinsic rate of increase (rm), finite rate of increase (λ), time necessary for the population to double (DT), were calculated. The intrinsic growth rate (rm) was calculated with Watt and White (Citation1977) formula: rm = 0.738 [(lnMd)/d], where d is the developmental period from birth to the beginning of the first reproduction and Md is the number of nymphs born in the period from time d. The net reproduction rates Ro = ∑(mx lx) – where lx and mx were cumulative daily survival and fertility, respectively; mean generation time T = lnR o/rm; the finite rate of increase λ = erm and doubling time DT = ln2/rm.
Enzyme activities in aphids
The activities of antioxidant [superoxide dismutase (SOD, EC 1.15.1.1.) and catalase (CAT, EC 1.11.1.6)], detoxifying [S-glutathione transferase (GST, EC 2.5.1.18) and β-glucosidase (β-GLU, EC 3.2.1.21)] and redox [peroxidase (POX, EC 1.11.1.7) and polyphenol oxidase (PPO, EC 1.10.3.1)] enzymes in the tissues of A. pisum were analysed. Twenty apterous female aphids per plant were applied to one-week-old pea seedlings pre-treated with sodium selenite or sodium selenate at the concentration of 10 and 20 μM. The aphids feeding on plants grown on nutrient solution without Se were used as a control. Adult aphids were collected for analysis (0 h) and after 24, 48, and 72 h. All of the aphids collected during the experiment were treated with liquid nitrogen and stored at −20°C until the analysis of enzymatic activities. Thirty collected aphids were homogenized for 2 min in 0.1 M phosphate buffer (pH 7.0) at 0°C. The homogenate was centrifuged (Eppendorf Centrifuge 5810 R) for 4 min at 9000 × g at 4°C. The supernatant was used for further determination of enzymatic activities, as described below. The enzymatic assays were carried out in three replications. One replicate consisted of 30 aphids.
Superoxide dismutase (SOD)
Activity of SOD was determined with Wang et al. (Citation2001) method in which superoxide anion radical reacts with nitroblue tetrazolium (NBT). Xantine and xanthine oxidase were used to generate superoxide anion. The reaction mixture consisted of 1.0 mL of 0.2 M phosphate buffer (pH 7.8), 0.1 mL of crude aphid extract and 0.1 mL of 0.25 mM NBT in 0.2 M phosphate buffer (pH 7.8), containing 0.25 mM of xanthine. The reaction started after the addition of 0.1 mL of the xanthine oxidase and was conducted at 25°C for 20 min. Absorbance at 560 nm was measured using a TECAN Infinite 200 microplate reader at 560 nm. One unit of activity was defined as the amount of enzyme necessary to inhibit the rate of superoxide anion production by 50%.
Catalase (CAT)
Activity of CAT was measured with the method described by Aebi (Citation1984) with slight modification. To 0.25 mL of crude aphid extract 0.25 mL of 30 mM H2O2 was added. The disappearance of H2O2 was measured using Carry 50 spectrophotometer at 240 nm during 1 min.
β-Glucosidase
β-Glucosidase activity was determined by a reaction in which this enzyme hydrolyses the substrate p-nitrophenyl-β-D-glucopyranoside using the method described by Katagiri (Citation1979). The reaction mixture consisting of 0.2 mL substrate (p-nitrophenyl-β-D-glucopyranoside), 0.1 mL of 0.2 M phosphate buffer (pH 5.8) and 0.2 mL of crude aphid extract was incubated for 30 min at 25°C. Then, 3 mL of freshly prepared 2% Na2CO3 was added to the mixture. The absorbance was measured using a TECAN Infinite 200 microplate reader at 400 nm.
Glutathione S-transferase assay (GST)
Activity of GST was determined by the reaction of conjugation of 1-chloro-2,4-dinitrobenzene (CDNB) with reduced glutathione (GSH) (Leszczyński & Dixon Citation1992). The reaction mixture consisting of 0.5 mL of crude aphid extract and 0.68 ml of 20 mM GSH in 0.2 M phosphate buffer (pH 6.5) was incubated for 10 min at 30°C. Then, 0.02 mL of 100 mM CDNB was added and the mixture was incubated for 5 min at 30°C. The increase of absorbance was measured using a TECAN Infinite 200 microplate reader at 340 nm.
Polyphenol oxidase (PPO)
PPO activity was determined with the use of the previously described method according to Miles (Citation1964) with modification by Laurema et al. (Citation1985). The reaction mixture contained 0.5 mL of 0.2 M phosphate buffer with pH 7.4, 0.25 mL of crude homogenate of aphids and 0.25 mL of 10 mM catechol solution. After 1 h at 30°C, changes in the absorbance of the reaction mixture were measured at 460 nm against a blank containing 0.25 mL of 0.2 M phosphate buffer (pH 7.4) instead of the substrate. The absorbance was measured using a TECAN Infinite 200 microplate reader at 460 nm.
Peroxidase (POD)
Activity of POD was determined using the Fehrmann and Dimond (Citation1969) method. The reaction mixture consisted of 0.1 mL of crude homogenate of aphids, 0.1 mL of 0.2 M pyrogallol, 0.5 mL of 0.1 M phosphate buffer (pH 7.0), 0.35 mL distilled water, and 0.05 mL of 3% H2O2. The mixture was incubated at 30°C for 25 min, and the reaction was stopped by the addition of 0.25 mL of 25% trichloroacetic acid solution. Absorbance was measured at 430 nm against a blank containing 0.1 mL of 0.1 M phosphate buffer (pH 7.0) instead of pyrogallol. TECAN Infinite 200 microplate reader at 430 nm was used for this measurement.
Protein
The concentration of protein within the tissues of aphids was determined using the method of Lowry et al. (Citation1951) and was used to express the activities of enzymes.
Analysis of plant material
Flavonols
Flavonol concentration was determined according to the method of Day (Citation1993). Samples of 0.5 g leaves were homogenized in the extraction mixture containing methanol, hydrochloric acid, and distilled water in the ratio 90:1:1 (v/v/v), stirred and incubated for 10 min at 60°C. Then samples were centrifuged for 20 min at 17,000 × g. Absorbance was measured at 254 nm (Jasco V-530 UV/VIS Spectrophotometer). The flavonol content in the sample was read from the curve prepared for quercetin and calculated per gram of dry weight (Stefova et al. Citation2001).
Carotenoids
A simple method without maceration was used for the extraction of carotenoids from the leaf (Hiscox & Israelstam Citation1979). To 0.1 g of leaves, 10 mL dimethyl sulfoxide (DMSO) was added. Samples were incubated at 65°C for 1 h. After cooling down the absorbance was measured at 480 nm, 469 nm, and 665 nm. Concentration of carotenoids was calculated using modified Arnon’s formula (Wellburn Citation1994) and calculated per gram of dry weight.
Statistical analysis
All determinations were performed in three replicates from three independent experiments. The results of flavonol and carotenoid concentrations in pea leaves, the activities of enzymes in the aphid tissues of A. pisum were subjected to statistical analysis using three-way analyses of variance (ANOVA) while the lifetable parameters of A. pisum were subjected to two-factor analyses. Means were compared using Duncan test at the significance level α ≤ 0.05. While EPG results were analyzed statistically using a non-parametric Kruskal-Wallis H test followed by post-hoc Dunn’s multiple comparison test. All calculations were performed using StatSoft, Inc. STATISTICA (data analysis software system), version 12, www.statsoft.com and the non-transformed data.
Results
Behavioral responses of A. pisum during probing in plant tissues
The electronic registration (EPG) of the influence of A. pisum probing and feeding behaviours on the control and Se-pretreated pea seedlings revealed all kinds of aphid activities related to plant penetration: non-probing, pathway activities in peripheral tissues “C” (including the short punctures of encountered cells “pd”), xylem sap uptake “G” and penetration of phloem tissue, i.e. phloem watery salivation ‘E1ʹ and phloem sap ingestion ‘E2ʹ ().
The pre-treatment with sodium selenite or sodium selenate at 10 and 20 µM concentrations of pea seedlings evoked a wide spectrum of changes in A. pisum probing and feeding behaviours. The typical behavior of A. pisum on untreated plants consisted mainly of activities associated with phloem phase – 78% of the experimental time (). All aphids started probing immediately after being given access to the plants at the beginning of the experiment. Generally, non-probing and pathway activities predominated during the initial 1 h of the experiment, later the proportion of non-probing and non-phloem probing phases decreased, while phloem phase was the main aphid activity on these plants (ranged from 74% to 93% for particular hours of registration) (). In contrast, on Se pre-treated pea seedlings, aphid activities associated with phloem activities were reduced and occupied 56% (20 µM selenite), 59% (10 µM selenate) and 52% (20 µM selenate) of the 8-h recording period. Moreover, non-probing time (20 µM selenate – 9%) and time of non-phloem penetration (20 µM selenite – 36%; 10 µM selenate – 33%; and 20 µM selenate – 39%) were significantly longer compared to 4% and 18% of all experimental time for control plants, respectively (). However, these phases predominated only during the initial 1–2 h of the EPG experiment on Se pre-treated plants, irrespective of Se concentration. From 3rd h of the experiment the proportion of phloem phase increased gradually to reach approximately 72% (10 µM selenite and 10 µM selenate) of all activities, 8 h after aphids got access to the plants. On the seedlings exposed to 20 µM selenite or 20 µM selenate, the proportion of phloem phase increased gradually between 3rd and 6th h of the experiment and later decreased, and occupied 50% of all activities in 8-h registration ().
Although aphid stylet penetration was significantly inhibited only on 20 µM selenate pre-treated plants, the mean duration of probing was 2.8 (10 µM selenite) and 3.5 (20 µM selenate) times shorter than on control plants. Furthermore, in both cases aphid probing was frequently interrupted; there were (2 times) more probes per aphid during the 8-h experiment. Aphids on plants exposed to 20 µM selenite, 10 µM and 20 µM selenate penetrated peripheral tissues (non-vascular – epidermis and mesophyll) two times longer, whereas they penetrated phloem tissue shorter (2.2, 1.5 and 2 h, respectively) compared to aphids on control plants. Likewise, the proportion of phloem phase in total probing was reduced by 21% (20 µM selenite), 17% (10 µM selenite) and 24% (20 µM selenate) in comparison to control plants (). However, the number of probes with the phloem phase was higher than in the control only in the case of aphids registered on seedlings pre-treated with sodium selenite at the concentration of 20 µM. The prior application of 20 µM selenite and 20 µM selenate caused a two-time increase in the total number of phloem phases per aphid in relation to control. Moreover, the number of sustained sap ingestion phases (E2 > 10 min) was higher than in control for aphids on pea seedlings pre-treated with 20 µM selenate. These outcomes demonstrate that in these cases the presence of Se interrupted and shortened the time of phloem phase and sustained the phloem sap ingestion phase ().
Figure 1. Temporal evolution of the probing behavior of Acyrthosiphon pisum on Pisum sativum seedlings pre-treated with sodium selenite or sodium selenate at concentrations of 10 and 20 µM, expressed as the proportion of time spent on different stylet activities during 8-h EPG recording: np, non-probing; C + G, probing in non-phloem tissues (probing in non-vascular tissues + xylem sap uptake); E1+ E2, total phloem phase (watery salivation + sap ingestion)
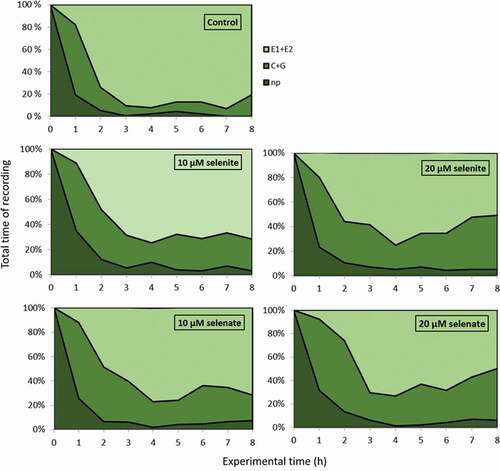
Figure 2. Probing and feeding behaviour of Acyrthosiphon pisum on Pisum sativum seedlings pre-treated with sodium selenite or sodium selenate at concentrations of 10 and 20 µM, shown as percentage of stylet activities during the 8-h experiment: np – non-probing; C + G – activities in non-phloem tissues (probing in non-vascular tissues + xylem sap uptake); E1 + E2 – phloem activities (watery salivation and sap ingestion). Different letters in the selected phase indicate statistically significant differences in relation to control and different concentrations of sodium selenite or sodium selenate at p < 0.05 (Kruskal- Wallis test followed by post-hoc Dunn’s multiple comparison test, p < 0.05)
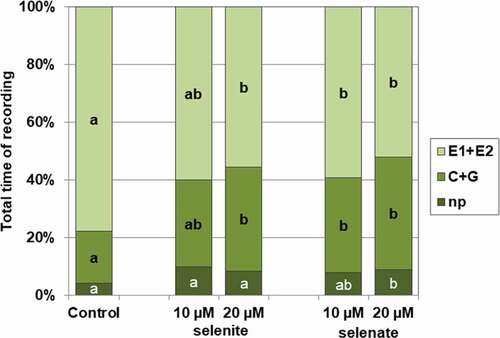
Figure 3. Mean number of activities of Acyrthosiphon pisum on Pisum sativum seedlings pre-treated with sodium selenite or sodium selenate at concentrations of 10 and 20 µM, recorded during the 8-h EPG experiment. Different letters indicate statistically significant differences in relation to control according to the Kruskal-Wallis test followed by multiple comparison (p < 0.05)
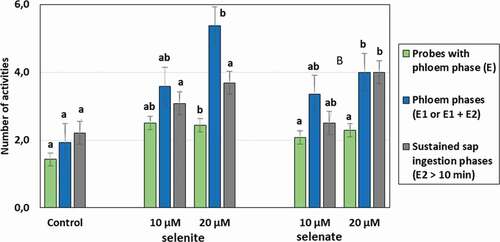
Table II. Probing activities (EPG parameters) of Acyrthosiphon pisum on Pisum sativum seedlings pre-treated with sodium selenite or sodium selenate at concentrations of 10 and 20 µM during 8-h EPG experiment
There were clear differences in probing behaviour of A. pisum before the first phloem phase on 10 µM selenite and 20 µM selenate pre-treated pea seedlings in relation to control plants, although the treatments did not affect the time needed for reaching phloem vessels. On plants exposed to sodium selenite (10 µM) or sodium selenate (20 µM), aphid probes that preceded the first phloem phase were numerous (2 or 2.3 times as many as on control plants, respectively) and were mainly of epidermal character, i.e. less than 3 min long (accounted for 89% − 10 µM selenite or 83% − 20 µM selenate of all probes before the first occurrence of phloem phase) ().
Statistically significant differences occurred in aphid activities in sieve elements. On 20 µM selenite pre-treated plants the duration of the first phloem phase was the shortest, nearly 3 h shorter than on control plants. Additionally, total duration of the phloem sap ingestion phase for aphids feeding on Se pre-treated plants was shorter by 1.8 h (20 µM selenite), 1.5 h (10 µM selenate) and 2.1 h (20 µM selenate) than for aphids on the control seedlings (). The mean duration of an individual period of phloem sap ingestion was significantly shorter on 10 µM sodium selenite and 20 µM sodium selenate pre-treated plants (2.5 and 3.4 times shorter in relation to the control, respectively). However, the phloem phase consisted mainly of passive sap ingestion activity for aphids from all variants with selenium, because the contribution of watery salivation into sieve elements to the phloem phase was relatively low (i.e. minimum 2.2% and maximum 4.5% of all activities in sieve elements) and similar to the control variant (1.8% of all activities in sieve elements).
Foliar concentrations of flavonoids and carotenoids in Pisum sativum
The concentration of flavonols in control seedlings not treated with Se and not colonized by aphids did not change significantly during the experiment (). Pre-treatment with Se caused changes in flavonol levels in pea leaves. In the seedlings pre-treated with selenite, the synthesis of these compounds was stimulated (). Their concentration was on average 34.5% higher in seedlings pre-treated with 10 µM selenite and 62.2% in those pre-treated with 20 µM selenite, compared to the control plants untreated with Se. Seedling colonization by aphids increased the concentration of flavonols only in seedlings pre-treated with 20 µM selenite in 24 and 48 h. The concentration of these compounds was higher in these seedlings by 14.8% on average, compared to those uncolonized by aphids and pre-treated with 20 µM selenite. In contrast, pre-treatment of seedlings with 20 µM selenate resulted in an average decrease of 33.9% in flavonol concentration for all time points, in comparison to control plants untreated with Se (). There were no significant changes in flavonol concentration as a result of aphid colonization.
Figure 4. Flavonol concentration in leaves of Pisum sativum seedlings pre-treated with sodium selenite (A) or sodium selenate (B) and colonized by Acyrthosiphon pisum. Statistical analyses three-way ANOVA, Duncan’s test was made for A and B individually. Different letters mean statistical differences at p < 0.05 level
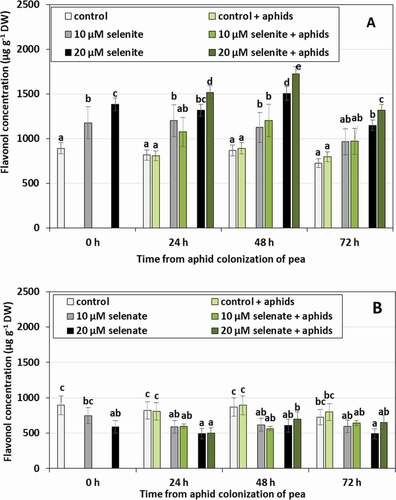
The concentration of carotenoids in control seedlings untreated with Se and uninhabited by aphids did not change significantly during the experiment (). Pre-treatment of pea seedlings with Se in the form of selenite resulted in an increase in the concentration of carotenoids ()). Under the influence of 10 µM selenite, the concentration of these compounds increased by 2.6 times, and under the influence of 20 µM selenite 3.1 times. However, no changes in the concentration of carotenoids as an effect of the colonization by aphids were found. Unlike selenite, selenate had no effect on changes in carotenoid concentration. The only significant change was the synergistic effect of selenate at the concentration of 10 µM and aphid feeding in 24 h, which increased the concentration of carotenoids by 63.9%, compared to control untreated with Se and not colonized by aphids.
Figure 5. Carotenoid concentration in leaves of Pisum sativum seedlings pre-treated with sodium selenite (A) or sodium selenate (B) and colonized by Acyrthosiphon pisum. Statistical analyses three-way ANOVA, Duncan’s test was made for A and B individually. Different letters mean statistical differences at p < 0.05 level
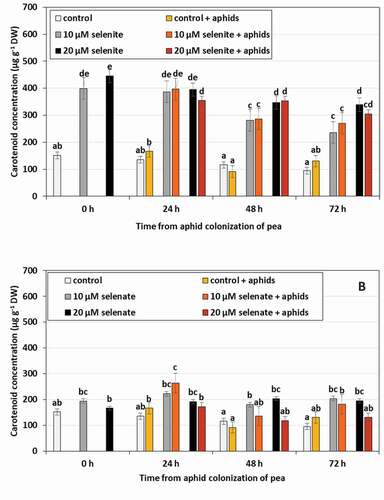
Activities of enzymes in aphid tissues
The feeding of aphids on pea seedlings resulted in an increase in the activity of superoxide dismutase (SOD) in their bodies (). After 24 and 48 h in aphids feeding on control seedlings, not treated with Se, there was an increase in the activity of the examined enzyme on average by 111.3% in comparison to the activity at 0 h. Elevated concentration of Se in pea seedlings caused the increase of the SOD activity in the aphids. In the case of pre-treatment of seedlings with 20 μM selenite, the activity of the examined enzyme in the aphids in 24 and 48 h of feeding was significantly higher on average by 50.7% than in the control. However, pre-treatment of seedlings with Se at a concentration of 10 μM selenite resulted in an increase in SOD activity more than twice at the same time, and by 70.1% at the concentration of 20 μM in comparison to the control. After 72 h of feeding, a decrease in the activity of the tested enzyme was found in all variants, but it was still significantly higher in aphids feeding on seedlings pre-treated with Se than on those not treated.
Figure 6. Superoxide dismutase (SOD) activity in the tissues of Acyrthosiphon pisum feeding on Pisum sativum seedlings pre-treated with sodium selenite and sodium selenite. Statistical analyses – three-way ANOVA, Duncan’s test. Different letters mean statistical differences at p < 0.05 level
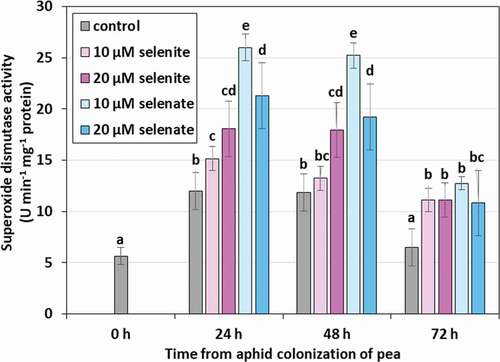
Catalase (CAT) activity in aphids feeding on seedlings not treated with Se remained throughout the duration of the experiment at a relatively low, constant level (). Feeding on selenium pre-treated seedlings significantly increased the activity of the tested enzyme especially at the concentration of 20 μM in 24, 48 and 72 h, on average more than twice as high as in the control. However, in aphids feeding on pre-treated selenium seedlings at both concentrations, CAT activity significantly increased in 24 h, on average more than four times compared to the control. However, the highest activity of the tested enzyme was found after 48 h in aphids feeding on seedlings pre-treated with 20 μM selenate and it was over nine times higher than in the aphids from control seedlings. In 72 h there was a decrease in CAT activity, but in aphids feeding on seedlings pre-treated with Se, it was still significantly higher than in the control.
Figure 7. Catalase (CAT) activity in the tissues of Acyrthosiphon pisum feeding on Pisum sativum seedlings pre-treated with sodium selenite and sodium selenate. Statistical analyses –three-way ANOVA, Duncan’s test. Different letters mean statistical differences at p < 0.05 level
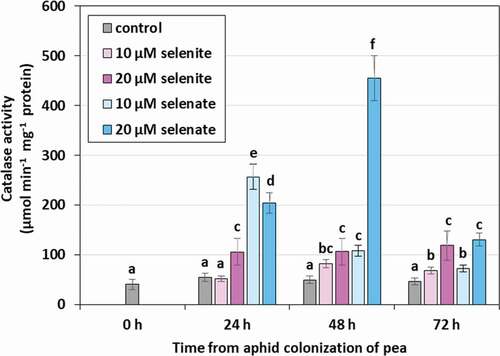
Aphid feeding on both control and pre-treated seedlings increased the β-glucosidase activity (). The activity of the studied enzyme in aphid feeding on control seedlings in 24, 48 and 72 h was on average 39.1% higher than in the 0 h period. Compared to the control, feeding on Se pre-treated seedlings increased the β-glucosidase activity by 12.9% on average, while on those pre-treated with selenate there was an average of 26.2%.
Figure 8. β-Glucosidase activity in the tissues of Acyrthosiphon pisum feeding on Pisum sativum seedlings pre-treated with sodium selenite and sodium selenate. Statistical analyses –three-way ANOVA, Duncan’s test. Different letters mean statistical differences at p < 0.05 level
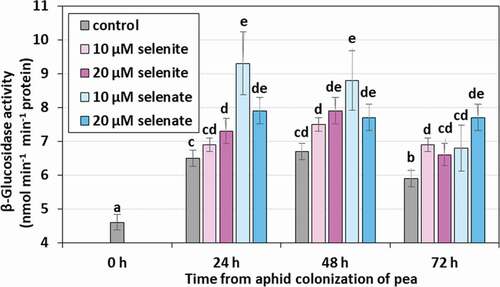
Like in the case of β-glucosidase, glutathione S-transferase (GST) activity increased as a result of aphid feeding on control seedlings, untreated with Se, as well as on pre-treated seedlings (). A significant increase in the activity of the tested enzyme by an average of 17.3%, compared to the 0 h, was found in the tissues of aphids feeding on control seedlings in 48 and 72 h of feeding. In contrast, stimulation of GST activity as a result of Se treatment of seedlings in both forms and concentrations was observed in 24 and 48 h, and for selenate in the concentration of 20 μM also in 72 h (on average by 19.7% in relation to control).
Figure 9. Glutathione S-transferase (GST) activity in the tissues of Acyrthosiphon pisum feeding on Pisum sativum seedlings pre-treated with sodium selenite and sodium selenate. Statistical analyses – three-way ANOVA, Duncan’s test. Different letters mean statistical differences at p < 0.05 level
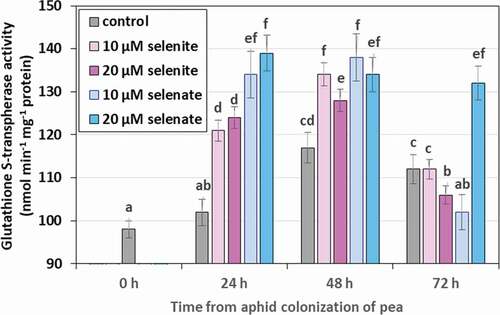
Peroxidase (POX) activity also increased in the tissues of aphids feeding on pea seedlings (). In the case of feeding on control seedlings, not treated with Se, the significant increase in the activity of the tested enzyme was observed in 24, 48, and 72 h compared to the 0 h, and it amounted to an average of 33.3%. However, only in the case of selenate treated seedlings in both concentrations in 48 and 72 h of aphid feeding, a significant increase in POX activity was observed and it was on average higher than in the control by 20.7%.
Figure 10. Peroxidase (POX) activity in the tissues of Acyrthosiphon pisum feeding on Pisum sativum seedlings pre-treated with sodium selenite and sodium selenate. Statistical analyses – three-way ANOVA, Duncan’s test. Different letters mean statistical differences at p < 0.05 level
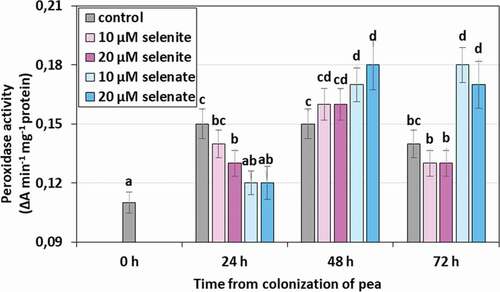
Activity of polyphenol oxidase (PPO) in aphids feeding on control seedlings, Se untreated, remained stable, except for 48 h, where a slight increase was noted compared to 0 h (). A significant increase in the activity of the studied enzyme compared to the control was found as a result of feeding aphids on seedlings, except for those treated with 10 µM selenite. The strongest effect of Se is manifested by the influence of 20 µM of selenite and 10 µM of selenate, which increased the PPO activity on average in 24, 48 and 72 h by 72.5% and 95.6%, respectively.
Demographic parameters
The presence of Se in pea tissues inhabited by aphids was of great importance in the development of A. pisum (, ). The addition of Se to the nutrient solution in which the plants grew significantly influenced the duration of individual developmental phases and insect fecundity (, ). The length of the pre-reproductive period lasted 7 to 10 d and was dependent on both the concentration and form of Se (). The longest pre-reproductive period was found for A. pisum feeding on plants treated with 20 μM selenite and 10 μM selenate. In relation to the total life expectancy of the aphids, this period accounted for 59% and 75%, respectively. The addition of 20 μM selenate to the medium resulted in 11 d of total mortality of larvae.
Table III. Effect of pre-treatment of Pisum sativum seedlings with selenium (Se) in form sodium selenite or sodium selenate on lifetable parameters of Acyrthosiphon pisum (two-way ANOVA, Duncan’s test, p < 0.05) (n = 25)
Table IV. Mean developmental time, life length, total fecundity and rate of development of Acyrthosiphon pisum on Pisum sativum seedlings pre-treated with sodium selenite or sodium selenate (n = 25)
The presence of Se in plants where aphids fed on, shortened the duration of the reproductive period. The most significant differences with respect to control were noted at 20 μM selenite and 10 μM selenate. These aphids gave birth to larvae for only 3 to 5 d, and the duration of this period constituted less than 30% of their total life. Selenium did not significantly affect the duration of the post-reproductive period. However, the addition of selenate to the nutrient solution and 20 μM selenite caused a significant reduction in the life of aphids.
Selenium significantly reduced the fecundity of A. pisum (, ). Treatment of plants with 20 μM selenite reduced the fecundity of aphids by 90% and 10 μM sodium selenate by as much as 95% compared to the control. The highest daily fecundity of aphids which inhabited the control plants were 10 larvae per female on the 5th d of reproduction (). In contrast, the addition of Se to the nutrient solution significantly reduced the daily number of larvae born by aphids.
Figure 12. Average daily fecundity of Acyrthosiphon pisum females feeding on Pisum sativum seedlings pre-treated sodium selenite or sodium selenate
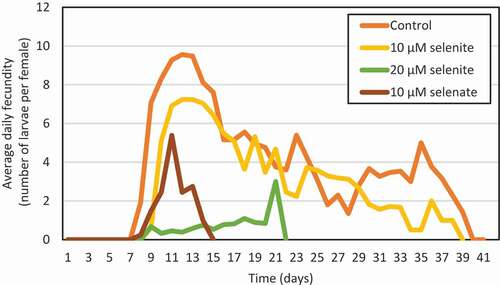
Calculated demographic indices of the population indicated the toxic effects of selenium on A. pisum (). Selenate and 20 μM selenite, as compared to controls, had an effect on the reduction of intrinsic rate of increase (rm), net reproductive rate (Ro), finite rate of increase (λ) and shortening of the generation time (T).
Discussion
The basis of plant survival in the natural environment is the adaptation of physiological processes and metabolism to changing environmental factors and its response to biotic and abiotic stress (Foyer et al. Citation2016). It has been shown that plant exposure to one stress can affect the intensity of response to another stress factor. This phenomenon is called cross-tolerance. There are relatively few signaling pathways specific for a given stress factor and plants use common signaling pathways for biotic and abiotic stress, thanks to which exposure to one stress factor results in increased resistance to other stressors (Foyer et al. Citation2016).
The main purpose of the research presented in this paper was to check how stress caused by the treatment of pea seedlings with Se compounds (the first stress factor) would affect the feeding of pea aphid (the second stress factor). The obtained research results show the effect of pea treatment with Se on feeding and demographic parameters of the pea aphid population and on the activity of antioxidant, detoxifying, and redox enzymes in the tissues of this phytophage, as well as on the induction of flavonol and carotenoid synthesis in pea plants.
The comparative analysis of EPG data exposed clear differences in crucial aspects of probing behavior of A. pisum on Se pre-treated plants, depending on the form and concentration of Se. The most distinctive modification of aphid behavior during probing was observed on plants treated with sodium selenite at the concentration of 20 µM or sodium selenate at the concentration of 10 µM and 20 µM.
In all experimental cases, the total duration of stylet penetration in non-vascular plant tissues during the 8-h EPG recording was elongated, but the duration of phloem penetration and phloem sap ingestion were reduced in relation to control plants. Krzyżanowski et al. (Citation2018) showed an extension of the total time of stylet penetration in non-vascular plant tissues of bird cherry-oat aphid (Rhopalosiphum padi (L.)) on winter wheat seeds (blank) after chlorophenoxy herbicides treatment. The mechanism of resistance of plant varieties to aphids is expressed in the reduction of time spent on ingestion of phloem sap (Sauge et al. Citation1998; Kordan et al. Citation2008). Besides, the presence of deterrent allelochemicals in the phloem sap results in the suppressing or termination of feeding (Nisbet et al. Citation1993; Dancewicz et al. Citation2008, Citation2016; Grudniewska et al. Citation2013; Gabryś et al. Citation2015). Treatment of plants with Se shortened the total duration of phloem sap ingestion in aphids compared to controls. Research by Goławska and Łukasik (Citation2012) showed that higher concentrations of luteolin and genistein reduced or completely inhibited aphid salivation and passive ingestion. The decrease of ingestion by aphids indicated the deterrent effect of a given compound on aphids (Goławska & Łukasik Citation2012; Halarewicz & Gabryś Citation2012; Dancewicz et al. Citation2020). Our studies have shown that the mean duration of probing is shorter in aphids preying on seedlings treated with 10 µM selenite and 20 µM selenate. The studies of Dancewicz et al. (Citation2020) on Myzus persicae (Sulzer) showed the short mean duration of probing, prolonged salivation and interruption of feeding in the case of artificial diets containing cyclotides. Moreover, the phloem phases and the sustained sap ingestion phases (E2 > 10 min) were more numerous and the proportion of phloem phase in aphid activities decreased in the course of time. The outcomes demonstrate that in these cases the presence of Se interrupted and shortened the time of the phloem phase and sustained the phloem sap ingestion phase. This may in turn delay the acceptance of phloem sap by A. pisum, since the ingestion of the phloem sap for longer than 10 min reflects the acceptance of the plant by the aphid (Tjallingii & Mayoral Citation1992).
Literature reports indicate that Se may affect insect development and limit reproduction. Studies on the beet armyworm (Spodoptera exigua (Hübner)) feeding on Atriplex have shown that high doses of Se reduce pupation and can also cause insect death in the early larval stages (Vickerman et al. Citation2002). In addition, it has been shown to reduce the survival of larvae by 70–75% of insects feeding on plants containing Se (Vickerman & Trumble Citation1999; Mechora Citation2019). Trumble et al. (Citation1998) showed that the addition of Se caused an extension of 22–30% of the time needed for the development of the aphid, from laying eggs until reaching puberty. Our research showed that treating pea plants with Se compounds had an effect on pea aphid development and determined the duration of individual developmental phases and insect fertility. This reaction depended on both the Se form and the concentration used. Selenium in the form of selenate at the concentration of 20 μM caused complete mortality of the larvae after 11 d of the experiment. In addition, 20 µM selenite and 10 µM selenate caused the extension of the pre-reproduction period and shortened the reproduction period in aphids. According to Obopile and Ositile (Citation2010), the length of the pre-reproduction period is associated with the resistance or sensitivity of plants to aphid feeding and is longer in resistant plants.
We also found that the presence of Se in plants inhabited by aphids significantly reduced female fertility. Se toxicity to aphids is confirmed by demographic indicators of the aphid population. Se in both forms at a concentration of 20 µM reduced intrinsic rate of increase (rm), net reproduction rate (Ro), finite growth rate (λ), and reduced the generation time (T). The decrease in rm relative to the variant without the addition of Se indicates that Se treatment of plants colonized by aphids reduced the reproductive potential of the insect. In the opinion of Obopile and Ositile (Citation2010), low values of this index prove antibiosis or antixenosis resulting in reduced survival and reproduction of aphids. Similar relationships were observed on plants with increased resistance to feeding by cowpea aphid (Laamari et al. Citation2008). In addition, lower Ro values in variants with 20 µM selenite and 10 µM selenate indicate that Se reduces the biotic potential of aphids.
Insect feeding has an effect on plant metabolism causing the production of secondary metabolites and triggers signaling pathways related to plant response to biotic stress. Metabolites produced by plants under stress conditions determine the degree of infestation by aphids (Hodges et al. Citation2013; Foyer et al. Citation2016). Flavonoids, including flavonols, play an important role in plant response to stress factors and are also produced by plants in response to insect feeding (Kubo Citation2006; Ateyyat et al. Citation2012; Araj et al. Citation2018). They can act deterrently by discouraging insects from feeding (Dreyer & Jones Citation1981; Al-Antary Ateyyat et al. Citation2018), and they have a toxic effect which disrupts development, reduces fertility and increases insect mortality (Stewart et al. Citation2000; Ruuhola et al. Citation2001). Numerous studies have shown that in higher plants these compounds perform antioxidant functions under stress conditions (Winkel-Shirley Citation2002; Agati et al. Citation2012). It was also found that flavonols accumulate in response to feeding of aphids in plant tissues (Sun et al. Citation2016), which is associated with their antioxidant properties (Cao et al. Citation2013; Anjali et al. Citation2017). Accumulation of flavonoids has been observed, among others, in corn tissues exposed to salinity stress and treated with Se compounds (Gul et al. Citation2017). Also, studies on wheat subjected to low-temperature stress have also showed that treating plants with Se compounds causes an increase in the content of antioxidant compounds, such as anthocyanins, flavonoids, and phenolic compounds (Chu et al. Citation2010).
In the submitted study it was observed that the treatment of pea seedlings with selenite increased the flavonol content, compared to the control. In addition, 24 and 48 h feeding of aphids on plants treated with 20 μM selenite increased the accumulation of flavonols, compared to plants not inhabited by aphids. On the other hand, selenate reduced the flavonol content. This result may suggest that selenite as a stress factor caused the mobilization of plant antioxidant systems. Higher content of flavonols in plants treated with 20 µM selenite could be one of the elements influencing the extension of the total duration of stylet penetration in non-vascular plant tissues and disturbances, shortened time of phloem phase as well as sustained phloem sap ingestion phase (). In studies by Goławska and Łukasik (Citation2012) it was also shown that plant flavonoids such as luteolin and genistein given in artificial diet caused the prolongation of the period of stylet probing in A. pisum and discouraged aphids from feeding. Kozak et al. (Citation2015) examining the bird-cherry aphid Rhopalosiphum padi during the settlement of winter wheat showed that the flavonoids studied by them had a deterrent influence on nymphs and apterous female aphids, with the latter being more sensitive. Deterrent effects of plant flavonoids have also been demonstrated in studies on beet armyworm (Spodoptera exigua) (Cipollini et al. Citation2008) and migratory locust (Locusta migratoria L.) treated with flavonoids isolated from Eucalyptus saligna (Sm.) (Simmonds Citation2003). In our studies, an extension of the pre-reproduction period and a shortening of the reproduction period were observed in aphids feeding on plants treated with 20 µM selenite with a greater accumulation of flavonols (). A reduction in the fertility of A. pisum females was also observed in this combination. The reason for this could be the association of plant flavonoids with the ecdysone hormone receptor, which consequently, could indirectly lead to a reduction in the development and fertility, and to an increase in mortality (Ruuhola et al. Citation2001). Research by Boué and Raina (Citation2003) showed that flavonoids of genistein and biochanine A have an impact on limiting fertility of termite (Coptotermes formosanus (Shiraki)).
Carotenoids, like flavonoids, belong to metabolites synthesized by plants in response to stress (Uarrota et al. Citation2018). Moreover, carotenoid oxidation products can act as signaling molecules under oxidative stress (Ramel et al. Citation2012; Havaux Citation2013; Uarrota et al. Citation2018).
In the presented studies, the influence of aphid colonization and Se treatment on the increase of carotenoids content was found. Similar results were obtained by Heng-Moss et al. (Citation2003) in studies conducted on wheat lines resistant and sensitive to the feeding of Russian wheat aphid (Diuraphis noxia (Mordvilko)). They observed an increase in the content of carotenoids in leaves of wheat resistant to this phytophage.
The plant defense response to damage caused by feeding aphids is the local production of reactive oxygen species (ROS), which are toxic to insects (Giordanengo et al. Citation2010; Łukasik & Goławska Citation2013). Studies on Arabidopsis thaliana ((L.) Heynh.) plants have shown that ROS are involved in early signaling during the feeding of the aphid Brevicoryne brassicae (L.) (Kuśnierczyk et al. Citation2008). At the same time, allelopathic compounds produced by plants can cause ROS generation in herbivores, which causes intestinal damage as a result of lipid peroxidation, which can lead to impaired mineral uptake and a number of other adverse processes (Bi & Felton Citation1995; Łukasik & Goławska Citation2013; Durak et al. Citation2018). Generating ROS in insect tissues can affect their development and reduce fertility (Durak et al. Citation2018).
Herbivorous insects have an enzymatic system, which consists of antioxidant, detoxifying, and redox enzymes, reducing the level of ROS, thus limiting their adverse effects on their organisms (Łukasik & Goławska Citation2013; Durak et al. Citation2018). The enzymes produced by herbivores are an important element of the host plant – insect interaction.
In aphid tissues feeding on pea seedlings treated with Se compounds an increase in SOD and CAT activity was demonstrated, compared to untreated control plants. The degree of the increase in the activity depended on the form and concentration of Se, as well as, on the breaking time. Studies on aphids inhabiting cereals have shown that the exposure of insects to compounds with allelopathic activity had a strong effect on the increase in SOD and CAT activity in their tissues (Łukasik Citation2007).
Studies on the aphid Cinara tujafilina (del Guercio) have shown that under stress conditions, such as host changes, there is an increase in SOD and CAT activity within the first 24 h of feeding (Durak et al. Citation2018). Changes in the level of antioxidant enzymes as a result of adaptation to different hosts have also been shown in the studies on Rhopalosiphum padi (Łukasik Citation2009) and Myzus persicae (Abdelsalam et al. Citation2016). Moreover, it is suggested that rapid SOD induction may be the initial response of phytophages to plant oxidant allelochemicals (Ahmad Citation1992). Magierowicz et al. (Citation2019) showed an increase in CAT activity in the tissues of Acrobasis advenella (Zinck.) larvae feeding on plants treated with essential oil from Satureja hortensis (L.) containing carvacrol – a compound with documented insecticidal and acaricidal activity.
Peroxidase (POX) and polyphenol oxidase (PPO) catalyzing redox reactions, are involved in the transformation of insect repellents, such as phenolic secondary metabolites into less toxic products (Urbańska et al. Citation1998; Cai et al. Citation2009; Durak et al. Citation2018). In addition, PPO catalyzes the hydroxylation of monophenols and their oxidation to quinones, which then polymerize into non-toxic melanin dyes (González-Santoyo & Córdoba-Aguilar Citation2012; Magierowicz et al. Citation2019). POX is involved in the oxidation of phenols and other aromatic compounds with hydrogen peroxide, thereby neutralizing their harmful effects (Deimann et al. Citation1991; Durak et al. Citation2018; Magierowicz et al. Citation2019). POX and PPO in the intestines of aphids also play a significant role in the digestive process (Urbańska et al. Citation2002; Chrzanowski et al. Citation2012). In the conducted studies, changes in the activity of oxidoreductases (PPO, POX) were observed in aphid tissues feeding on pea plants treated with Se compounds. After the beginning of the feeding, the activity of POX in aphid tissues increased significantly in all combinations. However, a significant increase in the activity of the tested enzyme compared to the control, took place only after 72 h in tissues of aphids from selenate pre-treated plants.
An increase in PPO activity compared to the control was found only in aphids feeding on plants treated with 10 μM selenate at 24 and 48 h. Durak et al. (Citation2018) observed an increase in PPO activity in Cinara tujafilina tissues 24 h after changing the host plant. Chrzanowski et al. (Citation2012) showed an increase in PPO and POX activity in the tissues of Sitobion avenae treated with insecticidal substances.
Detoxifying enzymes – glutathione S-transferase (GST) and β-glucosidase, play an important role in the response to oxidative stress and in insects’ detoxification processes in response to plant xenobiotics (Yu Citation1996; Durak et al. Citation2018). β-Glucosidase is involved in the hydrolysis of plant glycosides and also plays an important role in the osmoregulation in insect tissues (Magierowicz et al. Citation2019). GST has a significant contribution to insects’ resistance to insecticides and to allelopathic compounds. High activity of this enzyme was found in insects resistant to insecticide preparations (Yu Citation1996; Francis et al. Citation2005).
In the presented studies, changes in the activity of GST detoxification enzymes and β-glucosidase in aphid tissues were observed. The beginning of feeding caused the increase in the activities of β-glucosidase and glutathione S-transferase in the aphids, both from control and plants treated with Se, whereas feeding on Se treated plants increased the activities of tested enzymes even more. Magierowicz et al. (Citation2019) showed an increase in β-glucosidase activity in the tissues of Acrobasis advenella larvae treated with carvacrol. Chrzanowski et al. (Citation2012) showed that treatment of Sitobion avenae aphid with phenolic compounds with biocidal potential increased GST activity. Francis et al. (Citation2005) showed that GST is involved in the defense response of Myzus persicae to secondary metabolites from Brassica plants. Increased activity of this enzyme as a result of the treatment with secondary metabolites was also observed in Sitobion avenae, Rhopalosiphum padi and Lipapis erysimi (Kalt.) (Leszczyński & Dixon Citation1992; Arora et al. Citation2008; Łukasik et al. Citation2015; Durak et al. Citation2018). Durak et al. (Citation2018) suggest that increased GST activity is an indicator either of the adaptation of insects to xenobiotics present in plants with a higher level of resistance, or of the fact that this host is not a natural one for the given insect.
Conclusions
The obtained research results demonstrate that the Se pre-treatment of pea plants modifies the activity of aphids during feeding, as well as it affects demographic population parameters by limiting female fertility and reduces the reproductive potential of the insect. Moreover, Se pre-treatment of plants colonized by aphids increases the activity of antioxidant (SOD, CAT), detoxifying (PPO, POX) and redox (β-glucosidase and glutathione S-transferase) enzymes in the tissues of A. pisum. Both Se pre-treatment and probing and feeding activities of aphids increase the synthesis of flavonols and carotenoids in pea plants.
Disclosure statement
No potential conflict of interest was reported by the authors.
Additional information
Funding
References
- Abdelsalam SA, Awad AMA, Abdelrahman MAA, Nasser MAK, Abdelhamid NMR. 2016. Antioxidant defence response of the green peach aphid Myzus persicae against secondary metabolites of the host plants cumin, anise and coriander. Journal of Agricultural Science and Technology 18(6):1583–1592.
- Aebi H. 1984. Catalase in vitro. Methods in Enzymology 105:121–126. DOI: 10.1016/s0076-6879(84)05016-3.
- Agati G, Azzarello E, Pollastri S, Tattini M. 2012. Flavonoids as antioxidants in plants: Location and functional significance. Plant Science 196:67–76. DOI: 10.1016/j.plantsci.2012.07.014.
- Ahmad S. 1992. Biochemical defence of pro-oxidant plant allelochemicals by herbivorous insects. Biochemical Systematics and Ecology 20:269–296. DOI: 10.1016/0305-1978(92)90040-K.
- Al-Antary Ateyyat TM, Belghasem MA, Alaraj SA. 2018. Aphicidal activity of orange oil to the green peach aphid Sulzer (Homoptera: Aphididae). Fresenius Environmental Bulletin 27:1038–1402. DOI: 10.1016/j.pestbp.2018.01.010.
- Anjali MS, Sridevi G, Prabhakar M, Pushpavathi B, Laxmi NJ. 2017. Dynamic changes in carotenoid and flavonoid content and relative water content (RWC) by corn leaf aphid infestation on sorghum. Journal of Pharmacognosy and Phytochemistry 6(5):1240–1245.
- Araj A, Tawfiq M, Saleh M. 2018. Flavonoids analysis of buckwheat (Fagopyrum esculentum). Fresenius Environmental Bulletin 27(4):2410–2417.
- Arora GK, Rup PJ, Sohal SK. 2008. Studies on enzymatic activity in the nymphs of Lipaphis erysimi (Kalt.) under the influence of coumarin. Allelopathy Journal 22:221–229.
- Ateyyat M, Abu-Romman S, Abu-Darwish M, Ghabeish I. 2012. Impact of flavonoids against woolly apple aphid, Eriosoma lanigerum (Hausmann) and its sole parasitoid, Aphelinus mali (Hald.). Journal of Agricultural Science 4(2):227–236. DOI:10.5539/jas.v4n2p227.
- Bandurska H, Niedziela J, Chadzinikolau T. 2013. Separate and combined responses to water deficit and UV-B radiation. Plant Science 213:98–105. DOI: 10.1016/j.plantsci.2013.09.003.
- Bi JL, Felton GW. 1995. Foliar oxidative stress and insect herbivory: Primary compounds, secondary metabolites, and reactive oxygen species as components of induced resistance. Journal of Chemical Ecology 21(10):1511–1530. DOI:10.1007/BF02035149.
- Birch LC. 1948. The intrinsic rate of natural increase of an insect population. Journal of Animal Ecology 17:15–26. Available: https://www.jstor.org/stable/1605.
- Boué SM, Raina AK. 2003. Effects of plant flavonoids on fecundity, survival, and feeding of the Formosan subterranean termite. Journal of Chemical Ecology 29:2575–2584. DOI: 10.1023/A:1026318203775.
- Cai QN, Han Y, Cao YZ, Hu Y, Zhao X, Bi JL. 2009. Detoxification of gramine by the cereal aphid Sitobion avenae. Journal of Chemical Ecology 35:320–325. DOI: 10.1007/s10886-009-9603-yPMID:19224277.
- Cao J, Xia X, Chen X, Xiao J, Wang Q. 2013. Characterization of flavonoids from Dryopteris erythrosora and evaluation of their antioxidant, anticancer and acetylcholinesterase inhibition activities. Food and Chemical Toxicology 51:242–250. DOI: 10.1016/j.fct.2012.09.039.
- Chrzanowski G, Leszczyński B, Czerniewicz P, Sytykiewicz H, Matok H, Krzyżanowski R, Sempruch C. 2012. Effect of phenolic acids from black currant, sour cherry and walnut on grain aphid (Sitobion avenae F.) development. Crop Protection 35:71–77. DOI: 10.1016/j.cropro.2012.01.005.
- Chu J, Yao X, Zhang Z. 2010. Responses of wheat seedlings to exogenous selenium supply under cold stress. Biological Trace Elements Research 136:355–363. DOI: 10.1007/s12011-009-8542-3.
- Cipollini D, Stevenson R, Enright S, Eyles A, Bonello P. 2008. Phenolic metabolites in leaves of the invasive shrub, Lonicera maackii, and their potential phytotoxic and anti-herbivore effects. Journal of Chemical Ecology 34:144–152. DOI: 10.1007/s10886-008-9426-2.
- Combs Jr. GF. 2001. Selenium in global food systems. British Journal of Nutrition 85:517–547. DOI: 10.1079/BJN2000280.
- Dancewicz K, Gabryś B, Dams I, Wawrzeńczyk C. 2008. Enantiospecific effect of pulegone and pulegone-derived lactones on settling and feeding of Myzus persicae (Sulz.). Journal of Chemical Ecology 34(4):530–538. DOI:10.1007/s10886-008-9448-9.
- Dancewicz K, Paprocka M, Morkunas I, Gabryś B. 2018. Struggle to survive: Aphid-plant relationships under low-light stress. A case of Acyrthosiphon pisum (Harris) and Pisum sativum L. Arthropod-plant Interactions 12:97–111. DOI: 10.1007/s11829-017-9557-x.
- Dancewicz K, Ślązak B, Kiełkiewicz M, Kapusta M, Bohdanowicz J, Gabryś B. 2020. Behavioral and physiological effects of Viola spp. cyclotides on Myzus persicae (Sulz.). Journal of Insect Physiology 122:104025. DOI: 10.1016/j.jinsphys.2020.104025.
- Dancewicz K, Sznajder K, Załuski D, Kordan B, Gabryś B. 2016. Behavioral sensitivity of Myzus persicae to volatile isoprenoids in plant tissues. Entomologia Experimentalis Et Applicata 160:229–240. DOI: 10.1111/eea.12480.
- Day TA. 1993. Relating UV-B radiation screening effectiveness of foliage to absorbing-compound concentration and anatomical characteristics in diverse group of plant. Oecologia 95:542–550. DOI: 10.1111/eea.12480.
- Deimann W, Angermuller S, Stoward PJ, Fahimi HD. 1991. Peroxidase. In: Stuart P, Pearce AGE, editors. Histochemistry, Theoretical and Applied. Edinburgh: Churchil and Livingstone. pp. 95–115.
- Dreyer DL, Jones KC. 1981. Feeding deterrency of flavonoids and related phenolics towards Schizaphis graminum and Myzus persicae: Aphid feeding deterrents in wheat. Phytochemistry 20(11):2489–2493. DOI:10.1016/0031-9422(81)83078-6.
- Durak R, Dampc J, Dampc J. 2020. Role of temperature on the interaction between Japanese quince Chaenomeles japonica and herbivorous insect Aphis pomi (Hemiptera: Aphidoidea). Environmental and Experimental Botany 176:104100. DOI: 10.1016/j.envexpbot.2020.104100.
- Durak R, Molon M, Durak T, Chrzanowski G. 2018. The enzymatic markers of the adaptation of Cinara tujafilina to changing the host plant. Ethology Ecology and Evolution 30(5):416–429. DOI:10.1080/03949370.2017.1409272.
- FAOSTAT. 2016. Food and Agriculture Data. FAO-UN. Available: http://faostat.fao.org.
- Fehrmann H, Dimond AE. 1969. Peroxidase activity and phytophtora resistance in different organs of the potato plants. Phytopathology 57:69–72.
- Foyer CH, Rasool B, Davey JW, Hancock RD. 2016. Cross-tolerance to biotic and abiotic stresses in plants: A focus on resistance to aphid infestation. Journal of Experimental Botany 67(7):2025–2037. DOI:10.1093/jxb/erw079.
- Francis F, Vanhaelen N, Haubruge E. 2005. Glutathione S-transferases in the adaptation to plant secondary metabolites in the Myzus persicae aphid. Archives of Insect Biochemistry and Physiology 58(3):166–174. DOI:10.1002/arch.20049.
- Gabryś B, Dancewicz K, Gliszczyńska A, Kordan B, Wawrzeńczyk C. 2015. Systemic deterrence of aphid probing and feeding by β-damascone analogues. Journal of Pest Science 88(3):507–516. DOI:10.1007/s10340-014-0635-x.
- Giordanengo P, Brunissena L, Rusteruccia C, Vincent C, van Bel A, Dinant S, Girousse C, Faucher M, Bonnemain JL. 2010. Compatible plant-aphid interactions: How aphids manipulate plant responses. Comptes rendus biologies 333(6–7):516–523. DOI:10.1016/j.crvi.2010.03.007.
- Goławska S. 2007. Deterrence and toxicity of plant saponins for the pea aphid Acyrthosiphon pisum Harris. Journal of Chemical Ecology 33:1598–1606. DOI: 10.1007/s10886-007-9333-y.
- Goławska S, Łukasik I. 2012. Antifeedant activity of luteolin and genistein against the pea aphid Acyrthosiphon pisum. Journal of Pest Science 85:443–450. DOI: 10.1007/s10340-012-0452-z.
- González-Santoyo I, Córdoba-Aguilar A. 2012. Phenoloxidase: A key component of the insect immune system. Entomologia Experimentalis Et Applicata 142:1–16. DOI: 10.1111/j.1570-7458.2011.01187.x.
- Grudniewska A, Dancewicz K, Białońska A, Wawrzeńczyk C, Gabryś B. 2013. Piperitone-derived saturated lactones: Synthesis and aphid behavior-modifying activity. Journal of Agricultural and Food Chemistry 61:3364–3372. DOI: 10.1371/journal.pone.0131028.
- Gul H, Kinza S, Shinwari ZK, Hamayun M. 2017. Effect of selenium on the biochemistry of Zea mays under salt stress. Pakistan Journal of Botany 49(SI):25–32.
- Gupta M, Gupta S. 2017. An overview of plant uptake, metabolism, and toxicity in plants. Frontiers in Plant Science 7:2074. DOI: 10.3389/fpls.2016.02074.
- Halarewicz A, Gabryś B. 2012. Probing behavior of bird cherry-oat aphid Rhopalosiphum padi (L.) on native bird cherry Prunus padus L. and alien invasive black cherry Prunus serotina Erhr. in Europe and the role of cyanogenic glycosides. Arthropod-plant Interactions 6:497–505. DOI: 10.1007/s11829-012-9228-x.
- Hartikainen H, Xue H, Piironen V. 2000. Selenium as an antioxidant. Plant and Soil 225:193–200. DOI: 10.1023/A:1026512921026.
- Havaux M. 2013. Carotenoid oxidation products as stress signals in plants. The Plant Journal 79:597–606. DOI: 10.1111/tpj.12386.
- Heng-Moss TM, Ni X, Macedo T, Markwell JP, Baxendale FP, Quisenberry SS, Tolmay V. 2003. Comparison of chlorophyll and carotenoid concentrations among Russian wheat aphid (Homoptera: Aphididae)-infested wheat isolines. Journal of Economic Entomology 96(2):475–481. DOI:10.1093/jee/96.2.475.
- Hiscox JD, Israelstam GF. 1979. A method for the extraction of chlorophyll from leaf tissue without maceration. Canadian Journal of Botany 57(12):1332–1334. DOI:10.1139/b79-163.
- Hodges S, Ward JL, Beale MH, Bennett M, Mansfield JW, Powell G. 2013. Aphid-induced accumulation of trehalose in Arabidopsis thaliana is systemic and dependent upon aphid density. Planta 237:1057–1064. Available: jstor.org/stable/42568899.
- Holman J. 2009. Host plant catalog for Aphids. Palearctic region. Berlin/Heidelberg: Springer Science + Business Media B.V.
- Katagiri C. 1979. α-D-glucosidase in the serum of the American cockroach Periplaneta americana. Insect Biochemistry 9:199–204. DOI: 10.1016/0020-1790(79)90051-9.
- Kolbert Z, Lehotai N, Molnar A, Feigl G. 2016. “The roots” of selenium toxicity: A new concept. Plant Signaling and Behaviour 11(10):e1241935. DOI:10.1080/15592324.2016.1241935.
- Kordan B, Gabryś B, Dancewicz K, Lahuta L, Piotrowicz-Cieślak A, Rowińska E. 2008. European yellow lupine Lupinus luteus and narrow-leaf lupine Lupinus angustifolius as hosts for the pea aphid Acyrthosiphon pisum. Entomologia Experimentalis Et Applicata 128(1):139–146. DOI:10.1111/j.1570-7458.2008.00702.x.
- Kozak A, Chrzanowski G, Sempruch C, Klewek A, Chwedczuk M. 2015. Effect of selected flavonoids on the behaviour of the bird cherry-oat aphid (Rhopalosiphum padi L.) during the colonization of winter wheat. Progress in Plant Protection 55(2):202–206. DOI:10.14199/ppp-2015-033.
- Krzyżanowski R, Bednarczyk I, Cudziło-Abramczuk J. 2018. Effect of chlorophenoxy herbicides residues on probing behaviour of cereal aphid. Agronomy Science 73(3):29–36. DOI:10.24326/as.2018.3.3.
- Kubo I. 2006. New concept to search for alternate insect control agents from plants. In: Rai M, Carpinella M, editors. Naturally occurring bioactive compounds Vol. 3. Amsterdam: Elsevier. pp. 61–80. DOI:10.1016/S1572-557X(06)03004-2.
- Kuśnierczyk A, Winge P, Jurstad TS, Troczyńska J, Rossiter JT, Bones AM. 2008. Towards global understanding of plant defence against aphids-timing and dynamics of early Arabidopsis defence responses to cabbage aphid (Brevicoryne brassicae) attack. Plant, Cell & Environment 31(8):1097–1115. DOI:10.1111/j.1365-3040.2008.01823.x.
- Laamari M, Khelfa L, Cœur d’Acier A. 2008. Resistance source to cowpea aphid (Aphis craccivora Koch) in broad bean (Vicia faba L.). Algerian landrace collection. African Journal of Biotechnology 7:2486–2490. DOI: 10.5897/AJB07.804.
- Laurema S, Varis AL, Miettinen H. 1985. Studies on enzymes in the salivary glands of Lygus rugulipensis (Hemiptera: Miridae). Insect Biochemistry 15:211–224. DOI: 10.1016/0020-1790(85)90010-1.
- Leszczyński B, Dixon AFG. 1992. Resistance of cereal aphids: The interaction between hydroxamic acids and glutathione S-transferases in the grain aphid Sitobion avenae (F.) (Hom., Aphididae). Journal of Applied Entomology 113:61–67. DOI: 10.1111/j.1439-0418.1992.tb00636.x.
- Lowry OH, Rosebrough RT, Farr AL, Randall RJ. 1951. Protein measurement with folin phenol reagent. The Journal of Biological Chemistry 193:265–275.
- Łukasik I. 2007. Changes in activity of superoxide dismutase and catalase within cereal aphids in response to plant o-dihydroxyphenols. Journal of Applied Entomology 131(3):209–214. DOI:10.1111/j.1439-0418.2006.01136.x.
- Łukasik I. 2009. Effect of host plant alternation on some adaptive enzymes of the bird cherry-oat aphid, Rhopalosiphum padi (L.). Journal of Pest Science 82:203–209. DOI: 10.1007/s10340-008-0240-y.
- Łukasik I, Goławska S. 2013. Effect of host plant on levels of reactive oxygen species and antioxidants in the cereal aphids Sitobion avenae and Rhopalosiphum padi. Biochemical Systematics and Ecology 51:232–239. DOI: 10.1016/j.bse.2013.09.001.
- Łukasik I, Goławska S, Sytykiewicz H, Leszczyński B. 2015. Antioxidant defence based on glutathione in grain aphid Sitobion avenae (F.) and the bird cherry-oat aphid Rhopalosiphum padi: Responses to the host plant alteration. Allelopathy Journal 35:273–284.
- Łukaszewicz S, Politycka B, Smoleń S. 2018. Effect of selenium on the content of essential micronutrients and their translocation in garden pea. Journal of Elementology 23(4):1307–1317. DOI:10.5601/jelem.2017.22.4.1577.
- Magierowicz K, Górska-Drabik E, Sempruch C. 2019. The insecticidal activity of Satureja hortensis essential oil and its active ingredient carvacrol against Acrobasis advenella (Zinck.) (Lepidoptera, Pyralidae). Pesticide Biochemistry and Physiology 153:122–128. DOI: 10.1016/j.pestbp.2018.11.010.
- Mayoral AM, Tjallingii WF, Castañera P. 1996. Probing behaviour of Diuraphis noxia on five cereal species with different hydroxamic acid levels. Entomologia Experimentalis Et Applicata 78:341–348. DOI: 10.1111/j.1570-7458.1996.tb00799.x.
- Mechora Š. 2019. Selenium as a protective agent against pests: a review. Plants 8(8):e262. DOI:10.3390/plants8080262.
- Miles PW. 1964. Studies on the salivary physiology of plant bugs oxidase activity in the salivary apparatus and saliva. Journal of Insect Physiology 10:121–129. DOI: 10.1016/0022-1910(64)90100-3.
- Nisbet AJ, Woodford AT, Strang RHC, Connolly JD. 1993. Systemic antifeedant effects of azadirachtin on the peach-potato aphid Myzus persicae. Entomologia Experimentalis Et Applicata 68:87–98. DOI: 10.1111/j.1570-7458.1993.tb01692.x.
- Obopile M, Ositile B. 2010. Life table and population parameters of cowpea aphid, Aphis craccivora Koch (Homoptera: Aphididae) on five cowpea Vigna unguiculata (L. Walp.) varieties. Journal of Pest Science 83:9–14. DOI: 10.1007/s10340-009-0262-0.
- Pablociones MJ, Rodrigo SM, Santamaría O. 2013. Evaluation of the potential of peas (Pisum sativum L.) to be used in selenium biofortification programs under Mediterranean conditions. Biological Trace Elements Research 151:132–137. DOI: 10.1007/s12011-012-9539-x.
- Pavek PL 2012. Plant fact sheet for pea (Pisum sativum L.). USDA-Natural Resources Conservation Service. Washington: Pullman. DOI: 10.3390/agronomy2020074.
- Prado E, Tjallingii WF. 1994. Aphid activities during sieve element punctures. Entomologia Experimentalis Et Applicata 72:157─165. DOI: 10.1111/j.1570-7458.1994.tb01813.x.
- Ramel F, Birtic S, Ginies C, Soubigou-Taconnat L, Triantaphylidès C, Havaux M. 2012. Carotenoid oxidation products are stress signals that mediate gene responses to singlet oxygen in plants. Proceedings of the National Academy of Sciences of the United States of America 109(14):5535–5540. DOI:10.1073/pnas.1115982109.
- Ros GH, van Rotterdam AMD, Bussink DW, Bindraban PS. 2016. Selenium fertilization strategies for bio-fortification of food: An agro-ecosystem approach. Plant and Soil 404:99–112. DOI: 10.1007/s11104-016-2830-4.
- Ruuhola T, Tikkanen O, Tahvanainen J. 2001. Differences in host use efficiency of larvae of a generalist moth, Operophtera brumata on three chemically divergent Salix species. Journal of Chemical Ecology 27:1595–1615. DOI: 10.1023/A:1010458208335.
- Sauge MH, Kervella J, Rahbe Y. 1998. Probing behaviour of the green peach aphid Myzus persicae on resistant Prunus genotypes. Entomologia Experimentalis et Applicata 89:223–232. DOI: 10.1046/j.1570-7458.1998.00403.x.
- Simmonds MSJ. 2003. Flavonoid-insect interactions: Recent advances in our knowledge. Phytochemistry 64:21–30. DOI: 10.1016/S0031-9422(03)00293-0.
- Stefova M, Kulevanova S, Stafilov T. 2001. Assay of flavonols and quantification of quercitin in medicinal plants by HPLC with UV-diode array detection. Journal of Liquid Chromatography and Related Technology 24:2283–2292. DOI: 10.1081/JLC-100105140.
- Stewart AJ, Bozonnet S, Mullen W, Jenkins G, Lean ME, Crozier A. 2000. Occurrence of flavonols in tomatoes and tomato-based products. Journal of Agricultural and Food Chemistry 48(7):2663–2669. DOI:10.1021/jf000070p.
- Sun Y, Xia XL, Jiang JF, Chen SM, Chen FD, Lv GS. 2016. Salicylic acid-induced changes in physiological parameters and genes of the flavonoid biosynthesis pathway in Artemisia vulgaris and Dendranthema nankingense during aphid feeding. Genetics and Molecular Research 15(1):15017546. DOI:10.4238/gmr.15017546.
- Terry N, Zayed AM, de Souza MP, Tarun AS. 2000. Selenium in higher plants. Annual Review of Plant Physiology and Plant Molecular Biology 51:401–432. DOI: 10.1146/annurev.arplant.51.1.401.
- Tjallingii WF. 1985. Electrical nature of recorded signals during stylet penetration by aphids. Entomologia Experimentalis et Applicata 38:177–186. DOI: 10.1111/j.1570-7458.1985.tb03516.x.
- Tjallingii WF. 1988. Electrical recording of stylet penetration activities. In: Minks AK, Harrewijn P, editors. Aphids, their biology, natural enemies and control. Vol. 2B. Amsterdam: Elsevier. pp. 95–108.
- Tjallingii WF. 1994. Sieve element acceptance by aphids. European Journal of Entomology 91:47–52.
- Tjallingii WF. 1995. Regulation of phloem sap feeding by aphids. In: Chapman RF, de Boer G, editors. Regulatory mechanisms in insect feeding. Dordrecht: Springer. pp. 190–209.
- Tjallingii WF. 2001. Plant penetration by aphids as revealed by electrical penetration graphs. Aphid and Other Homopterous Insects 8:105–120.
- Tjallingii WF, Mayoral AM. 1992. Criteria for host plant acceptance by aphids. In: Menken SBJ, Visser JH, Harrewijn P, editors. Proceedings of 8th international symposium on insect-plant relationships. Dordrecht: Kluwer Academic Publishers. pp. 280–282. DOI:10.1007/978-94-011-1654-1_87.
- Trumble JT, Kund GS, White KK. 1998. Influence of form and quantity of selenium on the development and survival of an insect herbivore. Environmental Pollution 101(2):175–182. DOI:10.1016/S0269-7491(98)00086-4.
- Uarrota VG, Stefen DLV, Leolato LS, Gindri DM, Nerling D. 2018. Revisiting carotenoids and their role in plant stress responses: From biosynthesis to plant signaling mechanisms during stress. In: Gupta D, Palma J, Corpas F, editors. Antioxidants and antioxidant enzymes in higher plants. Cham: Springer. pp. 207–232. DOI:10.1007/978-3-319-75088-0_10.
- Urbańska A, Leszczyński B, Tjallingii WF, Matok H. 2002. Probing behaviour and enzymatic defence of the grain aphid against cereal phenolics. Electronic Journal of Polish Agriculture Universities 5(2):#02.
- Urbańska A, Tjalllingii WF, Dixon AFG, Leszczyński B. 1998. Phenol oxidizing enzymes in the grain aphids saliva. Entomologia Experimentalis et Applicata 86:197–203. DOI: 10.1046/j.1570-7458.1998.00281.x.
- van Helden M, Tjallingii WF. 1993. Tissue localisation of lettuce resistance to the aphid Nasonovia ribisnigri using electrical penetration graphs. Entomologia Experimentalis et Applicata 68:269–278. DOI: 10.1111/j.1570-7458.1993.tb01713.x.
- Vickerman DB, Trumble JT. 1999. Feeding preferences of Spodoptera exigua in response to form and concentration of selenium. Archives of Insect Biochemistry and Physiology 42:64–73. DOI: 10.1002/(SICI)1520-6327(199909)42:1<64::AID-ARCH7>3.0.CO;2-Y.
- Vickerman DB, Young JK, Trumble JT. 2002. Effect of selenium-treated alfalfa on development, survival, feeding, and oviposition preferences of Spodoptera exigua (Lepidoptera: Noctuidae). Environmental Entomology 31(6):953–959. DOI:10.1603/0046-225X-31.6.953.
- Wang Y, Oberley LW, Murhammer DW. 2001. Antioxidant defence systems of two lepidopteran insects cell lines. Free Radical Biology & Medicine 30:1254–1262. DOI: 10.1016/S0891-5849(01)00520-2.
- Watt IJ, White PF. 1977. Simple estimation of intrinsic rates for aphids and tetranychid mites. Journal of Applied Ecology 14:757–766. DOI: 10.2307/2402807.
- Wellburn AR. 1994. The spectral determination of chlorophylls a and b, as well as total carotenoids, using various solvents with spectrophotometers of different resolution. Journal of Plant Physiology 144:307–313. DOI: 10.1016/S0176-1617(11)81192-2.
- White PJ. 2016. Selenium accumulation by plants. Annals of Botany 117:217–235. DOI: 10.1093/aob/mcv180.
- White PJ, Broadley MR. 2009. Biofortification of crops with seven mineral elements often lacking in human diets – Iron, zinc, copper, calcium, magnesium, selenium and iodine. New Phytologist 182:49–84. DOI: 10.1111/j.1469-8137.2008.02738.x.
- Winkel-Shirley B. 2002. Biosynthesis of flavonoids and effect of stress. Current Opinion in Plant Biology 5:218–223. DOI: 10.1016/S1369-5266(02)00256-X.
- Woźniak A, Bednarski W, Dancewicz K, Gabryś B, Borowiak-Sobkowiak B, Bocianowski J, Samardakiewicz S, Rucińska-Sobkowiak R, Morkunas I. 2019. Oxidative stress links response to lead and Acyrthosiphon pisum in Pisum sativum L. Journal of Plant Physiology 240:152996. DOI: 10.1016/j.jplph.2019.152996.
- Yu SJ. 1996. Insect glutathione S-transferases. Zoological Studies 35(1):9–19.