Abstract
Effects of ocean acidification (OA) on the plant phenology and colonization/settlement pattern of the hydrozoan epibiont community of the leaves of the seagrass Posidonia oceanica have been studied at volcanic CO2 vents off Ischia (Italy). The study was conducted in shallow Posidonia stands (2.5–3.5 m depth), in three stations on the north and three on the south sides of the vent’s area (Castello Aragonese vents), distributed along a pH gradient. At each station, 10–15 P. oceanica shoots were collected every three months for one-year cycle (Sept 2009–2010). The shoot density of Posidonia beds in the most acidified stations along the gradient (pH < 7.4) was significantly higher than that in the control area (pH = 8.10). On the other hand, we recorded lower leaf lengths and widths in the acidified stations in the whole year of observations, compared to those in the control stations. However, the overall leaf surface (Leaf Area Index) available for epiphytes under ocean acidification conditions was higher on the south side and on both the most acidified stations because of the higher shoot density under OA conditions. The hydrozoan epibiont community on the leaf canopy accounted for seven species, three of which were relatively abundant and occurring all year around (Sertularia perpusilla, Plumularia obliqua, Clytia hemisphaerica). All hydroids species showed a clear tolerance to low pH levels, including chitinous and non-calcifying forms, likely favoured also by the absence of competition for substratum with the calcareous forms of epiphytes selected against OA.
Introduction
Ocean acidification (OA), combined with other types of natural and/or human stresses, can cause sharp changes in the structure/functioning of benthic communities (Kroeker et al. Citation2010, Citation2013; Teixido et al. Citation2018). Ocean acidification affects mainly the process of calcification of organisms with skeletons and shells composed of calcium carbonate (e.g. corals, molluscs, echinoderms, bryozoans, and many other organisms) (Royal Society Citation2005; Doney et al. Citation2009).
The use of natural sites, characterized by natural OA of the marine waters with decreasing pH, increasing pCO2, and alteration of the carbonate chemistry, has increased in these last decades with respect to mesocosm or laboratory experiments (Guilini et al. Citation2017; Foo et al. Citation2018; Gonzalez-Delgado & Hernadez Citation2018; Rastrick et al. Citation2018; Auriemma et al. Citation2019; Mecca et al. Citation2020). On the island of Ischia (Tyrrhenian Sea, Italy) there are several areas characterized by elevated CO2 emissions from the sea bottom (Tedesco Citation1996; Hall-Spencer et al. Citation2008; Gambi Citation2014; Foo et al. Citation2018; Gambi et al. Citation2020). Among these, the shallow vent system off the Castello Aragonese is the most investigated (Foo et al. Citation2018). These CO2 vents may be useful in examining the long-term response of the marine community to OA (Kroeker et al. Citation2011), especially in complex coastal systems, such as seagrass meadows, forming a model site to investigate how benthic communities associated with the seagrass Posidonia oceanica respond to OA (Garrard et al. Citation2014; Mecca et al. Citation2020). P. oceanica is the endemic and most abundant and relevant Mediterranean seagrass ecosystem, supporting a complex community of epiphytic organisms that follows a typical succession model of colonization of the leaves (Van der Ben Citation1971; Boudouresque et al. Citation2009). Epiphytes of seagrass contribute significantly to the primary production of the meadow (Buia et al. Citation1992; Nelson & Waaland Citation1997; Duarte et al. Citation2004), and are often more sensitive to environmental changes than the host plant (Delgado et al. Citation1999; Nesti et al. Citation2008; Brahim et al. Citation2010, Citation2014; Giovannetti et al. Citation2010), with shifts in species composition (Nesti et al. Citation2008; Fourqurean et al. Citation2010) and differences in their spatial distribution and heterogeneity (Piazzi et al. Citation2004; Martínez-Crego et al. Citation2010). In particular, epiphytic assemblages of P. oceanica leaves are characterized by short-living organisms that respond quickly to environmental alterations (Boero Citation1984; Boero & Fresi Citation1986). Therefore, changes in their ecology and community composition have been used to detect environmental and anthropic impacts (Piazzi et al. Citation2004; Leoni et al. Citation2006; Balata et al. Citation2008; Brahim et al. Citation2010, Citation2014; Giovannetti et al. Citation2010; Mabrouk et al. Citation2013). Furthermore, dynamics and role of epiphytic organisms in the food web are influenced by the seasonal phenology of the host seagrass (Casola et al. Citation1987; Lepoint et al. Citation1999; Mecca et al. Citation2020). Most of the previous studies have analysed the phenology and variation of the epiphytic community along the acidification gradient at CO2 vents (Martin et al. Citation2008; Garrard et al. Citation2014; Nogueira et al. Citation2017) providing generally single observations on short periods of investigation (Martin et al. Citation2008; Guilini et al. Citation2017; Nogueira et al. Citation2017), or are related to artificial Posidonia mimics (Donnarumma et al. Citation2014), as well as utilising the Free Ocean CO2 Enrichment (FOCE) systems and mesocosm experiments (Cox et al. Citation2015, Citation2016 and references herein). Recently, only the paper by Mecca et al. (Citation2020) provides observation of the leaf epiphyte community in a longer time period, coinciding with its higher development (June–October), although without detailed taxonomic analyses.
Among the animal groups that settle on Posidonia leaves, hydroids are very diverse and abundant in all Posidonia beds, together with bryozoans. Among them, a few species (Aglaophenia harpago Schenck, 1965, Sertularia perpusilla Stechow, Citation1919, Campanularia breviscyphia Sars, 1857, and Pachycordyle pusilla (Motz-Kossowska, Citation1905) are strictly associated with Posidonia, living only on the leaves of this host plant (Picard Citation1951a; Boero Citation1981a, Citation1984; Boero et al. Citation1985; Boero & Fresi Citation1986; Piraino & Morri Citation1990). This association is promoted by certain peculiarity of hydroids, as rapid growth and asexual reproduction by stolonization (Boero Citation1987). In previous studies, the potential use of benthic hydrozoans as indicators of environmental conditions is investigated (Mergner Citation1977, Citation1987; Gili & Hughes Citation1995; Megina et al. Citation2013, Citation2016; Gravili et al. Citation2017; Yilmaz et al. Citation2020). They often form characteristic assemblages in different marine habitats presenting a set of life-history traits (sessile colonies, key-actors role in marine food webs, rapid population responses to stress and disturbances) (Boero Citation1984; Gili & Hughes Citation1995) that make them suitable candidates for environmental monitoring. Morphological changes suffered by colonies of some species may be indicative of several disturbance factors, such as increased concentrations of heavy metals (Karbe Citation1972; Theede et al. Citation1979), particular hydrodynamic conditions (Riedl Citation1966; Mergner Citation1972, Citation1977, Citation1987; Wedler Citation1975; Da Silveira & Migotto Citation1991), climate change effects (Puce et al. Citation2009; Gonzalez-Duarte et al. Citation2014; Gravili et al. Citation2017; Rossi et al. Citation2019), and anthropogenic impact (Megina et al. Citation2013, Citation2016; Topçu et al. Citation2018; Yilmaz et al. Citation2020).
The aim of this study is, to highlight the effects of OA conditions on the composition and structure of the hydrozoan epiphytic community of the P. oceanica, and on the phenology of this seagrass along a pH gradient of the Castello-Ischia CO2 vents system, sampled every three months on a year-long period. In addition, to evaluate the biodiversity of the hydroids recorded in our study sites, a review of the ‘potential’ biodiversity of the Hydrozoa on Posidonia beds was undertaken, to assess both the number and the composition of the species found in a broader and comparative ecological context. Therefore, a further aim of this study is to provide a checklist of the epiphytic hydroids on P. oceanica meadows, based on an up-dated literature analysis.
Materials and methods
Study site
The study area is adjacent to the Castello Aragonese, a volcanic dome and islet located at the north-eastern side of Ischia Island (Gulf of Naples, Italy) (40°43.84ʹN 13°57.08ʹE) (Rittmann & Gottini Citation1981) (). Previous and recent gas analyses (Hall-Spencer et al. Citation2008; Foo et al. Citation2018; Gambi et al. Citation2020) showed that the seawater is acidified by gas comprising 90.1–95.3% CO2, 3.2–6.6% N2, 0.6–0.8% O2, 0.08–0.1% Ar and 0.2–0.8% CH4 (no sulphur present), while both water temperature and salinity do not change respect to normal ambient conditions. The seawater pH ranges from 8.17 (normal value) to as low as 6.57 along a continuous gradient occurring both at the south and the north sides of the Castello (Kroeker et al. Citation2011; Ricevuto et al. Citation2014). A shallow Posidonia oceanica meadow subjected to CO2 emission is present on both sides of the Castello islet (Buia et al. Citation2003; Garrard et al. Citation2014; Foo et al. Citation2018; Mecca et al. Citation2020). On the south side, the most intense venting activity includes also the Posidonia meadow; this side of the Castello is also more sheltered to wave action and water currents with respect to the north side that is exposed to the dominant north-western winds. At the north side, the active venting area is concentrated on the edge of the Posidonia meadow, and direct gas bubbling is quite limited inside the meadow. The collection of Posidonia shoots for the morphometric analyses and analyses of the epiphytic hydroids are conducted in the shallow Posidonia stands at 2.5–3.5 m depth, in three stations located on the south side (SC, S2, S3) and three on the north side (N1, N2, N3) distributed along a pH gradient (). The stations have been selected in relation to mean pH values recorded and proximity to CO2 emissions (see previous studies, Donnarumma et al. Citation2014, and for a review Foo et al. Citation2018). The S3 and N3 stations, acidified sites with extreme low pH, are located in an area with high and dense bubbling (>10 bubbles emissions to m2). Mean pH values are approximately 7.2 at the northern and 6.6 at the southern sites, near the rocky reef (Kroeker et al. Citation2011; Ricevuto et al. Citation2014). In these stations, both on the south and north sides, the P. oceanica meadow is very dense (700–800 shoots/m2) with very short leaves due to the frequent grazing by the herbivorous Sarpa salpa (Donnarumma et al. Citation2014; Mecca et al. Citation2020; Mirasole et al. Citation2021). The S2 and N2 stations are located approximately 60 m far from S3 and N3, where the bubbles emissions are reduced compared to the acidified area (<5 bubbles emissions to m2) and therefore subjected to low pH conditions. In fact, S2 and N2 have mean pH values around 7.7–7.8 (Kroeker et al. Citation2011; Ricevuto et al. Citation2014) but show a considerable variability in time, also at daily scale (Kroeker et al. Citation2011). The SC and N1 are control stations, located N1 approx. 80 m from N2, and SC approx. 30 m from S2, and where the CO2 emissions are absent, and the pH values are those of normal sea waters (8.10) (Ricevuto et al. Citation2014).
Figure 1. Map of the study area, the Castello vent system off Ischia (Italy) showing the locations of the stations under the influence of CO2 venting (N2, N3, S2, S3), and the control stations out of the venting area (N1, SC). (Background map from Gambi et al. Citation2020, modified)
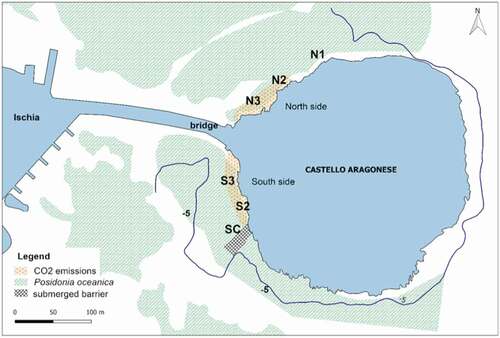
Sampling methods and data analysis
Samplings of Posidonia natural shoots (10–15 shoots) for the morphometric and epiphyte analysis were performed every three months for a whole year, in September and December 2009, March, June, and September 2010. At each station, Posidonia shoot density was measured in October 2010 by counting number of shoots in quadrats 40 × 40 cm (5 replicates per station). On the collected shoots in every station and period, a phenological analysis was performed according to the usual procedure, counting the number of leaves and measuring their length and width (Buia et al. Citation2004). From these parameters, the leaf surface area of each shoot was calculated, as well as the Leaf Area Index (mean leaf area per shoot x shoot density), which represents the leaf surface developed by the plant in a single bottom square meter (Buia et al. Citation2004). In addition, for each shoot, the hydroid species present on the leaves have been analysed by considering, position on the leaf (basal, intermediate, apical) distinguishing between internal and external leaf face; the percentage coverage for each species was estimated using a semi-quantitative code: 1 = low (less than 10% cover), 2 = medium (from 10 to 30%), 3 = high (above 30%), and in the case of branched colonies, their height as 1 = low (less than 3 mm); 2 = medium (from 3 to 6 mm); 3 = high (higher than 6 mm). Spatial and temporal trends in colonies’ height and position on the leaves were preliminarily investigated with visual data inspection and variance analysis, resulting not evident or significant. Therefore, they were not considered in further analysis. Trends in leaf coverage for each species and station/site were estimated as average of the semi-quantitative scores for leaf, independently from their position of the leaf shoot (Table S1, supplementary material). Trends in coverage across sites were estimated by ANOVA for the 3 most abundant species, using the 4 sampling periods, the different pH conditions (1, 2, 3), and the different locations (south or north sides) as categorical explanatory variables. The significance of the different variables was assessed via stepwise procedure. The similarity between sites and species was estimated by using Euclidean distance from the semi-quantitative classes of abundance.
Bibliographic survey on hydroids associated to Posidonia oceanica meadows
To better evaluate and compare our findings with previous works on epiphytic hydroids on Posidonia oceanica meadows, an inclusive bibliographic survey was performed (indexed and non-indexed journals, and grey literature). Papers have been obtained through Servizi Informatici Bibliotecari di Ateneo (S.I.B.A.) of the University of Salento (Lecce), “Web of Science” and the “Biological Abstracts” were consulted, as well. For the taxonomy, our survey follows the extensive revision of the information found in the World Register of Marine Species (Appeltans et al. Citation2011).
Results
Shoot density and morphometric analysis of Posidonia oceanica along the pH gradient
Posidonia oceanica shoot density showed, both at the north and south sides, a significant decrease (two-way ANOVA, F = 14.67, p = 0.000, d.f. 5) from the extreme low pH stations (S3 and N3) to the control ones (SC and N1). As outlined in Donnarumma et al. (Citation2014), the shoot density values were the highest at the most acidified stations: S3 = 1014 ± 73; N2 = 708 ± 98; N3 = mean 858 ± 85 (s.d.) shoot m2; S2 = 726 ± 123; N1 = 438 ± 88; SC = 494 ± 125. The mean leaf length as well as the mean shoot surface was higher on the south side respect to the north (two-way ANOVA, leaf surface F = 7.01; p = 0.013, d.f. 1; leaf length F = 5.25; p = 0.02, d.f. 1). The mean leaf length in stations S3, N2, and N3 always showed lower values than all the other ones (two-way ANOVA, F = 2.87; p = 0.03, d.f. 5, Tukey post-hoc comparisons). Leaf surface showed higher values on the south side and especially in the S2 station (two-way ANOVA, F = 3.97; p = 0.009, d.f. 5, Tukey post hoc comparisons). The Leaf Area Index showed higher values on the south side, respect to the north, and in the extreme low pH stations, with the following values: N1 = 3.3, N2 = 4.5; N3 = 5.4; S1 = 5.4; S2 = 11.4; S3 = 7.2. This indicates that although in the most acidified stations the leaves are shorter, the higher density compensates so that the space (surface) potentially available for epiphyte colonization is even higher than in the control stations.
Hydrozoan epiphytic assemblage along the pH gradient
Seven species of Hydrozoa were recorded in the Castello’ samples: Campanularia breviscyphia, Clytia hemisphaerica, Eudendrium simplex, Halecium pusillum, Obelia dichotoma, Plumularia obliqua, Sertularia perpusilla (). Among them, S. perpusilla and P. obliqua are relatively common (found, respectively, on 11% and 5% of the analysed P. oceanica leaves) and distributed among all sampling sites (). C. breviscyphia is less abundant (found on 2% of the analysed leaves) and has been observed mostly in SC and N1 sites. The other species are rare (<10 records in total). A sligthly higher abundance of C. breviscyphia in control sites with normal pH conditions (especially in SC) is the only significant spatial trend detectable from the semi-quantitative evaluation of the species presence and abundances ( and ). We did not observe any significant seasonal trend in overall community composition and species abundances and occurrences. Being common in all sites, S. perpusilla and P. obliqua are similar in their distribution (). However, P. obliqua is partially replaced by C. breviscyphia in the sites SC and N1, which are therefore associated, while all other associations among species, or species and sites are weak ().
Table I. List of the seven hydrozoan species found in this study on the CO2 vents, and their average abundance score (calculated from the semi-quantitative classification of abundance 1 = low, 2 = medium, 3 = high) for each station
Table II. Average abundance score (calculated from the semi-quantitative classification of abundance 1=low, 2=medium, 3=high) per leaf (± s.d.) of the seven hydrozoan species collected in this study, for each sampling period
Table III. Campanularia breviscyphia, ANOVA of average abundance score calculated from the semi-quantitative classification of abundance (1 = low, 2 = medium, 3 = high) per leaf according to the only significant descriptors
Posidonia oceanica hydroids checklist update
Based on a detailed literature survey, we have documented at least 86 species of hydrozoans living on P. oceanica leaves, on rhizomes, or on both layers (), updating previous data reported by Boero (Citation1981a), with an increase of 30% of the taxa if compared to the previous list that accounted for 66 species.
Table IV. List of the hydroid species recorded from Posidonia beds in the Mediterranean Sea updating previous data reported by Boero (Citation1981a)
Discussion and conclusion
The present study provides information on the composition and structure of the hydrozoan epiphytic community in Posidonia oceanica meadows and on the phenology of this seagrass exposed to a natural acidification gradient due to volcanic CO2 emissions. The results indicated that P. oceanica shoot density and plant phenology in the studied stations of the vent systems showed significant differences among pH conditions, consistently with the results of previous studies (Hall-Spencer et al. Citation2008; Donnarumma et al. Citation2014; Garrard et al. Citation2014; Mecca et al. Citation2020). Our observations confirm that Posidonia has higher levels of shoot density and shorter leaves in the stations characterized by the highest emission of bubbles, and where extremely low and high fluctuating pH conditions occur. The higher density, however, compensates for the shorter leaves with the consequence that the space/surface potentially available for epiphyte colonization (L.A.I.) is higher than in the control stations. As regards the hydrozoans, our results show that these organisms have the ability to withstand low pH values in all the periods considered, and relevant colonization by hydroids in P. oceanica subjected to ocean acidification, have been also observed in other studies both around Ischia (Donnarumma et al. Citation2014; Mecca et al. Citation2020) and in the Panarea Island (Gaglioti et al. Citation2019). This can be explained by the fact that the species found all belong to chitinous and not-calcifying forms and, in addition, being small organisms and living on the leaf surface and within the leaf canopy, hydroids can probably benefit from an attenuation of the negative effects of pH due to the plant’s photosynthesis activity (Hendriks et al. Citation2014). However, a further aspect to consider for hydroids abundance in our sites is that they could be also favoured by the reduced competition for substratum with the calcareous forms of many other epiphytes. In fact, in the studied samples, we observed a strong reduction or disappearance of calcareous organisms in the most acidified stations (e.g., coralline algae, spirorbid polychaetes, bryozoans) (Di Meglio Citation2016). This pattern was indeed observed also in previous studies on both natural and artificial/mimic P. oceanica shoots at the Castello vents (Martin et al. Citation2008; Donnarumma et al. Citation2014; Nogueira et al. Citation2017), as well as in the Posidonia meadows of other vent’s systems both around Ischia (Mecca et al. Citation2020), in the Aeolian Islands (Gaglioti et al. Citation2019), and also in shoots sampled inside special in situ mecosoms the FOCE systems (Cox et al. Citation2015, Citation2017). Tolerance to low pH conditions it is particularly evident in the more common observed hydrozoan species such as S. perpusilla and P. obliqua. Furthermore, hydroids are among the first colonizers of marine hard substrata with several species that grow on a limited range of surface types (Boero Citation1987; Boero & Hewitt Citation1992; Puce et al. Citation2005, Citation2007) and with several hydroid species that are obligate epiphytes of Posidonia leaves (Boero Citation1981a, Citation1987).
Based on a detailed literature survey, we have documented at least 86 species of hydrozoans living on P. oceanica leaves, on rhizomes, or on both layers () updating previous data reported by Boero (Citation1981a). According to Boero (Citation1981a, Citation1981b) and our updated checklist, among the epiphytic hydroids on Posidonia leaves at 2–4 m depth (the range considered in this study) have been reported the species Campanularia breviscyphia, Eudendrium simplex, Sertularia perpusilla (superficial and intermediate-superficial species); Clytia hemisphaerica, Halecium pusillum, Plumularia obliqua (species of leaves at all depths). Therefore, the biodiversity level observed in our samples (7 species) is consistent with the number of hydroids species generally associated to shallow Posidonia stands. Knowledge of their bathymetric distribution began with the studies by Boero (Citation1981a, Citation1981b), Balduzzi et al. (Citation1981, Citation1984), and Boero et al. (Citation1985) in P. oceanica meadows in the western Mediterranean Sea. Moreover, these results highlighted, as proposed by Boero (Citation1981a), the mainly shallow distributions of the exclusive epiphytic hydroids on Posidonia leaves could be explained by an association with Posidonia leaves started long ago, before the rise in sea level after the Messinian crisis (over 5–6 million years ago) according to the Den Hartog’s theory (Citation1973): Posidonia is a superficial plant that survives at greater depths owing to the Mediterranean Sea-level variations. Therefore, the distribution of the species strictly epiphytic of Posidonia leaves can be explained by their incapacity to tolerate the changes of physical-chemical conditions remaining within Posidonia optimal depths.
This association with the Posidonia leaves has been promoted by certain characteristics of hydroids such as rapid growth and asexual reproduction by stolonization (Philbert Citation1935; Von Schenck Citation1963). Moreover, the topographical/temporal separation between Posidonia and hard substrata has favoured the isolation of hydroid populations inhabiting Posidonia leaves leading to speciation among some of the Posidonia hydroids (Boero Citation1987). Furthermore, that association of hydroids with Posidonia leaves could be favoured by the good trophic conditions achieved in positions raised above the sea bottom also in shallow waters where water movement provides an equally high rate of water flow also over the rhizomes (Boero Citation1984). Adaptations by those species, probably, started because of the advantages of this substratum as great water flow around the near erect leaves and, since much of the space on Posidonia leaves is relatively free from epiphytes, showing a reduced competition for substratum (Boero Citation1987). On the other hand, hydroids have overcome the disadvantage as epiphytes of a living substratum with the rapid growth and turnover such as those of their host substratum. In fact, hydrozoan-Posidonia associated species have a rapid growth, and some species are able to pass from old leaves to new ones by stolonization (Philbert Citation1935; Von Schenck Citation1963). The hydrozoan epiphytic population of Posidonia is so typical and rich that its study can be an optimal tool for the evaluation of the conditions of the Posidonia meadows and its associated fauna. In addition, this study on the Castello vents confirms that these organisms represent some of the few “winner” organisms able to survive in view of the future scenario of ocean acidification in the global ocean.
Consent for publication
Being the corresponding author of the manuscript, I state that all authors agree to its submission and the Corresponding author has been authorized by the co-authors.
Ethical approval consent to participate
No animal testing was performed during this study.
Sampling and field studies
All necessary permits for sampling and observational field studies have been obtained by the authors from the competent Authorities and are mentioned in the acknowledgements. The study is compliant with CBD and Nagoya protocols.
Supplemental Material
Download PDF (736 KB)Acknowledgements
We wish to thank Dr Luigia Donnarumma for help in the morphological measures of Posidonia oceanica shoots, and Vincenzo Rando and Bruno Iacono for support in the field. Thanks are due to Martina Gaglioti for drawing the background map of the Castello in . The scientific field collections of the Stazione Zoologica Anton Dohrn (Naples) around Ischia are authorized by the local managing Authority of the Marine Protected Area “Regno di Nettuno”, which include also the Castello vent’s area.
Disclosure statement
No potential conflict of interest was reported by the authors.
Supplementary material
Supplemental data for this article can be accessed here.
References
- Appeltans W, Bouchet P, Boxshall GA, De Broyer C, de Voogd NJ, et al. 2011. World register of marine species. Available: http://www.marinespecies.org. Accessed Feb 2019 4.
- Auriemma R, De Vittor C, Esposito V, Gaglioti M, Gambi MC. 2019. Motile fauna associated to Cystoseira brachycarpa along a gradient of ocean acidification at a vent system off Panarea (Aeolian Islands, Italy). Biologia Marina Mediterranea 26:216–219. DOI: 10.13140/RG.2.2.29685.22246.
- Balata D, Bertocci I, Piazzi L, Nesti U. 2008. Comparison between epiphyte assemblages of leaves and rhizomes of the seagrass Posidonia oceanica subjected to different levels of anthropogenic eutrophication. Estuarine, Coastal and Shelf Science 79(3):533–540. DOI: 10.1016/j.ecss.2008.05.009.
- Balduzzi A, Bavestrello G, Belloni S, Boero F, Cattaneo R, Pansini M, Pronzato R. 1984. Valutazione dello stato di alcune praterie di Posidonia del Mar Ligure sottoposte a diverse coondizioni di inquinamento. International Workshop on Posidonia oceanica beds. Porquerrolles (France) 12–15 Octobre 1983. GIS Posidonie Marseille 1:73–78.
- Balduzzi A, Boero F, Pansini M, Pronzato R. 1981. A method for semiquantitative samplings of the sessile epifauna of Posidonia oceanica beds. Rapports et procés-verbaux des réunions Commission internationale pour l’exploration scientifique de la Mer Méditerranée 27:247–248.
- Billard A. 1936. Les fonds de pêche près d’Alexandrie. VI. Hydroïdea. Notes Mémoires Minutes Commission Industrie Égypte 13:1–11.
- Boero F. 1981a. Systematics and ecology of the hydroid population of two Posidonia oceanica meadows. Marine Ecology 2(3):181–197. DOI: 10.1111/j.1439-0485.1981.tb00093.x.
- Boero F. 1981b. Bathimetric distribution of the epifauna of a Posidonia meadow of the Isle of Ischia (Naples): Hydroids. Rapports et procés-verbaux des réunions Commission internationale pour l’exploration scientifique de la Mer Méditerranée 27:197–198.
- Boero F. 1984. The ecology of marine hydroids and effects of environmental factors: A review. Marine Ecology 5(2):93–118. DOI: 10.1111/j.1439-0485.1984.tb00310.x.
- Boero F. 1987. Evolutionary implications of habitat selection in the hydroids of Posidonia oceanica meadows. In: Bouillon J, Boero F, Cicogna F, Cornelius PFS, editors. Modern trends in the systematics, ecology and evolution of hydroids and hydromedusae. Oxford: Clarendon Press. pp. 251–256.
- Boero F, Chessa L, Chimenz C, Fresi E. 1985. The zonation of epiphytic hydroids on the leaves of some Posidonia oceanica (L.) Delile beds in the central Mediterranean. Marine Ecology 6(1):27–33. DOI: 10.1111/j.1439-0485.1985.tb00318.x.
- Boero F, Fresi E. 1986. Zonation and evolution of a rocky bottom hydroid community. Marine Ecology 7(2):123–150. DOI: 10.1111/j.1439-0485.1986.tb00152.x.
- Boero F, Hewitt C. 1992. A hydrozoan, Zanclella bryozoophyla n. g. n. sp. (Zancleidae), symbiotic with a Bryozoon. Canadian Journal of Zoology 70(9):1645–1651. DOI: 10.1139/z92-229.
- Boudouresque CF, Bernad G, Pergent G, Shili A, Verlaque M. 2009. Regression of Mediterranean seagrasses caused by natural processes and anthropogenic disturbances and stress: A critical review. Botanica Marina 52(5):395–418. DOI: 10.1515/BOT.2009.057.
- Brahim MB, Hamza A, Bouain A. 2010. Variability in the structure of epiphytic assemblages of Posidonia oceanica in relation to human interferences in the Gulf of Gabes, Tunisia. Marine Environmental Research 70:411–421. DOI: 10.1016/j.marenvres.2010.08.005.
- Brahim MB, Mabrouk L, Hamza A, Mahfoudhi M, Bouain A, Aleya L. 2015. Spatial scale variability in shoot density and epiphytic leaves of Posidonia oceanica on Kerkennah Island (Tunisia) in relation to current tide effects. Marine Ecology 36(4):1311–1331. DOI: 10.1111/maec.12231.
- Brahim MB, Mabrouk L, Hamza A, Mahfoudi M, Bouain A, Aleya L. 2014. Bathymetric variation of epiphytic assemblages on Posidonia oceanica (L.) Delile leaves in relation to anthropogenic disturbance in the southeastern Mediterranean. Environmental Science and Pollution Research 21(23):13588–13601. DOI: 10.1007/s11356-014-3315-8.
- Brinckmann A. 1964. Observations on the biology and development of Staurocladia portmanni sp. n. (Anthomedusae, Eleutheridae). Canadian Journal of Zoology 42(4):693–706. DOI: 10.1139/z64-061.
- Brinckmann-Voss A. 1970. Anthomedusae/Athecata (Hydrozoa, Cnidaria) of the Mediterranean. Part I. Capitata. Fauna e Flora del Golfo di Napoli 39:1–96.
- Brinckmann-Voss A. 1987. Seasonal distribution of hydromedusae (Cnidaria, Hydrozoa) from the Gulf of Naples and vicinity, with observations on sexual and asexual reproduction in some species. In: Bouillon J, Boero F, Cicogna F, Cornelius PFS, editors. Modern trends in the systematics, ecology and evolution of hydroids and hydromedusae. Oxford: Clarendon Press. pp. 133–141.
- Broch H. 1933. Zur Kenntis der Adriatischen Hydroidenfauna von Split. Arten und Variationen. Skrifter utgitt av det Norske Videnskaps-Akademi i Oslo, Mat.-Naturv. Klasse 4:1–115.
- Buia MC, Gambi MC, Lorenti M, Dappiano M, Zupo V. 2003. Aggiornamento sulla distribuzione e sullo stato ambientale dei sistemi a fanerogame marine (Posidonia oceanica e Cymodocea nodosa) delle isole Flegree. In: Gambi MC, De Lauro M, Iannuzzi F, editors. Ambiente marino costiero e territorio delle isole Flegree (Ischia Procida Vivara - Golfo di Napoli). Accademia Scienze Lettere Arti Napoli, Memorie Società Scienze Fisiche e Matematiche 5. Napoli: Liguori Editore. pp. 163–186.
- Buia MC, Gambi MC, Dappiano M. 2004. The seagrass systems. Biologia Marina Mediterranea 11(Suppl. 1):133–184.
- Buia MC, Zupo V, Mazzella L. 1992. Primary production and growth dynamics in Posidonia oceanica. Marine Ecology 13(1):2–16. DOI: 10.1111/j.1439-0485.1992.tb00336.x.
- Casola E, Scardi M, Mazzella L, Fresi E. 1987. Structure of the epiphytic community of Posidonia oceanica leaves in a shallow meadow. Marine Ecology 8(4):285–296. DOI: 10.1111/j.1439-0485.1987.tb00189.x.
- Cox TE, Gazeau F, Alliouane S, Hendriks I, Mahacek P, Le Fur A, Gattuso J-P. 2016. Effects of in situ CO2 enrichment on structural characteristics, photosynthesis, and growth of the Mediterranean seagrass Posidonia oceanica. Biogeosciences 13(7):2179–2194. DOI: 10.5194/bg-13-2179-2016.
- Cox TE, Nash M, Gazeau F, Déniel M, Legrand E, Alliouane S, Mahacek P, Le Fur A, Gattuso J-P, Martin S. 2017. Effects of in situ CO2 enrichment on Posidonia oceanica epiphytic community composition and mineralogy. Marine Biology 164(5):103. DOI: 10.1007/s00227-017-3136-7.
- Cox TE, Schenone S, Delille J, Díaz‐Castañeda V, Alliouane S, J-p G, Gazeau F. 2015. Effects of ocean acidification on Posidonia oceanica epiphytic community and shoot productivity. Journal of Ecology 103(6):1594–1609. DOI: 10.1111/1365-2745.12477.
- Da Silveira FL, Migotto AE. 1991. The variation of Halocordyle disticha (Cnidaria, Athecata) from the Brazilian coast: An environmental indicator species? Hydrobiologia 216(1):437–442. DOI: 10.1007/BF00026497.
- Delgado O, Ruiz J, Pérez M, Ballesteros E. 1999. Effects of fish farming on seagrass (Posidonia oceanica) in a Mediterranean bay: Seagrass decline after organic loading cessation. Oceanologica Acta 22(1):109–117. DOI: 10.1016/S0399-1784(99)80037-1.
- Di Meglio E. 2016. Analisi temporale della colonizzazione di epibionti su mimics di foglie di Posidonia oceanica lungo un gradiente di acidificazione marina in un sito di emissioni di CO2 dell’isola d’Ischia. Master Thesis. University Federico II. (In Italian).
- Doney SC, Fabry VJ, Feely RA, Kleypas JA. 2009. Ocean acidification: The other CO2 problem. Annual Review of Marine Science 1(1):169–192. DOI: 10.1146/annurev.marine.010908.163834.
- Donnarumma L, Lombardi C, Cocito S, Gambi MC. 2014. Settlement pattern of Posidonia oceanica epibionts along a gradient of ocean acidification: An approach with mimics. Mediterranean Marine Science 15(3):498–509. DOI: 10.12681/mms.677.
- Duarte CM, Borum J, Short FT, Walker DI. 2004. Seagrass ecosystems: Their global status and prospects. In: Polunin NVC, editor. Aquatic ecosystems: Trends and global prospects. Cambridge: Cambridge University Press. pp. 281–294.
- El Beshbeeshy ME. 1994. Descriptions of some thecate hydroids (Cnidaria-Hydrozoa) from the Egyptian Mediterranean waters. Part 3 family: Sertulariidae. Bulletin of National Institute of Oceanography & Fisheries 20:145–159.
- El Beshbeeshy ME. 1995. Some thecate hydroids associated with Posidonia oceanica (L.) Delile meadows in the Egyptian Mediterranean waters. Bulletin of National Institute of Oceanography & Fisheries 21:313–317.
- Foo SA, Byrne M, Ricevuto E, Gambi MC. 2018. The carbon dioxide vents of Ischia, Italy, a natural laboratory to assess impacts of ocean acidification on marine ecosystems: An overview of research and comparisons with other vent systems. Oceanography and Marine Biology - an Annual Review 56:237–310.
- Fourqurean JW, Muth MFA, Boyer JN. 2010. Epiphyte loads on seagrasses and microphytobenthos abundance are not reliable indicators of nutrient availability in oligotrophic coastal ecosystems. Marine Pollution Bulletin 60(7):971–983. DOI: 10.1016/j.marpolbul.2010.03.003.
- Fresi E, Chimenz C, Marchio G. 1982. Zonazione di Briozoi ed Idroidi epifiti in una prateria di Posidonia oceanica (L.) Delile. Naturalista Siciliano 3:499–508.
- Gaglioti M, Auriemma R, De Vittor C, Esposito V, Teixido N, Gambi MC. 2019. A pilot study on Posidonia oceanica features of a hydrothermal system at Panarea (Aeolian Islands, Italy). Biologia Marina Mediterranea 26:236–237.
- Galea HR. 2007. Hydrozoa, La Ciotat and nearby areas, Mediterranean coast of France. Check List 3(3):193–199. DOI: 10.15560/3.3.193.
- Gambi MC. 2014. Emissioni sommerse di CO2 lungo le coste dell’isola d’Ischia. Rilievi su altre aree come possibili laboratori naturali per lo studio dell’acidificazione e cambiamento climatico a mare. Notiziario SIBM 66:67–79. www.sibm.it.
- Gambi MC, Gaglioti M, Teixido N. 2020. I sistemi di emissione di CO2 dell’isola di Ischia (Mar Tirreno). Memorie Descrittive della Carta Geologica d’Italia 105:55–64.
- García Rubies A. 1992. Habitat differentiation among epiphytic hydroids of the seagrass Posidonia oceanica from the Medes Islands (NE Catalonia, Spain). In: Bouillon J, Boero F, Cicogna F, Gili JM, Hughes RG, editors. Aspects of hydrozoan biology. Scientia Marina 56:263–267.
- Garrard SL, Gambi MC, Scipione MB, Patti FP, Lorenti M, et al. 2014. Indirect effects may buffer negative responses of seagrass invertebrate communities to ocean acidification. Journal of Experimental Marine Biology and Ecology 461:31–38. DOI: 10.1016/jembe.2014.07.011.
- Gili JM. 1986. Estudio sistematico y faunistico de los Cnidarios de la costa Catalana. Thesis. University of Barcelona. pp. 1–565.
- Gili JM, Hughes RG. 1995. The ecology of marine benthic hydroids. Oceanography and Marine Biology - An Annual Review 33:351–426.
- Giovannetti E, Montefalcone M, Morri C, Bianchi CN, Albertelli G. 2010. Early warning response of Posidonia oceanica epiphyte community to environmental alterations (Ligurian Sea, NW Mediterranean). Marine Pollution Bulletin 60(7):1031–1039. DOI: 10.1016/j.marpolbul.2010.01.024.
- Gonzalez-Delgado S, Hernadez JC. 2018. The importance of natural acidified systems in the study of ocean acidification: What have we learned? Advances in Marine Biology 80:57–99. DOI: 10.1016/bs.amb.2018.08.001.
- Gonzalez-Duarte MM, Megina C, Piraino S. 2014. Looking for long-term changes in hydroid assemblages (Cnidaria, Hydrozoa) in Alboran Sea (South-Western Mediterranean): A proposal of a monitoring point for the global warming. Helgoland Marine Research 68(4):511–521. DOI: 10.1007/s10152-014-0406-3.
- Gravili C, Cozzoli F, Boero F. 2017. The historical reconstruction of distribution of the genus Halecium (Hydrozoa: Haleciidae): A biological signal of ocean warming? Marine Biological Research 13(5):587–601. DOI: 10.1080/17451000.2017.1290805.
- Guilini K, Weber M, de Beer D, Schneider M, Molari M, et al. 2017. Response of Posidonia oceanica seagrass and its epibiont communities to ocean acidification. PLoS ONE 12(8):e0181531. DOI: 10.1371/journal.pone.0181531.
- Hall-Spencer JM, Rodolfo-Metalpa R, Martin S, Ransome E, Fine M, et al. 2008. Volcanic carbon dioxide vents show ecosystem effects of ocean acidification. Nature 454(7200):96–99. DOI: 10.1038/nature07051.
- Hartog CD. 1973. Structure, function and classification in seagrasses communities. Leiden, Netherlands: International Seagrass Workshop. pp. 23.
- Hendriks IE, Olsen YS, Ramajo L, Basso L, Steckbauer A, Moore TS, Duarte CM. 2014. Photosynthetic activity buffers ocean acidification in seagrass meadows. Biogeosciences 11(2):333–346. DOI: 10.5194/bg-11-333-2014.
- Isinibilir M, Martell L, Topcu EN, Yilmaz IN, Piraino S. 2015. First inventory of the shallow-water benthic hydrozoan assemblages of Gokceada Island (northern Aegean Sea). Italian Journal of Zoology 82:281–290. DOI: 10.1080/11250003.2014.977970.
- Karbe L. 1972. Marine hydroiden als testorganismen zur prufung der toxizitat von abwasserstoffen. Die Wirkung von Schwermetallen auf Kolonien von Eirene viridula. Marine Biology 12(4):316–328. DOI: 10.1007/BF00366332.
- Kroeker KJ, Kordas RL, Crim RN, Singh GG. 2010. Meta-analysis reveals negative yet variable effects of ocean acidification on marine organisms. Ecology Letters 13(11):1419–1434. DOI: 10.1111/j.1461-0248.2010.01518.x.
- Kroeker KJ, Micheli F, Gambi MC. 2013. Ocean acidification causes ecosystem shifts via altered competitive interactions. Nature Climate Change 3(2):156–159. DOI: 10.1038/NCLIMATE1680.
- Kroeker KJ, Micheli F, Gambi MC, Martz TR. 2011. Divergent ecosystem responses within a benthic marine community to ocean acidification. Proceedings of the National Academy of Sciences USA 108(35):14515–14520. DOI: 10.1073/pnas.1107789108.
- Leoni V, Pasqualini V, Pergent-Martini C, Vela A, Pergent G. 2006. Morphological responses of Posidonia oceanica to experimental nutrient enrichment of the canopy water. Journal of Experimental Marine Biology and Ecology 339(1):1–14. DOI: 10.1016/j.jembe.2006.05.017.
- Lepoint G, Havelange S, Gobert S, Bouquegneau JM. 1999. Fauna vs flora contribution to the leaf epiphytes biomass in a Posidonia oceanica seagrass bed (Revellata Bay, Corsica). Hydrobiologia 394:63–67. DOI: 10.1023/A:1003557303904.
- Lo Bianco S. 1909. Notizie biologiche riguardanti specialmente il periodo di maturità sessuale degli animali del Golfo di Napoli. Mittheilungen aus der Zoologischen Station zu Neapel 19:513–763.
- Mabrouk L, Hamza A, Brahim MB, Bradai M-N. 2013. Variability in the structure of epiphyte assemblages on leaves and rhizomes of Posidonia oceanica in relation to human disturbances in a seagrass meadow off Tunisia. Aquatic Botany 108:33–40. DOI: 10.1016/j.aquabot.2013.03.002.
- Marinopoulos J. 1979. Biological survey of the eastern Mediterranean Sea: Hydroids (preliminary study). Rapports et procés-verbaux des réunions Commission internationale pour l’exploration scientifique de la Mer Méditerranée 2526:119–120.
- Marktanner-Turneretscher G. 1890. Die Hydroiden des k. k. naturhistorischen Hofmuseums. Annalen des Naturhist Hofmuseums 5:195–286.
- Martin S, Rodolfo-Metalpa R, Ransome E, Rowley S, Buia MC, et al. 2008. Effects of naturally acidified seawater on seagrass calcareous epibionts. Biology Letters 4(6):689–692. DOI: 10.1098/rsbl.2008.0412.
- Martínez-Crego B, Prado P, Alcoverro T, Romero J. 2010. Composition of epiphytic leaf community of Posidonia oceanica as a tool for environmental biomonitoring. Estuarine, Coastal and Shelf Science 88(2):199–208. DOI: 10.1016/j.ecss.2010.03.026.
- Mecca S, Casoli E, Ardizzone G, Gambi M. 2020. Effects of ocean acidification on phenology and epiphytes of the seagrass Posidonia oceanica at two CO2 vent systems of Ischia (Italy). Mediterranean Marine Science 21(1):70–83. DOI: 10.12681/mms.20795.
- Medel MD, Vervoort W. 1995. Plumularian hydroids (Cnidaria: Hydrozoa) from the Strait of Gibraltar and nearly areas. Zoologische Verhandelingen, Leiden 300:1–72.
- Megina C, González-Duarte MM, López-González PJ. 2016. Benthic assemblages, biodiversity and invasiveness in marinas and commercial harbours: An investigation using a bioindicator group. Biofouling 32(4):465–475. DOI: 10.1080/08927014.2016.1151500.
- Megina C, González-Duarte MM, López-González PJ, Piraino S. 2013. Harbours as marine habitats: Hydroid assemblages on sea-walls compared with natural habitats. Marine Biology 160(2):371–381. DOI: 10.1007/s00227-012-2094-3.
- Mergner H. 1972. The influences of severa1 ecological factors on the hydroid growth of some Jamaican coral cays. In: Mukundan C, Gopinadha Pillai CS, editors. Proceedings of the 1st international symposium on corals and coral reefs. Mandapan Camp, India: Marine Biological Association of India. pp. 275–290.
- Mergner H. 1977. Hydroids as indicator species of ecological parameters in Caribbean and Red Sea coral reefs. In: Taylor DL, editor. Proceedings of the 3rd international coral reef symposium, vol. 1 (Biology). Miami, Florida: Rosenstiel School of Marine and Atmospheric Science. pp. 119–125.
- Mergner H. 1987. Hydroids as indicator species of environmental factors on coral reefs. In: Bouillon J, Boero F, Cicogna F, Cornelius PFS, editors. Modern trends in the systematics, ecology, and evolution of hydroids and hydromedusae. Oxford: Oxford Science Publications. pp. 185–195.
- Mirasole A, Badalamenti F, Di Franco A, Gambi MC, Teixido Ullod N. 2021. Boosted fish abundance associated with Posidonia oceanica meadows in a temperate shallow CO2 vent. Science of the Total Environment 771: 145438. DOI: 10.1016/j.scitotenv.2021.145438.
- Morri C, Bavestrello G, Bianchi CN. 1991. Faunal and ecological notes on some benthic cnidarian species from the Tuscan Archipelago and eastern Ligurian Sea (western Mediterranean). Bollettino dei musei e degli Istituti Biologici dell’Universita di Genova 5455:27–47.
- Morri C, Bianchi CN. 1982. Contributo alla conoscenza degli idrozoi lagunari italiani: Distribuzione di idropolipi lungo un gradiente ambientale nella laguna di Chioggia (Nord Adriatico). Atti XIV Congresso Società italiana Biologia marina. Bollettino dei musei e degli istituti biologici dell’Università di Genova 50:270–274.
- Morri C, Bianchi N. 1976. Osservazioni sull’idroide Cladonema radiatum. Estratto Bollettino Gruppo Ricerche Biologia Marina “Cerianthus” Genova 4:11–17.
- Motz-Kossowska S. 1905. Contribution à la connaissance des hydraires de la Méditerranée occidentale. I. Hydraires gymnoblastiques. Archives de zoologie expérimentale et générale, ser 4(3):39–98.
- Motz-Kossowska S. 1911. Contribution à la connaissance des hydraires de la Méditerranée occidentale. II.- Hydraires calyptoblastiques. Archives de zoologie expérimentale et générale, ser 5(6):325–352.
- Nelson TA, Waaland JR. 1997. Seasonality of eelgrass, epiphyte, and grazer biomass and productivity in subtidal eelgrass meadows subjected to moderate tidal amplitude. Aquatic Botany 56(1):51–74. DOI: 10.1016/S0304-3770(96)01094-7.
- Nesti U, Piazzi L, Balata D. 2008. Variability in the structure of epiphytic assemblages of the Mediterranean seagrass Posidonia oceanic in relation to depth. Marine Ecology 30(3):276–287. DOI: 10.1111/j.1439-0485.2008.00275.x.
- Nogueira P, Gambi MC, Vizzini S, Califano G, Tavares AM, et al. 2017. Altered epiphyte community and sea urchin diet in Posidonia oceanica meadows in the vicinity of volcanic CO2 vents. Marine Environmental Research 127:102–111. DOI: 10.1016/j.marenvres.2017.04.002.
- Peña Cantero AL, García Carrascosa AM. 2002. The benthic hydroid fauna of the Chafarinas Islands (Alborán Sea, western Mediterranean). Zoologische Verhandelingen, Leiden 337:1–180.
- Philbert M. 1935. Le phénomène de stolonisation chez trois espèces d’hydraires fixés sur les Posidonies en Méditerranée. Bulletin de l’Institut océanographique de Monaco 663:1–8.
- Piazzi L, Balata D, Cinelli F, Benedetti-Cecchi L. 2004. Patterns of spatial variability in epiphytes of Posidonia oceanica. Differences between a disturbed and two reference locations. Aquatic Botany 79(4):345–356. DOI: 10.1016/j.aquabot.2004.05.006.
- Picard J. 1951a. Note sur les hydraires littoraux de Banyuls-sur-Mer. Vie Milieu 2:338–349.
- Picard J. 1951b. Les hydraires des formations coralligènes des côtes françaises de la Méditerranée. Vie Milieu 2:255–261.
- Picard J. 1952. Les Hydrozoaires des herbiers de Zostéracées des côtes françaises de la Méditerranée. Vie Milieu 2:217–233.
- Picard J, Le Roch S. 1949. Les cnidaires épiphytes des zostères de la Méditerranée (note préliminaire). Feuille Nature 4:93–94.
- Piraino S, Morri C. 1990. Zonation and ecology of epiphytic hydroids in a Mediterranean coastal lagoon: The ‘Stagnone’ of Marsala (north-west Sicily). Marine Ecology 11(1):43–60. DOI: 10.1111/j.1439-0485.1990.tb00227.x.
- Puce S, Bavestrello G, Di Camillo CG, Boero F. 2007. Symbiotic relationships between hydroids and bryozoans. Symbiosis 44:137–143.
- Puce S, Bavestrello G, Di Camillo CG, Boero F. 2009. Long-term changes in hydroid (Cnidaria, Hydrozoa) assemblages: Effect of Mediterranean warming? Marine Ecology 30(3):313–326. DOI: 10.1111/j.1439-0485.2009.00283.x.
- Puce S, Calcinai B, Bavestrello G, Cerrano C, Gravili C, Boero F. 2005. Hydrozoa (Cnidaria) symbiotic with porifera: A review. Marine Ecology 26(2):73–81. DOI: 10.1111/j.1439-0485.2005.00050.x.
- Ramil F, Vervoort W. 1992. Report on the Hydroida collected by the “BALGIM” expedition in and around the Strait of Gibraltar. Zoologische Verhandelingen, Leiden 277:1–262.
- Rastrick SSP, Graham H, Azetsu-Scott K, Calosi P, Chierici M, et al. 2018. Using natural analogues to investigate the effects of climate change and ocean acidification on Northern ecosystems. ICES Journal of Marine Science 75(7):2299–2311. DOI: 10.1093/icesjms/fsy128.
- Redier L. 1962a. Hydraires et bryozoaires de Méditerranée. I - Monaco. Cahier des Naturalistes 18:23–26.
- Redier L. 1962b. Hydraires et bryozoaires de Méditerranée. II - Banyuls-sur-Mer. Cahier des Naturalistes 18:33–38.
- Relini Orsi L, Zotti A, Relini G. 1977. Epifauna sessile di Posidonia oceanica in una prateria profonda del Mar Ligure. Atti del IX Congresso Società Italiana di Biologia Marina. In: Cinelli F, Fresi E, Mazzella L, editors. Firenze: Olschki A. Publisher. pp. 399–413.
- Repetto L, Montanari M, Martini F. 1977. Popolamenti di substrati artificiali posti su un fondo a coralligeno ed in una prateria di Posidonia - V: Idroidi. Atti del IX Congresso Società Italiana di Biologia Marina:415–425.
- Ricevuto E, Kroeker KJ, Ferrigno F, Micheli F, Gambi MC. 2014. Spatio-temporal variability of polychaete colonization at volcanic CO2 vents (Italy) indicates high tolerance to ocean acidification. Marine Biology 161(12):2909–2919. DOI: 10.1007/s00227-014-2555-y.
- Riedl R. 1966. Biologie der Meereshöhlen. Hamburg: Paul Parey.
- Rittmann A, Gottini V. 1981. L’isola d’Ischia. Geologia. Bollettino del Servizio Geologico d’Italia 101:131–274.
- Roca I. 1987. Hydroids on Posidonia in Majorcan waters. In: Bouillon J, Boero F, Cicogna F, Cornelius PFS, editors. Modern trends in the systematics, ecology and evolution of hydroids and hydromedusae. Oxford: Clarendon Press. pp. 209–214.
- Roca I, Moreno I, Barceló R. 1991. Distribución espacial y temporal de los hidroideos de Posidonia oceanica (L.) Delile en una predera del Illot del Sec (Bahía de Palma, Baleares). Boletin del Instituto Espanol de Oceanografia 7:67–74.
- Rossi L. 1961. Idroidi viventi sulle scogliere del promontorio di Portofino (Golfo di Genova). Annali del Museo Civico di Storia Naturale di Genova 72:69–85.
- Rossi L. 1971. Guida a cnidari e ctenofori della fauna italiana. Quaderni della Civica Stazione Idrobiologica di Milano 2:1–101.
- Rossi S, Gravili C, Milisenda G, Bosch-Belmar M, De Vito D, Piraino S. 2019. Effects of global warming on reproduction and potential dispersal of Mediterranean Cnidarians. The European Zoological Journal 86(1):255–271. DOI: 10.1080/24750263.2019.1631893.
- Royal Society. 2005. Ocean acidification due to increasing atmospheric carbon dioxide. London: The Royal Society UK.
- Schmidt HE. 1973. Biogeographical problems of the Red Sea area exemplified by hydroids. In: Zeitschel B, editor. The biology of the Indian Ocean (= J. Jacobs et al., Ecological studies, analysis and synthesis, 3). Heidelberg, New York: Springer-Verlag Berlin. pp. 283–287.
- Schuchert P. 1997. Review of the family Halopterididae (Hydrozoa, Cnidaria). Zoologische Verhandelingen, Leiden 309:1–162.
- Stechow E. 1919. Zur Kenntnis der Hydroidenfauna des Mittelmeeres, Amerikas und anderer Gebiete, nebst Angaben über einige Kirchenpauer’sche Typen von Plumulariden. Zoologische Jahrbücher/Abteilung für Systematik 42:1–172.
- Stechow E. 1923. Zur Kenntis der Hydroidenfauna des Mittelmeeres, Amerikas und anderer Gebiete. II. Teil. Zoologische Jahrbücher/Abteilung für Systematik 47:29–270.
- Svoboda A. 1979. Beitrag zur Okologie, Biometrie und Systematik der Mediterranen Aglaophenia Arten (Hydroidea). Zoologische Verhandelingen, Leiden 167:1–114.
- Svoboda A, Cornelius PFS. 1991. The European and Mediterranean species of Aglaophenia (Cnidaria: Hydrozoa). Zoologische Verhandelingen, Leiden 274:1–72.
- Tedesco D. 1996. Chemical and isotopic investigation of fumarolic gases from Ischia Island (Southern Italy): Evidence of magmatic and crustal contribution. Journal of Volcanology and Geothermal Research 74(3–4):233–242. DOI: 10.1016/S0377-0273(96)00030-3.
- Teixido N, Gambi MC, Parravicini V, Kroeker K, Micheli F, Villeger S, Ballesteros E. 2018. Functional biodiversity loss along natural CO2 gradients. Nature Communication 9. DOI: 10.1038/s41467-018-07592-1.
- Theede H, Scholz N, Fischer H. 1979. Temperature and salinity effects on the acute toxicity of cadmium to Laomedea loveni (Hydrozoa). Marine Ecology Progress Series 1:13–19. DOI: 10.3354/meps001013.
- Topçu NE, Martell LF, Yilmaz IN, Isinibilir M. 2018. Benthic hydrozoans as potential indicators of water masses and anthropogenic impact in the Sea of Marmara. Mediterranean Marine Science 19:273–283. DOI: 10.12681/mms.15117.
- Van der Ben D. 1971. Les épiphytes des feuilles de Posidonia oceanica Delile sur les côtes françaises de la Méditerranée. Mémoires Institut Royal des Sciences Naturelles de Belgique 168:1–101.
- Von Schenck DA. 1963. Spécialisation de la réproduction sexuelle de quelques hydroïdes vivants dans la Posidonia. Pubblicazioni della Stazione Zoologica di Napoli 32(suppl):117–122.
- Von Schenck DA. 1965. Aglaophenia harpago, a new species of the Plumulariidae (Hydroidea). Pubblicazioni della Stazione Zoologica di Napoli 34:211–215.
- Wedler E. 1975. Okologische Untersuchungen an Hydroiden des Felslitorals von Santa Marta (Kolumbien). Helgoländer Wissenschaftliche Meeresuntersuchungen 27(3):324–363. DOI: 10.1007/BF01611700.
- Yamada M. 1961. Polyp and medusa of Podocoryne hartlaubi Neppi & Stiasny (Hydrozoa) from the Gulf of Naples. Pubblicazioni della Stazione Zoologica di Napoli 32:134–143.
- Yilmaz IN, Martell L, Topçu NE, Isinibilir M. 2020. Benthic hydrozoan assemblages as potential indicators of environmental health in a Mediterranean marine protected area. Mediterranean Marine Science 21(1):36–46. DOI: 10.12681/mms.20593.