Abstract
Mitochondria are organelles that play a crucial role in cell physiology, cell death, and aging. They are among the first responders to different stressors that originate from the environment. Cadmium as a heavy metal affects different levels of body organization: from organs through tissues and cells to organelles. Based on our previous research results, we decided to check how the exposure to cadmium affects the functioning of mitochondria in different organs of soil living centipede Lithobius forficatus. The activity of mitochondria in somatic and germ cells has been analyzed using transmission electron microscopy (TEM), confocal microscopy, and flow cytometry. Changes in the mitochondrial membrane potential and mitochondrial dismutase (MnSOD) activity in relation to the accumulation of reactive oxygen species (ROS) caused by cadmium exposure have been studied. Individuals were divided into 3 experimental groups depending on cadmium concentration in soil. Changes in mitochondrial ultrastructure caused by cadmium are tissue-dependent and associated with an increase of ROS levels. The system of ROS and MnSOD activation works more efficiently in the case of gonads than in the digestive system. While the short-term cadmium exposure alters the fine structure of both the somatic and germ-line cells in gonads, the long-term cadmium exposure causes mitochondrial ultrastructure regeneration.
1. Introduction
Heavy metals such as cadmium can enter the freshwater, marine or terrestrial environment due to anthropogenic activity or natural geological processes. Cadmium is a metal which, due to its high concentrations in air, water, and soil, rapid transport in the soil-plant-animal trophic chain, and high toxicity, is considered to be one of the most dangerous to both aquatic and terrestrial organisms (Descamps et al. Citation1996; Satarug et al. Citation2003; Wang et al. Citation2013; Stalmach et al. Citation2015; Tarnawska et al. Citation2019). It affects different levels of body organization: from organs through tissues and cells to organelles and all the compounds synthesized (Vega et al. Citation1989; Siekierska & Urbańska-Jasik Citation2002; Siekierska Citation2003; Hödl et al. Citation2010; Babczyńska et al. Citation2011; Takacs et al. Citation2016; Yuan et al. Citation2016; Bednarska et al. Citation2016, Citation2019; Augustyniak et al. Citation2017). Mitochondria are one of the organelles considered to be intracellular targets of cadmium. Thus mitochondrial dysfunction may be due to this metal’s toxicity (Early et al. Citation1992; Miccadei & Floridi Citation1993; Wallace & Starkov Citation2000; Tang & Shaikh Citation2001; Sanni et al. Citation2008; Cannino et al. Citation2009; Hödl et al. Citation2010). Apart from being the main source of ATP, NADH, and GTP, they participate in the biosynthesis of amino acids, phospholipids, and reactive oxygen species (ROS), calcium signaling, oxidative stress, intermediary metabolism, programmed cell death, e.g., apoptosis, and aging (Detmer & Chan Citation2007; Hödl et al. Citation2010; Picard et al. Citation2013; Bratic & Larsson Citation2013; Kühlbrandt Citation2015; Włodarczyk et al. Citation2019). Mitochondria interact with other organelles (e.g., the endoplasmic reticulum, nuclei) and membranes, coordinating their cellular biological functions. When the crosstalk between mitochondria and other organelles fails, and the mitochondria are dysfunctional, cell homeostasis is disrupted. Hence, mitochondrial damage can lead to irreversible cell transformations (Nunnari & Suomalainen Citation2012; Xia et al. Citation2019). Because they play a crucial role in cell physiology (e.g., phosphorylation of ADP, signaling through mitochondrial ROS, calcium signaling, etc.), cell death, and aging, mitochondria are among the first responders to different stressors, including heavy metals (e.g., cadmium) (Hödl et al. Citation2010; Bednarska & Świątek Citation2016).
Previous studies have revealed the ultrastructural changes in mitochondria as well as the changes in the mitochondrial enzyme systems caused by cadmium (Early et al. Citation1992; Tang & Shaikh Citation2001; Cannino et al. Citation2009; Hödl et al. Citation2010; Wang et al. Citation2013). As it has been shown, the damage of the mitochondrial enzyme system by the accumulation of ROS (superoxide, hydrogen peroxide, hydroxyl radical) causes changes in the mitochondrial membrane potential (Koizumi et al. Citation1994; Cannino et al. Citation2009) and activation of antioxidant enzymes, e.g., dismutases (SOD) (Yao et al. Citation2007; Ramalho-Santos et al. Citation2009; Włodarczyk et al. Citation2019). Four classes of SOD enzymes have been distinguished depending on the metal cofactors: Manganese Superoxide dismutase/Mitochondrial Superoxide dismutase (MnSOD), Copper, Zinc Superoxide dismutase (Cu/ZnSOD), Iron Superoxide dismutase (FeSOD), and Nickel Superoxide dismutase (NiSOD) (Zelko et al. Citation2002). Numerous intracellular and extracellular factors can induce MnSOD expression. Hence it is treated as an essential antioxidant enzyme that plays a crucial role in protecting cells against oxidative stress (Holley et al. Citation2010; Sarsour et al. Citation2012; Candas & Li Citation2014). To better understand the impact of cadmium on animals and the processes of cell protection, we decided to analyze mitochondrial alterations caused by short- and long-term cadmium exposure in different organs. Although cadmium is considered as a mitochondria-damaging metal, little is still known how it affects the mitochondria of soil organisms. We chose the well-known and widespread European centipede Lithobius forficatus (Myriapoda, Chilopoda, Lithobiomorpha). Its body has a simple structure and it is an omnivorous species: it is a predator but also feeds on the litter with organic and inorganic matter. Due to the fact that this species plays an important role in soil ecosystems, it seems to be an interesting species in ecotoxicological studies (Eisenbeis & Wichard Citation1987; Ostrowska et al. Citation1991; Lewis Citation2009). Our previous studies on L. forficatus revealed different changes and mechanisms activated after short- and long-term cadmium exposure. Comparing different organs, i.e. the midgut, salivary glands and the fat body at the ultrastructural level, we found that they can react differently to the same stressor, as well as to the same concentration and time of exposure. Eventually, the crosstalk between different cell death types occurs, which could be caused by different mechanisms (Rost-Roszkowska et al. Citation2020a, Citation2020b). Therefore, here we wanted to investigate the precise relationship between the general changes of some organs’ structure and modifications connected with mitochondrial functioning. Due to the lack of data on the effect of heavy metals on various organs, the aim of these studies was to compare mitochondrial changes in somatic cells of different organs (salivary glands, midgut epithelial cells, somatic cells of gonads) and germ-line cells (e.g., oocytes, spermatocytes). The activity of mitochondria has been estimated by detecting the presence of mitochondrial superoxide dismutase (MnSOD), changes in the mitochondrial membrane potential, and their ultrastructure. It enabled us to assess the time-dependent and tissue-dependent differences caused by cadmium exposure.
2. Material and methods
2.1. Material
L. forficatus adults were harvested from May to October from non-polluted yards and forests of southern or central Poland (e.g., Żywiec, Poznań) and adapted to laboratory conditions for several weeks as described by Chajec et al. (Citation2012) and Rost-Roszkowska et al. (Citation2020a, Citation2020b).
2.2. Experiment
Animals were cultured in 30-liter terraria filled with the peat-based soil (room temperature, RT) with the properties described in our previous paper (Rost-Roszkowska et al. Citation2020a). Animals that were bred in cadmium-free soil were the control group in the experiment (C group). They were fed ad libitum with forest litter, detritus, and Chironomus larvae. The remaining animals were bred in soil with the addition of cadmium in the form of CdCl2.
Hence, the animals were divided into experimental groups () according to our previous studies (Rost-Roszkowska et al. Citation2020a, Citation2020b) and literature data (Descamps et al. Citation1996; Vandenbulcke et al. Citation1998a, Citation1998b): Cd1 – animals bred in a soil containing 80 mg Cd kg−1 for 12 days (short-term exposure); Cd2 – animals bred in a soil containing 80 mg Cd kg−1 for 45 days (long–term exposure) (). All the specimens were fed ad libitum as animals from the control group.
Table I. Number of specimens of each sex of L. forficatus examined
The animals were anesthetized with chloroform, and four organs were isolated from their body – the midgut, salivary glands, ovaries, and testis. The organs were prepared for the analysis using histological, histochemical, and immunohistochemical methods described in .
2.3. Methods
2.3.1. Light and transmission electron microscopy (TEM)
Isolated organs (midgut, salivary glands, ovaries, and testis) after fixation with 2.5% glutaraldehyde and 2% osmium tetroxide (4°C, 1.5 h each) were prepared (dehydrated, cut, and stained) according to standard methods used for TEM (Chajec et al. Citation2012; Rost-Roszkowska et al. Citation2020a, Citation2020b) Semi-thin (0.8 µm thick) sections were observed using an Olympus BX60 light microscope, while ultra-thin (70 nm) sections were examined using a Hitachi H500 transmission electron microscope.
2.3.2. Confocal microscopy
JC1 (5,5ʹ,6,6ʹ-tetrachloro-1,1ʹ,3,3ʹ-tetraethyl-benzimidazolyl-carbocyanine iodide) staining. JC-1 is commonly used to differentiate cells with polarized mitochondria (orange fluorescence) and a depolarized mitochondria (green fluorescence) (Salvioli et al. Citation1997). The isolated organs () were stained with JC-1 dye and DAPI, according to Włodarczyk et al. (Citation2017) and visualized using an Olympus FluoView FV1000 confocal microscope.
Dihydroethidium (DHE) – evaluation of reactive oxygen species (ROS) production. Isolated salivary glands, midgut, testis, and ovaries were labeled with DHE and DAPI, as was precisely described by Włodarczyk et al. (Citation2019) and examined with an Olympus FluoView FV1000 confocal microscope.
2.3.3. Fluorescent microscopy
Superoxide dismutase (SOD) detection. The isolated organs (midgut, salivary glands, ovaries, testis) without fixation were embedded in a tissue-freezing medium (Jung) and cryocut (5 μm thick sections). Then, the slides were blocked with BSA and incubated with the primary antibody: anti-MnSOD rabbit polyclonal antibody (1:500; Stressgen), goat anti-rabbit IgG secondary antibody conjugated with Alexa Fluor 488 (1:1000, Invitrogen) and DAPI according to protocols described by Włodarczyk et al. (Citation2019) and eventually analyzed with an Olympus BX-60 fluorescence microscope.
2.3.4. Western blot analysis
Individuals of L. forficatus from the control group were anesthetized, midguts were dissected (5 per sample), and eventually, total protein concentration was measured according to the Bradford method (Citation1976) and previously described in our paper (Włodarczyk et al. Citation2019). The detection of superoxide dismutase (MnSOD) was performed. The Western blot analysis was performed according to protocol described in our previous study (Włodarczyk et al. Citation2019).
2.3.5. Flow cytometry
The four organs isolated from each experimental group’s specimens were mechanically fragmented with scissors and homogenized for obtaining the cell suspension (Włodarczyk et al. Citation2019). In the case of gonads, these organs were examined without distinguishing between somatic and germ cells because flow cytometry does not differentiate cell types.
Muse Oxidative Stress Kit (Merck Millipore, № MCH100111) was used for the quantitative analysis of cell populations undergoing oxidative stress - ROS negative (live cells) and ROS positive cells (cells exhibiting ROS) were distinguished (Włodarczyk et al. Citation2019). The measurements were performed using the Muse Cell Analyzer (Millipore).
JC-1 – quantitative assessment of cells with depolarized mitochondria. The cell suspension was incubated with JC-1 solution according to Włodarczyk et al. (Citation2017) and analyzed using flow cytometry (Beckman Coulter Instrument FC 500) with a 488 nm argon laser using the MXP software Beckman Coulter program. The results were described as the percentage of cells with depolarized mitochondria.
2.3.6. Statistical analyses
Statistical elaboration (the Shapiro–Wilk test, Levene’s test of equality of error variances, the Tukey test; N = 5–6, p < 0.05) was accomplished with STATISTICA 13 (software package system, version 13.0, http://www.statsoft.com) (Wilczek et al. Citation2019).
3. Results
3.1. Populations of cells undergoing oxidative stress
The use of dihydroethidium (DHE) for L. forficatus salivary glands, midgut, ovaries, and testis has shown a diverse distribution of ROS in all experimental groups. Weak signals originated from some of the cells in all organs in the control group. The signals from midgut cells were stronger than in the remaining organs in all experimental groups. The signals emitted by cells of salivary glands, midgut, and ovaries were stronger in Cd1 and Cd2 experimental groups than in the animals from C group. However, the signals that originated from the testis of animals treated with cadmium for 12 days were the strongest in comparison to C and Cd2 groups ().
Figure 2. 3D representation of the DHE and DAPI staining of organs in L. forficatus. ROS-positive cells (red), nuclei (n, blue). Confocal microscope. (a) salivary glands in the C group. Scale bar = 16 µm. (b) salivary glands in the Cd1 group. Scale bar = 12 µm. (c) salivary glands in the Cd2 group. Scale bar = 12 µm. (d) midgut in the C group. Scale bar = 20 µm. (e) midgut in the Cd1 group. Scale bar = 20 µm. (f) midgut in the Cd2 group. Scale bar = 20 µm. (g) ovaries in the C group. Scale bar = 18 µm. (h) ovaries in the Cd1 group. Scale bar = 16 µm. (i) ovaries in the Cd2 group. Scale bar = 16 µm. (j) testis in the C group. Scale bar = 12 µm. (k) testis in the Cd1 group. Scale bar = 12 µm. (l) testis in the Cd2 group. Scale bar = 10 µm
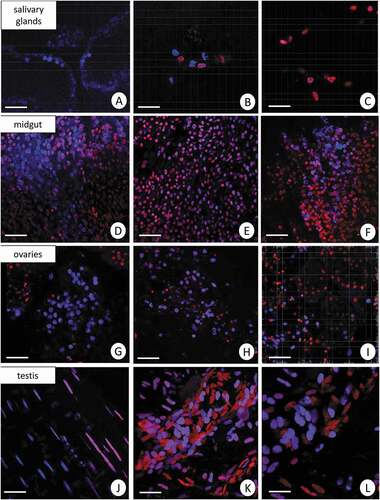
The quantitative analysis revealed that prolonging exposure to cadmium increased the percentage of ROS+ cells in analyzed organs, compared to the control group. Independently of organ, the highest number of ROS+ cells was recorded in individuals from the Cd2 group (salivary glands – 26.9 ± 2.0; midgut – 45.3 ± 2.1; ovaries – 33.3 ± 1.9; testis – 29.2 ± 3.4). The ROS+ percentage in the Cd2 group was almost twice as high as in the Cd1 group. Only in the testis were no statistically significant differences in the number of ROS+ and ROS- cells between the Cd1 and Cd2 groups ().
Figure 3. Percentage of ROS+ and ROS– cells (x± SD) in salivary glands (a), midgut (b), ovaries (c) and testis (d) of L. forficatus individuals from the control group (C) and exposed to metals (Cd1 and Cd2 groups). The different letters (a, b, c) indicate significant differences within each organ and parameter (Tukey test, p < 0.05; N = 5–6)
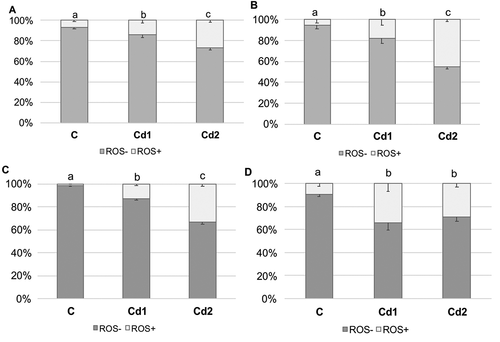
3.2. Ultrastructure of mitochondria
Mitochondria in all organs of the control group have an electro-dense matrix and distinct mitochondrial cristae. The ultrastructure of the mitochondria in salivary glands in both experimental groups Cd1 and Cd2, showed no alterations in comparison to the control group (). However, the most striking difference was the structural transformation of the mitochondria in the midgut digestive cells in the Cd1 group. The matrix of several mitochondria became electron-lucent, and the inner mitochondrial membranes are mainly smooth, and only some short cristae could be detected. However, numerous mitochondria exhibited structural alterations because they had vacuoles engulfing the nearby cytoplasm. The ultrastructure of mitochondria in animals originating from the Cd2 experimental group resembled that of the control group: normal-shaped mitochondria showed numerous cristae and an electron-opaque matrix (). No changes at the ultrastructural level were detected in the secretory or regenerative cells of the midgut epithelium. The transmission electron microscopy enabled us to distinguish two types of cells: the somatic and germ cells in both gonads (testis and ovaries); therefore, we could describe the differences between these two groups of cells. The ultrastructure of mitochondria in somatic cells of both gonads showed similar alterations to the midgut: changes appeared in mitochondria of the Cd1 experimental group. In contrast, in specimens from Cd2 experimental group, the mitochondria resembled those of the control animals (not shown). A similar relationship was detected after long-term cadmium exposure, and only in the germ cells of ovaries (e.g., oocytes) and testis (e.g., spermatocytes) several altered mitochondria were detected in the Cd1 experimental group ().
Figure 4. Transmission electron micrographs presenting the mitochondria in salivary glands (a–c), midgut (d–f), ovaries (g–i) and testis (j–l). Mitochondria (m), glycogen granules (g), nuclei (n), reserve material (rm). TEM. (a) C group. Scale bar = 2 µm. (b) Cd1 group. Scale bar = 1.2 µm. (c) Cd2 group. Scale bar = 2 µm. (d) C group. Scale bar = 2 µm. (e) Cd1 group. Scale bar = 1.2 µm. (f) Cd2 group. Scale bar = 2 µm. (g) C group. Scale bar = 1.1 µm. (h) Cd1 group. Scale bar = 1.6 µm. (i) Cd2 group. Scale bar = 1.3 µm. (j) C group. Scale bar = 1.5 µm. (k) Cd1 group. Scale bar = 1.4 µm. (l) Cd2 group. Scale bar = 1.1 µm
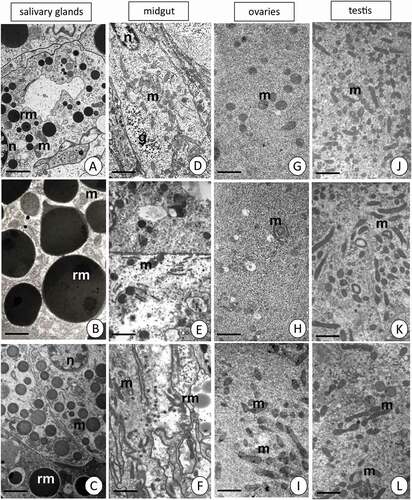
3.3. Mitochondrial membrane potential
The qualitative analysis using confocal microscopy revealed that the signals originating from active mitochondria with a high membrane potential (red signals) were strong in the midgut epithelium and salivary glands in specimens from the control group, but the midgut epithelium presented a more enormous amount of active mitochondria in comparison to salivary glands. In both organs, the signals originating from active mitochondria were weaker in Cd1 and Cd2 experimental groups. In contrast, numerous strong signals originating from inactive mitochondria with a low membrane potential (green signals) were detected in the control group (). In general, the midgut seems to be an organ with a much more considerable amount of active/non-active mitochondria. The confocal microscope enabled somatic and germ cells to be distinguished. We observed the differences in signals emitted by active and non-active mitochondria in animals from all experimental groups. Both cell types, the somatic and germ cells, in testis and ovaries showed a high number of active mitochondria (red signals), while sporadic green signals were emitted by the non-active mitochondria of germ cells (). After short-term cadmium treatment, the number of non-active mitochondria strongly increased in both types of cells in ovaries, resulting in an increase of green signals emitted by non-active mitochondria. Long-term cadmium exposure led to an increased number of active mitochondria in the somatic cells of the ovary (red signals). In contrast, signals emitted by non-active mitochondria were mainly detected in germ cells (). In the testis, the short-term cadmium exposure caused a slight increase in the number of non-active mitochondria, which emitted green signals in somatic cells. The germ cells still emitted green signals originating from non-active mitochondria. After 45 days of cadmium treatment, both the somatic and germ cells emitted only green signals originating from non-active mitochondria ().
Figure 5. Mitochondrial potential in the L. forficatus organs. Active mitochondria with a high membrane potential (red signals), inactive mitochondria with a low membrane potential (green signals), nuclei (blue signals). JC–1 cationic dye, DAPI staining. Confocal microscope. (a) salivary glands in the C group. Scale bar = 16 µm. (b) salivary glands in the Cd1 group. Scale bar = 16 µm. (c) salivary glands in the Cd2 group. Scale bar = 20 µm. (d) midgut in the C group. Scale bar = 12 µm. (e) midgut in the Cd1 group. Scale bar = 16 µm. (f) midgut in the Cd1 group. Scale bar = 16 µm. (g) ovaries in the C group. Scale bar = 12 µm. (h) ovaries in the Cd1 group. Scale bar = 10 µm. (i) ovaries in the Cd2 group. Scale bar = 10 µm. (j) testis in the C group. Scale bar = 16 µm. (k) testis in the Cd1 group. Scale bar = 16 µm. (l) testis in the Cd2 group. Scale bar = 10 µm
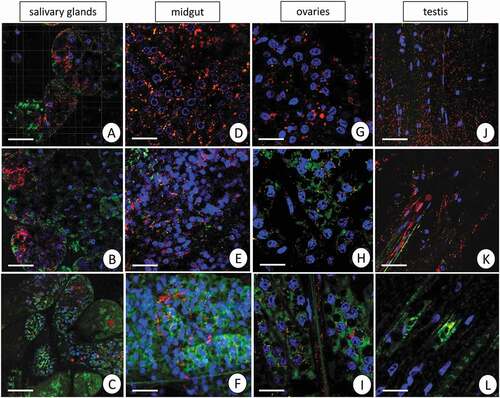
The quantitative analysis showed that independent of time exposure to cadmium, an increase in the number of cells with depolarized mitochondria was detected in analyzed organs compared to the control group ()). An almost threefold increase in the number of cells with low mitochondrial transmembrane potential was detected in salivary glands (P = 0.03) ()). In contrast, a fourfold increase in the ovary (P = 0.04) and a sevenfold increase in testis (P = 0.0002) were found (). In turn, 75% more cells with depolarized mitochondria were registered in the intestine of individuals exposed to cadmium for 45 days (P = 0.03; Cd2 group) ()) than the control group. Independently of organ, there was no statistically significant difference in the number of cells with depolarized mitochondria between the Cd1 and Cd2 experimental groups.
Figure 6. Percentage of cells with depolarized mitochondria (low ∆Ψm) in salivary glands (a), midgut (b), ovaries (c) and testis (d) of individuals from the control group and exposed to metals (Cd1 and Cd 2 groups). The different letters (a, b) significance differences within each organ (Tukey test, p < 0.05; N = 4–7)
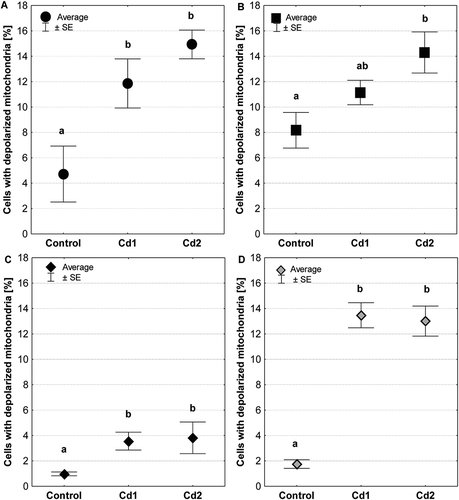
3.4. MnSOD activity
The specificity of the antibodies was confirmed by the Western blot technique (). The immunofluorescent method for detecting superoxide dismutase (MnSOD) at the light microscope level revealed a low level of this enzyme in the midgut epithelial cells and salivary glands in the control group of L. forficatus. Short-term cadmium exposure activates MnSOD expression, but a more enormous amount of MnSOD was detected after long-term Cd exposure (). However, in the case of gonads, we observed that germ cells emitted more signals originating from MnSOD localization in comparison to somatic cells. These signals were the strongest in ovaries and testis of animals from the Cd2 experimental group ().
Figure 7. Western blot analysis of Superoxide dismutase (MnSOD) in the L. forficatus intestine (25 µg of protein per each line)
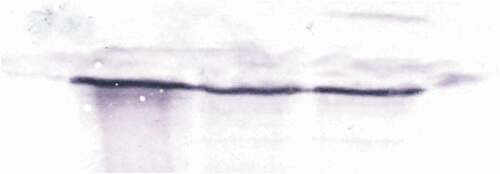
Figure 8. A fluorescent representation of the MnSOD localization (green) and DAPI staining in salivary glands, midgut, ovaries and testis of L. forficatus. Nuclei (blue). Fluorescent microscope. (a) salivary glands in the C group. Scale bar = 16 µm. (b) salivary glands in the Cd1 group. Scale bar = 16 µm. (c) salivary glands in the Cd2 group. Scale bar = 16 µm. (d) midgut in the C group. Scale bar = 24 µm. (e) midgut in the Cd1 group. Scale bar = 26 µm. (f) midgut in the Cd2 group. Scale bar = 26 µm. (g) ovaries in the C group. Scale bar = 26 µm. (h) ovaries in the Cd1 group. Scale bar = 16 µm. (i) ovaries in the Cd2 group. Scale bar = 16 µm. (j) testis in the C group. Scale bar = 24 µm. (k) testis in the Cd1 group. Scale bar = 20 µm. (l) testis in the Cd2 group. Scale bar = 18 µm
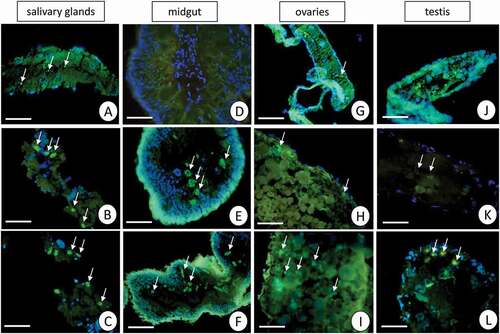
4. Discussion
Free radicals are derived from enzymatic and nonenzymatic reactions that naturally occur in cells or can be caused by many environmental factors. They are involved in different physiological processes, that enable proper cellular functioning, including regulating of many intercellular signaling transduction or activation of the immune system. In the case of the reproductive system, they are involved in gonads’ and germ cells’ development and differentiation, as well as in fertilization. Their level determines cell functioning. When the ROS level is low, the cell remains in a quiescent state (Neckameyer & Matsuo Citation2008; Zhou et al. Citation2014; Lyublinskaya et al. Citation2015; Dziewięcka et al. Citation2017). The loss of homeostasis between ROS synthesis and their elimination activates the phenomenon called oxidative stress (Golden et al. Citation2002; Martindale & Holbrook Citation2002; Stalmach et al. Citation2015; Redza-Dutordoir & Averill-Bates Citation2016; Ahmad et al. Citation2017; Włodarczyk et al. Citation2019; Tarnawska et al. Citation2019). Animals have developed numerous defense mechanisms responsible for the neutralization of free radicals in cells and consequently, regulating the functioning of cells. This antioxidant defense system is composed of antioxidants, e.g., dismutases (e.g., MnSOD), catalase, glutathione, or cytochrome c released from mitochondria (Luo Citation2001; Babczyńska et al. Citation2011; Kaminskyy & Zhivotovsky Citation2014; Suganya et al. Citation2016; Augustyniak et al. Citation2016, Citation2017; Oropesa et al. Citation2017; Haque et al. Citation2018; Włodarczyk et al. Citation2019).
Toxic effects of cadmium are connected with its dose, period of exposure, the environment, and the age or developmental stage of animals (Neckameyer & Matsuo Citation2008; Liu et al. Citation2009; Babczyńska et al. Citation2011; Bednarska & Świątek Citation2016). Our research on the terrestrial centipede, which lives in soil polluted with high cadmium contamination, revealed that the presence of this heavy metal in the environment, not in the animal’s food, can cause an increase in the level of ROS in somatic cells (here the salivary glands and midgut epithelium). The low level of ROS in analyzed organs in the control group related to natural physiological processes, while it gradually increased due to the prolongation of cadmium exposure. As it is known, the middle region of the digestive system – the midgut – is one of the barriers for the organism against external stressors (Leonard et al. Citation2009; Janeh et al. Citation2019). The relationship between oxidative stress and MnSOD activation has been registered in the midgut epithelium in various invertebrates (Zhang et al. Citation2007; Yu et al. Citation2011; Suganya et al. Citation2016; Oropesa et al. Citation2017; Haque et al. Citation2018; Włodarczyk et al. Citation2019). The low level of MnSOD in the midgut epithelial cells and salivary glands in the control group of L. forficatus suggests that the level of ROS is probably connected with physiological processes. The increase in the number of signals emitted by MnSOD in these organs after short- and long-term cadmium exposure shows its activation as the response to a higher ROS level. However, while in the salivary glands and midgut epithelium our study confirms earlier data, the information connected with gonads and germ-line cells is rather poor in animals living in soil contaminated with toxic metals. Our study showed that while the ovaries presented the same phenomenon in the increase of the ROS level connected with periods of cadmium exposure, the testis emitted stronger signals of ROS accumulation after short-term cadmium exposure in comparison to long-term exposure. Up to now, similar studies have been presented for the female reproductive system, when ROS have been associated with its pathologies, because they were mainly detected in the somatic cells of ovaries (e.g., ovarian follicles) or reproductive ducts (e.g., the fallopian tube) (Agarwal et al. Citation2005). Higher than physiological levels of ROS activate the apoptosis of granulosa cells and cause the reduction of nutrient transfer to oocytes (Ahmad et al. Citation2017; Khazaei & Aghaz Citation2017). In testis, ROS are generated by mitochondria in germ-line cells whose cytoplasm is rich in these organelles because they constantly require ATP for motility and development (Griveau & Le Lannou Citation1997; Ahmad et al. Citation2017) but also for their ability to fertilize (Pelliccione et al. Citation2011; Sousa et al. Citation2011). When the ATP is required in male germ-line cells, the concentration of molecular oxygen in the mitochondria increases, leading to intensive activation of the antioxidant defense system (Said et al. Citation2005; Małota et al. Citation2019). We observed that in both gonads – testis and ovaries – of L. forficatus germ cells emitted more signals originating from MnSOD localization in comparison to somatic cells. These signals were the strongest in ovaries and testis of animals from the Cd2 experimental group, suggesting that MnSOD as a defense enzyme has been strongly synthesized in these cells compared to the somatic cells of gonads. In the terrestrial earthworm Dendrobaena veneta, the different ROS distribution in different types of cells in ovaries has been observed. The level of ROS was lower in somatic cells than in e.g. younger germ-line cells and post-vitellogenic oocytes. The level of MnSOD corresponds to ROS accumulation in this species. A high level of MnSOD activity has been noted in germ-line cells in comparison to somatic cells of the ovary (Faron et al. Citation2015). Generally, it is suggested that germ-line cells in ovaries of invertebrates should be more active metabolically than somatic cells (Tourmente et al. Citation1990; Świątek et al. Citation2001, Citation2004). However, in the testis, the number of germ cells is higher than in the ovaries, explaining the higher signals originating from MnSOD and ROS in the male gonads. The high level of ROS in the testis of the centipede from the Cd1 experimental group in comparison to the control and Cd2 groups probably is related to the fact that MnSOD is strongly involved in sperm protection causing the reduction of ROS in these cells after long-term cadmium exposure. This mechanism must occur much faster and more strongly than in the case of ovaries. We can conclude that in testis, the oxidative stress occurs after the short-term heavy metal exposure, while in ovaries, salivary glands, and midgut epithelium, it is activated after 12 days of cadmium exposure, but a longer period is needed for the proper functioning of antioxidative defense. In addition, this system works more efficiently in the case of gonads.
Mitochondrial dysfunction is the mechanism of cytotoxicity of cadmium because Cd affects the mitochondrial morphology (Miccadei & Floridi Citation1993; Wallace & Starkov Citation2000; Sokolova Citation2004; Hödl et al. Citation2010). Both the short- and long-term cadmium exposure of adult specimens of soil centipede did not cause any ultrastructural alterations of mitochondria in salivary glands, even though the number of non-active organelles with low mitochondrial potential increased after short- and long-term cadmium exposure. Measurements of the transmembrane mitochondrial potential (ΔΨm) are considered as markers of all alterations that lead to cell death before any visible changes occur (Orrenius Citation2004; Zorova et al. Citation2018). The most striking differences were noted in the midgut epithelium in L. forficatus after short-term cadmium exposure. Numerous mitochondria showed signs of degeneration, and it was followed by an increase in the number of non-active mitochondria. It is suggested that mitochondrial dysfunction occurs according to inhibition or transformation of the respiratory complex rather than deletions of mitochondrial DNA (Siskova et al. Citation2010). The abnormalities in mitochondrial morphology, including abnormal cristae, their vacuolization, and disruption of the mitochondrial membranes are connected with an increase of ROS levels, while the overexpression of MnSOD decreases their level together with the preservation of mitochondrial morphology (DeMartino et al. Citation1979; Faron et al. Citation2015). As we mentioned above, MnSOD in the Cd1 group was detected at a very low level. However, after long-term cadmium exposure (Cd2), the ultrastructure of these organelles in the midgut epithelium resembles that of the control group. It corresponds with a higher amount of MnSOD after long-term metal exposure and the higher ROS level. The increase in the number of mitochondria with low mitochondrial potential after long-term cadmium exposure suggests that their ultrastructure has been regenerated, although they are still not active. A similar process has been described in freshwater shrimp midgut epithelium (Włodarczyk et al. Citation2019).
Changes in both mitochondrial ultrastructure and mitochondrial potential could be associated with the activation of cell death (Kaminskyy & Zhivotovsky Citation2014; Redza-Dutordoir & Averill-Bates Citation2016; Sonakowska et al. Citation2016; Włodarczyk et al. Citation2017) because mitochondria play an essential role in the activation of cell death (Orrenius Citation2004). The inner-membrane structural alterations and morphological changes of mitochondria have been implicated in processes connected with programmed cell death and as a response to oxidative stress in different tissues and organs (Mannella Citation2008). Our previous studies on L. forficatus revealed that short-term Cd exposure activates autophagy in both the salivary glands and the midgut epithelium. In contrast, after the long-term metal exposure, this process is inhibited, while cell death (both necrosis and apoptosis) is involved in the response against the stressor (Rost-Roszkowska et al. Citation2020a, Citation2020b). The relationship between cadmium exposure and autophagy has been studied mainly in mammalian cells and tissues (Luo et al. Citation2016), but such a correlation has also been detected in invertebrates (Bednarska et al. Citation2016; Chiarelli et al. Citation2016; Wilczek et al. Citation2019). Comparing the present study with the previous one, we can state that when autophagy protects cells against cell death, the mitochondrial dismutase is not activated. Activation of cell death (here apoptosis and necrosis) corresponds to the intensive synthesis of MnSOD in both organs– the salivary glands and the midgut epithelium. The different response to the stressor suggests that the midgut epithelium is more sensitive to heavy metal presence than the salivary glands. Damage of mitochondria occurs differently in organs with different regenerative abilities (Dai et al. Citation2009; Velasquez-Vottelerd et al. Citation2015; Brandt et al. Citation2017). Additionally, it confirms the statement that the midgut epithelium as the middle region of the digestive system is considered one of the barriers in the organism against any stressors (Leonard et al. Citation2009). Eventually we could state that changes in mitochondrial ultrastructure caused by cadmium are tissue-dependent.
The quantitative analysis showed a distinct increase in the number of depolarized (non-active) mitochondria in ovaries and testis of L. forficatus after short- and long-term cadmium exposure. However, the confocal microscope enabled us to distinguish somatic and germ cells. The results showed that mostly red fluorescence originated from the active mitochondria in somatic cells, and green fluorescence was emitted by depolarized mitochondria in the germ-line cells of both gonads. The activity of mitochondria in somatic cells measured by red fluorescence has been recorded in the Dendrobaena veneta ovary. The germ-line cells in this species presented the lower oxidative activity of mitochondria (Faron et al. Citation2015). Similar results were obtained for Xenopus laevis during the formation of vitellogenic and post-vitellogenic oocytes (Mignotte et al. Citation1987; Kogo et al. Citation2011). It has been concluded that the level of mitochondrial activity is low in germ-line cells of animals (Tarazona et al. Citation2006; Kogo et al. Citation2011; Faron et al. Citation2015). In Danio rerio, the number of active and non-active mitochondria was equal in both types of cells (Zhang et al. Citation2008) or depending on the stage of oogenesis, the germ-line cells could be completely inactive (Tarazona et al. Citation2006; Kogo et al. Citation2011). The level of mitochondrial activity in the germ-line cysts during spermatogenesis in D. veneta was low, and the percentage of polarized mitochondria decreased in consecutive spermatogenesis stages (Małota et al. Citation2019). The somatic cells’ main role in invertebrates gonads is the protection and support of germ-line cells, the synthesis and secretion of the material for the egg shells and hormonal regulation of oogenesis (Adiyodi & Adiyodi Citation1983a; Poprawa et al. Citation2002; Poprawa & Janelt Citation2019). Sometimes they participate in vitellogenesis (Telfer et al. Citation1982). In the testis, somatic cells take part in the separation of the developing group of germ cells, the nutritional support of the germ cells, the hormonal regulation of the spermatogenesis, and the secretion of the material for spermatophore (Adiyodi & Adiyodi Citation1983b; Minelli Citation2011). Therefore, the active state of mitochondria in somatic cells in both gonads of L. forficatus is connected with their functions.
The short-term cadmium treatment of soil centipedes caused an increase in the number of non-active mitochondria in somatic and germ-line cells in both gonads. However, the signals emitted were stronger in the case of ovaries than in testis. However, the changes were different after long-term cadmium exposure. In the testis, both the somatic and germ cells emitted only green signals originating from non-active mitochondria, while an increase in the number of active mitochondria in the somatic cells was detected in ovaries. Regardless of the transmission electron microscopy, the results suggest that in both somatic and germ-line cells, the alterations connected with e.g. vacuolization, only after the short-term cadmium exposure occurred. The long-term cadmium treatment did not alter the mitochondrial ultrastructure. Different periods of cadmium exposure can cause changes in respiratory activity followed by alterations in mitochondrial membrane potential (Cannino et al. Citation2008, Citation2009), deflecting mitochondrial membrane potential (Koizumi et al. Citation1994), and organelles bulging (Al-Nasser Citation2000). The early mitochondrial swelling and vacuolization seemed to be typical for Cd exposure, suggesting an obstruction of the oxidative metabolism (Ossola & Tomaro Citation1995). Because changes in mitochondrial ultrastructure and mitochondrial potential could be associated with activation of cell death (Orrenius Citation2004; Sonakowska et al. Citation2016; Włodarczyk et al. Citation2017), the analysis of cell death in gonads of L. forficatus exposed to cadmium is necessary. Additionally, the results connected with changes of mitochondria in gonads confirm our previous statement that mitochondria are still not active as after long-term Cd treatment, and their ultrastructure has been regenerated (Włodarczyk et al. Citation2019). Thus we suspect that additional mechanisms must be involved in processes related to mitochondrial activity, not only changes in their membrane potential and ultrastructural alterations.
5. Conclusions
Our research is the first in which changes in mitochondria between somatic cells of organs belonging to the digestive system, somatic cells in gonads and germ-line cells were compared according to cadmium presence in the environment of soil-dwelling organisms. We observed that: (1) abnormalities in mitochondrial morphology including abnormal cristae, their vacuolization and disruption of the mitochondrial membranes in all organs are connected with an increase of ROS levels; (2) changes in mitochondrial ultrastructure caused by cadmium are tissue-dependent; (3) oxidative stress strongly occurs after short-term metal exposure in testis, while in ovaries, salivary glands and midgut epithelium it is activated after short-term cadmium exposure, but a more extended period is needed for the proper functioning of antioxidative defense; (4) the system of ROS and MnSOD activation works more efficiently in the case of gonads than in the digestive system; (5) while the short-term cadmium exposure alters the ultrastructure of both the somatic and germ-line cells in ovaries and testis, the long-term Cd exposure causes the activation of mechanisms which enable the mitochondrial ultrastructure to regenerate; (6) the active state of mitochondria in somatic cells and non-active state of mitochondria in germ-line cells in both gonads in the non-treated animals are connected with their functions and are a common phenomenon. Additionally, comparing the results from this study and the previous one, we can conclude that when autophagy protects cells against cell death, the mitochondrial dismutase is not activated. Activation of cell death corresponds to the intensive synthesis of MnSOD in both the salivary glands and the midgut epithelium. An analysis of cell death processes in the gonads should be performed under experimental conditions to ensure this statement.
Ethical approval
All applicable international, national, and/or institutional guidelines for the care and use of animals were followed. This article does not concern any studies with human participants that were performed by any of the authors.
Acknowledgements
We are very thankful to Dr. Danuta Urbańska-Jasik (the University of Silesia in Katowice, Poland) for her technical assistance and Richard Ashcroft for the language correction.
Disclosure statement
No potential conflict of interest was reported by the author(s).
Additional information
Funding
References
- Adiyodi KG, Adiyodi RG. 1983a. Reproductive biology of invertebrates. Volume I. Oogenesis, oviposition, and oosorption. Chichester, UK: John Wiley & Sons Ltd.
- Adiyodi KG, Adiyodi RG. 1983b. Reproductive biology of invertebrates. Volume II. Spermatogenesis and sperm function. Chichester, UK: John Wiley & Sons Ltd.
- Agarwal A, Gupta S, Sharma RK. 2005. Role of oxidative stress in female reproduction. Reproductive Biology and Endocrinology 3(1):28. DOI: 10.1186/1477-7827-3-28.
- Ahmad G, Almasr M, Dhillon AS, Abuayyash MM, Kothandaraman N, Cakar Z. 2017. Overview and sources of reactive oxygen species (ROS) in the reproductive system. In: Agarwal A, Sharma R, Gupta S, Harlev A, Ahmad G, du Plessis SS, Esteves SC, Wang SM, Durairajanayagam D, editors. Oxidative stress in human reproduction. Cham, Switzerland: Springer. pp. 1–16.
- Al-Nasser IA. 2000. Cadmium hepatotoxicity and alterations of the mitochondrial function. Journal of Toxicology: Clinical Toxicology 38(4):407–413. DOI: 10.1081/clt-100100950.
- Augustyniak M, Płachetka-Bożek A, Kafel A, Babczyńska A, Tarnawska M, Janiak A, Dziewięcka M, Karpeta-Kaczmarek J, Zawisza-Raszka A. 2016. Phenotypic plasticity, epigenetic or genetic modifications in relation to the duration of Cd-exposure within a microevolution time range in the beet armyworm. PLoS One 11(12):e0167371. DOI: 10.1371/journal.pone.0167371.
- Augustyniak M, Tarnawska M, Babczyńska A, Kafel A, Zawisza-Raszka A, Adamek B, Płachetka-Bożek A. 2017. Cross tolerance in beet armyworm: Long-term selection by cadmium broadens tolerance to other stressors. Ecotoxicology 26(10):1408–1418. DOI: 10.1007/s10646-017-1865-5.
- Babczyńska A, Wilczek G, Wilczek P, Szulińska E, Witas I. 2011. Metallothioneins and energy budget indices in cadmium and copper exposed spiders Agelena labyrinthica in relation to their developmental stage, gender and origin. Comparative Biochemistry and Physiology 154(3):161–171. DOI: 10.1016/j.cbpc.2011.05.001.
- Bednarska AJ, Laskowski R, Pyza E, Semik D, Świątek Z, Woźnicka O. 2016. Metal toxicokinetics and metal-driven damage to the gut of the ground beetle Pterostichus oblongopunctatus. Environmental Science and Pollution Research 23(21):22047–22058. DOI: 10.1007/s11356-016-7412-8.
- Bednarska AJ, Świątek Z. 2016. Subcellular partitioning of cadmium and zinc in mealworm beetle (Tenebrio molitor) larvae exposed to metal-contaminated flour. Ecotoxicology and Environmental Safety 133:82–89. DOI: 10.1016/j.ecoenv.2016.06.033.
- Bednarska AJ, Świątek ZM, Łabęcka AM. 2019. Effects of cadmium bioavailability in food on its distribution in different tissues in the ground beetle Pterostichus oblongopunctatus. Bulletin of Environmental Contamination and Toxicology 103(3):421–427. DOI: 10.1007/s00128-019-02679-x.
- Bradford MM. 1976. A rapid and sensitive method for the quantitation of microgram quantities of protein utilizing the principle of protein-dye binding. Analytical Biochemistry 72(1–2):248–254. DOI: 10.1016/0003-2697(76)90527-3.
- Brandt T, Mourier A, Tain LS, Partridge L, Larsson NG, Kühlbrandt W. 2017. Changes of mitochondrial ultrastructure and function during ageing in mice and Drosophila. eLife 6:e24662. DOI: 10.7554/eLife.24662.
- Bratic A, Larsson NG. 2013. The role of mitochondria in aging. The Journal of Clinical Investigation 123(3):951–957. DOI: 10.1172/JCI64125.
- Candas D, Li JJ. 2014. MnSOD in oxidative stress response-potential regulation via mitochondrial protein influx. Antioxidants and Redox Signalling 20(10):1599–1617. DOI: 10.1089/ars.2013.5305.
- Cannino G, Ferruggia E, Luparello C, Rinaldi AM. 2008. Effects of cadmium chloride on some mitochondria-related activity and gene expression of human MDA-MB231 breast tumor cells. Journal of Inorganic Biochemistry 102(8):1668–1676. DOI: 10.1016/j.jinorgbio.2008.04.002.
- Cannino G, Ferruggia E, Luparello C, Rinaldi AM. 2009. Cadmium and mitochondria. Mitochondrion 9(6):377–384. DOI: 10.1016/j.mito.2009.08.009.
- Chajec Ł, Rost-Roszkowska MM, Vilimova J, Sosinka A. 2012. Ultrastructure and regeneration of midgut epithelial cells in Lithobius forficatus (Chilopoda, Lithobiidae). Invertebrate Biology 131(2):119–132. DOI: 10.1111/j.1744-7410.2012.00264.x.
- Chiarelli R, Martino C, Agnello M, Bosco L, Roccheri MC. 2016. Autophagy as a defense strategy against stress: Focus on Paracentrotus lividus sea urchin embryos exposed to cadmium. Cell Stress & Chaperones 21(1):19–27. DOI: 10.1007/s12192-015-0639-3.
- Dai DF, Santana LF, Vermulst M, Tomazela DM, Emond MJ, MacCoss MJ, Gollahon K, Martin GM, Loeb LA, Ladiges WC, et al. 2009. Overexpression of catalase targeted to mitochondria attenuates murine cardiac aging. Circulation 119(21):2789–2797. DOI: 10.1161/CIRCULATIONAHA.108.822403.
- DeMartino C, Floridi A, Marcante ML, Malorni W, Barcellona PS, Bellocci M, Silvestrini B. 1979. Morphological, histochemical and biochemical studies on germ cell mitochondria of normal rats. Cell and Tissue Research 196(1):1–22. DOI: 10.1007/BF00236345.
- Descamps M, Fabre MC, Grelle C, Gerard S. 1996. Cadmium and lead kinetics during experimental contamination and decontamination of the centipede Lithobius forficatus L. Archives of Environmental Contamination and Toxicology 31(3):350–353. DOI: 10.1007/BF00212673.
- Detmer SA, Chan DC. 2007. Functions and dysfunctions of mitochondrial dynamics. Nature Reviews. Molecular Cell Biology 8(11):870–879. DOI: 10.1038/nrm2275.
- Dziewięcka M, Karpeta-Kaczmarek J, Augustyniak M, Rost-Roszkowska M. 2017. Short-term in vivo exposure to graphene oxide can cause damage to the gut and testis. Journal of Hazardous Materials 328:80–89. DOI: 10.1016/j.jhazmat.2017.01.012.
- Early JL, Nonavinakere VK, Weaver A. 1992. Effect of Cd and/or selenium on liver mitochondria and rough endoplasmic reticulum in the rat. Toxicological Letters 62(1):73–83. DOI: 10.1016/0378-4274(92)90080-4.
- Eisenbeis G, Wichard W. 1987. Atlas on the biology of soil arthropods. Berlin, Germany: Springer-Verlag.
- Faron J, Bernaś T, Sas-Nowosielska H, Klag J. 2015. Analysis of the behavior of mitochondria in the ovaries of the earthworm Dendrobaena veneta Rosa 1839. PLoS One 10(2):e0117187. DOI: 10.1371/journal.pone.0117187.
- Golden TR, Hinerfeld DA, Melov S. 2002. Oxidative stress and aging: Beyond correlation. Aging Cell 1(2):117–123. DOI: 10.1046/j.1474-9728.2002.00015.x.
- Griveau JF, Le Lannou D. 1997. Reactive oxygen species and human spermatozoa: Physiology and pathology. International Journal of Andrology 20(2):61–69. DOI: 10.1046/j.1365-2605.1997.00044.x.
- Haque MN, Lee DH, Kim BM, Nam SE, Rhee JS. 2018. Dose- and age-specific antioxidant responses of the mysid crustacean Neomysis awatschensis to metal exposure. Aquatic Toxicology 201:21–30. DOI: 10.1016/j.aquatox.2018.05.023.
- Hödl E, Felder E, Chabicovsky M, Dallinger R. 2010. Cadmium stress stimulates tissue turnover in Helix pomatia: Increasing cell proliferation from metal tolerance to exhaustion in molluscan midgut gland. Cell and Tissue Research 341(1):159–171. DOI: 10.1007/s00441-010-0980-x.
- Holley AK, Xu Y, St Clair DK, St Clair WH. 2010. RelB regulates manganese superoxide dismutase gene and resistance to ionizing radiation of prostate cancer cells. Annals of NY Academy of Science 1201(1):129–136. DOI: 10.1111/j.1749-6632.2010.05613.x.
- Janeh M, Osman D, Kambris Z. 2019. Comparative analysis of midgut regeneration capacity and resistance to oral infection in three disease-vector mosquitoes. Scientific Reports 9(1):4556. DOI: 10.1038/s41598-019-50994-4.
- Kaminskyy VO, Zhivotovsky B. 2014. Free radicals in cross talk between autophagy and apoptosis. Antioxidants & Redox Signaling 21(1):86–102. DOI: 10.1089/ars.2013.5746.
- Khazaei M, Aghaz F. 2017. Reactive oxygen species generation and use of antioxidants during in vitro maturation of oocytes. International Journal of Fertility and Sterility 11(2):63–70. DOI: 10.22074/ijfs.2017.4995.
- Kogo N, Tazaki A, Kashimno Y, Morichika K, Orii H, Mochii M, Watanabe K. 2011. Germ-line mitochondria exhibit suppressed respiratory activity to support their accurate transmission to the next generation. Developmental Biology 349(2):462–469. DOI: 10.1016/j.ydbio.2010.11.021.
- Koizumi T, Yokota H, Shirakura H, Tatsumoto H, Suzuki KT. 1994. Potential mechanism of cadmium-induced cytotoxicity in rat hepatocytes: Inhibitory action of cadmium on mitochondrial respiratory activity. Toxicology 92(1–3):115–125. DOI: 10.1016/0300-483X(94)90171-6.
- Kühlbrandt W. 2015. Structure and function of mitochondrial membrane protein complexes. BMC Biology 13(1):89. DOI: 10.1186/s12915-015-0201-x.
- Leonard EM, Pierce LM, Gillis PL, Wood CM, O’Donnell MJ. 2009. Cadmium transport by the gut and malpighian tubules of Chironomus riparius. Aquatic Toxicology 92(3):179–186. DOI: 10.1016/j.aquatox.2009.01.011.
- Lewis JGE. 2009. The food and reproductive cycles of the centipedes Lithobius variegatus and Lithobius forficatus in Yorkshire woodland. Journal of Zoology 144:269–284.
- Liu J, Qu W, Kadiiska MB. 2009. Role of oxidative stress in cadmium toxicity and carcinogenesis. Toxicology and Applied Pharmacology 38(3):209–214. DOI: 10.1016/j.taap.2009.01.029.
- Luo B, Lin Y, Jiang S, Huang L, Yao H, Zhuang Q, Zhao R, Liu H, He C, Lin Z. 2016. Endoplasmic reticulum stress eIF2α–ATF4 pathway-mediated cyclooxygenase-2 induction regulates cadmium-induced autophagy in kidney. Cell Death & Disease 7(6):e2251. DOI: 10.1038/cddis.2016.78.
- Luo J. 2001. Manganese Superoxide dismutase (MnSOD). B-180 medical laboratories. Free radical and radiation biology program. Iowa City, USA: The University of Iowa.
- Lyublinskaya OG, Borisov YG, Pugovkina NA, Smirnova IS, Obidina JV, Ivanova JS, Zenin VV, Shatrova AN, Borodkina AV, Aksenov ND, et al. 2015. Reactive oxygen species are required for human mesenchymal stem cells to initiate proliferation after the quiescence exit. Oxidative Medicine and Cellular Longevity 2015:502105. DOI: 10.1155/2015/502105.
- Małota K, Student S, Świątek P. 2019. Low mitochondrial activity within developing earthworm male germ-line cysts revealed by JC-1. Mitochondrion 44:111–121. DOI: 10.1016/j.mito.2018.01.007.
- Mannella CA. 2008. Structural diversity of mitochondria: Functional implications. Annals of NY Academy of Science 1147(1):171–179. DOI: 10.1196/annals.1427.020.
- Martindale JL, Holbrook NJ. 2002. Cellular response to oxidative stress: Signaling for suicide and survival. Journal of Cell Physiology 192(1):1–15. DOI: 10.1002/jcp.10119.
- Miccadei S, Floridi A. 1993. Sites of inhibition of mitochondrial transport by cadmium. Chemo-Biological Interactions 89(2–3):159–167. DOI: 10.1016/0009-2797(93)90006-K.
- Mignotte F, Tourte M, Mounolou J-C. 1987. Segregation of mitochondria in the cytoplasm of Xenopus vitellogenic oocytes. Biology of the Cell 60(2):97–102. DOI: 10.1111/j.1768-322X.1987.tb00549.x.
- Minelli A. 2011. Chilopoda– Reproduction. In: Minelli A, editor. Treatise on zoology - Anatomy, taxonomy, biology. The Myriapoda, volume 1. Boston, USA: Brill. pp. 279–294.
- Neckameyer WS, Matsuo H. 2008. Distinct neural circuits reflect sex, sexual maturity, and reproductive status in response to stress in Drosophila melanogaster. Neuroscience 156(4):841–856. DOI: 10.1016/j.neuroscience.2008.08.020.
- Nunnari J, Suomalainen A. 2012. Mitochondria: In sickness and in health. Cell 148(6):1145–1159. DOI: 10.1016/j.cell.2012.02.035.
- Oropesa AL, Floro AM, Palma P. 2017. Toxic potential of the emerging contaminant nicotine to the aquatic ecosystem. Environmental Science and Pollution Research 24(20):16605–16616. DOI: 10.1007/s11356-017-9084-4.
- Orrenius S. 2004. Mitochondrial regulation of apoptotic cell death. Toxicological Letters 149(1–3):19–23. DOI: 10.1016/j.toxlet.2003.12.017.
- Ossola JO, Tomaro ML. 1995. Heme oxygenase induction by cadmium chloride: Evidence for oxidative stress involvement. Toxicology 104(1–3):141–147. DOI: 10.1016/0300-483X(95)03157-B.
- Ostrowska A, Gawliński S, Szczubiałka Z. 1991. Methods of analysis and evaluation of soil and plant properties. Catalogue (in Polish). Warsaw, Poland: Institute of Environmental Protection.
- Pelliccione F, Micillo A, Cordeschi G, D’Angeli A, Necozione S, Gandini L, Lenzi A, Francavilla F, Francavilla S. 2011. Altered ultrastructure of mitochondrial membranes is strongly associated with unexplained asthenozoospermia. Fertility and Sterility 95(2):641–646. DOI: 10.1016/j.fertnstert.2010.07.1086.
- Picard M, Shirihai OS, Gentil BJ, Burelle Y. 2013. Mitochondrial morphology transitions and functions: Implications for retrograde signaling? American Journal of Physiology– Regulatory, Integrative and Comparative Physiology 304(6):393–406. DOI: 10.1152/ajpregu.00584.2012.
- Poprawa I, Janelt K. 2019. Reproduction, gonad structure and oogenesis in tardigrades. In: Tworzydło W, Biliński S, editors. Evo-Devo: Non-model species in cell and developmental biology. Switzerland: Springer Nature. pp. 495–513.
- Poprawa I, Baran A, Rościszewska E. 2002. Structure of ovaries and formation of egg envelopes in the stonefly, Leuctra autumnalis Aubert, 1948 (Plecoptera: Leuctridae) ultrastructural studies. Folia Biologica (Kraków) 50:29–38.
- Ramalho-Santos J, Varum S, Amaral S, Mota PC, Sousa AP, Amaral A. 2009. Mitochondrial functionality in reproduction: From gonads and gametes to embryos and embryonic stem cells. Human Reproductive Update 15(5):553–572. DOI: 10.1093/humupd/dmp016.
- Redza-Dutordoir M, Averill-Bates DA. 2016. Activation of apoptosis signalling pathways by reactive oxygen species. Biochimica et Biophysica Acta 1863:L2977–2992.
- Rost-Roszkowska M, Poprawa I, Chajec Ł, Chachulska-Żymełka A, Leśniewska M, Student S. 2020b. Effects of short- and long-term exposure to cadmium on salivary glands and fat body of soil centipede Lithobius forficatus (Myriapoda, Chilopoda): Histology and ultrastructure. Micron 137:102915. DOI: 10.1016/j.micron.2020.102915.
- Rost-Roszkowska M, Poprawa I, Chajec Ł, Chachulska-Żymełka A, Wilczek G, Wilczek P, Student S, Skowronek M, Nadgórska-Socha A, Leśniewska M. 2020a. Influence of soil contaminated with cadmium on cell death in the digestive epithelium of soil centipede Lithobius forficatus (Myriapoda, Chilopoda). The European Zoological Journal 87(1):242–262. DOI: 10.1080/24750263.2020.1757168.
- Said TM, Agarwal A, Sharma RK, Thomas Jr AJ, Sikka SC. 2005. Impact of sperm morphology on DNA damage caused by oxidative stress induced by β-nicotinamide adenine dinucleotide phosphate. Fertility and Sterility 83(1):95–103. DOI: 10.1016/j.fertnstert.2004.06.056.
- Salvioli S, Ardizzoni A, Franceschi C, Cossarizza A. 1997. JC-1, but not DiOC 6 (3) or rhodamine 123, is a reliable fluorescent probe to assess Δ Ψ changes in intact cells: Implications for studies on mitochondrial functionality during apoptosis. FEBS Letters 411(1):77–82. DOI: 10.1016/S0014-5793(97)00669-8.
- Sanni B, Williams K, Sokolov EP, Sokolova IM. 2008. Effects of acclimation temperature and cadmium exposure on mitochondrial aconitase and LON protease from a model marine ectotherm, Crassostrea virginica. Comparative Biochemistry and Physiology Part C– Toxicology and Pharmacology 147(1):101–112. DOI: 10.1016/j.cbpc.2007.08.005.
- Sarsour EH, Kalen AL, Xiao Z, Veenstra TD, Chaudhuri L, Venkataraman S, Reigan P, Buettner GR, Goswami PC. 2012. Manganese superoxide dismutase regulates a metabolic switch during the mammalian cell cycle. Cancer Research 72(15):3807–3816. DOI: 10.1158/0008-5472.CAN-11-1063.
- Satarug S, Baker JR, Urbenjapol S, Haswell-Elkins M, Reilly PEB, Williams DJ, Moore MR. 2003. A global perspective on cadmium pollution and toxicity in non-occupationally exposed population. Toxicological Letters 137(1–2):65–83. DOI: 10.1016/S0378-4274(02)00381-8.
- Siekierska E. 2003. The structure of the ovary and oogenesis in the earthworm, Dendrobaena veneta (Annelida, Clitellata). Tissue & Cell 35(4):252–259. DOI: 10.1016/S0040-8166(03)00038-7.
- Siekierska E, Urbańska-Jasik D. 2002. Cadmium effect on the ovarian structure in earthworm Dendrobaena veneta (Rosa). Environmental Pollution 120(2):289–297. DOI: 10.1016/S0269-7491(02)00152-5.
- Siskova Z, Mahad J, Pudney C, Campbell G, Cadogan M, Asuni A, O’Connor V, Perry VH. 2010. Morphological and functional abnormalities in mitochondria associated with synaptic degeneration in prion disease. American Journal of Pathology 177(3):1411–1421. DOI: 10.2353/ajpath.2010.091037.
- Sokolova M. 2004. Cadmium effects on mitochondrial function are enhanced by elevated temperatures in a marine poikilotherm, Crassostrea virginica Gmelin (Bivalvia: Ostreidae). Journal of Experimental Biology 207(15):2639–2648. DOI: 10.1242/jeb.01054.
- Sonakowska L, Włodarczyk A, Wilczek G, Wilczek P, Student S, Rost-Roszkowska MM. 2016. Cell death in the epithelia of the intestine and hepatopancreas in Neocaridina heteropoda (Crustacea, Malacostraca). PLoS One 11(2):e0147582. DOI: 10.1371/journal.pone.0147582.
- Sousa AP, Amaral A, Baptista M, Tavares R, Caballero Campo P, Caballero Peregrín P, Freitas A, Paiva A, Almeida-Santos T, Ramalho-Santos J. 2011. Not all sperm are equal: Functional mitochondria characterize a subpopulation of human sperm with better fertilization potential. PLoS One 6(3):e18112. DOI: 10.1371/journal.pone.0018112.
- Stalmach M, Wilczek G, Wilczek P, Skowronek M, Mędrzak M. 2015. DNA damage in haemocytes and midgut gland cells of Steatoda grossa (Theridiidae) spiders exposed to food contaminated with cadmium. Ecotoxicology and Environmental Safety 113:353–361. DOI: 10.1016/j.ecoenv.2014.12.023.
- Świątek P, Klag J, Romek M. 2001. Do germ line cells in Allacma fusca (Insecta, Collembola, Symphypleona) have a higher metabolic rate than somatic cells. Folia Biologica (Cracow) 49:85–90.
- Świątek P, Vogelgesang M, Romek M, Klag J. 2004. Germ-line versus somatic cells. I. Stereological study of differentiating embryonic tissues of Tetrodontophora bielanensis (Hexapoda, Collembola). Canadian Journal of Zoology 82(5):714–725. DOI: 10.1139/z04-040.
- Suganya M, Karthi S, Subramanian Shivakumar M. 2016. Effect of cadmium and lead exposure on tissue specific antioxidant response in Spodoptera litura. Free Radicals and Antioxidants 6(1):90–100. DOI: 10.5530/fra.2016.1.11.
- Takacs V, Molnar L, Klimek B, Gałuszka A, Morgan AJ, Plytycz B. 2016. Exposure of Eisenia andrei (Oligochaeta; Lumbricidea) to cadmium polluted soil inhibits earthworm maturation and reproduction but not restoration of experimentally depleted coelomocytes or regeneration of amputated segments. Folia Biologica (Cracow) 64(4):275–284. DOI: 10.3409/fb64_4.275.
- Tang W, Shaikh ZA. 2001. Renal cortical mitochondrial dysfunction upon cadmium metallothionein administration to Sprague-Dawley rats. Journal of Toxicology and Environmental Health, Part A 63(3):221–235. DOI: 10.1080/15287390151101583.
- Tarazona AM, Rodriguez JI, Restrepo LF, Olivera-Angel M. 2006. Mitochondrial activity, distribution and segregation in bovine oocytes and in embryos produced in vitro. Reproduction in Domestic Animals 41:5–11.
- Tarnawska M, Kafel A, Augustyniak M, Rost-Roszkowska M, Babczyńska A. 2019. Microevolution or wide tolerance? Level of stress proteins in the beet armyworm Spodoptera eqigua Hübner (Lepidoptera: Noctuidae) exposed to cadmium for over 150 generations. Ecotoxicology and Environmental Safety 178:1–8. DOI: 10.1016/j.ecoenv.2019.04.017.
- Telfer WH, Huebner E, Smith DS. 1982. The biology of vitellogenic follicles in Hyalophora and Rhodnius. In: King RC, Akai H, editors. Insect ultrastructure. New York, USA: Plenum Press. pp. 118–149.
- Tourmente S, Lecher P, Degroote F, Renaud M. 1990. Mitochondrial development during Drosophila oogenesis: Distribution, density and in situ RNA hybridizations. Biology of the Cell 68(2):119–127. DOI: 10.1016/0248-4900(90)90296-F.
- Vandenbulcke F, Grelle C, Fabre M-C, Descamps M. 1998a. Implication of the midgut of the centipede Lithobius forficatusin the heavy metal detoxification process. Ecotoxicology and Environmental Safety 4(3):258–268. DOI: 10.1006/eesa.1998.1706.
- Vandenbulcke F, Grelle C, Fabre M-C, Descamps M. 1998b. Ultrastructural and autometallographic studies of the nephrocytes of Lithobius forficatus L. (Myriapoda, Chilopoda): Role in detoxification of cadmium and lead. International Journal of Insect Morphology 27(2):111–120. DOI: 10.1016/S0020-7322(98)00034-8.
- Vega MM, Marigomez JA, Angulo E. 1989. Quantitative alterations in the structure of the digestive cell of Littorina littorea on exposure to cadmium. Marine Biology 103(4):547–553. DOI: 10.1007/BF00399587.
- Velasquez-Vottelerd P, Anton Y, Salazar-Lugo R. 2015. Cadmium affects the mitochondrial viability and the acid soluble thiols concentration in liver, kidney, heart and gills of Ancistrus brevifilis (Eigenmann, 1920). Open Veterinary Journal 5(2):166–172.
- Wallace KB, Starkov AA. 2000. Mitochondrial targets of drug toxicity. Annual Review of Pharmacology and Toxicology 40(1):353–388. DOI: 10.1146/annurev.pharmtox.40.1.353.
- Wang J, Zhang P, Shen Q, Wang Q, Liu D, Li J, Wang L. 2013. The effects of cadmium exposure on the oxidative state and cell death in the gill of freshwater crab Sinopotamon henanense. PLoS One 8(5):e64020. DOI: 10.1371/journal.pone.0064020.
- Wilczek G, Karcz J, Rost-Roszkowska M, Kędziorski A, Wilczek P, Skowronek M, Wiśniewska K, Kaszuba F, Surmiak K. 2019. Evaluation of selected biological properties of the hunting web spider (Steatoda grossa, Theridiidae) in the aspect of short- and long-term exposure to cadmium. Science of the Total Environment 656:297–306. DOI: 10.1016/j.scitotenv.2018.11.374.
- Włodarczyk A, Sonakowska L, Kamińska K, Marchewka A, Wilczek G, Wilczek P, Student S, Rost-Roszkowska M. 2017. The effect of starvation and re-feeding on mitochondrial potential in the midgut of Neocaridina davidi (Crustacea, Malacostraca). PLoS One 12(3):e0173563. DOI: 10.1371/journal.pone.0173563.
- Włodarczyk A, Wilczek G, Wilczek P, Student S, Ostróżka A, Tarnawska M, Rost-Roszkowska M. 2019. Relationship between ROS production, MnSOD activation and periods of fasting and re-feeding in freshwater shrimp Neocaridina davidi (Crustacea, Malacostraca). PeerJ 7:e7399. DOI: 10.7717/peerj.7399.
- Xia MF, Zhang YZ, Jin K, Lu ZT, Zeng Z, Xiong W. 2019. Communication between mitochondria and other organelles: A brand-new perspective on mitochondria in cancer. Cell & Bioscience 9(1):27. DOI: 10.1186/s13578-019-0289-8.
- Yao CL, Wang AL, Wang ZY, Wang WN, Sun RY. 2007. Purification and partial characterization of Cu, Zn superoxide dismutase from haemolymph of Oriental river shrimp, Macrobrachium nipponense. Aquaculture 270(1–4):559–565. DOI: 10.1016/j.aquaculture.2007.04.068.
- Yu Z, He X, Fu D, Zhang Y. 2011. Two superoxide dismutase (SOD) with different subcellular localizations involved in innate immunity in Crassostrea hongkongensis. Fish & Shellfish Immunology 31(4):533–539. DOI: 10.1016/j.fsi.2011.06.022.
- Yuan H, Qin F, Guo W, Gu H, Shao A. 2016. Oxidative stress and spermatogenesis suppression in the testis of cadmium-treated Bombyx mori larvae. Environmental Science and Pollution Research International 23(6):5763–5770. DOI: 10.1007/s11356-015-5818-3.
- Zelko IN, Manriani YJ, Folz RJ. 2002. Superoxide dismutase multigene family: a comparison of the CuZn-SOD (SOD1), Mn-SOD (SOD2), and EC-SOD (SOD3) gene structures, evolution, and expression. Free Radical Biology and Medicine 33:337–349.
- Zhang QL, Li F, Wang B, Zhang J, Liu Y, Zhou Q, Xiang J. 2007. The mitochondrial manganese superoxide dismutase gene in Chinese shrimp Fenneropenaeus chinensis: Cloning, distribution and expression. Developmental and Comparative Immunology 31(5):429–440. DOI: 10.1016/j.dci.2006.08.005.
- Zhang Y-Z, Ouyang Y-C, Hou Y, Schatten H, Chen D-Y, Sun Q-Y. 2008. Mitochondrial behaviour during oogenesis in zebrafish: A confocal microscopy analysis. Development, Growth & Differentiation 50(3):189–201. DOI: 10.1111/j.1440-169X.2008.00988.x.
- Zhou D, Shao L, Spitz DR. 2014. Reactive oxygen species in normal and tumor stem cells. Advances in Cancer Research 122:1–67.
- Zorova LD, Popkov VA, Plotnikov EY, Silachev DN, Pevzner IB, Jankauskas SS, Babenko VA, Zorov SD, Balakireva AV, Juhaszova M, et al. 2018. Mitochondrial membrane potential. Analytical Biochemistry 552:50–59. DOI: 10.1016/j.ab.2017.07.009.