Abstract
In the past centuries, many of the Eurasian lynx populations declined and finally disappeared. In Poland, only two populations survived until the 20th century. In the 90s a new population was reintroduced in central Poland but presently, its fate is considered uncertain. While the recurring observations of lynx in the area confirm its presence, they are insufficient to evaluate the state of this population. Therefore, using a population viability model and the reintroduction program data, we analyzed the ambiguities in the vital rates assessments to find the most likely scenario for the development of the population since its reintroduction. Finally, we modeled different options to improve the survival chances of this one and possibly other small populations. Estimated parameters result in a declining population. In the majority of the simulations, lynx populations go extinct within 20 years. This can be improved by reintroduction or natural immigration of new individuals every 4 years, but only if the new individuals arrive within a short period of time after the initial reintroduction. Also, longer time intervals are insufficient to elevate the median time to extinction beyond 50 years. Separately, moderate changes in reproduction or survival rates have only a marginal effect on the population’s survival. Decreasing only the impact of mortality would increase this population’s persistence time, but not ensure its long-term survival. However, decreased mortality in combination with immigration should result in much more successful population development. With higher longevity and release every 4 years the population is more successful even if the new individuals start entering the population up to 20 years later. Even less frequent immigration combined with enhanced longevity still improves survival. Combined adjustment of individuals to their environment and regular immigration provide the best chances of long-term survival. One or both of those factors could explain why lynx is still present in the area.
Introduction
In the past centuries, many of the Eurasian lynx Lynx lynx (Linnaeus, 1758) populations declined and finally disappeared: first in the lowlands (around 18th century) and by the end of the 19th century also in the mountain regions. At present, lynx range covers Scandinavia and Eastern Europe, with its border in northeast Poland (Kaczensky et al. Citation2012). Smaller European ranges of lynx cover the Carpathian Mountains and the Middle East (Kaczensky et al. Citation2012; von Arx Citation2018). Several small populations in Western Europe are the result of the reintroductions that started in the 70s (Breitenmoser et al. Citation2000). In Poland, the decline process left only two populations (Białowieża and Carpathians) surviving till the 20th century. In the 20th century, numbers of lynx in Poland started temporally increasing, reaching the highest number of 600 individuals in the 80s. However, since the beginning of the 21st century, the total number of lynxes in Poland is estimated only at around 200 individuals (Jędrzejewski et al. Citation2002; von Arx et al. Citation2004).
At the beginning of the 90s, the reintroduction of lynx in the Kampinos National Park (Kampinos NP) was initiated. The park is located in central Poland, around 300 km from Białowieża National Park - the closest surviving population of the lynx. The landscape between the two national parks, as well as the rest of central Poland, is highly populated and fragmented with a dense road network (Niedziałkowska et al. Citation2006). The population in Białowieża is considered highly isolated (Kowalczyk et al. Citation2015) and clear genetic differences between it and the Carpathian population suggest some barriers between these populations (Schmidt Citation2008). Since no natural recoveries in the areas within former lynx distribution range in Poland were observed (Schmidt Citation2008) this led to the conclusion that the natural return of the species to the Kampinos area was unlikely.
Kampinos NP management saw a pressing need to reduce the numbers of ungulates (esp. roe deer Capreolus capreolus) which presented a good opportunity for reintroduction (Böer et al. Citation1994; Kowalski et al. Citation2003; Reklewski Citation2006). Also, the independent assessment of the roe dear population size in 1996 as well as in 2013 (Nowicki & Kowalski Citation2003; Biedka Citation2014) showed that the introduced population of 15 lynx individuals would theoretically require approximately one-third of the overall Kampinos NP roe deer (which is slightly more than the observed 26% predation in Bialowieski NP; Schmidt Citation2011; Okarma et al. Citation1997). Besides the large numbers of roe deer, also the abundance of complementary prey like hare, rodents and ground-nesting birds (Jędrzejewski et al. Citation1993, Schmidt Citation2008) provided necessary conditions especially for the younger, less-skilled individuals or the ones migrating through open areas. The number of species of small mammals (around 20 species of insectivores and rodents) and birds (around 115 breeding species) in Kampinos NP is typical for the central Poland area (Andrzejewski Citation2003; Cygan et al. Citation2003; Lesiński et al. Citation2013).
Between 1994 and 2001, 30 individuals (17 females and 13 males) coming from 10 European Zoos were released (Böer et al. Citation1995; Reklewski Citation2006; Danyłow Citation2010) (see methods section). All individuals went through an adaptation phase and most of them (except for five) showed natural behavior typical for wild individuals and had no problems hunting live prey (Reklewski Citation2006). Radio telemetry observations showed further that lynx adjusted well to their new area (Böer et al. Citation1995; Reklewski Citation2006). Eight out of 17 collared females were observed to produce offspring, most of them only once in their life (except of one female that bred three times and two females that bred two times). Two females were observed to have two offspring, the rest only one (Reklewski Citation2006). A total of 14 offspring were observed during the whole monitoring period, eight of them surviving until winter. Later observations (1999–2001) showed also three wild females (probably first or second generation) with seven offspring, three of which survived until winter (Reklewski Citation2006).
Despite the initial assumption that the reintroduced population will be isolated, seven individuals migrated outside the national park area (five females and two males, in two main directions; Böer et al. Citation1995, Citation2000; Reklewski Citation2006). Lynx migrating west along the Vistula Valley reached the Iłowskie Forests and further Gostynińsko-Włocławskie Forests. Surprisingly, some individuals headed south through the agricultural landscape with dense road infrastructure. Individuals choosing this direction reached Bolimów Forest and one male reached the area of Forest District Włoszczowa, covering the distance of at least 160 km (Reklewski Citation2006). Individuals migrating in this direction might have followed the Bzura Valley (Böer et al. Citation1995, Citation2000). Probably some of the migrating females had offspring outside the park area. Collared females with young were observed three times in the area of Bolimów Forests, twice in Łąck and once in Jabłonna Forest district. By the end of the reintroduction program, it was estimated that the population in Kampinos NP comprised 19 individuals (Böer et al. Citation2000). Between the years 2000 and 2004, the size of this population was estimated to range between 10 and 15 adult individuals, mostly born in the wild (Reklewski Citation2006) and more recently at around 10 (Danyłow Citation2010) and then 8 individuals (Biedka Citation2014). However, these estimates were based only on winter tracking and coincidental observations. In 2012, four different lynx tracks (including a female with offspring) were observed and individuals were heard during the mating season (Romanowski Citation2014). In June 2014, a lynx track was documented near the cemetery of Palmiry. In summer 2015, a camera trap recorded an adult individual in the park (Olszewski Citation2016). In 2018, a lynx was captured on camera traps in 7 different localities in Kampinos NP within a month (T. Diserens personal communication, Mysłajek et al. Citation2019).
Currently, we lack any recent data confirming the presence of lynx in the initially observed migration destinations. The last cases of confirmed lynx sightings in Gostynińsko-Włocławskie Forest (in the area of Łąck Forest District) were noted in 2001 (remains of a roe-deer) and in 2002 (collared lynx from Kampinos NP) (Romanowski Citation2014). The majority of lynx observations in this area were made in 2000 and 2001. The latest reliable observation was made in 2004 in the area of Duninów, and in 2006 most likely a lynx track was found in the Gostynin Forest District (Romanowski Citation2014).
Reintroductions of carnivores are much more difficult than those of the herbivores and even more so when the released animals come from captivity (Vandel et al. Citation2006; Jule et al. Citation2008; Chapron et al. Citation2014; Wilson Citation2018). Large carnivores avoid urban areas (Niedziałkowska et al. Citation2006), they require larger areas and due to low densities are much more vulnerable to loss of genetic variability (Schmidt Citation2008). There is also less acceptance for the reintroductions of large carnivores, for example due to fear for domestic animals (Schadt et al. Citation2002; Chapron et al. Citation2014). Data on lynx reintroductions in Europe show how unpredictable the outcome can be (e.g. Cop & Frkovic Citation1998; Vandel et al. Citation2006; Linnell et al. Citation2009; Wilson Citation2018). Only a third of 15 lynx reintroductions conducted throughout Europe at the end of the 20th and the beginning of the 21st century can be considered successful (Linnell et al. Citation2009). Many of the programs analyzed by Linnell et al. (Citation2009) were not preceded by detailed research and planning nor public campaigns. Furthermore, some of the earlier reintroductions were conducted unofficially. Similarly, in the official programs, the fate of the animals is often unknown due to the relatively common transmitter malfunctions, and little to no monitoring phase. High mortality due to poaching and vehicle collisions, bad adaptation, and small number of released individuals belong to the most common causes of reintroduction failure (Linnell et al. Citation2009). Similarly, in case of the Kampinos NP reintroduction, vehicle collisions, illness and poaching were the main causes of death and the fate of many individuals remained unknown due to transmitter malfunctions, migrations, or other unidentified reasons (Böer et al. Citation1995; Reklewski Citation2006) At this point, the results of the reintroduction in Kampinos NP are generally considered uncertain. While the recurring observations of lynx in the area of the park provide enough evidence to confirm its presence, these observations are insufficient to evaluate the size or the development of this population.
Following up on the concerns about the development of the lynx population in Kampinos NP, we decided to evaluate the viability of the population. Using a population viability model, we analyzed the ambiguities in the vital rates assessments to find the most likely scenario for the development of the population since its reintroduction. In this context, we aimed to address three main questions: 1. Which factors from the population dynamics point of view are the most important for Kampinos’ population survival? 2. Is the long-term survival of this population as a part of a metapopulation feasible? 3. Whether any and what further actions are necessary to ensure its long-term survival. In order to address these questions, we compared the parameters from field and modeling studies of other lynx populations with the vital rates estimated in the Kampinos NP reintroduction program (see ). Finally, we modeled different options to improve the survival chances of this and possibly other small populations.
Table I. Number of lynx released in the reintroduction program
Table II. Compilation of parameters used in the model and other reintroduction programs in Europe
Materials and methods
The study area
The study area is located in central Poland and includes three separate large forested areas protected in Kampinos National Park, Gostynin-Włocławek Landscape Park, and Bolimów Landscape Park (). Kampinos NP (385 km2) forest cover is 73% and consists mostly of pine woods and mixed pine-oak woods (43.5% and 21% of the forest surface; Kloss Citation2003). In Gostynin-Włocławek Landscape Park (390 km2) 62% of the area is covered by forests, mainly pine woods, and includes dense hydrological network of lakes and rivers (Wacnik et al. Citation2011) (). Bolimów Landscape Park (231 km2) protects remnants of historic vast forests in the valley of the Bzura river (). It is to 70% covered by forests dominated by pine (Pinus silvestris) (Jakubowska-Gabara & Markowski Citation2002). Kampinos NP and Gostynin-Włocławek Landscape Park are located at a distance of approximately 55 km from each other, within the Vistula River valley, which is recognized as a dispersion corridor for mammals (Romanowski Citation2007). The Vistula river banks and the floodplain are covered by diverse, seminatural vegetation: permanent meadows, shrubs, and riparian forests, as well as arable lands. Bolimów Landscape Park is located approximately 30 km south of Kampinos NP and the area between the parks represents a mixed landscape of arable lands, orchards, and developed areas. Bzura River valley serves as a potential dispersion corridor for mammals including lynx (Böer et al. Citation1995; Reklewski Citation2006).
Population structure and development
Using the RAMAS GIS metapopulation model (Akçakaya Citation2005), we built sex- and age-structured model. We simulated the reintroduced population as a network of three patches with one main subpopulation where the individuals were released (in Kampinos NP) and two potential subpopulations in Gostynin-Włocławek and Bolimów landscape parks.
In the reintroduction program, under the assumption that the average female home-range is 50 km2 and male home-range can overlap with up to three female home-ranges the park area (around 350 km2) was estimated to contain seven females and three to seven males (Reklewski Citation2006). In our model, to achieve the same number of females in the Kampinos NP we set a ceiling carrying capacity (i.e. individuals above the set number are removed from the population) of 15 adult individuals (i.e. seven to eight females) for this population, five for Gostynin-Włocławek, and three for Bolimów subpopulations. The model, like the reintroduction program, was initialized with seven individuals (first release of two males and five females) and for six consecutive years population was supplemented with new individuals (see ; Böer et al. Citation1994; Böer et al. Citation1995; Reklewski Citation2006). Using captive-bred animals for the reintroduction allowed selecting the release time when the animals were close to the breeding age (assuming the individuals were born in May their age at the release time ranged between 1.4 and 5.8 years old with the mean age of 2.35 years (Reklewski Citation2006). If possible, the individuals were paired prior to release and released during the mating time to further improve the breeding success. To provide a similar situation, i.e. that the released individuals are able to reproduce, individuals introduced in our model were adults. Demographic parameters were modeled after monitoring data of the Kampinos NP lynx population after final release (see ; Reklewski Citation2006).
In our base scenario, an individual’s lifecycle comprised of three life stages (young, subadult, and adult individuals), each characterized by different survival and fecundity rates. The adult survival rate decreases after their fourth year of life to account for the short life span of many individuals in the released group (see and discussion). For the base scenario, we recorded the growth rate (lambda), and the median time to extinction from 1000 repetitions and simulation time of 50 years ().
Table III. Growth rate and median time to extinction for all simulated scenarios
Sensitivity analysis
In besides the parameters obtained from the monitoring of the Kampinos population, we compiled the parameters used in other European lynx studies. Those parameters served as range information for testing the impact of each parameter on the development of the population. For each value of the tested parameter, we recorded the changes in growth rate (lambda), and the median time to extinction from 1000 repetitions and simulation time of 50 years ().
We tested how population survival is affected by changes in the survival of juvenile individuals, the survival of subadults and adults, and adult individual’s lifespan. We simulated the dynamics of the metapopulation with the mortality rates increased and decreased by 2, 5, 7, and 10% points for all stages excluding the juveniles and separately affecting only the juvenile stage (see ). To test the effect of longevity, we increased the survival rate of the last stage of our model (individuals older than four years) to 50% and 72% (base rate for subadult and adult stage). We also simulated the effects of different breeding success with one, three, and four offspring per female and compared it with the base breeding success of two offspring per female.
In the planning phase of the reintroduction program, it was assumed that the large distances from the release site (Kampinos NP) to other large forest complexes would prevent any migration resulting in the isolated population (see ). Therefore, we simulated the dynamics of the Kampinos NP subpopulation without connection to any other subpopulations. Finally, we also simulated different dispersal rates (0.05, 0.1, and 0.15) between all subpopulations.
Management options
To assess the management options, we simulated further reintroduction/immigration for the base scenario first and in combination with varied breeding and survival rates. For this purpose, we selected three parameters having the highest influence on the success of the population: 1. breeding rate, 2. longevity, 3. changes in the mortality rate of subadults and adults (see results section). For those parameters, while keeping the base exchange rate of individuals between the subpopulations (see ), we tested different supplementation scenarios and their effect on population survival.
To find the lowest necessary supplementation scheme resulting in population survival over 100 years we analyzed different time intervals between incoming individuals with different numbers of individuals arriving per time interval: (a pair every 4, 5 or 6 years or single individuals (females and males alternating) every two to three years. Supplementation in this context can be understood in two ways: as an artificial introduction of new individuals (bred in captivity or translocated from larger populations) or as natural migration of individuals from further populations. Since natural migration could have been potentially present since the release of the individuals in the program we tested different initial points of immigration starting in the eighth year of the simulation which equals the end of the original reintroduction program or starting every five years after that till present (i.e. starting year 8, 13, 18, 23, 28, 33). This can also allow us to find a point in the population development at which artificial supplementation is no longer necessary or no longer can improve survival chances.
Results
The simulation with the base setting resulted in a growth rate (lambda) of 0.9097 and a median time of approximately 17 years to extinction. All the simulated parameter settings resulted in population extinction within 50 years. From the tested parameters, a decrease in the mortality rate of subadults and adults, increased breeding rate, and longevity had a slightly positive influence on the success of the population. However, only in case of the simulations with the longer life span, the median time to extinction exceeded 25 years (20.8 years in case of 10% decrease in mortality, 20.6 and 24.3 for the breeding rate of three and four offspring respectively and 29.9 with longer life span). In the case of the two most favorable simulations, the deterministic predictions of the growth rate differed slightly from the stochastic simulations. According to the growth rate (lambda) increasing the breeding to four offspring would have been the most beneficial scenario, however, in the stochastic simulations, the longer life span resulted in the higher median time to extinction. Changes in juvenile mortality rates and different dispersal rates between the subpopulations had an overall low impact on population survival. Mortality rates of subadult and adult individuals had a slightly stronger impact on metapopulation survival. The same could be observed in the differences in the growth rate (lambda) ().
Considering the follow-up reintroduction or natural migration of individuals in the base setup of parameters, the most successful scenarios were the ones assuming the incoming of one new female and male individual every 4 years with a two-year shift between them (female-male alternating pattern). In the best case, the median time of extinction increased to 80 years. However, in the case of base parameter setup, only those scenarios with the immigration starting immediately or 5 years after the reintroduction program had any influence on the population’s success. Similarly, the second-best option was the immigration/release of a pair of individuals every 4 years starting immediately or 5 years after the reintroduction program. Any later, the addition of new individuals did not have any strong influence on the population’s survival. In the case of the scenarios where the individuals were immigrating every 6 years, both in case of alternating individuals or a pair release only the immediate or 5 years later release increased the median time to extinction above 20 years. Five and six years’ time intervals were too long to elevate the median time to extinction above 50 years. In all cases, only the migration or supplementation initiated immediately or within a short period (5 years) after the reintroduction had any impact on population survival ().
Figure 2. Estimated extinction risk after 100 years for all simulated scenarios. Columns: pattern of incoming individuals per time interval. Left column: a pair (male and female individuals arriving simultaneously), Right column: alternating single male and female individuals (both arriving within the same time interval but at different points in time). Rows: time intervals for incoming individuals, top row: 4-year cycle, middle row: 5-year cycle, bottom row: 6-year cycle
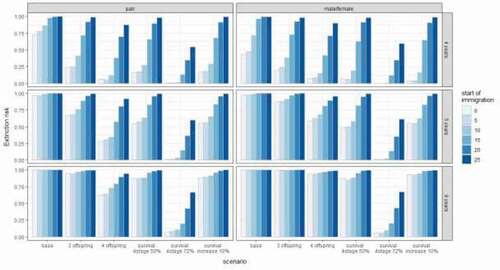
Figure 3. Median time to extinction for all simulated scenarios. Columns: pattern of incoming individuals per time interval. Left column: a pair (male and female individuals arriving simultaneously), Right column: alternating single male and female individuals (both arriving within the same time interval but at different points in time). Rows: time intervals for incoming individuals, top row: 4-year cycle, middle row: 5-year cycle, bottom row: 6-year cycle
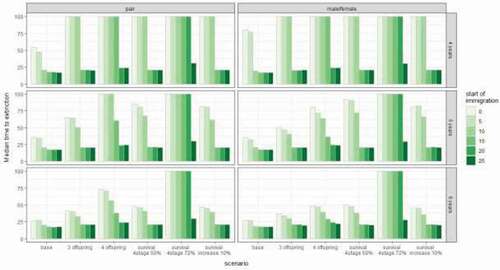
Immigration scenarios in combination with higher breeding rates, lower mortality, or increased longevity of the last stage of individuals presented a much more successful population development. When the individuals were released every 4 years the longevity of individuals had the strongest influence in both single individuals and pair release options. In this case, the risk of extinction within 100 years was reduced almost to zero and it was also very successful even if the new reintroductions or natural connection to other populations were established late (up to 20 years after the reintroduction program) (). In the case of releasing a pair every 4 years, the second most successful scenario-parameter combination was the breeding rate of four offspring, followed by 50% longevity, the increase of survival, and finally the weakest option breeding rate of three offspring. However, in the case of the male-female alternating release option, the order of the importance of parameters varied. The increase of survival was the second-best option followed by 50% longevity and breeding rates of four and three offspring respectively (). Overall more frequent reintroductions provide a much higher success rate for the population, but even the reintroduction or immigration only every 6 years worked well in combination with the increased longevity of individuals. Also, the breeding rate of four offspring when a pair of individuals was released every 6 years decreased the extinction risk, however, the effect was much weaker than the longevity (). Looking at the median time to extinction we saw that when initiated early, mostly up to 15 years after the initial reintroduction program, introduction/immigration of new individuals every 4 years had a strong effect on population success, but the longer the time between the releases the lower was the effect. In the case of every 6 years only the strongest parameter – longevity still improved survival ().
Discussion
Our simulations show that the parameters estimated during the reintroduction program result in a declining population. In the majority of the stochastic simulations, lynx populations go extinct within twenty years. This quick decline in our base simulation does not, however, match with the more recent observations and local experts’ more optimistic estimations. It is likely that the initially estimated parameters changed or some external factors like immigration play a role in the population’s survival.
Previous studies found that the majority of lynx deaths are due to human activity (Andrén et al. Citation2006; Červený et al. Citation2019; Heurich et al. Citation2018; Kowalczyk et al. Citation2015; Schmidt-Posthaus et al. Citation2002; Wölfl & Schwaiger Citation2017). For example, more than 60% of death cases in Switzerland, and 70% in Białowieża, Poland (Jędrzejewski et al. Citation1996; Breitenmoser et al. Citation2007) were identified as the effects of poaching or traffic accidents. According to monitoring data from Kampinos NP, most of the identified causes of death were also due to anthropological reasons (Böer et al. Citation1995, Citation2000; Reklewski Citation2006). Released individuals were initially much more vulnerable, presumably because they were bred in captivity and thus were used to human presence (Reklewski Citation2006; Jule et al. Citation2008). Some individuals could even associate the sound of a car with food delivery. Additionally, some of the released individuals had problems adapting to their new environment, they were then captured and, if possible, released again after given the necessary help (Böer et al. Citation1995, Citation2000; Reklewski Citation2006). Therefore, it is possible that without human intervention, the actual mortality rates of the released individuals would have been even higher. Lynx is reported to live up to 17 years in the wild and some modeling studies assume this as a maximum age of lynx (Boehme et al. Citation2000; Kramer‐Schadt et al. Citation2004; Ovenden et al. Citation2019) (see ). However, empirical studies show a wide range of longevity rates. The mean survival time (from release to recorded death) of the reintroduced individuals in Kampinos NP was on average 272 days (47–952 days), additionally, five individuals were captured and re-released (up to three times) which most likely prevented their death (Böer et al. Citation2000; Reklewski Citation2006). Similarly, for natural populations, Kubala et al. (Citation2019) noted a big population turnover with low recapture rate and mean persistence of lynx 12.7 months (ranging from 2 to 23 months) and according to Jędrzejewski et al. (Citation1996), only 24% of individuals survive 3 years, also mostly due to anthropogenic reasons. As confirmed by our results, high adult mortality and short life span are likely to be the main cause of the poor situation of the reintroduced population in Kampinos NP (). Nonetheless, it is possible that due to the learning effect or change of conditions, the wild-born individuals more successfully avoided danger and their yearly survival and associated life span increased. Learning to avoid danger could have a large effect on lynx survival. The magnitude of such an effect might be comparable to conservation measures that aim at reducing human related-mortality. For example, implementing conservation measures such as under- and overpasses, reduced speed zones, and fencing (Simon et al. Citation2012) decreased the effect of road mortality on Iberian lynx (Lynx pardinus) in Doñana from 16.7% (in the 80s) to 8.6% of the total annual mortality rate (Garrote et al. Citation2018).
According to our simulations, decreasing the mortality in the Kampinos population would increase its persistence time. However, since such a small population is highly vulnerable to stochastic events, only a decrease in mortality would not be sufficient to ensure its long-term survival. Similarly to mortality, we expect that breeding success has a very strong impact on population survival (). In a single reproductive event, lynx give birth to one to four, usually two to three young (see ). However, based on observations in Kampinos NP the number was more likely to be lower (between one and two; Reklewski Citation2006). Additionally, in Kampinos NP only 35% of the released females were observed breeding (Böer et al. Citation2000). Similarly, Cop and Frkovic (Citation1998) reported the breeding success in the first 6 years of the reintroduction programs in Slovakia at around 1.6 young per female. In this case, we rather optimistically assumed all females reproducing with the breeding success of two cubs per female. Higher breeding rates were observed in some populations (for example 3.3 in Jędrzejewski et al. Citation1996) but according to Nilsen et al. (Citation2012) litter sizes seem to be rather constant across study sites and age classes. Therefore, one of our scenarios with the breeding success of four offspring is rather unrealistic and should not be considered as the actual solution to the reintroduction failure. Despite that, we still decided to include this scenario to evaluate how much the number of offspring affected the long-term survival of the population.
The mean age of animals reintroduced in Kampinos NP was slightly above two years. In our model, only the adult individuals (in their third year of life and older) reproduce, and we did not include any variation in breeding rates depending on age. This of course is a simplification, since we do not have enough data from the next generations of wild-born lynx in the reintroduction program. The literature data shows that the number of breeding females is the main variation factor in lynx fertility, and is especially pronounced between females breeding for the first time (two-year-olds) and the older females (Nilsen et al. Citation2012; Axnér et al. Citation2013). In our model, we did not make this assumption, and therefore we probably overestimated not only the number of offspring but also the breeding rate (for the effect of lower breeding rates see ). Still, the rates we used in the model are insufficient for ensuring the long-term survival of the Kampinos NP population ().
Another important factor affecting the long-term survival of the population is the available area. According to Schadt et al. (Citation2002) 2000 km2 might be necessary to sustain a viable population of 20 resident adults. However, such a requirement is much larger than initially assumed in the reintroduction program in Kampinos NP, only Kampinos NP combined with the neighboring national parks would be theoretically sufficient for sustaining the same number of individuals. Furthermore, home ranges of lynx vary greatly, in Białowieża they ranged between 80 and 150 km2 in adult individuals (Jędrzejewski et al. Citation1996) but can reach up to several hundred km2 (Wölfl et al. Citation2001). Kampinos NP individuals’ home ranges based on 6 months of observations were in accordance with the initial assumption of 50 km2. However, the yearly home ranges were already double that size (Reklewski Citation2006).
Since Kampinos NP is located relatively far from other large forest complexes, in the planning phase of the reintroduction program it was falsely assumed that dispersal of individuals outside the Park area is not likely (Reklewski Citation2006). Lynx can easily cover large distances (see for example Herrero et al. Citation2021) and the other parks included in our simulation are located within average dispersal distance. For example, in Białowieża subadult lynxes were observed to cover up to 129 km (Schmidt Citation1998). As shown by the simulation results () population isolation might have been slightly more beneficial for the short-term survival of the Kampinos population. In reality, it turned out quickly that several individuals left the main subpopulation and were able to cover long distances to finally reach one of the two other patches (Böer et al. Citation1995, Citation2000; Reklewski Citation2006). As concluded by Reklewski (Citation2006) most of the migrations occurring within the first month after the reintroduction suggested an already high concentration of lynx and the lack of unoccupied territory. Also, the bias toward migrating females pointed to the lack of resources or territory (see for example Herrero et al. Citation2021). This gave us a reason to assume a relatively high dispersal rate in our model. The low carrying capacity of the main patch in the Kampinos NP caused its quick saturation and forced individuals to migrate. Studies on the Iberian lynx in Doñana show that dynamic processes in the even smaller destination patches resulted in local extinctions and faster depletion of the overall number of individuals (Ferreras Citation2001; Ferreras et al. Citation2004). For our base population, this is a short-term effect impacting only the first years of the reintroduction program when the number of individuals is the highest. While the lack of connectivity between the subpopulations is not a realistic assumption, according to our model the rate of dispersal within the population is also not one of the most influential parameters on its survival (). On a larger spatial scale, the two smaller patches could have a positive effect if they serve as a stepping stone to connect the Kampinos population with other larger populations (Kramer-Schadt et al. Citation2011). However, at present, we are not able to assess whether their location really serves this purpose. On the other hand, their location and paths chosen by dispersing individuals clearly show the importance of river valleys as corridors for lynx dispersal and indicate further ways of connecting the Kampinos population (Böer et al. Citation2000; Romanowski Citation2007).
It is possible for isolated lynx populations to grow despite the genetic diversity loss. However, the small size of the initial group and long-term inbreeding can significantly lower the success chance of the reintroduced population (Schmidt et al. Citation2011; Sindičić et al. Citation2013; Bull et al. Citation2016; Mueller et al. Citation2020). While we lack the genetic data for the Kampinos NP and cannot directly assess its role in the development of the population, we emphasize the importance of exchange of individuals between populations. Already the original reintroduction program discussed the potential need for further supplementation of this population. Considering the initially assumed isolation and small carrying capacity, it was known that the future supplementation of the already established population might be necessary (Reklewski Citation2006). Soon after reintroduction when the population was considered successful, Böer et al. (Citation2000) suggested introducing new genetic founders every decade. However, we assume that additional individuals might also come from other natural or reintroduced populations e.g. in Piska forest, located approximately 200 km from Kampinos NP (Krzywiński et al. Citation2012). We assumed different patterns of adding new individuals to illustrate both the reintroduction release patterns and the naturally migrating individuals. For our base scenario, a single individual appearing every 2 years had the strongest impact on population survival, increasing the time to survival to up to 80 years. This is a much longer population survival than any of the ones achieved by reducing mortality or increasing the number of offspring. However, with only one year difference between the releases (two–three for a single individual and 5 years for a pair) the effect of supplementation is already much lower (time to extinction below 40) and in any case only if taking place from the beginning or shortly after the initial release (see results ). Because we tried to recreate the short life span and low breeding success, the short generation length clearly influenced the required reintroduction frequency. Furthermore, the small increase in the chance of population survival observed in some cases is actually caused by the presence of the regularly supplemented individuals and persists only as long as the supplementation process. Retrospectively, for the Kampinos NP population a continuation of the reintroduction program would be necessary, following immediately or with only a few years delay but with a much less intense release schedule. While for small and declining populations influx of new individuals is a crucial issue according to our simulations, later actions would be ineffective. This only further underlines the need of careful monitoring of the population after the reintroduction (Sindičić et al. Citation2013; Bull et al. Citation2016). As shown by our model, it is possible to identify a time frame within which new individuals need to be reintroduced. After that time, population will not be able to recover even with a regular supplementation effort. Nonetheless, regularly obtaining new individuals for reintroduction is challenging. For example, the latest estimates of the Slovakian population, which was often a source of individuals for reintroductions, despite good habitat conditions, show numbers below carrying capacity pointing to an unfavorable condition (Kubala et al. Citation2019). Probably a more plausible solution for future reintroduction programs would be choosing the location within the migration distance of other lynx populations.
According to our simulations, it is the combination of individuals adjusting to their environment and regular immigration that provides the best chances of long-term survival for the Kampinos population. At the moment we have several indications that this population is not thriving: 1) the decreasing number of lynx observations in Kampinos NP, 2) the lack of any signs in the two migration destinations in the last 15 years, 3) our results that indicate high extinction probabilities (). Despite the limited data, our model gives us a good idea of what could have gone wrong in this program. A small carrying capacity and a lack of connections to other existing populations could have been the first indicators of future issues. Furthermore, based on the monitoring data, without providing new individuals the population had rather low chances of long-term survival. It is, however, another issue whether supplying new captive individuals would be ethical knowing the costs and that it still might not ensure the success of this population (for more ethical deliberations on reintroduction see for example Thulin & Röcklinsberg Citation2020).
However, small numbers of lynx are still observed in this area (T. Diserens pers. comm.; Mysłajek et al. Citation2019) and their presence could be partially explained by immigration. We have evidence of individuals being able to cover long distances, e.g. an individual from the lynx reintroduction program in north-western Poland covering hundreds of kilometers (Rysie.org Citation2020 but see also Cop & Frkovic Citation1998; Krzywiński et al. Citation2012; Nowak et al. Citation2013). This could then suggest that the lowland lynx populations are adapting to the more fragmented, human-dominated habitats resulting in much more dispersed populations (Chapron et al. Citation2014). Undoubtedly, large-scale long-term monitoring including genetic monitoring is necessary to properly assess such trends (Sindičić et al. Citation2013; Bull et al. Citation2016; Kubala et al. Citation2019; Mueller et al. Citation2020). As observed by Wölfl et al. (Citation2001) there is already a large amount of data available on the local or national level, but on the European scale the situation of Lynx populations remains unclear. Animal reintroductions should extend far beyond local animal releases (Kramer-Schadt et al. Citation2005; Thulin & Röcklinsberg Citation2020). Reconstructing existing habitats and planning large-scale landscape connectivity are some of the most important conservation strategies to support highly dispersed populations (Schmidt Citation2008; Wilson Citation2018). As pointed out by Kramer-Schadt et al. (Citation2005), connecting populations is crucial. Tools for such habitat reconstruction and connectivity planning are already available (see Kramer‐Schadt et al. Citation2004; Kramer-Schadt et al. Citation2005) and the next step would be to use them on a European scale.
Acknowledgements
We would like to thank Jan Danyłow for the help on the initial version of this project, Tom Diserens for the information about recent lynx observations in Kampinos NP, Julie Furxhi for correcting English, Dr. Mathias Franz and anonymous reviewers for the useful comments on the previous version of the manuscript.
Disclosure statement
No potential conflict of interest was reported by the author(s).
References
- Akçakaya HR. 2005. RAMAS GIS: Linking spatial data with population viability analysis (version 5). Applied Biomathematics. Setauket, New York.
- Andrén H, John DC, Linnell OL, Andersen R, Danell A, Karlsson J, John Odden PF, Moa PA, Kvam T, Franzén R, Peter S. 2006. Survival rates and causes of mortality in Eurasian lynx (Lynx lynx) in multi-use landscapes. Biological Conservation 131(1):23–32. DOI:10.1016/j.biocon.2006.01.025.
- Andrzejewski R. 2003. Drobne ssaki owadożerne i gryzonie. In: Andrzejewski R, editor. Przyroda Kampinoskiego Parku Narodowego. Vol. I. Izabelin: Kampinoski Park Narodowy. pp. 655–663.
- Axnér E, Payan-Carreira R, Setterlind P, Åsbrink J, Söderberg A. 2013. Collection of field reproductive data from carcasses of the female Eurasian lynx (Lynx lynx). Theriogenology 80(8):839–849. DOI:10.1016/j.theriogenology.2013.06.015.
- Biedka A. 2014. Ochrona zwierząt. In: Kronika Kampinoskiego Parku Narodowego 2013. Vol. XLVII. Izabelin.
- Boehme S, Schubert P, Ehrlacher J. 2000. Species conservation of Lynx lynx in the Kampinoski National Park Poland. Unpublished.
- Böer M, Reklewski J, Śmiełowski J, Tyrała P. 1994. Reintroduction of the European lynx to the Kampinoski Nationalpark/Poland-a field experiment with zooborn individuals. Part I: Selection, adaptation and training. Der Zoologische Garten 64(6):366–378.
- Böer M, Reklewski J, Śmiełowski J, Tyrała P. 2000. Reintroduction of the European Lynx to the Kampinoski Nationalpark/Poland-a field experiment with zooborn individuals. Part III: Demographic development of the population from December 1993 until January 2000. Der Zoologische Garten 70(5):304–312.
- Böer M, Śmiełowski J, Tyrała P. 1995. Reintroduction of the European lynx (Lynx lynx) to the Kampinoski National Park, Poland–a field experiment with zooborn individuals part II: Release phase: Procedures and activities of Lynxes during the first year after. Der Zoologische Garten 65(5):333–342.
- Breitenmoser U, Breitenmoser-Würsten C, Capt S, Molinari-Jobin A, Molinari P, Zimmermann F. 2007. Conservation of the Lynx Lynx lynx in the Swiss Jura Mountains. Wildlife Biology 13(4):340–355. DOI:10.2981/0909-6396(2007)13[340:COTLLL]2.0.CO;2.
- Breitenmoser U, Breitenmoser-Würsten C, Okarma H, Kaphegyi T, Kaphygyi U, Müller Wallmann UM. 2000. Action plan for the conservation of the Eurasian lynx in Europe (Lynx lynx). Nature and Environment No. 18-112. Council of Europe.
- Breitenmoser U, Breitenmoser-Würsten C, von Arx M, Zimmermann F, Ryser A, Angst C, Molinari-Jobin A, Molinari P, Linnell J, Siegenthaler A. 2006. Guidelines for the Monitoring of Lynx. Kora Bericht 33:2005–2009.
- Bull JK, Heurich M, Saveljev AP, Schmidt K, Fickel J, Förster DW. 2016. The effect of reintroductions on the genetic variability in Eurasian lynx populations: The cases of Bohemian–Bavarian and Vosges–Palatinian populations. Conservation Genetics 17(5):1229–1234. DOI:10.1007/s10592-016-0839-0.
- Červený J, Krojerová-Prokešová J, Kušta T, Koubek P. 2019. The change in the attitudes of Czech hunters towards Eurasian lynx: Is poaching restricting Lynx population growth? Journal for Nature Conservation 47:28–37. DOI:10.1016/j.jnc.2018.11.002.
- Chapron G, Kaczensky P, Linnell JDC, Von Arx M, Huber D, Andrén H, López-Bao JV, et al. 2014. Recovery of large carnivores in Europe’s modern human-dominated landscapes. Science 346(6216):1517–1519. DOI: 10.1126/science.1257553.
- Cop J, Frkovic A. 1998. The re-introduction of the Lynx in Slovenia and its present status in Slovenia and Croatia. Hystrix 10(1):65–76.
- Cygan P, Kowalski M, Olech B, Sierakowski K, Wasilewski A. 2003. Awifauna lęgowa. In: Andrzejewski R, editor. Przyroda Kampinoskiego Parku Narodowego. Vol. I. Izabelin: Kampinoski Park Narodowy. pp. 621–636.
- Danyłow J. 2010. Reintrodukcja rysia (Lynx lynx) w Kampinoskim Parku Narodowym. In: Wolfram K, editor. NA Tropach rysia on Lynx trails. Supraśl: UROCZYSKO. pp. 75–83.
- Ferreras P. 2001. Landscape structure and asymmetrical inter-patch connectivity in a metapopulation of the endangered Iberian lynx. Biological Conservation 100(1):125–136. DOI:10.1016/S0006-3207(00)00213-5.
- Ferreras P, Delibes M, Palomares F, Fedriani JM, Calzada J, Revilla E. 2004. Proximate and ultimate causes of dispersal in the Iberian Lynx lynx Pardinus. Behavioral Ecology 15(1):31–40. DOI:10.1093/beheco/arg097.
- Garrote G, Fernández–López J, López G, Ruiz G, Simón MA. 2018. Prediction of Iberian lynx road–mortality in southern Spain: A new approach using the MaxEnt algorithm. Animal Biodiversity and Conservation 41(2):217–225. DOI:10.32800/abc.2018.41.0217.
- Herrero A, Klütsch CFC, Holmala K, Maduna SN, Kopatz A, Eiken HG, Hagen SB. 2021. Genetic analysis indicates spatial-dependent patterns of sex-biased dispersal in Eurasian lynx in Finland. Plos One 16(2):e0246833. DOI:10.1371/journal.pone.0246833.
- Heurich M, Schultze-Naumburg J, Piacenza N, Magg N, Červený J, Engleder T, Herdtfelder M, Sladova M, Kramer-Schadt S. 2018. Illegal hunting as a major driver of the source-sink dynamics of a reintroduced Lynx population in Central Europe. Biological Conservation 224:355–365. DOI:10.1016/j.biocon.2018.05.011.
- Jakubowska-Gabara J, Markowski J. 2002. Bolimowski Park Krajobrazowy: Monografia przyrodnicza: Praca zbiorowa. Łódź: Regionalne Centrum Edukacji Ekologicznej.
- Jędrzejewski W, Jędrzejewska B, Okarma H, Schmidt K, Bunevich AN, Milkowski L. 1996. Population dynamics (1869–1994), demography, and home ranges of the Lynx in Białowieza Primeval Forest (Poland and Belarus). Ecography 19(2):122–138. DOI:10.1111/j.1600-0587.1996.tb00163.x.
- Jędrzejewski W, Nowak S, Schmidt K, Jędrzejewska B. 2002. Wilk i ryś w Polsce - wyniki inwentaryzacji w 2001 roku. Kosmos 51(4):491–499.
- Jędrzejewski W, Schmidt K, Miłkowski L, Jędrzejewska B, Okarma H. 1993. Foraging by lynx and its role in ungulate mortality: The local (Białowieża Forest) and the Palaearctic viewpoints. Acta Theriologica 38(4):385–403.
- Jule KR, Leaver LA, Lea SEG. 2008. The effects of captive experience on reintroduction survival in carnivores: A review and analysis. Biological Conservation 141(2):355–363. DOI:10.1016/j.biocon.2007.11.007.
- Kaczensky P, Chapron G, von Arx M, Huber D, Andrén H, Linnell J. 2012. Status, management and distribution of large carnivores – Bear, Lynx, Wolf & Wolverine – In Europe. Part 1- Europe summaries. Report: 1-72. A Large Carnivore Initiative for Europe Report prepared forthe European Commission.
- Kloss M. 2003. Zbiorowiska leśne i zaroślowe. In: Andrzejewski R, editor. Przyroda Kampinoskiego Parku Narodowego. Vol. I. Izabelin: Kampinoski Park Narodowy. pp. 285–313.
- Kowalczyk R, Górny M, Schmidt K. 2015. Edge effect and influence of economic growth on Eurasian lynx mortality in the Białowieża Primeval Forest, Poland. Mammal Research 60(1):3–8. DOI:10.1007/s13364-014-0203-z.
- Kowalski M, Misiak J, Reklewski J. 2003. Gatunki ssaków introdukowane do Puszczy Kampinoskiej. In: Andrzejewski R, editor. Przyroda Kampinoskiego Parku Narodowego. Vol. I. Izabelin: Kampinoski Park Narodowy. pp. 675–684.
- Kramer-Schadt S, Kaiser TS, Frank K, Wiegand T. 2011. Analyzing the effect of stepping stones on target patch colonisation in structured landscapes for Eurasian Lynx. Landscape Ecology 26(4):501–513. DOI:10.1007/s10980-011-9576-4.
- Kramer-Schadt S, Revilla E, Wiegand T. 2005. Lynx reintroductions in fragmented landscapes of Germany: Projects with a future or misunderstood wildlife conservation? Biological Conservation 125(2):169–182. DOI:10.1016/j.biocon.2005.02.015.
- Kramer‐Schadt S, Revilla E, Wiegand T, Breitenmoser U. 2004. Fragmented landscapes, road mortality and patch connectivity: Modelling influences on the dispersal of Eurasian lynx. Journal of Applied Ecology 41(4):711–723. DOI:10.1111/j.0021-8901.2004.00933.x.
- Krzywiński A, Kobus A, Jakimiuk S. 2012. Reintrodukcja Rysia Do Puszczy Piskiej Metodą ‘born to be free’. Ochrona Gatunkowa Rysia, Wilka i Niedzwiedzia w Polsce. Raport z Projektu Nr PL 349:129–145.
- Kubala J, Smolko P, Zimmermann F, Rigg R, Tám B, Iľko T, Foresti D, Breitenmoser-Würsten C, Kropil R, Breitenmoser U. 2019. Robust monitoring of the Eurasian lynx Lynx lynx in the Slovak Carpathians reveals lower numbers than officially reported. Oryx 53(3):548–556. DOI:10.1017/S003060531700076X.
- Lesiński G, Romanowski J, Gryz J, Olszewski A, Kowalski M, Krauze-Gryz D, Olech B, Pepłowska-Marczak D, Tarłowski A. 2013. Small mammals of Kampinos National Park and its protection zone, as revealed by analyses of the diet of tawny owls Strix aluco Linnaeus, 1758. Fragmenta Faunistica 56(1):65–81. DOI:10.3161/00159301FF2013.56.1.065.
- Linnell JDC, Breitenmoser U, Breitenmoser‐Würsten C, Odden J, von Arx M. 2009. Recovery of Eurasian lynx in Europe: What part has reintroduction played? In: Conservation science and practice series. Reintroduction of top-order predators. Hayward MW, Somers MJ, editors. Wiley-Blackwell. pp. 72–91
- Mueller SA, Reiners TE, Middelhoff TL, Anders O, Kasperkiewicz A, Nowak C. 2020. The rise of a large carnivore population in Central Europe: Genetic evaluation of Lynx reintroduction in the Harz Mountains. Conservation Genetics 21(3):577–587.
- Mysłajek RW, KwiatkowskaI, Diserens TA, Haidt A, Nowak S. 2019. Occurrence of Eurasian lynx in Western Poland after two decades of strict protection. CATnews 69:12–13.
- Niedziałkowska M, Jędrzejewski W, Mysłajek RW, Nowak S, Jędrzejewska B, Schmidt K. 2006. Environmental correlates of Eurasian lynx occurrence in Poland–Large scale census and GIS mapping. Biological Conservation 133(1):63–69. DOI:10.1016/J.BIOCON.2006.05.022.
- Nilsen EB, Linnell JDC, Odden J, Samelius G, Henrik A. 2012. Patterns of variation in reproductive parameters in Eurasian lynx (Lynx lynx). Acta Theriologica 57(3):217–223. DOI:10.1007/s13364-011-0066-5.
- Nowak S, Kasprzak A, Mysłajek RW, Tomczak P. 2013. Stwierdzenia rysia Lynx lynx w Puszczy Noteckiej. Przeglad Przyrodniczy 24:4.
- Nowicki A, Kowalski M. 2003. Ssaki kopytne. In: Andrzejewski R, editor. Przyroda Kampinoskiego Parku Narodowego. Vol. I. Izabelin: Kampinoski Park Narodowy. pp. 665–673.
- Okarma H, Jędrzejewski W, Schmidt K, Kowalczyk R, Jędrzejewska B. 1997. Predation of Eurasian lynx on roe deer and red deer in Białowieża Primeval Forest, Poland. Acta theriologica 42:203–234. DOI:10.4098/AT.arch.97-22.
- Olszewski A. 2016. Zastosowanie fotopułapek jako nieinwazyjnej metody badania zwierząt w Kampinoskim Parku Narodowym - pierwsze wyniki. Studia i Materialy Centrum Edukacji Przyrodniczo-Lesnej 18 no. 4[49A]:42-49.
- Ovenden TS, Palmer SCF, Travis JMJ, Healey JR. 2019. Improving reintroduction success in large carnivores through individual-based modelling: How to reintroduce Eurasian lynx (Lynx lynx) to Scotland. Biological Conservation 234:140–153. DOI:10.1016/j.biocon.2019.03.035.
- Reklewski J. 2006. Ryś w Puszczy Kampinoskiej. Izabelin: Kampinoski Park Narodowy.
- Romanowski J. 2007. Vistula River Valley as the ecological corridor for mammals. Polish Journal of Ecology 55:4.
- Romanowski J. 2014. Strategia ochrony reintrodukowanej populacji rysia (Lynx lynx) w Kampinoskim Parku Narodowym. Unpublished.
- Rysie.org. 2020. Powrót rysia do północno-zachodniej Polski. Podróżnik Pako. Rysie.org. Available: http://www.rysie.org/news/article/podroznik-pako Accessed May 2020 22
- Schadt S, Knauer F, Kaczensky P, Revilla E, Wiegand T, Trepl L. 2002. Rule‐based assessment of suitable habitat and patch connectivity for the Eurasian lynx. Ecological Applications 12(5):1469–1483. DOI:10.1890/1051-0761(2002)012[1469:RBAOSH]2.0.CO;2.
- Schmidt K. 2011. Program ochrony rysia Lynx lynx w Polsce – Projekt. SGGW, Warszawa 1–56.
- Schmidt K. 1998. Maternal behaviour and juvenile dispersal in the Eurasian lynx. Acta Theriologica 43(4):391–408. DOI:10.4098/AT.arch.98-50.
- Schmidt K. 2008. Factors shaping the Eurasian lynx (Lynx lynx) population in the North- Eastern Poland. Nature Conservation 65:3–15.
- Schmidt K, Ratkiewicz M, Konopiński MK. 2011. The importance of genetic variability and population differentiation in the Eurasian lynx Lynx lynx for conservation, in the context of habitat and climate change. Mammal Review 41(2):112–124. DOI:10.1111/j.1365-2907.2010.00180.x.
- Schmidt-Posthaus H, Breitenmoser-Wörsten C, Posthaus H, Bacciarini L, Breitenmoser U. 2002. Causes of mortality in reintroduced Eurasian lynx in Switzerland. Journal of Wildlife Diseases 38(1):84–92. DOI:10.7589/0090-3558-38.1.84.
- Simón MA, Gil-Sánchez JM, Ruiz G, Garrote G, McCain EB, Fernández L, López-Parra M, Rojas E, Arenas-Rojas R, Del Rey T, García-Tardío M, López G. 2012. Reverse of the decline of the endangered Iberian lynx. Conservation Biology 26(4):731–736. DOI:10.1111/j.1523-1739.2012.01871.x.
- Sindičić M, Polanc P, Gomerčić T, Jelenčič M, Huber Đ, Trontelj P, Skrbinšek T. 2013. Genetic data confirm critical status of the reintroduced dinaric population of Eurasian lynx. Conservation Genetics 14(5):1009–1018. DOI:10.1007/s10592-013-0491-x.
- Thulin C-G, Röcklinsberg H. 2020. Ethical considerations for wildlife reintroductions and rewilding. Frontiers in Veterinary Science 7:163. DOI:10.3389/fvets.2020.00163.
- Vandel J-M, Stahl P, Herrenschmidt V, Marboutin E. 2006. Reintroduction of the Lynx into the Vosges Mountain Massif: From animal survival and movements to population development. Biological Conservation 131(3):370–385. DOI:10.1016/j.biocon.2006.02.012.
- von Arx M. 2018. IUCN red list of threatened species: Eurasian lynx. Available: IUCN Red List of Threatened Species. https://www.iucnredlist.org/en Accessed May 2020 6
- von Arx M, Breitenmoser-Würsten C, Zimmermann F, Breitenmoser U. 2004. Status and Conservation of the Eurasian lynx (Lynx lynx) in Europe in 2001. KORA Bericht No. 19: 1-330.
- Wacnik A, Ralska-Jasiewiczowa M, Madeyska E. 2011. Late glacial and holocene history of vegetation in Gostynin Area, Central Poland. Acta Palaeobot 51(2):249–278.
- Wilson SM 2018. Lessons learned from past reintroduction and translocation efforts with an emphasis on carnivores. Life Lynx. Available: https://Www.Lifelynx.Eu/Wpcontent/Uploads/2018/10/Lessons-Carnivore-Reintroduction-Efforts-Final-Version-4.0-2018.Pdf Accessed May 2020 7
- Wölfl M, Bufka L, Červenỳ J, Koubek P, Heurich M, Habel H, Huber T, Poost W. 2001. Distribution and status of Lynx in the border region between Czech Republic, Germany and Austria. Acta Theriologica 46(2):181–194. DOI:10.4098/AT.arch.01-20.
- Wölfl S, Schwaiger M. 2017. Luchs-Monitoring mittels Fotofallen. Fotofalleneinsatz in Ostbayern im Monitoringjahr 2016/2017. Bayerisches Landesamt für Umwelt. Augsburg.