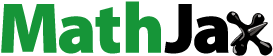
Abstract
Human-induced biological introductions pose a major threat to global biodiversity, and this is especially frequent in the eastern Mediterranean region, which is a globally important biodiversity hotspot area of high conservation value. To predict at which level introduced species in this region might become invasive under current and projected climate conditions, 232 non-native aquatic organisms were screened using the Aquatic Species Invasiveness Screening Kit. Based on receiver operative characteristic curve analysis, thresholds were identified to distinguish between low, medium and high risk species. The “top invasive” (very high risk) species identified were: brown bullhead Ameiurus nebulosus, blue crab Callinectes sapidus, gibel carp Carassius gibelio, Philippine catfish Clarias batrachus, Chinese mitten crab Eriocheir sinensis, bluespotted cornetfish Fistularia commersonii, silver carp Hypophthalmichthys molitrix, silver-cheeked toadfish Lagocephalus sceleratus, half-smooth golden pufferfish Lagocephalus spadiceus, Suez pufferfish Lagocephalus suezensis, signal crayfish Pacifastacus leniusculus, fathead minnow Pimephales promelas, channeled applesnail Pomacea canaliculata, red swamp crayfish Procambarus clarkii, devil firefish Pterois miles and European catfish Silurus glanis. The risk of being invasive of more than half of the screened species increased after taking global warming predictions into account, and several species considered to be globally invasive (cf. high risk) were classified as posing only a medium risk for the eastern Mediterranean region. Region-specific risk screenings, as implemented in this study, are therefore essential for setting priorities in preventative management for the conservation of key biodiversity hotspots and the optimal allocation of resources in view of full risk assessment for the species identified as (very) high risk.
1 Introduction
The number of introduced non-native species (NNS) worldwide has increased exponentially in recent times (Hulme et al. Citation2008, Citation2009; Vilà et al. Citation2010). As a result, species invasions are now widely recognised as a major threat to biodiversity that leads to biotic homogenisation at large spatial scales (McKinney & Lockwood Citation1999; Villéger et al. Citation2011) and represents a major contributor to animal extinctions (Clavero & García-Berthou Citation2005; Clavero et al. Citation2009). The threats posed by invasive NNS include both direct impacts due to predation, competition, hybridisation, habitat modification and transmission of novel diseases (Gozlan et al. Citation2006; McDowall Citation2006; Yonekura et al. Citation2007; Hatcher et al. Citation2012; Muhlfeld et al. Citation2014), and indirect impacts related to economic costs and associated risks (Andersen et al. Citation2004; Gozlan et al. Citation2010).
The Mediterranean region is one of the world’s main aquatic biodiversity hotspots (Coll et al. Citation2010, Citation2012; Lejeusne et al. Citation2010; Tedesco et al. Citation2012) and one of the longest human-inhabited and most densely populated regions in the world due to its suitable climate for agricultural products. This long history of elevated human settlement has resulted in dramatic habitat alteration, water pollution, high levels of water extraction and regulation, shipping, aquaculture, as well as deliberate and accidental introductions of 500+ NNS (Katsanevakis et al. Citation2014; Nunes et al. Citation2015). In the Mediterranean Sea, there are ≈ 17,000 species with a high rate (20–30%) of endemism (Coll et al. Citation2010) and several ecological hotspots with high concentration of endangered, threatened or vulnerable species, as in the case of most western Mediterranean shelves (e.g. Strait of Gibraltar and Alboran Sea, north-west African coasts, Adriatic and Aegean seas: Coll et al. Citation2010). The Mediterranean region hosts several range-restricted species (e.g. Barbieri et al. Citation2015) and some 350 freshwater fish species of which nearly two thirds are endemic (Smith et al. Citation2014). Of these species, more than 40% are currently classified as “threatened with extinction” due to their restricted distribution and habitat requirements, which makes them of high conservation value. Also, despite its predominant semi-arid/arid conditions, the eastern Mediterranean region (EMR) hosts a large number of freshwater species (Smith et al. Citation2014) most of which are endemic and threatened by water extraction, dams, pollution and NNS introductions, with fish and crustacean decapods being the most detrimentally affected (Smith et al. Citation2014). Further, within the EMR, the Levantine Basin is highly dominated by the invasion of species entering via the Suez Canal (Galil Citation2011; Edelist et al. Citation2013; Katsanevakis et al. Citation2014; Rilov et al. Citation2018).
The application of decision-support tools to identify and prioritise potentially-invasive NNS is a crucial step in the risk analysis process, which involves: risk identification (= screening), comprehensive risk assessment, risk management, and risk communication (e.g. Copp et al. Citation2016a). These tools, which aim to assess and quantify invasion risks, can provide information about key recipient areas of NNS introductions to aid implementation of surveillance and prevention measures, such as those for compliance with the European Regulation 1143/2014 on the prevention and management of the introduction and spread of invasive alien species (European Commission Citation2014). The purpose of the present study was to evaluate the level of risk of invasiveness of a variety of aquatic (i.e. freshwater, brackish and marine) organisms for the EMR by collating into a meta-analysis a number of risk screening applications for several risk assessment (RA) areas in this region. This study has therefore special relevance for the increasingly impacted EMR, which is subject to multiple invasion threats and pathways of introduction, thus requiring a greater understanding of NNS risks to inform appropriate management and policy actions. As these fall within the remit of environmental managers and stakeholders, the outcomes of this study are expected to inform guidelines for the optimal allocation of resources with regard to full RA for the species identified as (very) high risk. In this respect, it must be emphasised that the present study will focus on the first step of the NNS risk analysis process, namely “risk screening” (i.e. hazard identification), which aims to identify the NNS that are likely to pose an elevated threat to native species and ecosystems and, therefore, warrant more comprehensive, i.e. full, RA (Copp et al. Citation2005b).
2 Materials & methods
2.1 Risk screening
Risk screenings were carried out by one or two assessors on a range of aquatic taxa, including reptiles, fishes, invertebrates, plants, protists, and bacteria (Supplementary Material, Table S1) for nine RA areas, either entirely or partially within the EMR (), and consisting of extensive geographical areas, regions, countries, water bodies, and marine regions. The risk screenings were undertaken using a multilingual, taxon-generic decision-support tool, namely the Aquatic Species Invasiveness Screening Kit (AS-ISK: www.cefas.co.uk/nns/tools/), which is described in detail elsewhere (Copp et al. Citation2016b, Citation2021). Briefly, the AS-ISK consists of 55 questions of which the first 49 comprise the Basic Risk Assessment (BRA) and the last six the Climate Change Assessment (CCA). To achieve a valid screening, for each question the assessor must provide a response, a level of confidence in their response, and a justification. Upon completion of the screening, the species receives both a BRA score and a BRA+CCA (composite) score (ranging from −20 to 68 and from −32 to 80, respectively). Scores < 1 suggest that the species is unlikely to become invasive (sensu Copp et al., Citation2005b) in the RA area and is therefore classified as “low risk” (Pheloung et al. Citation1999). Higher scores classify the species as posing either a “medium risk” or a “high risk” of becoming invasive. Distinction between medium- and high-risk levels depends upon setting a “threshold” value (see Statistical analysis). Within the high-risk species, a further distinction is generally made of the “very high risk” species (i.e. based on an ad hoc threshold weighted according to the range of high-risk score values for both the BRA and BRA+CCA), which are then prioritised in terms of allocation of resources for a full RA (e.g. Clarke et al. Citation2020; Interesova et al. Citation2020; Killi et al. Citation2020; Uyan et al. Citation2020; Moghaddas et al. Citation2021; Radočaj et al. Citation2021; Ruykys et al. Citation2021; Wei et al. Citation2021).
Table I. Risk assessment (RA) areas either entirely or partially within the eastern Mediterranean region (EMR) for which aquatic non-native species were screened with the Aquatic Species Invasiveness Screening Kit (AS-ISK). The type of RA area is indicated together with the assessor(s) (initials from authors of this study) and the number of species screened (Screenings)
The ranked levels of confidence (1 = low; 2 = medium; 3 = high; 4 = very high) associated with each response in the AS-ISK mirror the confidence rankings recommended by the Intergovernmental Panel on Climate Change (IPCC Citation2005; see also Copp et al. Citation2016a). Based on the confidence level (CL) allocated to each response, a confidence factor (CF) is obtained as:
where CLQi is the CL for Question i (Qi), 4 is the maximum achievable value for confidence (i.e. very high: see above) and 55 is the total number of questions comprising the AS-ISK questionnaire. The CF ranges from a minimum of 0.25 (i.e. all 55 questions with confidence level equal to 1) to a maximum of 1 (i.e. all 55 questions with confidence level equal to 4). Based on all 55 Qs of the AS-ISK questionnaire, the 49 Qs comprising the BRA and the six Qs comprising the CCA: for the CL, the CLTotal, CLBRA and CLCCA are computed, respectively; and for the CF, the CFTotal, CFBRA and CFCCA.
2.2 Statistical analysis
Screenings consisted of individual contributions by assessors involved in the evaluation of one (or more) aquatic NNS within their field of expertise and of published/unpublished applications for a certain RA area, which was either part of or included the EMR (). In the latter case (e.g. Tarkan et al. Citation2017a, 2017b; Bilge et al. Citation2019; Killi et al. Citation2020), the selection of species included both extant (i.e. already present in the RA area) and “horizon” ones (i.e. likely to enter the RA in the near future). Following computation of the BRA and BRA+CCA scores, Receiver Operating Characteristic (ROC) analysis (Bewick et al. Citation2004) was used to evaluate the predictive ability of the AS-ISK to discriminate between species posing a high risk and those posing a medium or low risk of being invasive for the RA area. For ROC analysis to be implemented, species must be categorised a priori in terms of their documented invasiveness (i.e. non-invasive or invasive). The a priori categorisation followed the protocol described in Clarke et al. (Citation2020).
A ROC curve is a graph of sensitivity vs 1 – specificity for each threshold value, where in the present context sensitivity and specificity will be the proportion of a priori invasive and non-invasive species, respectively, that are correctly identified by the AS-ISK as such. A measure of the accuracy of the calibration analysis is the Area Under the Curve (AUC), with AUC values interpreted as follows: 0.7 ≤ AUC < 0.8 = acceptable discriminatory power, 0.8 ≤ AUC < 0.9 = excellent, 0.9 ≤ AUC = outstanding (Hosmer et al. Citation2013). If the AUC is equal to 1, then there are neither “false positives” (i.e. a priori non-invasive species classified as high risk, hence invasive) nor “false negatives” (i.e. a priori invasive species classified as low or medium risk, hence non-invasive). Conversely, if the AUC is equal to 0.5, then the test cannot discriminate between “true positives” (i.e. a priori invasive species classified as high risk, hence invasive) and “true negatives” (i.e. a priori non-invasive species classified as low or medium risk, hence non-invasive).
Following ROC analysis, the best threshold value that maximises the true positive rate and minimises the false positive rate was determined using Youden’s J statistic; whereas, the “default” threshold of 1 was set to distinguish between low-risk and medium-risk species (Copp et al. Citation2005b). ROC analysis was implemented with package pROC (Robin et al. Citation2011) for R x64 v3.6.3 (R Development Core Team Citation2020) using 2000 bootstrap replicates for the confidence intervals of specificities, which were computed along the entire range of sensitivity points (i.e. 0 to 1, at 0.1 intervals). Group-specific thresholds were estimated for those groups of aquatic organisms for which a representative sample size and “near-balanced” proportion of a priori categorised native and non-native species for successful ROC curve computation was available; whereas for the other groups with small sample sizes, similar to Clarke et al. (Citation2020) the threshold for the combined “nearest” taxonomic group was used whenever possible (i.e. AUC > 0.5), else a global threshold was computed based on pooling together all species screened.
3 Results
In total, 303 screenings were carried out by 26 assessors on 232 taxa comprising 228 species, three sub-species and one hybrid (Supplementary Material, Table S1) for the nine RA areas (). Notably, the number of screenings was larger than the number of taxa as some of these were screened by more than one assessor and/or for more than one RA area (Supplementary Material, Table S2). The 232 screened taxa (hereafter, loosely referred to as “species”) consisted of the following aquatic organism groups: reptiles, freshwater and marine fishes, freshwater, brackish and marine invertebrates, freshwater and marine plants, marine protists and marine bacteria (Supplementary Material, Table S1). However, most of the screened species were freshwater and marine fishes and marine invertebrates, with the other groups including one or two species except for freshwater invertebrates ().
Table II. Taxonomic group-specific thresholds (Thr) for the Basic Risk Assessment (BRA) and the BRA plus Climate Change Assessment (BRA+CCA) for the aquatic non-native species screened with AS-ISK for the EMR (see Appendix Table A1). Mean, lower confidence interval (LCI) and upper confidence interval (UCI) for the Area Under the Curve (AUC) are provided. In italics thresholds and corresponding AUCs and CIs based on pooling with other groups (see footnotes)
The BRA scores ranged from −15.0 (“türgi hink” Cobitis kurui for Lake Marmara) to 55.0 (Chinese mitten crab Eriocheir sinensis for the Mediterranean Sea; red swamp crayfish Procambarus clarkii for southern Europe), with mean = 14.4, median = 12.5, and 5th and 95th percentiles equal to −12.0 and 41.0 (). The BRA+CCA scores ranged from −27.0 (Cobitis kurui) to 67.0 (Procambarus clarkii), with mean = 17.0, median = 15.0, and 5th and 95th percentiles equal to −20.0 and 52.0 (). The CCA increased the BRA score in 172 (56.8%) of the screenings, decreased it in 55 (18.2%), and did not change in the remaining 76 (25.0%) (). Also, 33 species (16.3% of the total) achieved the largest positive change in score of 12 when CCA scores were included, and 17 (8.4%) the largest negative change in score of −12 (Supplementary Material, Tables S3 and S4).
Figure 1. (a) Frequency distribution of the Basic Risk Assessment (BRA) scores for the aquatic NNS screened with the Aquatic Species Invasiveness Screening Kit (AS-ISK) for the eastern Mediterranean region; (b) same for the BRA+CCA (Climate Change Component) scores; (c) same for delta values (i.e. differences between the BRA+CCA and the BRA scores) with proportions
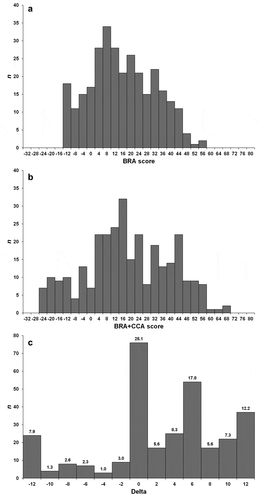
Following ROC analysis, BRA and BRA+CCA thresholds were computed for the three most abundant groups of aquatic organisms, namely freshwater and marine fishes, and marine invertebrates. However, threshold computation for freshwater fishes excluded the screenings for Lake Marmara (Supplementary Material, Table S4). This is because the dataset included mainly translocated species, the majority of which had very low (outlier) scores, which would have biased thresholds towards consistently lower levels. For freshwater and brackish invertebrates, a combined threshold was computed based on all invertebrate species screened; whereas, for reptiles, freshwater and marine plants, marine protists and marine bacteria, a combined threshold was computed based on all aquatic species screened (again excluding the Lake Marmara dataset). All resulting AUCs had near-excellent to excellent discriminatory power (), indicating that the AS-ISK was able to discriminate reliably between non-invasive and invasive species for the RA area. Youden’s J provided BRA thresholds ranging from 5.5 (marine invertebrates). This was also the case for freshwater and brackish invertebrates, based on the combined one) to 25.5 (freshwater fishes), with a threshold of 15.25 for all other aquatic organism groups. BRA+CCA thresholds ranged from 7.0 (marine invertebrates) to 28.75 (freshwater fishes), with a threshold of 19.25 for all other aquatic organism groups.
The above group-specific thresholds were used for calibration of the risk outcomes at the aquatic organism-group level. Accordingly, of the 216 species screened in total (i.e. excluding Lake Marmara):
based on the BRA thresholds:
99 (45.8%) species were classified as high risk, 97 (44.9%) as medium risk, and 20 (9.3%) as low risk;
of the 114 species categorised a priori as invasive, 77 were true positives, two false negatives (i.e. Cotylorhiza erythraea and Caucasian dwarf goby Knipowitschia caucasica), and 35 medium risk;
of the 102 species categorised a priori as non-invasive, 22 were false positives, 18 true negatives, and 62 medium risk;
based on the BRA+CCA thresholds:
120 (55.6%) species were classified as high risk, 77 (35.6%) as medium risk, and 19 (8.8%) as low risk;
of the 114 species categorised a priori as invasive, 86 were true positives, two false negatives (i.e. Atlantic salmon Salmo salar and Knipowitschia caucasica), and 26 medium risk;
of the 102 species categorised a priori as non-invasive, 34 were false positives, 17 true negatives, and 51 medium risk.
According to the BRA scores, 24 (24.2%) of the 99 high-risk species and 8 (8.2%) of the 97 medium-risk species are listed in the Global Invasive Species Database (GISD: www.iucngisd.org). According to the BRA+CCA scores, 28 (13.0%) of the 120 high-risk, (3.9%) of the 77 medium-risk, and one of the low-risk (Atlantic salmon Salmo salar) species are listed in the GISD (Supplementary Material, Tables S1 and S2).
The highest-scoring (most likely to be invasive) species (BRA scores ≥ 40 and BRA+CCA scores ≥ 50, respectively, taken as ad hoc “very high risk” thresholds) were: silver carp Hypophthalmichthys molitrix, Suez pufferfish Lagocephalus suezensis, fathead minnow Pimephales promelas, golden apple snail Pomacea canaliculata, red swamp crayfish Procambarus clarkii and devil firefish Pterois miles (for both the BRA and BRA+CCA); brown bullhead Ameiurus nebulosus, blue crab Callinectes sapidus, gibel carp Carassius gibelio, Philippine catfish Clarias batrachus, Chinese mitten crab Eriocheir sinensis, signal crayfish Pacifastacus leniusculus and European catfish (a.k.a. sheatfish) Silurus glanis (for the BRA only); bluespotted cornetfish Fistularia commersonii, silver-cheeked toadfish Lagocephalus sceleratus and half-smooth golden pufferfish L. spadiceus (for the BRA+CCA only) ().
Figure 2. (a) Mean (± SE, whenever applicable) BRA score for the “very high-risk” aquatic NNS screened with AS-ISK for the eastern Mediterranean region based on an ad hoc threshold of 40; (b) same for the BRA+CCA score, based on an ad hoc threshold of 50. In black, very high-risk species for both the BRA and the BRA+CCA
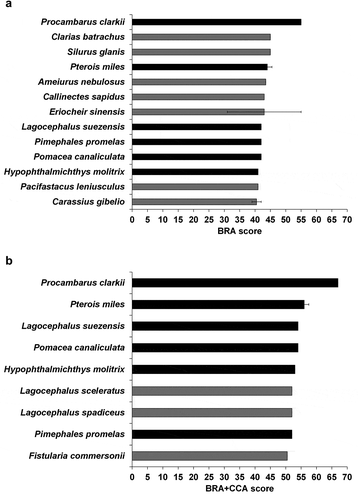
Figure 3. “Top invasive” (i.e. very high risk) species identified for the eastern Mediterranean region
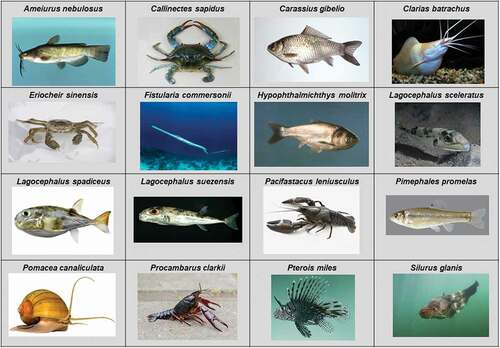
Regarding confidence, the mean CL was 2.51 ± 0.03 SE, the mean CLBRA 2.55 ± 0.03, and the mean CLCCA 2.20 ± 0.04 (hence, in all cases indicating medium to high confidence); the mean CF was 0.628 ± 0.008, the mean CFBRA 0.638 ± 0.008, and the mean CFCCA 0.550 ± 0.011. Also, the CLBRA was significantly higher than CLCCA (F#1,604 = 51.57, P# < 0.001; # = permutational value).
4 Discussion
This screening study has identified 16 species, encompassing freshwater and marine fishes as well as freshwater, brackish and marine invertebrates, that pose the highest risk of being invasive under current and/or future climate conditions in the EMR. Of these species, 13 received a BRA score > 40 and nine a BRA+CCA score > 50 (). These risk rankings mirror those attributed to these species using either the AS-ISK or one of its predecessor toolkits (see Copp Citation2013). For example, the highest-scoring species under both current and future climate conditions, Procambarus clarkii, received the highest score using the FI-ISK (Freshwater Invertebrate Invasiveness Screening Kit) for Italy (Tricarico et al. Citation2010); and the species with the second-highest BRA score, Clarias batrachus, received amongst the highest FISK (Fish Invasiveness Screening Kit) scores for Florida, USA (Lawson et al. Citation2015). The species receiving the second-highest BRA+CCA score, Pterois miles, received the highest AS-ISK scores (both BRA and BRA+CCA) for coastal waters of South Korea (Uyan et al. Citation2020). This similarity of risk rankings for the EMR and freshwater and marine ecosystems worldwide (e.g. Glamuzina et al. Citation2017; Semenchenko et al. Citation2018; Dodd et al. Citation2019; Clarke et al. Citation2020; Interesova et al. Citation2020; Uyan et al. Citation2020) provides additional support for the increasing evidence that this decision-support tool is a reliable, accurate means to discriminate between non-invasive and invasive species for a pre-defined RA area.
The accuracy of the findings for the EMR was evidenced by the very low percentage (0.9%) of false negatives (cf. Kumschick & Richardson Citation2013), and the medium confidence rankings in the responses overall reflected the level of expertise amongst assessors, which in several cases was limited by the scanty literature information available for some of the lesser-studied species (e.g. Tarkan et al. Citation2017a). Also, the overall lower confidence level for climate change predictions (i.e. CCA) compared to the BRA is in accord with other AS-ISK applications (see above) and can be explained by the higher level of uncertainty intrinsic to future climate change projections.
The main vectors for the introduction of the 16 highest-scoring species () are intentional and accidental (species-specific profiles in Supplementary Material, Appendix A1). The intentional introduction vectors include the ornamental trade (Clarias batrachus, Pomacea canaliculata, Procambarus clarkii,) and aquaculture, with both husbandry and stocking practices (Ameiurus nebulosus, Carassius gibelio, Hypophthalmichthys molitrix, Pacifastacus leniusculus, Silurus glanis). Accidental introduction vectors include ballast water transport and discharge (Callinectes sapidus, Eriocheir sinensis), releases of unwanted fish bait (Pimephales promelas), and natural dispersal via shipping canals (Fistularia commersonii, Lagocephalus sceleratus, L. spadiceus, L. suezensis, Pterois miles). This latter phenomenon is also well known for inland waters (Panov et al. Citation2009).
With regard to intentional introductions, most invasive freshwater species, which emanate mainly from Asia, Africa and South America, are introduced in the EMR mainly for aquaculture and the ornamental trade (Tricarico Citation2012; Papavlasopoulou et al. Citation2014; Nunes et al. Citation2015; Emiroğlu et al., Citation2016; Tarkan et al. Citation2017b). Aquaculture is a historical vector for freshwater species and in the EU there is a dedicated Regulation (708/2007 with the following implementation 506/2008, 535/2008, 304/2011) to manage the introduction of NNS for this purpose. The ornamental trade also is becoming an increasingly important introduction vector, as the importation of some of the very high-risk species found in the present study is already prohibited (see below), even though their online trade still remains an important unregulated “gateway” for their introduction (e.g. Mazza et al. Citation2015). In this respect, increased public awareness is an important tool to reduce the introduction of NNS for ornamental purposes (Chan et al. Citation2019).
Regarding accidental introductions, the opening of the Suez Canal has resulted in bioinvasions in the eastern Mediterranean Sea by species from the Red Sea (Por Citation2012) – a process that is generally referred to as the “Lessepsian migration” or the “Erythrean migration”. This is an on-going process responsible for both positive (cf. fishing industry) and negative impacts on the marine ecosystem (Farrag et al. Citation2016). Detrimental impacts are mainly related to the poisonous and traumatogenic properties of several Lessepsian fishes, with neurotoxic and histamine-related effects on humans. Also, several Lessepsian invaders have created ecological niches without competing with extant native species. For example, the “rabbitfish” dusky spinefoot Siganus luridus and marbled spinefoot S. rivulatus (classified as posing a high risk in present study: Supplementary Information, Table S2) initially became well established in the Levantine Basin and then invaded the Central Mediterranean, aided by the absence of herbivorous competitors and/or the presence of abundant food resources (Lundberg & Golani Citation1995; Goren & Galil Citation2005; Galil Citation2007). The impact of these herbivorous rabbitfishes can be extensive due to their grazing activity with dramatic reductions in canopy algae and overall benthic biomass including invertebrates leading to depauperate native communities (Vergés et al. Citation2014; Yeruham et al. Citation2020). The evolution of the Eastern Mediterranean Transient (Theocharis & Lascaratos Citation2000; Galil & Kevrekidis Citation2002), combined with other factors such as unexploited resources and warming of both the Mediterranean Sea in general (Bianchi & Morri Citation2003; Bianchi Citation2007) and of the Aegean Sea in particular (Theocharis Citation2008), has facilitated the maintenance and spread of invading of non-native fishes from the Red Sea (https://elnais.hcmr.gr/). Also, and notably, the Lessepsian invasion is not limited to the EMR (Galil Citation2006) but is undergoing considerable expansion from the south Aegean Sea through to the central Mediterranean and up to the east of Sicily, Adriatic Sea, and even as far as the Western Mediterranean (cf. Lessepsian Province: Por Citation2012).
The increase in the BRA score for more than half of the species after taking climate change projections into account (i.e. CCA), is in line with climate warming predictions for the RA area (e.g. Lelievield et al., Citation2014; Önol & Unal Citation2014; Zittis et al. Citation2016; Givan et al. Citation2017, Citation2018). These warmer conditions would provide even more favourable habitat conditions especially for species of sub-tropical and tropical origin (but see Yeruham et al. Citation2020). This was exemplified in the present study by the Lessepsian fishes Fistularia commersonii, Lagocephalus sceleratus and L. spadiceus all of which ranked as “very high risk” based on the CCA (but not on the BRA) after achieving the corresponding maximum score value of 12 (Supplementary Information, Table S2). On the other hand, most of the species attributed the lowest CCA score (−12) were a priori non-invasive species screened for the RA areas of Lake Marmara and the Aegean Region of Turkey. These species consisted mostly of local and endemic freshwater fish species that have been, or could be, translocated within Turkey and are likely to suffer from projected increases in water temperature (Caddy et al. Citation1995). This finding, of a further increase in the risk of invasiveness of several species under predicted global warming for the EMR, is similar to other screening studies for both fresh waters (e.g. Glamuzina et al. Citation2017, Citation2021; Li et al. Citation2017; Paganelli et al. Citation2018; Semenchenko et al. Citation2018; Dodd et al. Citation2019; Interesova et al. Citation2020; Moghaddas et al. Citation2021) and marine ecosystems (e.g. Lyons et al. Citation2020; Uyan et al. Citation2020; Stasolla et al. Citation2021). On the other hand, this contrasts a recent screening study of aquatic species for the Arabian/Persian Gulf and Sea of Oman (Clarke et al. Citation2020), where the naturally extreme conditions of the region are already at the upper end of the temperature tolerances of several species. In the case of Lessepsian fishes, their ability to colonise the EMR has been shown to depend upon their maximum thermal tolerances in their native areas of distribution, where temperature extremes can in some cases be less pronounced than those sometimes experiences in their invasive range of the Mediterranean (Belmaker et al. Citation2013; Givan et al. Citation2018).
Despite the availability of global databases of invasive species such as the GISD, the outcomes of this study are far from being “trivial” given that only a small percentage of the screened species classified both as high and medium risk for the RA area are listed in the above global database. Species ranked as posing a high risk (or very high risk) of being invasive in the EMR may warrant being subjected to a more comprehensive (i.e. full) RA; this would however entail considerable financial investment (Copp et al. Citation2005a, Citation2016a). This is because a full RA examines in detail the risks of introduction (entry), establishment (of one or more self-sustaining populations), dispersal (more widely within the RA area, i.e. so-called secondary spread or introductions), and impacts (to native biodiversity, ecosystem function and services, and the introduction and transmission of diseases), and is therefore generally limited to the high-risk (or, in some cases, very high risk) species only, as this normally involves a major staff-time (i.e. financial) commitment.
5 Conclusion
The present study has identified potentially-invasive NNS for the EMR, thereby informing NNS policy-makers and environmental managers as to which species require comprehensive risk assessment. The outcomes of a comprehensive risk assessment, which include risk summaries (of entry, establishment, dispersal/spread and impact) as well as the assessor’s confidence in the evidence upon which their responses were based, contribute to the development and implementation of management measures to prevent unwanted introductions, the further spread of high-risk aquatic species already present, and thereby mitigate their impacts (Copp et al. Citation2005a; Baker et al. Citation2008). The conception of NNS management strategies and their implementation are constrained by three main factors, i.e. available resources, species-specific traits and the character of the invaded ecosystem (Britton et al. Citation2011a, Citation2011b). A potential fourth factor is the prevailing institutional and legislative framework of the country or region (Copp et al. Citation2005b). Consideration of these factors is recommended for the development of an effective decision-support system, which should provide a quantitative framework with which to identify the most appropriate management strategy (i.e. prevention and/or control) for each species within the environmental and conservation context of the invaded ecosystem (Britton et al. Citation2011a), the available resources and the likelihood of a management measure being successful (Britton et al. Citation2011b).
An effective NNS risk management strategy is particularly important for the EMR, which is known to be prone to high levels of biological invasion (Galil et al. Citation2018) and where the biological and socio-economic impacts of some NNS could be underestimated due to a lack of relevant information. Also, contrary to the opposite pattern usually observed in aquatic environments (Tricarico et al. Citation2016), the presence of the Suez Canal has resulted in a similar or even higher number of NNS in the Mediterranean Sea than in the Region’s inland waters. As the EMR is continuously prone to changes and the introduction of NNS is steadily increasing, the risk screenings provided in the present study should be updated whenever new data and/or information become available. The outcomes of the present study also emphasise the need to monitor potential further expansion of several Lessepsian species into the western Mediterranean. On a broader perspective, the use of multilingual decision-support tools such as the AS-ISK, which offers assessors all of Mediterranean national languages (Copp et al. Citation2021), provides a means to facilitate collaboration and communication between scientists, fisheries biologists, environmental managers, policy makers and other stakeholders of the Mediterranean Region for the development, prioritisation and implementation of appropriate conservation management strategies for the region’s aquatic habitats, resources, and ecosystem services.
Supplemental Material
Download MS Word (223.4 KB)Acknowledgements
The participation of GHC was made possible by Cefas’ Science Excellence fund.
Disclosure statement
No potential conflict of interest was reported by the author(s).
Supplementary material
Supplemental data for this article can be accessed here.
References
- Andersen MC, Adams H, Hope B, Powell M. 2004. Risk assessment for invasive species. Risk Analysis 24(4):787–793. DOI:10.1111/j.0272-4332.2004.00478.x.
- Baker RHA, Black R, Copp GH, Haysom KA, Hulme PE, Thomas MB, Brown A, Brown M, Cannon RJC, Ellis J, Ellis M, Ferris R, Glaves P, Gozlan RE, Holt J, Howe L, Knight JD, MacLeod A, Moore NP, Mumford JD, Murphy ST, Parrott D, Sansford CE, Smith GC, St-Hilaire S, Ward NL. 2008. The UK risk assessment scheme for all non-native species. In: Rabitsch W, Essl F, Klingenstein F, editors. BiologicalInvasions – from ecology to conservation. Neobiota. Vol. 7. Berlin: Neobiota. pp. 46–57.
- Barbieri R, Zogaris S, Kalogianni E, Stoumboudi MT, Chatzinikolaou Y, Giakoumi S, Kapakos Y, Kommatas D, Koutsikos N, Tachos V, Vardakas L, Economou AN. 2015. Freshwater fishes and lampreys of Greece: An annotated checklist. Monographs on Marine Sciences No. 8. Hellenic Centre for Marine Research: Athens, Greece. Available: https://epublishing.ekt.gr/sites/ektpublishing/files/ebooks/FINAL_MARCH_2015_FreshwaterFishlist-1.pdf
- Belmaker J, Parravicini V, Kulbicki M. 2013. Ecological traits and environmental affinity explain Red Sea fish introduction into the Mediterranean. Global Change Biology 19(5):1373–1382. DOI:10.1111/gcb.12132.
- Bewick V, Cheek L, Ball J. 2004. Statistics review 13: Receiver operating characteristic curves. Critical Care 8(6):508–512. DOI:10.1186/cc3000.
- Bianchi CN. 2007. Biodiversity issues for the forthcoming tropical Mediterranean Sea. Hydrobiologia 580(1):7. DOI:10.1007/s10750-006-0469-5.
- Bianchi CN, Morri C. 2003. Global sea warming and “tropicalization” of the Mediterranean Sea: Biogeographic and ecological aspects. Biogeographia 24.
- Bilge G, Filiz H, Yapıcı S, Tarkan AS, Vilizzi L. 2019. A risk screening study on the potential invasiveness of Lessepsian fishes in the south-eastern coasts of Anatolia. Acta Ichthyologica Et Piscatoria 49(1):23–31. DOI:10.3750/AIEP/02422.
- Britton JR, Copp GH, Brazier M, Davies GD. 2011a. A modular assessment tool for managing introduced fishes according to risks of species and their populations, and impacts of management actions. Biological Invasions 13(12):2847–2860. DOI:10.1007/s10530-011-9967-0.
- Britton JR, Gozlan RE, Copp GH. 2011b. Managing non-native fish in the environment. Fish and Fisheries 12(3):256–274. DOI:10.1111/j.1467-2979.2010.00390.x.
- Caddy JF, Refk R, Do-Chi T. 1995. Productivity estimates for the Mediterranean: Evidence of accelerating ecological change. Ocean & Coastal Management 26(1):1–18. DOI:10.1016/0964-5691(95)00015-T.
- Chan FT, Beatty SJ, Gilles JAS, Hill JE, Kozic S, Luo D, Morgan DL, Pavia RTB, Therriault TW, Verreycken H, Vilizzi L, Wei H, Yeo DCJ, Zeng Y, Zięba G, Copp GH. 2019. Leaving the fish bowl: The ornamental trade as a global vector for freshwater fish invasions. Aquatic Ecosystem Health & Management 22(4):417–439. DOI:10.1080/14634988.2019.1685849.
- Clarke SA, Vilizzi L, Lee L, Wood LE, Cowie WJ, Burt JA, Mamiit RJE, Ali H, Davison PI, Fenwick GV, Harmer R, Skóra M, Kozic S, Aislabie LR, Kennerley A, Le Quesne W, Copp GH, Stebbing PD. 2020. Identifying potentially invasive non-native marine and brackish water species for the Arabian Gulf and Sea of Oman. Global Change Biology 26(4):2081–2092. DOI:10.1111/gcb.14964.
- Clavero M, Brotons L, Pons P, Sol D. 2009. Prominent role of invasive species in avian biodiversity loss. Biological Conservation 142(10):2043–2049. DOI:10.1016/j.biocon.2009.03.034.
- Clavero M, García-Berthou E. 2005. Invasive species are a leading cause of animal extinctions. Trends in Ecology & Evolution 20(3):110. DOI:10.1016/j.tree.2005.01.003.
- Coll M, Piroddi C, Albouy C, Ben Rais Lasram F, Cheung WWL, Christensen V, Karpouzi VS, Guilhaumon F, Mouillot D, Paleczny M, Palomares ML. 2012. The Mediterranean Sea under siege: Spatial overlap between marine biodiversity, cumulative threats and marine reserves. Global Ecology and Biogeography 21(4):465–481. DOI:10.1111/j.1466-8238.2011.00697.x.
- Coll M, Piroddi C, Steenbeek J, Kaschner K, Ben Rais Lasram F, Aguzzi J, Ballesteros E, Bianchi CN, Corbera J, Dailianis T, Danovaro R. 2010. The biodiversity of the Mediterranean Sea: Estimates, patterns, and threats. PLoS One 5:article e11842.
- Copp GH. 2013. The Fish Invasiveness Screening Kit (FISK) for non-native freshwater fishes – A summary of current applications. Risk Analysis 33(8):1394–1396. DOI:10.1111/risa.12095.
- Copp GH, Bianco PG, Bogutskaya NG, Erős T, Falka I, Ferreira MT, Fox MG, Freyhof J, Gozlan RE, Grabowska J, Kováč V, Moreno-Amich R, Naseka AM, Peňáz M, Povž M, Przybylski M, Robillard M, Russell IC, Stakėnas S, Šumer S, Vila-Gispert A, Wiesner C. 2005b. To be, or not to be, a non-native freshwater fish? Journal of Applied Ichthyology 21(4):242–262. DOI:10.1111/j.1439-0426.2005.00690.x.
- Copp GH, Garthwaite R, Gozlan RE 2005a. Risk identification and assessment of non-native freshwater fishes: Concepts and perspectives on protocols for the UK. Cefas Science Technical Report. Lowestoft, UK: Cefas. Available: www.cefas.co.uk/publications/techrep/tech129.pdf
- Copp GH, Russell IC, Peeler EJ, Gherardi F, Tricarico E, Macleod A, Cowx IG, Nunn D, Occhipinti-Ambrogi A, Savini D, Mumford J, Britton JR. 2016b. European non-native species in aquaculture risk analysis scheme – a summary of assessment protocols and decision support tools for use of alien species in aquaculture. Fisheries Management and Ecology 23(1):1–11. DOI:10.1111/fme.12074.
- Copp GH, Vilizzi L, Tidbury H, Stebbing PD, Tarkan AS, Miossec L, Goulletquer P. 2016a. Development of a generic decision-support tool for identifying potentially invasive aquatic taxa: AS-ISK. Management of Biological Invasions 7(4):343–350. DOI:10.3391/mbi.2016.7.4.04.
- Copp GH, Vilizzi L, Wei H, Li S, Piria M, Al-Faisal AJ, Almeida D, Atique U, Al-Wazzan Z, Bakiu R, Bašić T, Bui TD, Canning-Clode J, Castro N, Chaichana R, Çoker T, Dashinov D, Ekmekçi FG, Erős T, Ferincz Á, Ferreira T, Giannetto D, Gilles Jr AS, Głowacki Ł, Goulletquer P, Interesova E, Iqbal S, Jakubčinová K, Kanongdate K, Kim J-E, Kopecký O, Kostov V, Koutsikos N, Kozic S, Kristan P, Kurita Y, Lee H-G, Leuven RSEW, Lipinskaya T, Lukas J, Marchini A, González Martínez AI, Masson L, Memedemin D, Moghaddas SD, Monteiro J, Mumladze L, Naddafi R, Năvodaru I, Olsson KH, Onikura N, Paganelli D, Pavia Jr, RT, Perdikaris C, Pickholtz R, Pietraszewski D, Povž M, Preda C, Ristovska M, Rosíková K, Santos JM, Semenchenko V, Senanan W, Simonović P, Smeti E, Števove B, Švolíková K, Ta KAT, Tarkan AS, Top N, Tricarico E, Uzunova E, Vardakas L, Verreycken H, Zięba G, Mendoza R. 2021. Speaking their language – Development of a multilingual decision-support tool for communicating invasive species risks to decision makers and stakeholders. Environmental Modelling and Software 135:article 104900. DOI:10.1016/j.envsoft.2020.104900.
- Dodd JA, Vilizzi L, Bean CW, Davison PI, Copp GH. 2019. At what spatial scale should risk screenings of translocated freshwater fishes be undertaken – River basin district or climo-geographic designation? Biological Conservation 230:122–130. DOI:10.1016/j.biocon.2018.12.002.
- Edelist D, Rilov G, Golani D, Carlton JT, Spanier E. 2013. Restructuring the Sea: Profound shifts in the world’s most invaded marine ecosystem. Diversity & Distributions 19(1):69–77. DOI:10.1111/ddi.12002.
- Emiroğlu Ö, Ekmekçi FG, Aksu S, Başkurt S, Atalay MA, Tarkan AS. 2016. Introduction and establishment of tropical ornamental fish, Pterygoplichthys spp. (Actinopterygii: Siluriformes: Loricariidae) in hot springs: Aquarium trade as a potential risk for biodiversity in Turkey. Acta Ichthyologica Et Piscatoria 46(4):351–356. DOI:10.3750/AIP2016.46.4.07.
- European Commission. 2014. Regulation (EU) No 1143/2014 of the European Parliament and of the Council of 22 October 2014 on the prevention and management of the introduction and spread of invasive alien species. Official Journal of the European Union. Available: http://eur-lex.europa.eu/legal-content/EN/TXT/?uri=OJ:JOL_2014_317_R_0003
- Farrag M, El-Haweet AA, Akel E-S. 2016. Occurrence of puffer fishes (Tetraodontidae) in the eastern Mediterranean, Egyptian coast – filling in the gap. BioInvasions Records 5(1):47–54. DOI:10.3391/bir.2016.5.1.09.
- Galil BS. 2006. The marine caravan–the Suez Canal and the Erythrean invasion. In: Gollasch S, Galil BS, Cohen AN, editors. Bridging divides. Dordrecht: Springer. pp. 207–300.
- Galil BS. 2007. Loss or gain? Invasive aliens and biodiversity in the Mediterranean Sea. Marine Pollution Bulletin 55(7–9):314–322. DOI:10.1016/j.marpolbul.2006.11.008.
- Galil BS. 2011. The alien crustaceans in the Mediterranean Sea: An historical review. In: Galil B, Clark P, Carlton J, editors. In the wrong place – Alien marine crustaceans: Distribution, biology and impacts. Invading Nature – Springer Series in Invasion Ecology, Vol. 6. Dordrecht: Springer. pp. 377–401
- Galil BS, Kevrekidis K. 2002. Exotic decapods and a stomatopod off Rhodes Island (Greece) and the Eastern Mediterranean Transient. Crustaceana 75(7):925–930. DOI:10.1163/156854002321210758.
- Galil BS, Marchini A, Occhipinti-Ambrogi A. 2018. East is east and west is west? Management of marine bioinvasions in the Mediterranean Sea. Estuarine, Coastal and Shelf Science 201:7–16. DOI:10.1016/j.ecss.2015.12.021.
- Givan O, Edelist D, Sonin O, Belmaker J. 2018. Thermal affinity as the dominant factor changing Mediterranean fish abundances. Global Change Biology 24(1):e80–e89. DOI:10.1111/gcb.13835.
- Givan O, Parravicini V, Kulbicki M, Belmaker J. 2017. Trait structure reveals the processes underlying fish establishment in the Mediterranean. Global Ecology and Biogeography 26(2):142–153. DOI:10.1111/geb.12523.
- Glamuzina B, Tutman P, Glamuzina L, Vidović Z, Simonović P, Vilizzi L. 2021. Quantifying current and future risks of invasiveness of non-native aquatic species in highly urbanised estuarine ecosystems–A case study of the River Neretva Estuary (Eastern Adriatic Sea: Croatia and Bosnia–Herzegovina). Fisheries Management and Ecology 28:138–146. DOI:10.1111/fme.12463.
- Glamuzina B, Tutman P, Nikolić V, Vidović Z, Pavlicević J, Vilizzi L, Copp GH, Simonović P. 2017. Comparison of taxon-specific and taxon-generic risk screening tools to identify potentially invasive non-native fishes in the River Neretva catchment (Bosnia and Herzegovina and Croatia). River Research and Applications 33(5):670–679. DOI:10.1002/rra.3124.
- Goren M, Galil BS. 2005. A review of changes in the fish assemblages of Levantine inland and marine ecosystems following the introduction of non-native fishes. Journal of Applied Ichthyology 21(4):364–370. DOI:10.1111/j.1439-0426.2005.00674.x.
- Gozlan RE, Britton JR, Cowx IG, Copp GH. 2010. Current knowledge on non-native freshwater fish introductions. Journal of Fish Biology 76(4):751–786. DOI:10.1111/j.1095-8649.2010.02566.x.
- Gozlan RE, Peeler EJ, Longshaw M, St-Hilaire S, Feist SW. 2006. Effect of microbial pathogens on the diversity of aquatic populations, notably in Europe. Microbes and Infection 8(5):1358–1364. DOI:10.1016/j.micinf.2005.12.010.
- Hatcher MJ, Dick JTA, Dunn AM. 2012. Disease emergence and invasions. Functional Ecology 26(6):1275–1287. DOI:10.1111/j.1365-2435.2012.02031.x.
- Hosmer Jr DW, Lemeshow S, Sturdivant RX. 2013. Applied logistic regression. Vol. 398. New York: John Wiley & Sons.
- Hulme PE, Bacher S, Kenis M, Klotz S, Kühn I, Minchin D, Nentwig W, Olenin S, Panov V, Pergl J, Pyšek P. 2008. Grasping at the routes of biological invasions: A framework for integrating pathways into policy. Journal of Applied Ecology 45(2):403–414. DOI:10.1111/j.1365-2664.2007.01442.x.
- Hulme PE, Pyšek P, Nentwig W, Vilà M. 2009. Will threat of biological invasions unite the European Union? Science 324(5923):40–41. DOI:10.1126/science.1171111.
- Interesova E, Vilizzi L, Copp GH. 2020. Risk screening of the potential invasiveness of non-native freshwater fishes in the River Ob basin (West Siberian Plain, Russia). Regional Environmental Change 20(2):64. DOI:10.1007/s10113-020-01644-3.
- IPCC. 2005. Guidance notes for lead authors of the IPCC fourth assessment report on addressing uncertainties. Intergovernmental Panel on Climate Change, WMO & UNEP. Available: https://wg1.ipcc.ch/publications/supportingmaterial/uncertainty-guidance-note.pdf
- Katsanevakis S, Coll M, Piroddi C, Steenbeek J, Ben Rais Lasram F, Zenetos A, Cardoso AC. 2014. Invading the Mediterranean Sea: Biodiversity patterns shaped by human activities. Frontiers in Marine Science 1:32. DOI:10.3389/fmars.2014.00032.
- Killi N, Tarkan AS, Kozic S, Copp GH, Davison PI, Vilizzi L. 2020. Risk screening of the potential invasiveness of non-native jellyfishes in the Mediterranean Sea. Marine Pollution Bulletin 150:110728. DOI:10.1016/j.marpolbul.2019.110728.
- Kumschick S, Richardson DM. 2013. Species-based risk assessments for biological invasions: Advances and challenges. Diversity & Distributions 19(9):1095–1105. DOI:10.1111/ddi.12110.
- Lawson Jr LL, Hill JE, Hardin S, Vilizzi L, Copp GH. 2015. Evaluation of the Fish Invasiveness Screening Kit (FISK v2) for peninsular Florida. Management of Biological Invasions 6(4):413–422. DOI:10.3391/mbi.2015.6.4.09.
- Lejeusne C, Chevaldonne P, Pergent-Martini C, Boudouresque CF, Perez T. 2010. Climate change effects on a miniature ocean: The highly diverse, highly impacted Mediterranean Sea. Trends in Ecology & Evolution 25(4):250–260. DOI:10.1016/j.tree.2009.10.009.
- Lelieveld J, Hadjinicolaou P, Kostopoulou E, Giannakopoulos C, Pozzer A, Tanarhte M, Tyrlis E. 2014. Model projected heat extremes and air pollution in the eastern Mediterranean and Middle East in the twenty-first century. Regional Environmental Change 14(5):1937–1949. DOI:10.1007/s10113-013-0444-4.
- Li S, Chen J, Wang X, Copp GH. 2017. Invasiveness screening of non-native fishes for the middle reach of the Yarlung Zangbo River, Tibetan Plateau, China. River Research and Applications 33(9):1439–1444. DOI:10.1002/rra.3196.
- Lundberg B, Golani D. 1995. Diet adaptations of Lessepsian migrant rabbitfishes, Siganus luridus and S. rivulatus, to the algal resources of the Mediterranean coast of Israel. Marine Ecology 16(1):73–89. DOI:10.1111/j.1439-0485.1995.tb00395.x.
- Lyons TJ, Tuckett QM, Durland Donahou A, Hill JE. 2020. Risk screen of lionfishes, Pterois, Dendrochirus, and Parapterois, for southeastern United States coastal waters of the Gulf Of Mexico and Atlantic Ocean. Biological Invasions 22(5):1573–1583. DOI:10.1007/s10530-020-02203-x.
- Mazza G, Aquiloni L, Inghilesi AF, Giuliani C, Lazzaro L, Ferretti G, Lastrucci L, Foggi B, Tricarico E. 2015. Aliens just a click away: The online aquarium trade in Italy. Management of Biological Invasions 6(3):253–261. DOI:10.3391/mbi.2015.6.3.04.
- McDowall R. 2006. Crying wolf, crying foul, or crying shame: Alien salmonids and a biodiversity crisis in the southern cool-temperate galaxioid fishes? Reviews in Fish Biology and Fisheries 16:233–422.
- McKinney ML, Lockwood JL. 1999. Biotic homogenization: A few winners replacing many losers in the next mass extinction. Trends in Ecology & Evolution 14(11):450–453. DOI:10.1016/S0169-5347(99)01679-1.
- Moghaddas SD, Abdoli A, Kiabi BH, Rahmani H, Vilizzi L, Copp GH. 2021. Identifying invasive fish species threats to RAMSAR wetland sites in the Caspian Sea region – A case study of the Anzali Wetland Complex (Iran). Fisheries Management and Ecology 28:28–39.
- Muhlfeld CC, Kovach RP, Jones LA, Al-Chokhachy R, Boyer MC, Leary RF, Lowe WH, Luikart G, Allendorf FW. 2014. Invasive hybridization in a threatened species is accelerated by climate change. Nature Climate Change 4(7):620–624. DOI:10.1038/nclimate2252.
- Nunes AL, Tricarico E, Panov V, Cardoso A, Katsanevakis S. 2015. Pathways and gateways of freshwater invasions in Europe. Aquatic Invasions 10(4):359–370. DOI:10.3391/ai.2015.10.4.01.
- Önol B, Unal YS. 2014. Assessment of climate change simulations over climate zones of Turkey. Regional Environmental Change 14(5):1921–1935. DOI:10.1007/s10113-012-0335-0.
- Paganelli D, Pandolfi A, Sconfietti R, Marchini A, Vilizzi L. 2018. Potential invasiveness by non-indigenous macrozoobenthos in the secondary hydrographic system of a temperate-climate river catchment. Ecological Indicators 88:274–281. DOI:10.1016/j.ecolind.2018.01.037.
- Panov VE, Alexandrov B, Arbačiauskas K, Binimelis R, Copp GH, Grabowski M, Lucy F, Leuven RSEW, Nehring S, Paunović M, Semenchenko V, Son MO. 2009. Assessing the risks of aquatic species invasions via European inland waterways: From concepts to environmental indicators. Integrated Environmental Assessment and Management 5(1):110–126. DOI:10.1897/IEAM_2008-034.1.
- Papavlasopoulou I, Perdikaris C, Vardakas L, Paschos I. 2014. Enemy at the gates: Introduction potential of non-indigenous freshwater crayfish in Greece via the aquarium trade. Central European Journal of Biology 9:11–18.
- Pheloung PC, Williams PA, Halloy SR. 1999. A weed risk assessment model for use as a biosecurity tool evaluating plant introductions. Journal of Environmental Management 57(4):239–251. DOI:10.1006/jema.1999.0297.
- Por FD. 2012. Lessepsian migration: The influx of Red Sea biota into the Mediterranean by way of the Suez Canal. Ecological Studies: Analysis and Synthesis, 23. Berlin: Springer Verlag.
- R Development Core Team. 2020. R: A language and environment for statistical computing. Vienna, Austria: R Foundation for Statistical Computing. Available: www.R-project.org/.
- Radočaj T, Špelić I, Vilizzi L, Povž M. 2021. Identifying threats from introduced and translocated non-native freshwater fishes in Croatia and Slovenia under current and future climatic conditions. Global Ecology and Conservation 27(article):e01520. DOI:10.1016/j.gecco.2021.e01520.
- Rilov G, Peleg O, Yeruham E, Garval T, Vichik A, Raveh O. 2018. Alien turf: Overfishing, overgrazing and invader domination in south-eastern Levant reef ecosystems. Aquatic Conservation: Marine and Freshwater Ecosystems 28(2):351–369. DOI:10.1002/aqc.2862.
- Robin X, Turck N, Hainard A, Tiberti N, Lisacek F, Sanchez J-C, Müller M. 2011. pROC: An open-source package for R and S+ to analyze and compare ROC curves. BMC Bioinformatics 12(1):77. DOI:10.1186/1471-2105-12-77.
- Ruykys L, Ta KAT, Bui TD, Vilizzi L, Copp GH. 2021. Risk screening of the potential invasiveness of non-native aquatic species in Vietnam. Biological Invasions 23(7):2047–2060. DOI:10.1007/s10530-020-02430-2.
- Semenchenko V, Lipinskaya T, Vilizzi L. 2018. Risk screening of non-native macroinvertebrates in the major rivers and associated basins of Belarus using the Aquatic Species Invasiveness Screening Kit. Management of Biological Invasions 9(2):127–136. DOI:10.3391/mbi.2018.9.2.06.
- Smith KG, Barrios V, Darwall WRT, Numa C, Eds. 2014. The status of freshwater biodiversity in the Eastern Mediterranean. Switzerland and Cambridge, UK: IUCN, Gland.
- Stasolla G, Tricarico E, Vilizzi L. 2021. Risk screening of the potential invasiveness of non-native marine crustacean decapods and barnacles in the Mediterranean Sea. Hydrobiologia 848(9):1997–2009. DOI:10.1007/s10750-020-04432-6.
- Tarkan AS, Sarı HM, İlhan A, Kurtul I, Vilizzi L. 2017a. Risk screening of non-native and translocated freshwater fish species in a Mediterranean-type shallow lake: Lake Marmara (West Anatolia). Zoology in the Middle East 63(1):48–57. DOI:10.1080/09397140.2017.1269398.
- Tarkan AS, Vilizzi L, Top N, Ekmekçi FG, Stebbing PD, Copp GH. 2017b. Identification of potentially invasive freshwater fishes, including translocated species, in Turkey using the Aquatic Species Invasiveness Screening Kit (AS-ISK). International Review of Hydrobiology 102(1–2):47–56. DOI:10.1002/iroh.201601877.
- Tedesco PA, Leprieur F, Hugueny B, Brosse S, Dürr H, Beauchard O, Busson F, Oberdorff T. 2012. Patterns and processes of global riverine fish endemism. Global Ecology and Biogeography 21(10):977–987. DOI:10.1111/j.1466-8238.2011.00749.x.
- Theocharis A. 2008. Do we expect significant changes in the thermohaline circulation in the Mediterranean in relation to observed surface layers warming? In: Briand F,editor. Climate warming and related changes in Mediterranean marine biota. (CIESM Workshop Monographs No. 35, Monaco). pp. 25–29.
- Theocharis A, Lascaratos A. 2000. Possible causes, origin, evolution and some consequences of the Eastern Mediterranean Transient during the period 1987–1999. In: Lascaratos A, Briand F, editors. The Eastern Mediterranean Climatic Transient, its origin, evolution and impact on the ecosystem. (CIESM Workshop Series, Monaco, Vol. 10). pp. 1–86.
- Tricarico E. 2012. A review on pathways and drivers of use regarding non-native freshwater fish introductions in the Mediterranean region. Fisheries Management and Ecology 19(2):133–141. DOI:10.1111/j.1365-2400.2011.00834.x.
- Tricarico E, Junqueira A, Dudgeon D. 2016. Alien species in aquatic environments: A selective comparison of coastal and inland waters in tropical and temperate latitudes. Aquatic Conservation: Marine and Freshwater Ecosystems 26(5):872–891. DOI:10.1002/aqc.2711.
- Tricarico E, Vilizzi L, Gherardi F, Copp GH. 2010. Calibration of FI-ISK, an invasiveness screening tool for nonnative freshwater invertebrates. Risk Analysis 30(2):285–292. DOI:10.1111/j.1539-6924.2009.01255.x.
- Uyan U, Oh C-W, Tarkan AS, Top N, Copp GH, Vilizzi L. 2020. Risk screening of the potential invasiveness of non-native marine fishes for South Korean coastal waters. Marine Pollution Bulletin 153(article):111018. DOI:10.1016/j.marpolbul.2020.111018.
- Vergés A, Tomas F, Cebrian E, Ballesteros E, Kizilkaya Z, Dendrinos P, Karamanlidis AA, Spiegel D, Sala E. 2014. Tropical rabbitfish and the deforestation of a warming temperate sea. Journal of Ecology 102(6):1518–1527. DOI:10.1111/1365-2745.12324.
- Vilà M, Basnou C, Pyšek P, Josefsson M, Genovesi P, Gollasch S, Nentwig W, Olenin S, Roques A, Roy D, Hulme PE. 2010. How well do we understand the impacts of alien species on ecosystem services? A pan-European, cross-taxa assessment. Frontiers in Ecology and the Environment 8(3):135–144. DOI:10.1890/080083.
- Villéger S, Blanchet S, Beauchard O, Oberdorff T, Brosse S. 2011. Homogenization patterns of the world’s freshwater fish faunas. Proceedings of the National Academy of Science 108(44):18003–18008. DOI:10.1073/pnas.1107614108.
- Wei H, Chaichana R, Vilizzi L, Daengchana P, Liu F, Nimtim M, Zhu Y, Li S, Hu Y, Copp GH. 2021. Do non-native ornamental fishes pose a similar level of invasion risk in neighbouring regions of similar current and future climate? Implications for conservation and management. Aquatic Conservation: Marine and Freshwater Ecosystems. DOI: 10.1002/aqc.3609.
- Yeruham E, Shpigel M, Abelson A, Rilov G. 2020. Ocean warming and tropical invaders Erode the performance of a key herbivore. Ecology 101(2):e02925. DOI:10.1002/ecy.2925.
- Yonekura R, Kohmatsu Y, Yuma M. 2007. Difference in the predation impact enhanced by morphological divergence between introduced fish populations. Biological Journal of the Linnean Society 91(4):601–610. DOI:10.1111/j.1095-8312.2007.00821.x.
- Zittis G, Hadjinicolaou P, Fnais M, Lelieveld J. 2016. Projected changes in heat wave characteristics in the eastern Mediterranean and the Middle East. Regional Environmental Change 16(7):1863–1876. DOI:10.1007/s10113-014-0753-2.