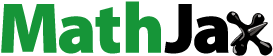
Abstract
Bats are particularly susceptible to environmental changes because of their low reproductive rate, longevity, and high metabolic rates, which lead to relatively high food requirements. Thus, bat populations take a relatively long time to recover from increased mortality rates, and monitoring schemes should cover long time periods. In this work, we analyzed the population trajectories of two bat species, Rhinolophus hipposideros and Myotis myotis, the most numerous in five caves in southern Poland, which are known as important bat hibernacula on a continental scale. Data were collected by regular counts in 1985–2020, depending on the particular cave; in addition, previous data on the number of hibernating bats in these caves, available since 1951, were taken from existing publications. We analyzed time-series data using average locality indices and TRIM (TRends and Indices for Monitoring data) methods, and both produced similar results. Generally, the populations of the two studied bat species showed recent increasing trends, especially visible as an effect of recovery after years of decline. The situation recorded in southern Poland is very similar to that described in other places in Europe, where recoveries of bat populations have also been observed in the last decades. Although it is difficult to present results from formal analyses, because of the lack of good data, at least some factors—less exposure to contaminants (pesticides, heavy metals), improving food availability due to climate change, and a lower predation rate (including human pressure), both in the breeding season and during wintering—positively affected both species.
1. Introduction
Bats are particularly susceptible to environmental changes because of their low reproductive rate, longevity, and high metabolic rates, which lead to relatively high food requirements (Voigt & Kingston Citation2016). Thus, bat populations take a relatively long time to recover from increased mortality rates (Racey & Entwistle Citation2000; Fleischer et al. Citation2017). Moreover, bat populations show responses to environmental stressors, ranging from alterations in habitat quality to climate change, as well as direct exploitation (Russo & Ancillotto Citation2015; Jung & Threlfall Citation2016; Gottfried et al. Citation2020), and bats are thus recognized as excellent indicators of anthropogenic changes in the environment (Jones et al. Citation2009; Russo & Jones Citation2015; Zukal et al. Citation2015).
Important for methodological, statistical and conservation purposes are the existing long-term series, coming mainly from winter bat censuses, especially in caves (Furey & Racey Citation2016; Zukal et al. Citation2017). For example, bats have been counted since 1944 in the Schenkgroeve, an artificial limestone cave in south Limburg in the Netherlands (Grol & Voute Citation2010), since 1946 in Hermann’s cave in Lower Austria (Spitzenberger & Engelberger Citation2013), and since 1957 in some caves in the Moravian Karst, Czech Republic (Gaisler et al. Citation2006). Thanks to the results obtained, it was discovered that the European bat populations, particularly the lesser horseshoe bat Rhinolophus hipposideros and the greater mouse-eared bat Myotis myotis, declined dramatically in the second half of the 20th century (Stebbings Citation1989; Řehák & Gaisler Citation1999; Bontadina et al. Citation2000; Uhrin et al. Citation2010). After the period of decline, since the 1990s bat populations have begun to recover (Van der Meij et al. Citation2015). An increase in the number of some species of hibernating bats has been reported from many European countries: Austria (Spitzenberger & Engelberger Citation2013), Belgium (Kervyn et al. Citation2009), the Czech Republic (Bufka & Červený Citation2012; Chytil & Gaisler Citation2012), the UK (Barlow et al. Citation2015), Italy (Toffoli & Calvini Citation2019), Ireland (McAney Citation2014), the Netherlands (Grol & Voute Citation2010), Poland (Fuszara et al. Citation2010), Slovakia (Uhrin et al. Citation2010), Spain (Machado et al. Citation2017), Sweden (Rydell et al. Citation2019) and Switzerland (Bontadina et al. Citation2000).
The reasons for these changes in population trends have not been conclusively identified (Bontadina et al. Citation2000; Tournant et al. Citation2013; Afonso et al. Citation2016; Froidevaux et al. Citation2017). It is believed that the bat population declines and subsequent increases may be caused by a combination of various factors, such as the spread of chemical pollutants, habitat destruction, changes in landscape structure, disturbance and destruction of roost sites, climate change, declines in insect prey, competition for prey, genetic inbreeding, and diseases (Arlettaz et al. Citation2000; Bontadina et al. Citation2000; Zahn et al. Citation2007; Van der Meij et al. Citation2015; Auteri & Knowles Citation2020).
Both species selected for this study, Rhinolophus hipposideros and Myotis myotis have similar preferences for shelters. In winter both species hibernate in caves, mines and other cave-like structures. They prefer places with high humidity (over 80%) and stable temperatures of 6–9°C. In summer the females form maternity colonies in caves (Southern Europe) or in buildings with spacious roofs such as church attics and castles (Central Europe), where they give birth and nurse their offspring (Dietz et al. Citation2009; Berková et al. Citation2014; Jan et al. Citation2017). Both species are insectivorous, but they differ slightly in their manner of foraging and their diet. R. hipposideros forages exclusively in woodlands, preferentially in dense areas, capturing its prey using echolocation in flight. It preys mainly on moths and Diptera. M. myotis preys on large, ground-dwelling arthropods such as beetles, crickets, and spiders, gleaning them from the ground (Motte & Libois Citation2002; Rudolph et al. Citation2009). The two species are the most numerous hibernating species in the caves of southern Poland (Grzywiński et al. Citation2015). These bat species are excellent for monitoring population trends, as they are easy to recognize and are relatively easy to count, because they do not hide in crevices (Dietz et al. Citation2009; Rudolph et al. Citation2009).
Both bat species occupy different ecological niches, especially in terms of foraging strategies, and then we test a hypothesis on differences between study species in relation to long-term environmental changes. Saying more precisely, the aim of the study was to determine long-term population trends of R. hipposideros and M. myotis and the probable causes of changes in the numbers of hibernating bats of these two species.
2. Material and methods
2.1. Study area
The five studied caves () are located in the Kraków-Częstochowa Upland (also known as the Polish Jura) in the southern part of Poland (). The upland has elevations between 300 and 513 m a.s.l. The area is formed by upper Jurassic limestone, which creates a plate with single inselbergs several meters in height. This region is characterized by karst processes with numerous deep gorges, sinkholes, and caves (Kondracki Citation2001). Over 1800 caves and rock shelters of total length over 31 km are known in this area. Most of them are small: only 150 caves exceed a length of 40 m (Gradziński & Szelerewicz Citation2004). 20% of the region is covered by forests, dominated by deciduous and mixed types.
Figure 1. Location of the studied caves: 1 – Racławicka, 2 – Nietoperzowa, 3 – Łokietka, 4 – Ciemna, and 5 – Wierzchowska Górna (Source of spatial data: OpenStreetMap.org)
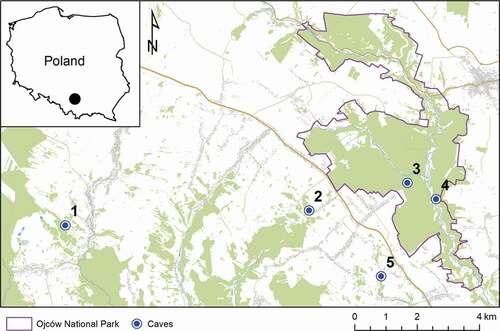
Table I. Characteristics of the studied caves
2.2. Data collection
Standardized and regular annual censuses during the hibernation period (in the first half of February) have been conducted in the caves since 1985 (Nietoperzowa and Ciemna), 1991 (Łokietka), 2000 (Racławicka) and 2001 (Wierzchowska Górna). All bats roosting in the caves are counted. The counting protocol includes visual species determination and counts of visible hibernating bats with the aid of torches and binoculars. Previous data on the number of hibernating bats in these caves, available from 1951, were taken from existing publications (Kowalski Citation1953; Wołoszyn Citation1976; Harmata Citation1981; Godawa & Wołoszyn Citation1990; Nowak & Kozakiewicz Citation2000; Grzywiński et al. Citation2015).
Climate data for the years 1951–2019—average annual temperature (°C), total precipitation (mm), number of days with rainfall—were obtained from the nearest meteorological station in Kraków (20 km to the south).
2.3. Data analysis
2.3.1. Average locality index (ALI)
Following Loman and Andersson (Citation2007) and Kyek et al. (Citation2017) we calculated the average locality index (ALI), which allowed us to compare the average population changes of the two bat species over the years, even though the numbers of both species are very different. In the first step, we calculated a locality index for each cave (LIys) using the formula
where:
- the number of individuals counted for a particular species, cave, and year
- the average number counted for a particular species at a given cave during the whole study period
Values below 1 indicated a relatively low count of individuals, while numbers above 1 indicated a relatively high count. Then we calculated the average locality index (ALI) in all caves for each year, using the formula
which represents the overall population trend for both species. Finally, we adjusted the linear and nonlinear trend of population change over time and tested the significance of Beta coefficients using a t-test.
In the case of monotonic functions, such as a quadratic function,
for each species we calculated an extreme point of the function according to the equation
which shows up to which year the population decreased in number, and analogously, from which year the number of individuals increased.
2.3.2. TRIM
The ALI method does not provide test statistics significant for population change, nor does it provide standard errors and 95% confidence limits. Thus, as a second approach to the analysis of the population trends we used TRIM (TRends & Indices for Monitoring data method) (Pannekoek & van Strien Citation2005) implemented in the rtrim library for R. This procedure makes better use of the available data, especially when some data for the years are absent—a common issue in long-term time series (in our case in the years 1950–1980)—calculating standard errors and confidence limits and offering various test statistics; it also takes into account overdispersion and serial correlation of data (Pannekoek & van Strien Citation2005). TRIM is also capable of categorizing data by covariates and testing their influence on the observed changes, using Wald tests.
TRIM fits log-linear models and indices that represent the effect of change between years, which indicates the relative variation of the total population size. Two types of indices are estimated: (i) model-based indices, which are the values predicted by the model; and (ii) imputed indices, which equal the observed count if an observation is made, and the model prediction for missing counts (Pannekoek & van Strien Citation2005). Dissimilarity between the two indices reflects a mismatch between observed (i.e. imputed indices) and model predictions (i.e. model-based indices) and, therefore, a lack of fit of the statistical model applied. In the next step, indices are used to estimate a mean annual change rate (Pannekoek & van Strien Citation2005). This technique has been widely employed for the analysis of temporal series in bird populations (Paradis et al. Citation2002; Gregory et al. Citation2007; Lehikoinen et al. Citation2019) and also bat populations (Uhrin et al. Citation2010; Van der Meij et al. Citation2015; Froidevaux et al. Citation2017; Machado et al. Citation2017; Toffoli & Calvini Citation2019). We developed models with and without covariates (five caves). The best-fit models were selected according to goodness-of-fit tests (the Likelihood Ratio (LR) and chi-squared tests) and the Akaike information criterion (AIC). A significance value for a model greater than 0.05 indicates that the data fit a Poisson distribution and, therefore, that the model can be accepted. Indices, overall slope and Wald tests remain reliable in case of lack of fit (Pannekoek & van Strien Citation2005). In case of overdispersion or serial correlation (default TRIM thresholds: >3.0 and >0.4, respectively) the Wald test for the significance of slope was employed (Pannekoek & van Strien Citation2005).
All calculations were performed in the language R 4.0.2 using the stats, rtrim, psych and ggcorrplot libraries (R Core Team Citation2018).
3. Results
3.1. Population size
The detected number of individual bats between 1951 and 2020 was very variable. The total number of Rhinolophus hipposideros in the five studied caves was over 300 individuals in the early 1950s. In the 1980s and early 1990s, it did not exceed 40 individuals, and in the last decade (2011–2020), it averaged 665 individuals (max 1050 ind. in 2020). The highest number of R. hipposideros was observed in Ciemna cave (max 531 ind. in 2020, min 5 ind. in 1986). Myotis myotis was a less abundant bat species. A total of over 50 individuals were observed in the five studied caves in the 1950s. In the 1980s and early 1990s, fewer than 40 individuals were usually recorded. In the last decade of observations, there were on average 88 individuals (max 112 ind. in 2016). M. myotis was most abundant in Nietoperzowa cave (max 55 ind. in 2019, min 6 ind. in 1982).
3.2. Analysis based on average locality indices
For both species, the β-coefficients for linear and quadratic functions were significant (). However, for both species, a lack-of-fit test showed that a quadratic function was better than a linear one (). Calculation of the extreme point of the function for R. hipposideros showed that the population decreased up to the year 1979, after which it increased (). In the case of M. myotis, the extreme point occurred in 1980 (). We did not find any significant differences between the two values (chi-square = 0.98, p = 0.86).
Figure 2. Average locality index (ALI) with quadratic regression for Rhinolophus hipposideros (a) and Myotis myotis (b)
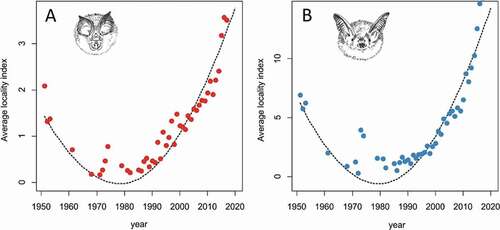
Table II. Comparing the directional factor (β) for a population trend between a linear and a quadratic function
3.3. TRIM
All of the tests showed the same slope for both species. R. hipposideros and M. myotis are stable and show a moderate increase. Models using the five caves as covariates have higher AIC, smaller Wald statistics and higher standard deviation than models without them (). The goodness-of-fit tests for both species are significant. The overall slope of the linear trend model for R. hipposideros and M. myotis represents a moderate increase. The indices show a sharp drop in the years 1950–1973; thereafter, the indices are stable again until 2001. The early increase in 2002 was mainly driven by the population dynamics in the Ciemna and Wierzchowska caves. The imputed overall index for 2010 is equal to the index from 1951.
Table III. Test statistics for the different TRIM models for Rhinolophus hipposideros and Myotis myotis.
The overall slope of the linear trend model for M. myotis shows an increase (p < 0.01). The indices show a negative trend between 1951 and 1953, followed by a moderate increase to 1981. The first peak of the increase in 1991 is mainly driven by the population dynamics in the Nietoperzowa cave.
Despite the differences between the species, their numbers (expressed as a TRIM index of year-to-year changes) were moderately correlated (). We also found a positive relationship between the average annual temperature and the numerical change in the TRIM index for both species, while no such significant relationship was found for precipitation or the number of days with rainfall in a particular year ().
Figure 3. Changes in relative abundance according to TRIM models for Rhinolophus hipposideros and Myotis myotis. A blue or red circle indicates the means, bars indicate standard error, while the dashed lines - 95% confidential limits. Bottom part: DDT production (GEF Citation2004) and important heavy metal (ZN, Pb, Cu and Ni) emission in Poland – data available since 1980 (GUS Citation2020)
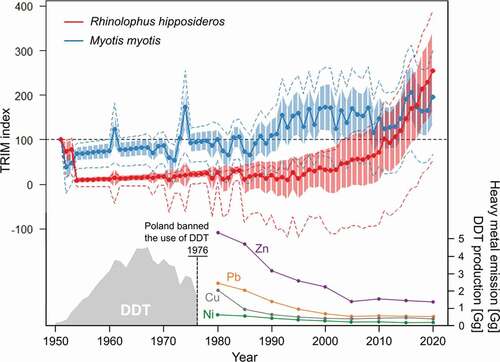
Figure 4. Correlation matrix between the TRIM index of year-to-year changes in the number of bats for Rhinolophus hipposideros (trim RHH) and Myotis myotis (trim MYM) and weather conditions: average annual temperature (Temp), total precipitation (Prec) and number of days with rainfall (DwP) in study years
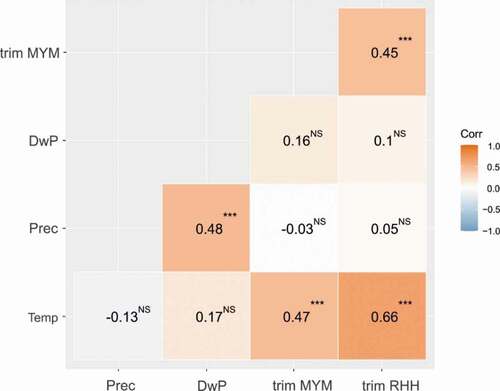
4. Discussion
4.1. Population trends
Both analytical methods produce similar results: a recent increase in population size, and an especially visible effect of recovery after years of decline. The situation is very similar to that described in other places in Europe, where recoveries of many bat populations in the last decades have been reported (Van der Meij et al. Citation2015). Rhinolophus hipposideros is the bat species that has faced the most dramatic decline in western and central Europe in the 1950s–1980s, becoming locally extinct (Stebbings Citation1989; Kokurewicz Citation1990; Bontadina et al. Citation2000; Afonso et al. Citation2016), a subsequent reversing trend and population rebound have been observed since the 1990s (Uhrin et al. Citation2010; Bufka & Červený Citation2012; Chytil & Gaisler Citation2012; Spitzenberger & Engelberger Citation2013). At the same time, similar but not as spectacular trends were observed in the European population of Myotis myotis (Zahn et al. Citation2006; Kervyn et al. Citation2009; Uhrin et al. Citation2010; Van der Meij et al. Citation2015).
The caves we studied are among those where bat observations have been conducted for the longest time in the world. Our findings confirm the importance of long-term bat monitoring, especially winter censuses that have been ongoing for many decades at some sites (Spitzenberger & Engelberger Citation2013; Furey & Racey Citation2016; Zukal et al. Citation2017). The results of short-term observations can be disturbed by annual fluctuations in numbers. In addition, bat populations take many years to recover after disturbances (Racey & Entwistle Citation2000; Fleischer et al. Citation2017), so only long-term observations can identify such changes (Jones et al. Citation2009; Russo & Jones Citation2015).
For effective bat conservation, it is important not only to perform long-term bat monitoring, but also to identify drivers of their population decline (Bontadina et al. Citation2000; Zahn et al. Citation2007; Afonso et al. Citation2016). Because the bat species we studied have a similar pattern of population trends at many sites in Europe, it is likely that the same (or very similar) factors influenced them. Therefore, results obtained locally may be relevant at the continental scale. Below we discuss a set of potential factors that may have affected local bat populations, also paying attention to potential limitations in the explanation of population trajectories.
4.2. Factors affecting long-term population trends
4.2.1. Exposure to contaminants (Pesticides, heavy metals)
Exposure to organochlorine insecticides, especially DDT (dichlorodiphenyltrichloroethane), has been identified as a possible cause of declining bat populations (Luckens & Davis Citation1964; Jefferies Citation1972; Clark et al. Citation1978). DDT was used ubiquitously for pest control in agriculture and forestry in Poland in the years 1946–1976, but since then the amount of DDT in the environment has been systematically decreasing (Falandysz et al. Citation2001; Bayat et al. Citation2014). However, due to their relatively long lifetime and their high daily food intake, insectivorous bats may be exposed to higher concentrations of cumulative chemicals such as heavy metals, which accumulate through the food chain (Zukal et al. Citation2015; Hernout et al. Citation2016).
The caves studied are located between the Kraków agglomeration and the Upper Silesia industrial region, where there are hundreds of industrial facilities (metallurgical works, chemical and cement plants, power stations). In the last decades of the 20th century, this region was the most polluted in Poland and one of the most polluted in Europe (Dmuchowski & Bytnerowicz Citation1995). As a result of the political and economic transformations in Poland at the end of the 1980s, industrial production, including that of heavy metals, declined considerably.
4.2.2. Changes in the structure of habitats
The area where the caves are located has not changed significantly since World War II. For both studied species, the availability of woodlands (foraging areas) is crucial (Motte & Libois Citation2002; Rudolph et al. Citation2009). However, the absence of significant changes in land use, particularly reduction in forest cover, indicates that changes in the physical (vertical) structure of habitats could not have been the main reason for the long-term changes in bat populations.
4.2.3. Decline of roosts
Both studied bat species (R. hipposideros and M. myotis) have similar preferences for winter and summer roosts (Dietz et al. Citation2009). In winter, bats hibernate mostly in caves and other underground places. In the Polish Jura, the number of caves available for bats has not changed noticeably in the 20th and 21st centuries (Gradziński & Szelerewicz Citation2004). In the caves we monitored, the conditions of hibernation have not changed since the early 1950s (Kowalski Citation1953). The summer roosts in this area are not well recognized and have not been monitored. However, there is no information about a significant number of building renovations that might have caused the loss of summer bat colonies.
4.2.4. Climate changes
We found a significant positive correlation between the population trend of both species (R. hipposideros and M. myotis) and the average annual temperature in 1951–2020, but we did not find such a correlation with precipitation or with the number of days with rainfall in particular years (). Numerous earlier studies have demonstrated the impact of climatic conditions on the activity, survival, and reproductive success of bats (Sherwin et al. Citation2013). Climate influences food availability (Arlettaz et al. Citation2001; Ciechanowski et al. Citation2007), timing of hibernation (Hope & Jones Citation2012; Jones & Rebelo Citation2013), frequency and duration of torpor (Stawski et al. Citation2014), rate of energy expenditure (Zahn et al. Citation2007; Jan et al. Citation2017), reproduction and the development rates of juveniles (Adams & Hayes Citation2008; Burles et al. Citation2009; Lučan et al. Citation2013). Global warming may influence the species richness and distribution of bats (Rebelo et al. Citation2010; Wu Citation2016). However, there are few studies showing the impact of temperature on bat population trends. Froidevaux et al. (Citation2017) found that the annual growth rate of maternity colonies of the greater horseshoe bat (Rhinolophus ferrumequinum) in the United Kingdom was strongly correlated with spring temperatures and precipitation. Jones and Rebelo (Citation2013) believed it highly likely that warmer conditions have contributed to considerable increases in abundance since 1997 for two species of horseshoe bats (R. ferrumequinum and R. hipposideros). Zahn et al. (Citation2007) compared the impact of severe weather in Portugal and Germany on the body condition of M. myotis. They concluded that foraging constraints due to severe weather may contribute to poor body conditions, even when food resources are abundant. Thus, bouts of bad weather may cause high mortality in bats. On the other hand, Bowler et al. (Citation2015) emphasized that none of the temperature variables showed a significant relationship with long-term bat population trends. Mehr et al. (Citation2011) found that land use had a much greater effect than climate on bat species richness and community composition on a regional scale.
Our finding that long-term trends in bat populations were correlated with average annual temperature does not necessarily mean that temperature was the only factor affecting bat population changes. The correlation may be accidental, resulting from comparing two growing trends at the same time. Both studied bat species are thermophilic, which may be a reason for the effect of increasing temperature (Dietz et al. Citation2009), and can benefit from warming. However, temperature does not explain the decreasing trends between 1950 and 1980. Bontadina et al. (Citation2000) also suggested that the fact that R. hipposideros was an abundant species early this century, when the climate was not significantly warmer than today, contradicts the scenario of a large thermic dependence as a single influencing factor.
4.2.5. Food availability
We have no information on food availability for bats on a local scale. However, Przybyłowicz and Buszko (Citation2008) observed that in the last few dozen years, the species richness of Lepidoptera in the Ojców National Park has decreased. On the other hand, monitoring of forest tree pests (insects) suggested that the most important insect pests have a tendency to outbreak in forests (Perlińska & Hamera-Dzierżanowska Citation2016). In the vicinity of the study area, the only tree pest whose numbers increased was the pine sawfly (Acantholyda posticalis), and in the years 1971–2018 the fluctuations in its numbers were very small (Sławski & Sławska Citation2019). There is no information supporting the hypothesis that a shortage of insects could be the main cause of bat population changes. In Switzerland, Bontadina et al. (Citation2008) found that changes in prey abundance are unlikely to explain the demography of R. hipposideros. However, the same factors that affected bat numbers may also have affected the number of insects.
4.2.6. Diseases
Bats have been considered to have a particularly effective immune system, but numerous bacteria and viruses apparently remain non-pathogenic in bats, likely due to a long process of co-evolution (Afelt et al. Citation2018). Although bacterial, viral and parasitic infections may be among the main causes of bat deaths, no mass mortality from epidemics has been observed in Europe (Mühldorfer et al. Citation2011). There is very little information about bat diseases in Poland. No mass mortality of bats or visible disease symptoms were observed in the caves of the Polish Jura during the winter censuses. We believe that diseases were not the main cause of long-term changes in bat numbers, but bats affected by other factors such as pollution may have been more susceptible to infection.
4.2.7. Predation, including human disturbance
Temperate zone bats face a very low risk of predation. In particular, there are no predators specialized on bats in Europe (Lima & O’Keefe Citation2013). Owls are the only nocturnal predators that can prey on bats in flight, but this is a rare and opportunistic phenomenon, and only two species of European owls, the barn owl Tyto alba and the tawny owl Strix aluco, feed on bats more frequently (Sieradzki & Mikkola Citation2020). Occasionally, bats in roosts may be killed by domestic cats (Ancillotto et al. Citation2013) and martens (De Marinis & Masseti Citation1995). Predation is therefore a marginal factor with little impact on bat mortality.
On the other hand, the Ojców National Park is exposed to relatively high tourist pressure, due to its small area (2146 ha), attractiveness, and location close to the city of Kraków. This pressure can be estimated on the basis of the number of visitors to the Łokietka Cave, one of the Park’s greatest attractions. Sales of tickets to this cave have been recorded since 1960. There is no visible trend in the number of visitors. The current number of visitors (120 thousand in 2019) is close to the average for the whole period and to that of the early 1960s. The cave is only visited outside the bat hibernation season. Despite the high human pressure, no deliberate killing or disturbance of bats has been recorded in the caves of the Polish Jura.
4.3. Assessment of factors
Both studied species showed positive trends in population size over the long time period (1951–2020). Because the study has a correlational character, and because there was no access to detailed spatial and temporal environmental (and other) data, we discuss the main potential factors affecting both bat species according to the proposal of Bontadina et al. (Citation2000), and we rank their influence ().
Table IV. Assessment of factors that may have caused changes in long-term population trends of Rhinolophus hipposideros and Myotis myotis. List of factors after Bontadina et al. (Citation2000)
5. Conclusions
Both studied species, Rhinolophus hipposideros and Myotis myotis, have shown a significant increase in wintering population size over the last 70 years. We noted two directions of change: until the 1980s the population of both species was decreasing, and after that time it was increasing. Similar trends have been observed throughout Europe (Van der Meij et al. Citation2015).
Although the search for factors affecting population size has only a correlative character, we must note that reduced exposure to contamination was probably the most important factor in the long-term changes in the populations of both of these bat species. However, other factors, including climate change, food shortage and diseases, may also play some role in changes in bat populations.
Acknowledgements
We would like to thank the participants of the winter bat censuses, in particular: Przemysław Adamus, Jolanta Cerek, Małgorzata Hoppe, Barbara Karwowska, Anna Kmiecik, Paweł Kmiecik, Katarzyna Kozakiewicz, Katarzyna Malak, Wojciech Olma, Krzysztof Polowy, Rafał Sadowy, Marta Wieczorek and Mikołaj Zbonik. We would also like to thank numerous participants of winter censuses from: Student Foresters’ Club of the Poznań University of Life Sciences, Student Naturalist Society at the Jagiellonian University and Cracow Caving Club.
Disclosure statement
No potential conflict of interest was reported by the author(s).
Data availability statement
The data that support the findings of this study are available from the corresponding author upon reasonable request.
Additional information
Funding
References
- Adams RA, Hayes MA. 2008. Water availability and successful lactation by bats as related to climate change in arid regions of western North America. Journal of Animal Ecology 77:1115–1121. DOI: 10.1111/j.1365-2656.2008.01447.x.
- Afelt A, Devaux C, Serra-Cobo J, and Frutos R. 2018. Bats, bat-borne viruses, and environmental changes. In: Mikkola H, editor. Bats. London: IntechOpen. DOI: 10.5772/intechopen.74377.
- Afonso E, Tournant P, Foltête J-C, Giraudoux P, Baurand P-E, Roué S, Canella V, Vey D, Scheifler R. 2016. Is the lesser horseshoe bat (Rhinolophus hipposideros) exposed to causes that may have contributed to its decline? A non-invasive approach. Global Ecology and Conservation 8:123–137. DOI: 10.1016/j.gecco.2016.09.002.
- Ancillotto L, Serangeli MT, Russo D. 2013. Curiosity killed the bat: Domestic cats as bat predators. Mammalian Biology 78:369–373. DOI: 10.1016/j.mambio.2013.01.003.
- Arlettaz R, Christe P, Lugon A, Perrin N, Vogel P. 2001. Food availability dictates the timing of parturition in insectivorous mouse-eared bats. Oikos 95:105–111. DOI: 10.1034/j.1600-0706.2001.950112.x.
- Arlettaz R, Godat S, Meyer H. 2000. Competition for food by expanding pipistrelle bat populations (Pipistrellus pipistrellus) might contribute to the decline of lesser horseshoe bats (Rhinolophus hipposideros). Biological Conservation 93:55–60. DOI: 10.1016/S0006-3207(99)00112-3.
- Auteri GG, Knowles LL. 2020. Decimated little brown bats show potential for adaptive change. Scientific Reports 10:3023. DOI: 10.1038/s41598-020-59797-4.
- Barlow KE, Briggs PA, Haysom KA, Hutson AM, Lechiara NL, Racey PA, Walsh AL, Langton SD. 2015. Citizen science reveals trends in bat populations: The national bat monitoring programme in Great Britain. Biological Conservation 182:14–26. DOI: 10.1016/j.biocon.2014.11.022.
- Bayat S, Geiser F, Kristiansen P, Wilson SC. 2014. Organic contaminants in bats: Trends and new issues. Environment International 63:40–52. DOI: 10.1016/j.envint.2013.10.009.
- Berková H, Pokorný M, Zukal J. 2014. Selection of buildings as maternity roosts by greater mouse-eared bats (Myotis myotis). Journal of Mammalogy 95:1011–1017. DOI: 10.1644/13-MAMM-A-102.
- Bontadina F, Arlettaz R, Fankhauser T, Lutz M, Mühlethaler E, Theiler A, Zingg P. 2000. The lesser horseshoe bat Rhinolophus hipposideros in Switzerland: Present status and research recommendations. Le Rhinolophe 14:69–83.
- Bontadina F, Schmied SF, Beck A, Arlettaz R. 2008. Changes in prey abundance unlikely to explain the demography of a critically endangered Central European bat. Journal of Applied Ecology 45:641–648. DOI: 10.1111/j.1365-2664.2007.01417.x.
- Bowler DE, Haase P, Kröncke I, Tackenberg O, Bauer HG, Brendel C, Brooker RW, Gerisch M, Henle K, Hickler T, Hof C, Klotz S, Kühn I, Matesanz S, O‘Hara R, Russell D, Schweiger O, Valladares F, Welk E, Wiemers M, Böhning-Gaese K. 2015. A cross-taxon analysis of the impact of climate change on abundance trends in central Europe. Biological Conservation 187:41–50. DOI: 10.1016/j.biocon.2015.03.034.
- Bufka L, Červený J. 2012. Population increase of Rhinolophus hipposideros in the Šumava Mts. region, SW Bohemia. Vespertilio 16:115–130.
- Burles DW, Brigham RM, Ring RA, Reimchen TE. 2009. Influence of weather on two insectivorous bats in a temperate Pacific Northwest rainforest. Canadian Journal of Zoology 87:132–138. DOI: 10.1139/Z08-146.
- Chytil J, Gaisler J. 2012. Development of the Rhinolophus hipposideros population in southern Moravia, Czech Republic. Vespertilio 16:131–137.
- Ciechanowski M, Zając T, Biłas A, Dunajski R. 2007. Spatiotemporal variation in activity of bat species differing in hunting tactics: Effects of weather, moonlight, food abundance, and structural clutter. Canadian Journal of Zoology 85:1249–1263. DOI: 10.1139/Z07-090.
- Clark Jr. DR, Kunz TH, Kaiser TE. 1978. Insecticides applied to a nursery colony of little brown bats (Myotis lucifugus): Lethal concentrations in brain tissues. Journal of Mammalogy 59:84–91. DOI: 10.2307/1379877.
- De Marinis AM, and Masseti M. 1995. Feeding habits of the pine marten Martes martes L., 1758, in Europe: A review. Hystrix 7:143–150. DOI: 10.4404/hystrix-7.1-2-4063.
- Dietz C, Von Helversen O, and Nill D. 2009. Bats of Britain, Europe and northwest Africa. London: A & C Black.
- Dmuchowski W, Bytnerowicz A. 1995. Monitoring environmental pollution in Poland by chemical analysis of Scots pine (Pinus sylvestris L.) needles. Environmental Pollution 87:87–104. DOI: 10.1016/S0269-7491(99)80012-8.
- Falandysz J, Brudnowska B, Kawano M, Wakimoto T. 2001. Polychlorinated biphenyls and organochlorine pesticides in soils from the southern part of Poland. Archives of Environmental Contamination and Toxicology 40:173–178. DOI: 10.1007/s002440010160.
- Fleischer T, Gampe J, Scheuerlein A, Kerth G. 2017. Rare catastrophic events drive population dynamics in a bat species with negligible senescence. Scientific Reports 7:7370. DOI: 10.1038/s41598-017-06392-9.
- Froidevaux JSP, Boughey KL, Barlow KE, Jones G. 2017. Factors driving population recovery of the greater horseshoe bat (Rhinolophus ferrumequinum) in the UK: Implications for conservation. Biodiversity and Conservation 26:1601–1621. DOI: 10.1007/s10531-017-1320-1.
- Furey NM, Racey PA. 2016. Conservation ecology of cave bats. In: Voigt CC, Kingston T, editors. Bats in the Anthropocene: Conservation of bats in a changing world. Cham: Springer International Publishing. pp. 463–500.
- Fuszara E, Fuszara M, Kowalski M, Lesiński G, Cygan J, Nitkiewicz T, Szarlik A, Wojtowicz B. 2010. Population changes in Natterer’s bat Myotis nattereri and Daubenton’s bat M. daubentonii in winter roosts of central Poland. Polish Journal of Ecology 58:769–782.
- Gaisler J, Řehák Z, Zukal J. 2006. Výzkum netopýrů v Moravském krasu: Historie a současný stav [Bat research in the Moravian Karst: Its history and present state]. Vespertilio 9–10:75–85.
- GEF. 2004. National programme for the implementation of the Stockholm convention. Report of Project GF/POL/01/004 entitled “Enabling Activities to Accelerate the Implementation of the Stockholm Convention on Persistent Organic Pollutants”, Warszawa.
- Godawa J, Wołoszyn BW. 1990. Nietoperze (Mamalia, Chiroptera) Ojcowskiego Parku Narodowego [Bats (Mamalia, Chiroptera) of Ojców National Park]. Prądnik 1:143–148.
- Gottfried I, Gottfried T, Lesiński G, Hebda G, Ignaczak M, Wojtaszyn G, Jurczyszyn M, Fuszara M, Fuszara E, Grzywiński W, Błachowski G, Hejduk J, Jaros R, Kowalski M. 2020. Long-term changes in winter abundance of the barbastelle Barbastella barbastellus in Poland and the climate change - Are current monitoring schemes still reliable for cryophilic bat species? PLoS ONE 15:e0227912. DOI: 10.1371/journal.pone.0227912.
- Gradziński M, Michalska B, Wawryka M, Szelerewicz M. 2007. Jaskinie Ojcowskiego Parku Narodowego [Caves of Ojców National Park]. Ojców: OPN.
- Gradziński M, Szelerewicz M. 2004. Liczba i rozmieszczenie jaskiń Wyżyny Krakowsko-Wieluńskiej – Stan obecny [Number and distribution of caves in the Kraków-Wieluń Upland - Current state]. Jaskinie 3:28–30.
- Gregory RD, Vorisek P, Van Strien A, Gmelig Meyling AW, Jiguet F, Fornasari L, Reif J, Chylarecki P, Burfield IJ. 2007. Population trends of widespread woodland birds in Europe. Ibis 149:78–97. DOI: 10.1111/j.1474-919X.2007.00698.x.
- Grol BPFE, Voute AM. 2010. Hibernating bats in the Schenkgroeve, an artificial limestone cave in south Limburg, the Netherlands. Lutra 53:29–46.
- Grzywiński W, Nowak J, Kozakiewicz K, Węgiel A. 2015. Zimowy monitoring nietoperzy w jaskiniach Ojcowskiego Parku Narodowego [Winter bat monitoring in the caves of the Ojców National Park]. Prądnik 25:89–104.
- GUS. 2020. Statistical analyses. Environment. Warsaw: Statistics Poland (GUS).
- Harmata W. 1981. Zmiany populacji nietoperzy (Chiroptera) w niektórych jaskiniach Wyżyny Krakowsko-Częstochowskiej w latach 1945-1979 [Changes in the population of bats (Chiroptera) in some caves of the Kraków-Częstochowa Upland between 1945 and 1979]. Rocznik Muzeum Okregowego W Czestochowie 5:23–30.
- Hernout BV, Arnold KE, McClean CJ, Walls M, Baxter M, Boxall ABA. 2016. A national level assessment of metal contamination in bats. Environmental Pollution 214:847–858. DOI: 10.1016/j.envpol.2016.04.079.
- Hope PR, Jones G. 2012. Warming up for dinner: Torpor and arousal in hibernating Natterer’s bats (Myotis nattereri) studied by radio telemetry. Journal of Comparative Physiology B 182:569–578. DOI: 10.1007/s00360-011-0631-x.
- Jan P-L, Farcy O, Boireau J, Le Texier E, Baudoin A, Le Gouar P, Puechmaille SJ, Petit EJ. 2017. Which temporal resolution to consider when investigating the impact of climatic data on population dynamics? The case of the lesser horseshoe bat (Rhinolophus hipposideros). Oecologia 184:749–761. DOI: 10.1007/s00442-017-3901-9.
- Jefferies DJ. 1972. Organochlorine insecticide residues in British bats and their significance. Journal of Zoology 166:245–263. DOI: 10.1111/j.1469-7998.1972.tb04088.x.
- Jones G, Jacobs DS, Kunz TH, Willig MR, Racey PA. 2009. Carpe noctem: The importance of bats as bioindicators. Endangered Species Research 8:93–115. DOI: 10.3354/esr00182.
- Jones G, Rebelo H. 2013. Responses of bats to climate change: Learning from the past and predicting the future. In: Adams RA, Pedersen SC, editors. Bat evolution, ecology, and conservation. New York, NY: Springer. pp. 457–478.
- Jung K, Threlfall CG. 2016. Urbanisation and its effects on bats - A global meta-analysis. In: Voigt CC, Kingston T, editors. Bats in the Anthropocene: Conservation of bats in a changing world. Cham: Springer International Publishing. pp. 13–33.
- Kervyn T, Lamotte S, Nyssen P, Verschuren J. 2009. Major decline of bat abundance and diversity during the last 50 years in southern Belgium. Belgian Journal of Zoology 139:124–132.
- Kokurewicz T. 1990. The decrease in abundance of the lesser horseshoe bat Rhinolophus hipposideros Bechstein, 1800 (Chiroptera, Rhinolophidae) in winter quarters in Poland. Myotis 28:109–118.
- Kondracki J. 2001. Geografia regionalna Polski [Regional geography of Poland]. Warszawa: PWN.
- Kowalski K. 1953. Materiały do rozmieszczenia i ekologii nietoperzy jaskiniowych w Polsce [Materials for the distribution and ecology of cave bats in Poland]. Fragmenta Faunistica 6:541–567.
- Kyek M, Kaufmann PH, Lindner R. 2017. Differing long term trends for two common amphibian species (Bufo bufo and Rana temporaria) in alpine landscapes of Salzburg, Austria. PLoS ONE 12:e0187148. DOI: 10.1371/journal.pone.0187148.
- Lehikoinen A, Brotons L, Calladine J, Campedelli T, Escandell V, Flousek J, Grueneberg C, Haas F, Harris S, Herrando S, Husby M, Jiguet F, Kålås JA, Lindström Å, Lorrillière R, Molina B, Pladevall C, Calvi G, Sattler T, Schmid H, Sirkiä PM, Teufelbauer N, Trautmann S. 2019. Declining population trends of European mountain birds. Global Change Biology 25:577–588. DOI: 10.1111/gcb.14522.
- Lima SL, O’Keefe JM. 2013. Do predators influence the behaviour of bats? Biological Reviews 88:626–644. DOI: 10.1111/brv.12021.
- Loman J, Andersson G. 2007. Monitoring brown frogs Rana arvalis and Rana temporaria in 120 south Swedish ponds 1989–2005. Mixed trends in different habitats. Biological Conservation 135:46–56. DOI: 10.1016/j.biocon.2006.09.017.
- Lučan RK, Weiser M, Hanák V. 2013. Contrasting effects of climate change on the timing of reproduction and reproductive success of a temperate insectivorous bat. Journal of Zoology 290:151–159. DOI: 10.1111/jzo.12021.
- Luckens MM, Davis WH. 1964. Bats: Sensitivity to DDT. Science 146:948. DOI: 10.1126/science.146.3646.948.a.
- Machado MC, Monsalve MA, Castelló A, Almenar D, Alcocer A, Monrós JS. 2017. Population trends of cave-dwelling bats in the Eastern Iberian Peninsula and the effect of protecting their roosts. Acta Chiropterologica 19:107–118. DOI: 10.3161/15081109ACC2017.19.1.008.
- McAney K. 2014. An overview of Rhinolophus hipposideros in Ireland (1994–2014). Vespertilio 17:115–125.
- Mehr M, Brandl R, Hothorn T, Dziock F, Förster B, Müller J. 2011. Land use is more important than climate for species richness and composition of bat assemblages on a regional scale. Mammalian Biology 76:451–460. DOI: 10.1016/j.mambio.2010.09.004.
- Motte G, Libois R. 2002. Conservation of the lesser horseshoe bat (Rhinolophus hipposideros Bechstein, 1800) (Mammalia: Chiroptera) in Belgium. A case study of feeding habitat requirements. Belgian Journal of Zoology 132:47–52.
- Mühldorfer K, Speck S, Kurth A, Lesnik R, Freuling C, Müller T, Kramer-Schadt S, Wibbelt G. 2011. Diseases and causes of death in European bats: Dynamics in disease susceptibility and infection rates. PLoS ONE 6:e29773. DOI: 10.1371/journal.pone.0029773.
- Nowak J, Kozakiewicz K. 2000. Zimowe spisy nietoperzy na Wyżynie Krakowskiej w latach 1993–1999 [Winter bat censuses in the Kraków Upland in 1993–1999]. Studia Chiropterologica 1:43–56.
- Pannekoek J, van Strien AJ. 2005. Trim 3 manual (TRends & Indices for Monitoring data). Netherlands: Statistics.
- Paradis E, Baillie SR, Sutherland WJ, Gregory RD. 2002. Exploring density-dependent relationships in demographic parameters in populations of birds at a large spatial scale. Oikos 97:293–307. DOI: 10.1034/j.1600-0706.2002.970215.x.
- Perlińska A, Hamera-Dzierżanowska A. 2016. Gradacje szkodników pierwotnych sosny w Lasach Państwowych [Outbreaks of the primary Scots pine pests in state forests]. Studia I Materialy CEPL W Rogowie 46:32–42.
- Przybyłowicz Ł, and Buszko J. 2008. Lepidoptera of the Ojców National Park. In: Klasa A, and Partyka J, editors. Monograph of the Ojców National Park. Nature. Ojców: Ojców National Park. pp. 577–588.
- R Core Team. 2018. R: A language and environment for statistical computing. Vienna, Austria: R Foundation for Statistical Computing.
- Racey PA, Entwistle AC. 2000. Life-history and reproductive strategies of bats. In: Crichton EG, Krutzsch PH, editors. Reproductive biology of bats. London: Academic Press. pp. 363–414.
- Rebelo H, Tarroso P, Jones G. 2010. Predicted impact of climate change on European bats in relation to their biogeographic patterns. Global Change Biology 16:561–576. DOI: 10.1111/j.1365-2486.2009.02021.x.
- Řehák Z, Gaisler J. 1999. Long-term changes in the number of bats in the largest man-made hibernaculum of the Czech Republic. Acta Chiropterologica 1:113–123.
- Rudolph B-U, Liegl A, Helversen OV. 2009. Habitat selection and activity patterns in the greater mouse-eared bat Myotis myotis. Acta Chiropterologica 11:351–361.
- Russo D, Ancillotto L. 2015. Sensitivity of bats to urbanization: A review. Mammalian Biology 80:205–212. DOI: 10.1016/j.mambio.2014.10.003.
- Russo D, Jones G. 2015. Bats as bioindicators: An introduction. Mammalian Biology 80:157–158. DOI: 10.1016/j.mambio.2015.03.005.
- Rydell J, Eklöf J, Fransson H, Lind S. 2019. Long-term increase in hibernating bats in Swedish mines - Effect of global warming? Acta Chiropterologica 20:421–426. DOI: 10.3161/15081109ACC2018.20.2.012.
- Sherwin HA, Montgomery WI, Lundy MG. 2013. The impact and implications of climate change for bats. Mammal Review 43:171–182. DOI: 10.1111/j.1365-2907.2012.00214.x.
- Sieradzki A, and Mikkola H. 2020. A review of European owls as predators of bats. In: Mikkola H, editor. Owls. London: IntechOpen. DOI: 10.5772/intechopen.90330.
- Sławski M, and Sławska M, 2019. Problemy prognozowania masowych pojawów osnui gwiaździstej Acantholyda posticalis Mats [Problems of forecasting mass appearances of Acantholyda posticalis]. Materials of the conference “Current Problems of Forest Protection”, 15–17 October 2019, Ameliówka, LKP “Puszcza Świętokrzyska”.
- Spitzenberger F, Engelberger S. 2013. Negative trend reversal after 16 years of constant growth: The case of Rhinolophus hipposideros in an Austrian mass hibernaculum (Chiroptera: Rhinolophidae). Lynx 44:149–156.
- Stawski C, Willis CKR, Geiser F. 2014. The importance of temporal heterothermy in bats. Journal of Zoology 292:86–100. DOI: 10.1111/jzo.12105.
- Stebbings RE. 1989. Conservation of European bats. London: Christopher Helm.
- Szelerewicz M, Górny A. 1986. Jaskinie Wyżyny Karkowsko-Wieluńskiej [Caves of Kraków-Wieluń Upland]. Warszawa-Kraków: PTTK Kraj.
- Toffoli R, Calvini M. 2019. Long term trend of an endangered bat species in north-western Italy. Folia Zoologica 68:95–99. DOI: 10.25225/fozo.006.2019.
- Tournant P, Afonso E, Roué S, Giraudoux P, Foltête J-C. 2013. Evaluating the effect of habitat connectivity on the distribution of lesser horseshoe bat maternity roosts using landscape graphs. Biological Conservation 164:39–49. DOI: 10.1016/j.biocon.2013.04.013.
- Uhrin M, Benda P, Obuch J, Urban P. 2010. Changes in abundance of hibernating bats in central Slovakia (1992–2009). Biologia 65:349–361. DOI: 10.2478/s11756-010-0020-z.
- Van der Meij T, Van Strien AJ, Haysom KA, Dekker J, Russ J, Biala K, Bihari Z, Jansen E, Langton S, Kurali A, Limpens H, Meschede A, Petersons G, Presetnik P, Prüger J, Reiter G, Rodrigues L, Schorcht W, Uhrin M, Vintulis V. 2015. Return of the bats? A prototype indicator of trends in European bat populations in underground hibernacula. Mammalian Biology 80:170–177. DOI: 10.1016/j.mambio.2014.09.004.
- Voigt CC, Kingston T. 2016. Bats in the Anthropocene. In: Voigt CC, Kingston T, editors. Bats in the Anthropocene: Conservation of bats in a changing world. Cham: Springer International Publishing. pp. 1–9.
- Wołoszyn BW. 1976. Bemerkungen zur Populationsentiwicklunk der Klainen Hufeisennase, Rhinolophus hipposideros (Bechstein, 1800) in Polen [Remarks on the population development of the Lesser horseshoe bat, Rhinolophus hipposideros (Bechstein, 1800) in Poland]. Myotis 14:37–52.
- Wu J. 2016. Detection and attribution of the effects of climate change on bat distributions over the last 50 years. Climatic Change 134:681–696. DOI: 10.1007/s10584-015-1543-7.
- Zahn A, Rodrigues L, Rainho A, Palmeirim JM. 2007. Critical times of the year for Myotis myotis, a temperate zone bat: Roles of climate and food resources. Acta Chiropterologica 9:115–125. DOI: 10.3161/1733-5329(2007)9[115:CTOTYF]2.0.CO;2.
- Zahn A, Rottenwallner A, Güttinger R. 2006. Population density of the greater mouse-eared bat (Myotis myotis), local diet composition and availability of foraging habitats. Journal of Zoology 269:486–493. DOI: 10.1111/j.1469-7998.2006.00081.x.
- Zukal J, Berková H, Banďouchová H, Kováčová V, and Pikula J. 2017. Bats and caves: Activity and ecology of bats wintering in caves. In: Karabulut S, and Çinku M, editors. Cave investigation. London: IntechOpen. DOI: 10.5772/intechopen.69267.
- Zukal J, Pikula J, Bandouchova H. 2015. Bats as bioindicators of heavy metal pollution: History and prospect. Mammalian Biology 80:220–227. DOI: 10.1016/j.mambio.2015.01.001.