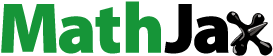
Abstract
The red swamp crayfish, Procambarus clarkii, and the marbled crayfish, Procambarus virginalis, are invasive species expanding their ranges on a global scale. Their spread is favored by the fact that these crayfish are also aquaculture species of great economic importance (P. clarkii) or promising prospects in this regard (P. virginalis). This study represents the first attempt to assess the impact of diet supplementation with fatty acids on growth, survival, and fatty acid content in these crayfish species, as well as the first record of fatty acid patterns in the obligate parthenogenetic marbled crayfish. Our results showed that supplementation with saturated palmitic acid increased P. clarkii survival, which has not previously been reported for crustaceans. This positive effect on the part of palmitic acid supplementation was not observed for P. virginalis individuals. Marbled crayfish had significantly higher survival rates than red swamp crayfish regardless of the diet used. The growth of both species was not affected by fatty acid supplementation. Our study indicated that young marbled crayfish had a poorer fatty acid profile than juvenile conspecifics and red swamp crayfish. Fatty acid supplementation and type of diet significantly affected the fatty acid composition in the crayfish tissues. There was an increase in unsaturated α-linolenic acid for P. clarkii and palmitic acid for P. virginalis. Palmitic acid quantitatively dominated in both species regardless of the experimental treatment, with the exception of juvenile P. virginalis, which was fed an oligochaete diet rich in EPA.
1. Introduction
Freshwater crayfish invasions are an emerging problem worldwide and can have a negative impact on the biodiversity of aquatic ecosystems (Gherardi Citation2007). Two invasive species that are particularly expanding their ranges are red swamp crayfish Procambarus clarkii (Girard 1852) and marbled crayfish Procambarus virginalis (Lyko Citation2017; Decapoda, Cambaridae). Each of these species is included on the list of Invasive Alien Species of the European Union 2014. The first record of marbled crayfish is from the German aquarium trade in 1995 (Scholtz et al. Citation2003; Lyko Citation2017). This species became widely distributed in subsequent years in many European countries (Germany, Belgium, France, Czech Republic, Estonia, Italy, Malta, Romania, Slovakia, Hungary, Croatia, Ukraine, and recently in Poland; Mazurska et al. Citation2019) and on Madagascar, where it represents a quickly expanding population (Andriantsoa et al. Citation2020). This species likely separated from Procambarus fallax, which is native to the American states of Florida and Georgia (Taylor et al. Citation2007; Dorn & Volin Citation2009), because of major genetic changes (Martin et al. Citation2016). Compared to the red swamp crayfish, the obligate parthenogenetic marbled crayfish appears to be even more resistant to various environmental conditions (in localities that freeze over) and have higher fertility (Kozák et al. Citation2015). In contrast to the low commercial value of the marbled crayfish (which is locally consumed, e.g., in Madagaskar; Adriantsoa et al. Citation2020), the red swamp crayfish is an aquaculture species of significant commercial value that accounts for 12% of total crustacean aquaculture production (FAO Yearbook 2018), as well as one of the most promising freshwater species for aquaculture in China (Jin et al. Citation2019). The original habitat of this species is water bodies and wetlands from Florida to Mexico and from the southern outflow of the Mississippi River to Illinois and southwestern Indiana (Nagy et al. Citation2019). The red swamp crayfish adapts well to a wide range of habitats, including lakes, rivers, streams, ponds, seasonally flooded wetlands, canals, reservoirs, rice fields, and ditches with mud or sandy bottoms and significant amounts of detritus (Gherardi et al. Citation1999). This species tolerates salinity of up to 12 ppt, a pH of 3.8, and hypoxia (dissolved oxygen ≤ 2 mg/L) and is resistant to pollution (Suárez-Serrano et al. Citation2010). The marbled crayfish is a tolerant and adaptable taxon with low demands for living conditions, both in nature and in the laboratory (Jimenez & Faulkes Citation2009; Kaldre et al. Citation2015). Procambarus clarkii and P. virginalis, like most crayfish, are opportunistic omnivores that consume plant material, animals, detritus, and sediment (Gutiérrez-Yurrita et al. Citation1998; Alcorlo et al. Citation2004; Pérez-Bote Citation2004; Gherardi & Barbaresi Citation2007; Kozák et al. Citation2015).
Diet type and fatty acid content are important factors affecting the life-history traits of various living organisms (Barrento et al. Citation2010, Kaliszewicz et al. Citation2018). Fatty acid supplementation can cause both positive and negative reactions or, in some cases, have no specific consequences for individuals. A large number of data relate to the fatty acid requirements of non-native marine shrimps that dominate global aquaculture (Wickins & O’C Lee Citation2002; Parakarma et al. Citation2009). A study on juvenile Litopenaeus vannamei shrimps showed that n-6 and n-9 fatty acids are essential, although n-3 fatty acids promoted faster growth than n-6 fatty acids (Lim et al. Citation1997). Highly unsaturated fatty acids (20- and 22-carbon fatty acids with three or more double bonds), i.e., arachidonic, eicosapentaenoic (EPA), and DHA acids, when used to supplement young L. vannamei individuals, offered greater nutritional value in terms of higher final weight and growth as compared to polyunsaturated fatty acids (PUFA, containing more than one double bond), which included α-linolenic and linoleic acids (Gonzalez-Felix et al. Citation2003). In contrast to the results concerning shrimps, studies on the effect of fatty acid supplementation on crayfish are scarce. There is only a small amount of information on the fatty acid content of a few species: Faxonius rusticus, Pacifastacus leniusculus, and Procambarus clarkii (Ackefors et al. Citation1997; Adey & Larson Citation2020). To our knowledge, there are a lack of data on the effect of diet supplementation with fatty acids on growth, survival, and fatty acid patterns in the crayfish we studied.
In this study, we tested the effect of the diet supplementation with fatty acids on growth, survival, and fatty acid content in juvenile and young members of two invasive crayfish species: P. clarkii and P. virginalis. The three chosen types of diets differed in fatty acid composition, especially n-3 PUFA, and were enriched with saturated palmitic fatty acid and, additionally, unsaturated (n-3, n-6, and n-9) fatty acids. We put forward four hypotheses: (1) both invasive crayfish species have similar fatty acid patterns and demands, (2) the result of a particular fatty acid supplementation may vary depending on the diet, (3) diet supplementation with palmitic acid, which predominates in crayfish tissues, could be beneficial for growth and survival, and (4) a diet rich in long-chain polyunsaturated fatty acid (LC-PUFA) can promote the growth and survival of crayfish.
2. Materials and methods
2.1. The cultivation of study animals
Individuals of P. clarkii originated from a commercial culture. Individuals of P. virginalis were captured from invasive populations in Morskie Oko pond, in Warsaw (52°21′ N, 21°02′E), Poland. Adult crayfish were adapted to laboratory conditions. Females and males of P. clarkii were kept in pairs. Females of P. virginalis were kept three per 50-L aquarium at 22 ± 1°C with a 12 L:12D photoperiod. The crayfish had a varied diet consisting of commercial food, macrophytes, boiled nettle leaves, aquatic invertebrates (brine shrimp, Chironomidae larvae, and Tubifex oligochaetes), and fish tissue. These conditions were favorable for the growth and reproduction of both species. Females that carried eggs on abdominal pereopods were kept separately, with one female per 50-L aquarium. When juvenile crayfish of both species hatched (up to 180 per female), siblings of the same age were used for the laboratory experiment.
2.2. Analysis of fatty acid content in food
Before the experiments with crayfish were conducted, we analyzed the fatty acid content in the food used: the aquatic oligochaete Tubifex sp. (Diet 1), fish tissue of Oreochromis niloticus (Diet 2), and nettle leaves (Diet 3). The materials were pulverized in a mortar and then placed in an 80°C water bath with 2 mL of 0.5 M sodium methoxide (NaOCH3) for 15 min. Then, 4 mL of 14% boron trifluoride/methanol (BF3/MeOH) were added. The sample was heated for 20 min. After cooling, 4 mL of hexane were added and mixed, and 4 mL of sodium chloride were added. The lipid-containing fraction was evaporated until dry with nitrogen and resuspended in 1 mL of hexane. This BF3/MeOH method has been described as applicable to biological samples (Igarashi et al. Citation2004). Fatty acid methyl esters (FAMEs) were analyzed using a single quadrupole gas chromatograph-mass spectrometer Shimadzu GCMS-QP2010 Ultra equipped with a capillary column (30 m x 0.25 mm x 0.25 µm film) with the following configuration: oven 40°C (2 min) to 320°C at 6°C/min, detector temperature 280°C, and injector 280°C (total run time 49 min/sample). The carrier gas was helium at a flow rate of 1.42 mL/min. A 1-µl aliquot of each sample was injected. Then, FAMEs were identified by comparison to retention time standards and quantified by comparison to internal standards of known concentrations. The reference methyl esters of fatty acids were purchased from Sigma-Aldrich.
2.3. Experiments with fatty acid supplementation in P. clarkii
Two experiments on two different diets enriched with a single polyunsaturated or saturated fatty acid were conducted to analyze the effect on the survival, growth and fatty acid patterns of P. clarkii crayfish.
In the first experiment, Diet 1, consisting of the live freshwater oligochaete Tubifex sp and scalded nettle leaves, was used only as a supplement. The fatty acids composition in Diet 1 is given in . Diet 1 was enriched with the chosen fatty acids. There were five experimental treatments. Four treatments involved additional supplementation with the following fatty acids: (1) α-linolenic (18:3 n-3), an essential fatty acid that can only be synthetized by plants; (2) linoleic (18:2 n-6), often the third most prevalent fatty acid in P. clarkii; (3) oleic (18:1 n-9), the major fatty acid in P. clarkii; and (4) palmitic (16:0), the most common saturated fatty acid found in live crayfish, (5) the control treatment was without the enrichment of any additional fatty acids. Ninety-five juvenile (11 mm long) P. clarkii siblings were randomly divided into five treatments (). The crayfish were placed individually in containers 190 mm in diameter filled with 750 mL of prepared tap water. The aeration of the water in each container was provided by the air-pump. Depending on the experimental treatment, the food was prepared in the following way. A 10 mm diameter disk of nettle leaves and 10 mm fragments of Tubifex sp. were soaked for 10 min in one of the following fatty acids: (1) 70% α-linolenic (Sigma-Aldrich), (2) ≥99% linoleic (Sigma-Aldrich), (3) 99% oleic (REKO), or (4) ≥ 99% palmitic acid (Sigma-Aldrich) pulverized in a mortar, or they were (5) placed in clean water (control treatment).
Table I. Mean fatty acid content (mol% of the total fatty acids) of the components of a diet for crayfish without the addition of fatty acids. Statistically significant differences at p < 0.05 are indicated by different letters (one-way ANOVA post-hoc Tukey HSD test). N = 3
Figure 1. Schematic diagram summarizing the experimental design. Differences between Diet 1, Diet 2, and Diet 3 are explained in the text. Numbers in squares indicate the total number of crayfish individuals used and the number of replicates per treatment. The number of individuals per the container is shown in parentheses. The experimental treatments involved additional supplementation with the fatty acids: (P) palmitic, (O) oleic, (L) linoleic, (αL) α-linolenic, and (C) control.
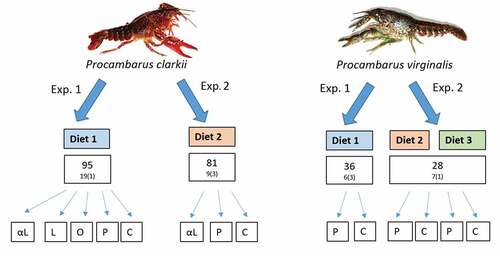
In the second experiment, Diet 2, consisting of the freshwater fish tissue of Oreochromis niloticus, was used. The content and proportion of fatty acids in Diet 2 are given in . The differences in fatty acid content between Diet 1 and Diet 2 are shown in . Diet 2 was enriched with the following fatty acids: (1) supplementation with α-linolenic 70% fatty acid (Sigma-Aldrich) and (2) supplementation with palmitic ≥ 99% fatty acid (Sigma-Aldrich), as well as (3) a control without fatty acid supplementation (C). Eighty-one juvenile (11 mm long) P. clarkii siblings were divided randomly into three treatments (). The crayfish were placed three per container, and each container was filled with 12 L of prepared tap water. A filter that aerated the water was added to each container.
2.4. Experiments with fatty acid supplementation in P. virginalis
Two experiments with three diets enriched with saturated palmitic fatty acid were conducted to analyze the effect on the survival, growth, and fatty acid patterns of P. virginalis crayfish.
In the first experiment, Diet 1, which was similar to that fed to P. clarkii, was used: live freshwater oligochaete Tubifex sp. and scalded nettle leaves as a supplement. Thirty-six juvenile (11 mm long) P. virginalis siblings were randomly divided into two treatments: (1) additional supplementation with ≥ 99% palmitic fatty acid (Sigma-Aldrich) and (2) control without fatty acid supplementation (C; ). The crayfish were placed three per container, and each container was filled with 3 L of prepared tap water. The aeration of the water in each container was provided by an air pump. Depending on the experimental treatment, the food was prepared. Ten mm fragments of Tubifex sp. were enriched with ≥ 99% palmitic acid (Sigma-Aldrich) pulverized in a mortar or went without fatty acid supplementation (control treatment).
In the second experiment, two diets enriched with the saturated palmitic fatty acid were used. Diet 2 was the same as that used for P. clarkii and consisted of freshwater fish tissue (Oreochromis niloticus). Diet 3 was a plant-based diet that only consisted of scalded nettle leaves. The differences in fatty acid content between Diet 2 and Diet 3 are shown in . Twenty-eight young (47 mm long) P. virginalis siblings were divided randomly into four experimental treatments: (1) fish tissue with additional supplementation with palmitic acid, (2) fish tissue without fatty acid supplementation (control F), (3) nettle leaves with additional supplementation with palmitic acid, and (4) nettle leaves without fatty acid supplementation (control N). The crayfish were placed individually in containers filled with 3 L of prepared tap water. On the bottom of the containers, 100 ml of sand was placed as a substrate. The aeration of the water in each container was provided by an air pump.
Figure 3. The differences in fatty acid contents between Diet 2 and Diet 3 of Procambarus virginalis.
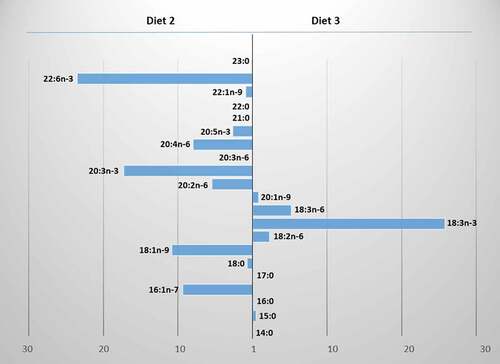
In order to enrich the food with fatty acid, a 10 mm long fragment of Tubifex sp, a 20 mm disk of nettle leaves, and 10 or 100 mm3 of fish tissue, depending on the experimental treatment and the size of the crayfish, were enriched with fatty acid. All food components easily absorb chemical compounds; are perfect vectors for various organic substances, including fatty acids; and were readily consumed by the crayfish. There were ~3 mg of acid added per food item for juvenile crayfish and ~9 mg of fatty acid added per food item for young crayfish. For control treatments, food was placed in clean water for the same amount of time as the supplemented food items. More food was delivered than the crayfish could eat in 48 hours. Excess food was removed before adding new portions. Thirty percent of the water volume was changed once a week. The water temperature was 22 ± 1°C, with a photoperiod of 8 L:16D. The crayfish were counted and measured every two weeks. Length (mm) was measured from rostrum to telson. Growth and survival were calculated using the following formulae:
At the end of the experiments (60–65-th day), the middle pereopod of each crayfish was cut off and frozen at −20°C for fatty acid analysis. The duration of the experiments was set at 60–65 days as a result of the minimum time needed to show differences of the growth rate and survival of the crayfish and the need to change experimental conditions related to water volume per crayfish individual.
2.5. Analyses of fatty acid content in tissues of crayfish
Lipids were extracted from the pereopods of juvenile and young crayfish (one pereopod per specimen) at the end of the experiments. From four to twelve samples were analyzed per treatment depending on the experiment. Additionally, the analysis of fatty acid content was carried out in young P. clarkii crayfish that were 45 mm long (N = 4) and had been fed Diet 1 and kept in control 35-L aquaria. The fatty acids were extracted, and their content was analyzed in the same manner as the food (see 2.2).
2.6. Statistical analyses
The data were analyzed for normality with the Shapiro–Wilk test. The differences in the growth and survival of crayfish fed different diets enriched with fatty acids and the fatty acid content in crayfish tissues between species and treatments were analyzed using one-way ANOVA followed by a post-hoc Tukey’s test. The survival of crayfish, placed individually or three per container, was transformed into the same unit (%), allowing comparison between the experiments. The effects of diet supplementation with fatty acids were analyzed by the comparison within a particular experiment. To determine the effects of different diets on growth, survival rate, and fatty acid profile, the control crayfish were compared between experiments. A significance level of α = 0.05 was used for statistical analysis. All statistical analyses were performed using Statistica 13 (StatSoft, Inc., Tulsa, OK, USA).
3. Results
3.1. Effect of diet on the growth and survival of crayfish
Diet 2 (fish-based), which was naturally rich in DHA, significantly promoted the growth of juvenile P. clarkii individuals as compared to the individuals fed Diet 1 with a natural predominance of EPA and α-linolenic acid (ANOVA, F1,7 = 8.41, p < 0.0001; ).
Figure 4. Somatic growth expressed as a percentage of increase in body size for P. clarkii fed two different diets, Diet 1 rich in EPA or Diet 2 rich in DHA, and supplemented in different treatments with (P) palmitic, (O) oleic, (L) linoleic, or (αL) α-linolenic fatty acids. (C) Control crayfish went without supplementation. Bars sharing the same letter are not significantly different. Error bars represent ± 1SE.
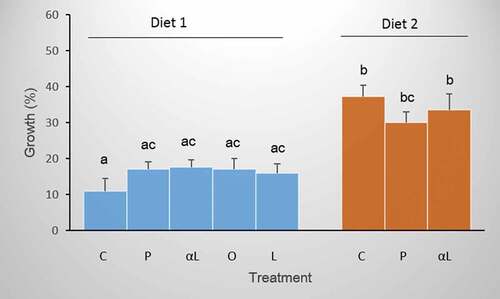
The marbled crayfish reacted quite differently. There were no observed significant differences in the rate of growth of young control P. virginalis fed Diet 2 (fish naturally rich in DHA) and Diet 3 (nettle leaves rich in natural α-linolenic acid; ANOVA, p > 0.05).
The survival rate of both crayfish species was different P. virginalis had significantly higher survival than P. clarkii but the results were not dependent on diet (ANOVA, p > 0.05).
3.2. Additional dietary supplementation with fatty acids and its impact on crayfish growth and survival
In contrast to the effect of diet, additional supplementation with fatty acids affected the survival of P. clarkii crayfish (ANOVA, F1,7 = 5.59, p < 0.0001; ). Individuals fed Diet 1 supplemented with palmitic acid (P treatment) had higher survival than crayfish in all other treatments (Tukey’s test, p < 0.03), with the exception of the crayfish fed Diet 1 enriched with α-linolenic acid (αL treatment). In contrast to the treatment with palmitic acid supplementation, dietary supplementation with unsaturated n-3, n-6, and n-9 fatty acids did not affect the survival of P. clarkii crayfish (Tukey’s test, p > 0.05). Interestingly, the positive effect of palmitic acid supplementation on the survival of P. clarkii was observed only in the experiment with Diet 1. Crayfish fed Diet 2, based on fish tissue enriched with palmitic or α-linolenic acid, did not survive more often than individuals in the control group (Tukey’s test, p > 0.05).
Figure 5. The survival of P. clarkii fed two diets, Diet 1 rich in EPA or Diet 2 rich in DHA, and supplemented in different treatments with (P) palmitic, (O) oleic, (L) linoleic, or (αL) α-linolenic fatty acids. (C) Control crayfish went without supplementation. Bars sharing the same letter are not significantly different. Error bars represent ± 1SE.
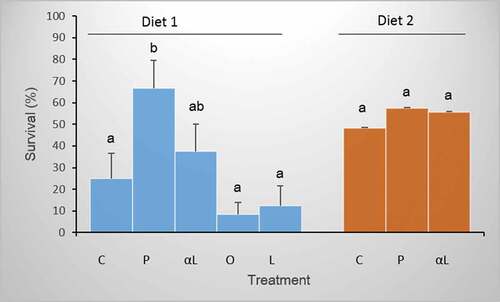
The survival of juvenile P. virginalis did not differ between experimental treatments (94% ±5) but was significantly higher than in juvenile P. clarkii (8–37% ±12; ANOVA, F6,65 = 9.64, p < 0.0001) with the exception of red swamp crayfish supplemented with palmitic acid (67% ±13; p > 0.05). The survival of juvenile P. virginalis (94% ±5) did not differ from the survival of young P. virginalis (86% ±14-100%; ANOVA, p > 0.05).
Analyses showed no significant effect on the part of dietary supplementation on the growth rate of both species (ANOVA, p > 0.05).
3.3. Fatty acid content in crayfish fed different diets without supplementation
The diets used in the experiment affected the content of fatty acids in crayfish tissues. Palmitic acid was the most abundant fatty acid in the juvenile P. clarkii (). Other acids included, by quantity were: oleic >arachidonic >EPA >linoleic >stearic >DHA in the control crayfish fed Diet 1 and arachidonic >stearic >oleic >EPA >DHA >linoleic in the control crayfish fed Diet 2 (). In the juvenile P. virginalis, which fed Diet 1 (nettle leaves rich in natural α-linolenic acid and oligochaetes rich in EPA) the most abundant was EPA, than arachidonic and palmitic fatty acids. Other acids included, by quantity were: oleic >stearic >linoleic > eicosadienoic >DHA >γ-linolenic ().
Table II. Mean fatty acid content (mol% of the total fatty acids) of tissues of Procambarus clarkii from treatments that differ in diet (Diet 1 – oligochaetes, Diet 2 - fish tissue) and supplementation: C—control, no fatty acid added; O—oleic acid added; L—linoleic acid added; αL—α-linolenic acid added; P—palmitic acid added. The highest significant values are marked in bold. Statistically significant differences at p < 0.05 between particular treatments are indicated by different letters (one-way ANOVA post-hoc Tukey HSD test for different N). N = 3–9
Table III. Mean fatty acid content (mol% of the total fatty acids) of tissues of Procambarus virginalis from treatments that differ in diet (Diet 1- oligochaetes, Diet 2- fish tissue, Diet 3 - nettle leaves) and supplementation: C—control, no fatty acid added; P—palmitic acid added. The highest significant values are marked in bold. Statistically significant differences at p < 0.05 between particular treatments are indicated by different letters (one-way ANOVA post-hoc Tukey HSD test). N = 7–12
The young marbled crayfish had a much poorer fatty acid composition than juvenile conspecifics and red swamp crayfish. Again palmitic acid was the most abundant fatty acid (). In the young P. virginalis other acids included, by quantity were: stearic >arachidonic >EPA >linoleic >DHA >oleic in the control crayfish fed Diet 2 (fish naturally rich in DHA) and stearic >arachidonic >EPA >α-linolenic >γ-linolenic >linoleic in the control crayfish fed plant-based Diet 3 (nettle leaves rich in natural α-linolenic acid).
The analysis of the fatty acid content in the crayfish tissues of both species indicated differences in the effect of a diet. Juvenile P. clarkii fed Diet 1 displayed more linoleic and less behenic fatty acid compared to the individuals fed Diet 2 (Tukey’s test, p < 0.0002, p < 0.0008, respectively; ). The young P. virginalis fed Diet 3 had more α-linolenic and γ-linolenic acids (Tukey’s test, p = 0.001, p = 0.04, respectively) and less palmitic, stearic and oleic fatty acids compared to the individuals fed Diet 2 (Tukey’s test, p = 0.01, p = 0.0002, p = 0.0002 respectively; ).
The ratio of n-3 to n-6 PUFAs in the tissues of P. clarkii fed Diet 1 and those fed Diet 2 was significantly different (Tukey’s test, p = 0.003). There were no such significant difference for P. virginalis fed Diet 2 and those fed Diet 3 (Tukey’s test, p > 0.05).
The Diet 2 naturally rich in DHA increased the content of this acid in the bodies of both crayfish species. The individuals of P. clarkii had a higher ratio of DHA to EPA (RDHA/EPA = 0.68) than those fed Diet 1 rich in α-linolenic and EPA acids (RDHA/EPA = 0.54). The P. virginalis crayfish fed Diet 2 had also a higher ratio of DHA to EPA (RDHA/EPA = 0.74) than those fed Diet 3 rich in α-linolenic acid (RDHA/EPA = 0.21). The dominance of these acids in the diet reflects their pattern in the crayfish tissues and confirms their assimilation from food by two studied species. Interestingly, the content of EPA, which can be converted from the 18-carbon α-linolenic acid, was higher in the P. virginalis crayfish fed Diet 3 naturally rich in α-linolenic acid, but the difference was not statistically significant (Tukey’s test, p > 0.05; ).
3.4. Fatty acid content in crayfish fed different diets supplemented with fatty acids
Diet 2, which was enriched with α-linolenic fatty acid, caused a significant increase in the content of this fatty acid in P. clarkii tissues (Tukey’s test, p = 0.03; ). Additionally, a higher percentage of EPA was observed in the red swamp crayfish supplemented with α-linolenic acid independent of diet, but the difference was not statistically significant (Tukey’s test, p > 0.05; ). These findings suggest the ability of P. clarkii to convert 20-carbon EPA from the precursor 18-carbon α-linolenic acid, which is comparable to the results for P. virginalis fed plant-based Diet 3. A significant increase in palmitic acid content was observed in the tissue of P. virginalis individuals fed Diet 3, which was supplemented with this fatty acid (Tukey’s test, p = 0.002; ). In turn, Diet 1 supplemented with palmitic acid led to significantly higher amount of stearic acid in P. virginalis (Tukey’s test, p = 0.001; ).
Significant differences were observed in the ratio of DHA to EPA (ANOVA, F1,6 = 12.18, p < 0.00001). The RDHA/EPA ratio was higher for juvenile P. clarkii (RDHA/EPA in the range of 0.37–0.67) as compared to the RDHA/EPA (0.20) for juvenile P. virginalis (Tukey’s test, p = 0.0002). There were differences between treatments with supplementation for P. clarkii: individuals fed Diet 2 supplemented with palmitic fatty acid had a significantly higher RDHA/EPA (0.67) as compared to siblings from other treatments (RDHA/EPA in the range of 0.37–0.54; Tukey’s test, p = 0.0002), with the exception of the treatment involving the α-linolenic supplementation of Diet 2 (RDHA/EPA = 0.61).
In contrast to the results for the DHA to EPA ratio, there were no significant differences in the ratio of n-3 to n-6 PUFAs between species and treatments with supplementation (ANOVA, p > 0.05). The ratio of n-3 to n-6 PUFAs for juvenile P. clarkii (Rn-3/n-6 = 0.79) was similar to the ratio for juvenile P. virginalis (Rn-3/n-6 = 0.87).
3.5. Comparison of fatty acid content in juvenile and young individuals of both crayfish species
The results revealed that young P. virginalis individuals have a poorer fatty acid profile than juvenile crayfish of both species (). Saturated pentadecanoic and margaric acids, unsaturated palmitoleic acid, and dihomo-γ-linolenic acid were not detected in young P. virginalis, independent of diet. Saturated myristic and behenic and unsaturated oleic and eicosenoic acids were measured at significantly lower levels (ANOVA, F14,79 = 3.1, p < 0.001; p < 0.00001; p < 0.01; p < 0.00001; respectively). In contrast, saturated fatty acids, such as palmitic and stearic, were measured at significantly higher values in young P. virginalis as compared to juvenile conspecifics and juvenile P. clarkii (ANOVA, F14,79 = 22.1, p = 0.001). The young P. virginalis individuals fed Diet 3, based on nettle leaves, have a significantly higher amount of α-linolenic acid in their muscle tissue than juvenile crayfish of both species (ANOVA, F14,79 = 7.7, p < 0.00001).
There were no differences in fatty acid profile between juvenile and young P. clarkii.
The RDHA/EPA ratio significantly differed between juvenile (RDHA/EPA = 0.20) and young P. virginalis (RDHA/EPA in the range of 0.80–0.94; ANOVA, F5,38 = 7.8, p = 0.00004). On the other hand, there were no differences between the RDHA/EPA ratios of juvenile (0.37–0.67) and young P. clarkii (0.56).
The ratio of n-3 to n-6 PUFAs did not differ between juvenile (Rn-3/n-6 = 0.87 for P. virginalis and 0.79 for P. clarkii, respectively) and young (Rn-3/n-6 = 0.81–0.90 for P. virginalis and 0.77 for P. clarkii, respectively) individuals of both species, independent of diet (ANOVA, p > 0.05).
4. Discussion
Diet supplementation affected fatty acid patterns in both invasive crayfish species, red swamp Procambarus clarkii and marbled Procambarus virginalis. The P. clarkii crayfish fed a diet based on fish supplemented by α-linolenic acid showed a higher content of this n-3 fatty acid in their tissues, indicating its good assimilation from the diet. This indicates that commercial decapod diet supplementation may be a method of enriching the nutritional value of these animals. High levels of n-3 fatty acids are recommended for human consumption (Gebauer et al. Citation2006). The marbled crayfish, P. virginalis, showed a higher palmitic acid content in its tissues when fed a diet based on nettle leaves supplemented with this saturated fatty acid. Interestingly, supplementation with palmitic acid did not affect the growth or survival of this species but appeared beneficial for the survival of red swamp crayfish, P. clarkii. To our knowledge, the positive effect of supplementation with palmitic acid on the survival of crustaceans has not yet been reported. Most crustacean species prefer saturated fatty acids in their diet as an energy source (Chen et al. Citation2015). The beneficial effect of menhaden, which is oil rich in palmitic acid and n-3 fatty acids compared to other diets, has been described for the growth and survival of Litopenaeus vannamei shrimp (Lim et al. Citation1997). However, the additional supplementation of palmitic acid in a diet rich in other saturated and unsaturated fatty acids has not yet been described. Quantitatively, palmitic acid was a dominant fatty acid in the tissues of both crayfish species studied in this research, independent of the diet supplementation. The literature indicated a large amount of palmitic acid in P. clarkii tissues and organs (Okajima et al. Citation1984; Zaglol & Eltadawy Citation2009), but to our knowledge, there is lack of information about the fatty acid profile in marbled crayfish. The present study represents the first record of fatty acid patterns in juvenile and young individuals of this parthenogenetic species. In addition to palmitic acid, the other major acids, by quantity, are stearic, arachidonic, and EPA. Young P. virginalis crayfish have a higher proportion of palmitic and stearic acids as compared to P. clarkii and juvenile conspecifics but significantly less oleic and palmitoleic acids and a poorer fatty acid profile in general. Both crayfish species, although invasive, have different fatty acid profiles, which indicates the falsity of our hypothesis regarding their similarity in terms of fatty acid patterns and demands. Despite the large amount of palmitic acid in the tissues of both studied crayfish species, the positive effect of supplementation with palmitic acid was observed only for red swamp crayfish, P. clarkii. These findings allow us to reject the hypothesis that diet supplementation with palmitic acid could be beneficial for crayfish, independent of species. The positive effect of palmitic acid supplementation was not observed for the P. clarkii crayfish fed a diet of fish rich in DHA, which confirms our hypothesis that the effect of a particular fatty acid supplementation on closely related individuals may vary depending on the diet. It is likely that, in crayfish farms, when a plant-based diet is used, palmitic acid should be supplemented to avoid cannibalism. It is possible that this effect also applies to other aquaculture crustaceans. The diet based on fish Oreochromis niloticus, which is naturally rich in DHA, promoted the growth of P. clarkii crayfish. This 22-carbon long-chain polyunsaturated fatty acid should be provided in the diet and is essential for many aquaculture crustaceans (Mourente & Rodríguez Citation1997). Neither a diet rich in EPA (20-carbon, n-3 fatty acid) nor supplementation with shorter 18-carbon fatty acids, such as α-linolenic (n-3) or linoleic acid (n-6), increased the growth or survival of P. clarkii or P. virginalis crayfish. However, an increase in EPA was observed in both crayfish species fed the oligochaete diet rich in EPA and juvenile P. virginalis fed nettle leaves rich in α-linolenic acid. This may suggest ability of these species to assimilate 20-carbon EPA from food and convert this n-3 PUFA from the 18-carbon precursor. The 22-carbon fatty acids, as well as 20-carbon EPA, cannot be synthetized de novo by crustaceans and must be converted from 18-carbon α-linolenic acid. This process requires chain elongation and desaturation steps, two times as many for DHA as for EPA production (Sprecher Citation2000). The ability to convert α-linolenic acid to EPA and DHA can vary across life stages (e.g., in Penaeus japonicus), and some species have been reported to be unable to perform this conversion (Takeuchi & Murakami Citation2007). The highest growth rate and survival of Penaeus chinensis shrimps were obtained with a diet rich in DHA, as compared to a diet containing arachidonic acid or a mixture of linoleic and α-linolenic acids (Xu et al. Citation1993). The results reported for another crayfish species, Astacus leptodactylus, indicated the most growth, molting, and survival on a diet of Clupeidae fish oil rich in DHA, as compared to individuals fed a diet of soybean oil rich in n-6 fatty acids and EPA (Valipour et al. Citation2011). However, the importance of a dietary DHA supply over EPA cannot be generalized to all crustaceans or crayfish. For instance, juvenile Cherax quadricarinatus, red claw crayfish, grew equally well on a diet containing α-linolenic, linoleic, and oleic acids as compared to a diet rich in DHA and EPA (Thompson et al. Citation2010; Li et al. Citation2011).
Studies on the effects of fatty acids on commercial organisms are very timely. Various effects on the part of given fatty acids and environmental conditions on organisms can be observed, as well as differences in the content of individual substances in body parts. The red swamp crayfish, due to its richer fatty acid composition, seems to be a more valuable aquaculture species than the marbled crayfish. However, the P. virginalis crayfish has recently been indicated as a promising object for aquaculture as a cheap protein source (Jurmalietis et al. Citation2019). This species displayed traits such as high survival even on plant diet, a poor fatty acid profile, potentially low fatty acid requirements, and parthenogenetic reproduction, which could make it an easy-farming species. Marbled crayfish mature quickly and reproduce several times a year, providing large numbers of female offspring. In the case of meat food deficiency, in contrast to other crayfish species, plant food is sufficient for the growth of this species. This could lead to competition with other crayfish species and ultimately their displacement from the environment.
5. Conclusions
A positive effect on the part of supplementation with palmitic fatty acid on the survival of red swamp crayfish, P. clarkii, was indicated, which has not yet been reported for crustaceans. Importantly, this effect depended on having a certain diet. The positive effect was not observed for crayfish fed a diet of fish naturally rich in DHA. This diet promoted the growth of P. clarkii. The hypothesis that the effect of a particular fatty acid supplementation and its content in tissues may vary depending on diet was confirmed. There were no positive effects on the part of diet and supplementation on the growth and survival of marbled crayfish, P. virginalis. Regardless of diet, the marble crayfish grew at the same rate. Palmitic acid was a dominant fatty acid in tissues of both crayfish species. Young P. virginalis crayfish, as compared to juvenile conspecifics and P. clarkii, have a poorer fatty acid profile, but saturated fatty acids, such as palmitic and stearic, were observed at significantly higher values. Marbled crayfish, with good tolerance for a plant diet and a higher survival rate, may have a higher dispersal ability than red swamp crayfish.
Acknowledgements
We thank the students of Faculty of Biology and Environmental Sciences at Cardinal Stefan Wyszyński University in Warsaw for their help in conducting the fatty acid experiment. We thank three anonymous reviewers for their constructive comments and suggestions. This research was funded by the Ministry of Science and Higher Education, PBBNS-13/17.
Disclosure statement
No potential conflict of interest was reported by the author(s).
Additional information
Funding
References
- Ackefors A, Castell J, Örde-Öström I-L. 1997. Preliminary results on the fatty acid composition of freshwater crayfish, Astacus astacus and Pacifastacus leniusculus, held in captivity. Journal of the World Aquaculture Society 28:97–105. DOI: 10.1111/j.1749-7345.1997.tb00967.x.
- Adey AK, Larson ER. 2020. Dominance and diet are unrelated within a population of invasive crayfish. Hydrobiologia 847(7):1587–1602. DOI: 10.1007/s10750-019-04088-x.
- Alcorlo P, Geiger W, Otero M. 2004. Feeding preferences and food selection of the red swamp crayfish, Procambarus clarkii, in habitats differing in food item diversity. Crustaceana 77:435–453. DOI: 10.1163/1568540041643283.
- Andriantsoa R, Jones J, Achimescu V, Randrianarison H, Raselimanana M, Andriatsitohaina M, Rasamy J, and Lyko F. 2020. Perceived socio-economic impacts of the marbled crayfish invasion in Madagascar. PLoS One 15(4):e0231773. DOI:10.1371/journal.pone.0231773
- Barrento S, Marques A, Teixeira B, Mendes R, Bandarra NM, Vaz-Pires P, and Nunes ML . 2010. Chemical composition, cholesterol, fatty acid and amino acid in two populations of brown crab Cancer pagurus: ecological and human health implications. Journal of Food Composition and Analysis 23:716–725.
- Chen K, Li EC, Xu ZX, Li TY, Xu C, Qin JG et al. 2015. Comparative transcriptome analysis in the hepatopancreas tissue of Pacific white shrimp Litopenaeus vannamei fed different lipid sources at low salinity. PLoS One 10(12):e0144889. DOI: 10.1371/journal.pone.0144889.
- Dorn N, Volin JC. 2009. Resistance of crayfish (Procambarus spp.) populations to wetland. Journal of the North American Benthological Society 28:766–777. DOI: 10.1899/08-151.1.
- Gebauer SK, Psota TL, Harris WS, Kris-Etherton PM. 2006. n−3 fatty acid dietary recommendations and food sources to achieve essentiality and cardiovascular benefits. The American Journal of Clinical Nutrition 83:1526S–1535S. DOI: 10.1093/ajcn/83.6.1526S.
- Gherardi F. 2007. Understanding the impact of invasive crayfish. In: Gherardi F, editor. Biological invaders in inland waters: Profiles, distribution, and threats. Dordrecht: Springer. pp. 507–542.
- Gherardi F, Barbaresi S. 2007. Feeding preferences of the invasive crayfish, Procambarus clarkii. Bulletin Français de la Pêche et de la Pisciculture 385:07–20. DOI: 10.1051/kmae:2007014.
- Gherardi F, Raddi A, Barbaresi S, Salvi G. 1999. Life history patterns of the red swamp crayfish, Procambarus clarkii, in an irrigation ditch in Tuscany. Crustacean Issues 12:99–108.
- Gonzalez-Felix ML, Gatlin Iii D, Lawrence A, and Perez‐Velazquez M. 2003. Nutritional evaluation of fatty acids for the open thelycum shrimp, Litopenaeus vannamei: II. Effect of dietary n‐3 and n‐6 polyunsaturated and highly unsaturated fatty acids on juvenile shrimp growth, survival, and fatty acid composition. Aquaculture Nutrition 9(2):115–122.
- Gutiérrez-Yurrita PJ, Sancho G, Bravo MÁ, Baltanás Á, Montes C. 1998. Diet of the red swamp crayfish Procambarus clarkii in natural ecosystems of the donana national park temporary fresh-water marsh (Spain). Journal of Crustacean Biology 18:120–127. DOI: 10.2307/1549526.
- Igarashi M, Tsuzuki T, Kambe T, Miyazawa T. 2004. Recommended methods of fatty acid methylester preparation for conjugated dienes and trienes in food and biological samples. Journal of Nutritional Science and Vitaminology 50(2):121–128. DOI: 10.3177/jnsv.50.121.
- Jimenez S, Faulkes Z. 2009. Establishment of a research colony of Marmorkrebs, a parthenogenetic crayfish species. Integrative and Comparative Biology 49:e249.
- Jin S, Jacquin L, Xiong M, Li R, Lek S, Li W, Zhang T. 2019. Reproductive pattern and population dynamics of commercial red swamp crayfish (Procambarus clarkii) from China: Implications for sustainable aquaculture management. PeerJ 7:e6214. DOI: 10.7717/peerj.6214.
- Jurmalietis R, Grickus A, Elstina A. 2019. Marbled crayfish (Procambarus virginalis) as a promising object for aquaculture industry. Environmental Technology Resources, Proceedings of the 12th International Scientific and Practical Conference 1:92–95.
- Kaldre K, Meženin A, Tiit P, and Kawai T. 2015. A preliminary study on the tolerance of marble crayfish Procambarus fallax f. virginalis to low temperature in nordic climate. In: Kawai T, Faulkes Z, and Scholtz G, editors. Freshwater crayfish: Global overview. CRC Press. pp. 54–62.
- Kaliszewicz A, Jarząbek K, Szymańska J, Karaban K, and Sierakowski M. 2018. Alpha-Linolenic acid, but not palmitic acid, negatively impacts survival, asexual reproductive rate, and clonal offspring size in Hydra oligactis. Lipids 53(4):447–456. DOI:10.1002/lipd.12026
- Kozák P, Duris Z, Petrusek A et al. 2015. Crayfish biology and culture. Ceske Budejovice: University of South Bohemia. pp 456.
- Li JY, Guo ZL, Gan XH, Wang DL, Zhang MF, Zhao YL. 2011. Effect of different dietary lipid sources on growth and gonad maturation of pre-adult female Cherax quadricarinatus (von Martens). Aquaculture Nutrition 17:e853–e860. DOI: 10.1111/j.1365-2095.2011.00852.x.
- Lim C, Ako H, Brown CL, Hahn K. 1997. Growth response and fatty acid composition of juvenile Penaeus vannamei fed different sources of dietary lipid. Aquaculture 151:143–153. DOI: 10.1016/S0044-8486(96)01500-1.
- Lyko F. 2017. The marbled crayfish (Decapoda: Cambaridae) represents an independent new species. Zootaxa 4363:544–552. DOI: 10.11646/zootaxa.4363.4.6.
- Martin P, Thonagel S, Scholtz G. 2016. The parthenogenetic Marmorkrebs (Malacostraca: Decapoda: Cambaridae) is a triploid organism. Journal of Zoological Systematics and Evolutionary Research 54(1):13–21. DOI: 10.1111/jzs.12114.
- Mazurska K, Baranowski A, Ceryngier P, Cygańska A, Fuszara M, Hayatli F, Jarska A, Kaźmierska A, Koperski P, Ptaszyński M, Romanowski J, Rozwałka R, Sikorski P, Skłodowski M, Strużyński W, Zięba G. 2019. Raport z inwentaryzacji bioblitz przeprowadzonej na terenie parku Morskie Oko w Warszawie. Zarząd Zieleni m. st. Warszawy (in Polish). Available: http://www.zzw.waw.pl
- Mourente G, Rodríguez A. 1997. Effects of salinity and dietary DHA (22:6 n - 3) content on lipid composition and performance of Penaeus kerathurus postlarvae. Marine Biology 128:289–298. DOI: 10.1007/s002270050094.
- Nagy R, Fusaro A, Conard W, Morningstar C. 2019. Procambarus clarkii (Girard, 1852). Gainesville, FL: U.S. Geological Survey, Nonindigenous Aquatic Species Database. Available: https://nas.er.usgs
- Okajima H, Ishii K, Watanabe H. 1984. Studies on lipids of crayfish, Procambarus clarkii. I. Furanoid fatty acids. Chemical and Pharmaceutical Bulletin 32:3281–3286. DOI: 10.1248/cpb.32.3281.
- Parakarma MGIS, Rawat KD, Venkateshwarlu G, Reddy AK. 2009. Feeding vitamins, antioxidants and cod liver oil enriched formulated feed influences the growth, survival and fatty acid composition of Macrobrachium rosenbergii (de Man, 1879) postlarvae. Sri Lanka Journal of Aquatic Sciences 14:59–74. DOI: 10.4038/sljas.v14i0.2200.
- Pérez-Bote JL. 2004. Feeding ecology of the exotic red swamp crayfish, Procambarus clarkii (Girard, 1852) in the Guadiana River (SW Iberian Peninsula). Crustaceana 77:1375–1387.
- Scholtz G, Braband A, Tolley L, Reimann A, Mittmann B, Lukhaup C, Steuerwald F, Vogt G. 2003. Parthenogenesis in an outsider crayfish. Nature 421:806. DOI: 10.1038/421806a.
- Sprecher H. 2000. Metabolism of highly unsaturated n-3 and n-6 fatty acids. Biochimica et Biophysica Acta (BBA). BBA Molecular and Cell Biology of L 1486:219–231.
- Suárez-Serrano A, Alcaraz C, Ibáñez C, Barata C. 2010. Procambarus clarkii as a bioindicator of heavy metal pollution sources in the lower Ebro River and Delta. Ecotoxicology and Environmental Safety 73:280–286. DOI: 10.1016/j.ecoenv.2009.11.001.
- Takeuchi T, Murakami K. 2007. Crustacean nutrition and larval feed, with emphasis on Japanese spiny lobster, Panulirus japonicus. Bulletin of Fisheries Research Agency 20:15–23.
- Taylor CA, Schuster GA, Cooper JE, DiStefano RJ, Eversole AG, Hamr P, Hobbs III HH, Robison HW, Skelton CE, Thoma RF. 2007. A reassessment of the conservation status of crayfishes of the United States and Canada after 10+ years of increased awareness. Fisheries 32:372–389. DOI: 10.1577/1548-8446(2007)32[372:AROTCS]2.0.CO;2.
- Thompson KR, Bailey TJ, Metts LS, Brady Y. 2010. Growth response and fatty acid composition of juvenile red claw crayfish (Cherax quadricarinatus) fed different sources of dietary lipid. Aquaculture Nutrition 16:604–615. DOI: 10.1111/j.1365-2095.2009.00697.x.
- Valipour A, Shariatmadari F, Abedian A, Seyfabadi SJ, Zahmatkesh A. 2011. Growth, molting and survival response of juvenile narrow clawed crayfish, Astacus leptodactylus, fed two sources of dietary oils. Iranian Journal of Fisheries Sciences 10:505–518.
- Wickins JF, O’C Lee D. 2002. Crustacean farming: Ranching and culture. London: Blackwell Science.
- Xu X, Ji W, Castell JD, O’Dor R. 1993. The nutritional value of dietary n-3 and n-6 fatty acids for the Chinese prawn (Penaeus chinensis). Aquaculture 118:277–285. DOI: 10.1016/0044-8486(93)90462-8.
- Zaglol NF, Eltadawy F. 2009. Study on chemical quality and nutritional value of fresh water crayfish (Procambarus clarkii). Journal of the Arabian Aquaculture Society 4:1–18.