Abstract
Nematodes represent the most abundant benthic metazoan of all seas and oceans, and their relative importance increases with increasing water depth. Understanding the biodiversity patterns of this dominant phylum could be a critical step towards our comprehension of the evolutionary patterns across the largest biome of the biosphere. For instance, it has been assumed for a long time that nematodes are ubiquitous across depths, latitudes and biogeographic regions, but there is still little scientific evidence for this lack of endemism. The present study is based on a meta-analysis of nematode biodiversity data collected from 246 deep-sea sites of the Atlantic Ocean and Mediterranean Sea. We explored the cosmopolitanism, rareness and potential endemism of nematode genera in deep-sea sediments. The results of this analysis indicate that only one-third of nematode families are widely distributed and could potentially be cosmopolitan, whereas 94% of the nematode genera are linked to specific habitats or bathymetric ranges. Singleton nematode genera (i.e. genera presenting as a single individual only in one specific habitat) increased in importance with increasing water depth. We conclude that rareness and endemism may be a far more common feature than previously thought in deep-sea nematode assemblages and hypothesise that the deep ocean interior could be a huge reservoir of endemic nematode species.
Introduction
The deep sea covers nearly 95% of the world ocean seafloor and of the oceans’ volume (Danovaro et al. Citation2020). The deep seafloor (> 200 m depth), the largest biome of the biosphere with ca 350 million of km2 (vs ca 150 million of km2 of terrestrial systems), is likely to host a substantial proportion of the oceans’ and Earth’s biodiversity (Costello & Chaudhary Citation2017). Nematodes represent the most abundant metazoan phylum in deep-sea sediments, comprising typically >90% of all benthic meiofauna (Appeltans et al. Citation2012; Danovaro et al. Citation2014). They are considered important indicators of habitat heterogeneity in marine environments since they are common, numerous and speciose, and in close contact with seafloor-related processes (Miljutin et al. Citation2010; Vanreusel et al. Citation2010). Amongst meiofaunal organisms, nematodes are expected to have a high species richness, with an estimated ca 50,000 to >100,000 species (based on expert evaluation; Appeltans et al. Citation2012) and 86% of the existing species remaining to be discovered. Moreover, it has been estimated that field surveys have so far described only 6–56% of the nematode diversity present (Lee et al. Citation2017).
We also lack an accurate analysis of the nematode species diversity and phylogeographic patterns, and this makes it difficult to provide accurate estimates of the global nematode diversity. Many deep-sea genera are widespread and hypothesised to be cosmopolitan in their occurrence (Coomans Citation2000; Bhadury et al. Citation2008; Derycke et al. Citation2008; Bik et al. Citation2010), which is surprising given that benthic meiofaunal organisms typically do not have planktonic larval stages (Bhadury et al. Citation2008). On one hand, the small size and the low potential mobility should limit the dispersal abilities of the nematodes (Giere Citation2009), but on the other hand these animals can be resuspended in the water column and potentially transported over wide spatial scales, as evidenced by their recovery from sediment traps located more than 500 m above the seafloor (Danovaro et al. Citation2017).
The limited information on nematode species distribution over large spatial scales makes it difficult to ascertain whether deep-sea nematodes possess large or limited dispersal capabilities (Guilini et al. Citation2011). As a result, we currently do not understand what proportion of the deep-sea nematode species is cosmopolitan, or rare, or endemic to a specific habitat (e.g. hydrothermal vents, anoxic zones, cold seeps, etc.).
Although we have now censused ca 15,000 free-living marine nematode species (Costello & Chaudhary Citation2017), it has been hypothesised that this phylum is hyper-diverse or constituted only by a relatively limited number of cosmopolitan genera/species (Miljutin et al. Citation2010). We do not even know if deep-sea species originally represented invasions from shallower habitats or developed “endemic” lineages that over time expanded at shallower depths (Bik et al. Citation2010).
The present study is based on a meta-analysis of the biodiversity of deep-sea nematodes in a wide range of biogeographic regions and latitudes and over a wide range of water depths. The study aims to elucidate the biogeographic and bathymetric patterns of deep-sea nematode families/genera to identify the cosmopolitan genera, and those that are abundant, rare, or endemic to specific habitats. This information is crucially important for quantifying the global nematode biodiversity and thus the global metazoan biodiversity.
Material and methods
We report here the results of a large data set produced from the analysis of 82 deep-sea sites. We compiled an inventory of 246 data sets of nematode biodiversity (identified to the genus level) spanning from the Atlantic Ocean to the Mediterranean Sea (). Our database covers latitudes from 34 to 57°N and depths from approximately 200 m to more than 4700 m (information for each data set is provided in Table S1).
Figure 1. Sampling areas along the European margins. Overall, 246 sites (depth range: 200–4700 m) were sampled along 12 open slopes, nine canyons (Whittard, Cascais, Nazarè, Cap de Creus/Sete, Lacaze-Duthiers, two in the South Adriatic and one in Cretan margin) and one deep-water coral bank.
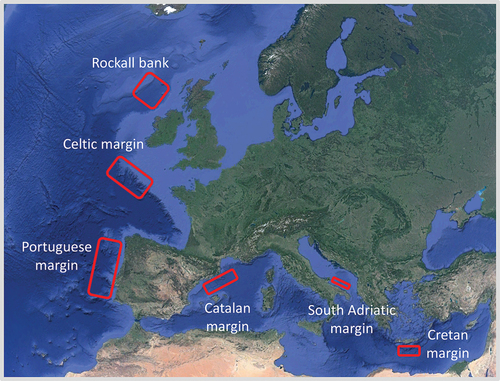
Sampling was carried out in the north and central Atlantic Ocean (31 sites), and the western, central and eastern Mediterranean Sea (51 sites). The investigated areas included only open-ocean sites, continental-margin systems and specific hotspot ecosystems (i.e. deep-water coral sites, canyons and slopes). The sampling design and techniques were consistent across areas. Sediment samples were collected from all areas at standard water depths, along the main axis of the canyons and the adjacent open slopes at standard depths (i.e. 200, 500, 1000, 2000, 3000, 4000 and 5000 m depth, according to the bottom depths of each region; Danovaro et al. Citation2009a, Citation2009b, Bongiorni et al. Citation2010; Gambi & Danovaro Citation2016). Overall, ~32% of the sampling sites were located at depths from 200 to 500 m, ~33% from 501 to 1000 m, ~21% from 1001 to 2000 m, ~21% from 2001 to 3000 m, ~9% from 3001 to 4000 m and ~6% at >4001 m. Sediment samples were collected using a multiple corer and/or a NIOZ-type box corer, which allows the recovery of virtually undisturbed sediment samples. The two sampling devices proved to be equivalent in the sampling of biotic variables (Danovaro et al. Citation1998). At all sampling stations, 3–5 sediment cores (internal diameter 3.6 cm) from the independent deployments (whenever possible) were analysed for nematode diversity (0 to 1 cm).
All collected samples are methodologically homogeneous and consistent, as nematode genera and families were obtained using the same standardised protocols (Danovaro et al. Citation2009a), taxonomic experts and references (Danovaro et al. Citation2009a, 2009b, Bongiorni et al. Citation2010; Gambi & Danovaro Citation2016).
Benthic biodiversity
For meiofaunal extraction, sediment samples were sieved through 1000 μm mesh, and a 20 μm mesh was used to retain the smallest organisms. The fraction remaining on the latter sieve was resuspended and centrifuged 3 times with Ludox HS40 silica (density 1.31 g cm−3) according to Heip et al. (Citation1985). All meiobenthic animals were counted under a stereomicroscope and classified per higher taxon after staining with Rose Bengal (0.5 g L−1). All animals except for nematodes were identified to higher taxon, and these data are reported elsewhere (Danovaro et al. Citation2009a, Citation2009b; Bongiorni et al. Citation2010; Gambi & Danovaro Citation2016). All the nematodes were identified to the family and genus level, according to Platt and Warwick (Citation1983, Citation1988), Warwick et al. (Citation1998) and all genera were compared to the NeMys database to ensure consistency (Nemys Citation2022). Because most indices of species diversity are sample-size dependent, the rarefaction method was applied so that all samples could be reduced to the same size, with EG(100) as the expected number of genera in a hypothetical random sample of 100 individuals. Previous studies have shown that this approach enables the provision of robust data on species richness in the deep sea and the expected species number is the best density-independent index for the comparison of areas with a no standardised sampling size and effort (Danovaro et al. Citation2009a).
Statistical analyses
To assess separately the differences in nematode composition among sampling regions and habitats at similar depth intervals (500, 1000 and 2000 m) we employed two-way distance-based permutational analysis of variance (PERMANOVA) using unrestricted permutations of the raw data. The analyses were carried out using region (north and central Atlantic; western, central and eastern Mediterranean) and habitat (slope, canyon and deep-water corals) fixed levels as the main sources of variance and on Bray–Curtis similarity matrices of square-root-transformed data using 999 permutations of the residuals under a reduced model. For data obtained from samples collected at 3000 and >4000 m depth intervals, we employed one-way distance-based permutational analyses of variance using unrestricted permutations of the raw data. The analyses were carried out using region (north and central Atlantic and eastern Mediterranean) fixed level as the main source of variance and on Bray–Curtis similarity matrices of square-root-transformed data using 999 permutations of the residuals under a reduced model.
For PERMANOVA tests indicating significant differences among regions and habitats, pairwise tests were also carried out. Because of the restricted number of unique permutations in the pairwise tests, p values were obtained from Monte Carlo samplings (Anderson & Robinson Citation2003).
SIMPER analyses were performed to assess the percentage of dissimilarity in the nematode community composition among regions and habitats at similar depth intervals. A ranked matrix of Bray–Curtis similarities, constructed on previously square-root-transformed data, was used as input for the SIMPER tests. The nematode community composition among regions and habitats at similar depth intervals was also used as inputs for the shade plots.
The similarity matrix of nematode composition, based on the Bray–Curtis similarity, was applied to produce a non-metric multidimensional scaling two-dimensional plot (MDS) for each depth intervals. The PERMANOVA, SIMPER and MDS analyses and shade plots were carried out using the routines included in the PRIMER v. 7 and PERMANOVA software (Clarke & Gorley Citation2015; Anderson Citation2017).
Results
Overall, in the present study we elaborated the data from ca 19,000 nematodes, belonging to 41 families and 199 genera. In the Atlantic Ocean, 38 families were reported; among them, Xyalidae, Chromadoridae, Cyatholaimidae and Comesomatidae were the most abundant, with 19, 17, 12 and 10 genera, respectively (). In the Mediterranean Sea, 36 families were identified; among them, Comesomatidae, Xyalidae, Cyatholaimidae and Chromadoridae were characterised by the presence of more than 10 genera (14, 14, 12 and 11 genera, respectively, ). In both macro-regions, some nematode families were present with a single genus while others were completely absent (Haliplectidae, Pandolaimidae and Siphonolaimidae for the Atlantic Ocean and Camacolaimidae, Epsilonematidae, Monoposthiidae, Peresianidae and Tripyloididae for the Mediterranean Sea).
Figure 2. List of all nematode families identified in (a) the Atlantic Ocean and (b) the Mediterranean Sea. The number of genera belonging to each family is also reported.
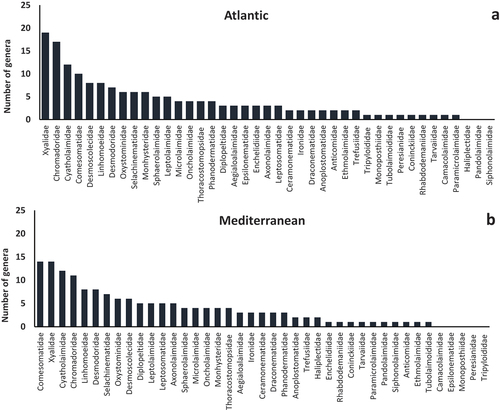
The PERMANOVA analyses reveal that nematode genus composition changed significantly among regions at all selected depths (). In detail, changes in nematode genus composition occurred between slopes and canyons in both the Atlantic Ocean and the Mediterranean Sea, between slopes and deep-water corals in the Rockall bank and between slopes and canyons in the Catalan margin at 500 m (). At 1000 m depth nematode genus composition changed significantly in the Atlantic Ocean and in the Mediterranean Sea among the slope habitats, while for canyons differences occurred between regions (Atlantic vs Mediterranean) but not among the western, central, and eastern basin within the Mediterranean Sea. Nematode genus composition changed between canyon and slope in the North Atlantic, and in the western and eastern Mediterranean. At 2000 m depth, nematode composition changed significantly between regions and within each region for both slope and canyon habitats, while at the deepest sites (3000 and 4000 m), the nematode genus composition changed significantly among regions both in the Atlantic Ocean and in the Mediterranean Sea ().
Table I. Output of the PERMANOVA analysis carried out to test for differences in nematode genus composition among regions and habitats (df = degrees of freedom; MS = mean square; Pseudo-F = F statistic; P(MC) = probability level); *** = P < .001; * = P < .05. ns: not significant. N Atl (North Atlantic including Rockall bank and Celtic margin), C Atl (central Atlantic: Portuguese margin), W Med (western Mediterranean: Catalan margin), C Med (central Mediterranean: South Adriatic margin) and E Med (eastern Mediterranean: Cretan margin)
The output of the MDS analyses revealed that nematode genus composition clustered in different regions and habitats both in the Atlantic Ocean and in the Mediterranean Sea, at all selected depth intervals ().
Figure 3. Multidimensional scaling analysis carried out on the genus composition of nematodes collected in the six European margins and different habitats (slope, canyon and deep-water corals) at different depth intervals: 500, 1000, 2000, 3000 and >4000 m.
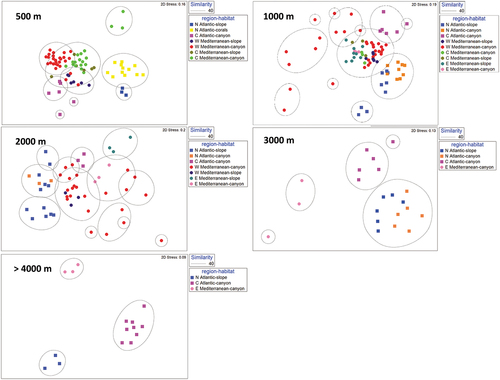
The expected genus number [EG(100)] ranged from 23 (canyons of the Portuguese margin) to 37 (slope of the South Adriatic margin) at 500 m depth, from 23 (canyons of the Portuguese margin) to 36 (canyon of Bay of Biscay) at 1000 m depth, from 16 (slope and canyon of the Cretan margin) to 29 (Catalan margin) at 2000 m depth, from 14 (canyon of the Cretan margin) to 27 (slope of the Bay of Biscay) at 3000 m depth, and from 21 (canyon of the Cretan margin) to 33 (slope of the Bay of Biscay) at >4000 m depth ().
Figure 4. Nematode diversity in different habitats and within regions at different depths. The expected genus number [EG(100)] is reported.
![Figure 4. Nematode diversity in different habitats and within regions at different depths. The expected genus number [EG(100)] is reported.](/cms/asset/6aaed796-5b95-4d7e-a0e9-057faf140136/tizo_a_2040621_f0004_oc.jpg)
The results of the SIMPER analyses revealed that the genus turnover was generally higher than 50% comparing different regions within the same habitat (canyon vs slope) and different habitats (deep-water corals, canyons, and slopes) within the same region (). The genus turnover was 58–78% at 500 m depth, 48–76% at 1000 m depth, 55–85% at 2000 m depth, 59–89% at 3000 m depth and 65% at more than 4000 m depth.
Table II. Turnover diversity among nematode assemblages’ composition in different habitats within the same region and between regions within slope and canyon. The turnover diversity was obtained from the SIMPER analysis
The shade plots () provide an image of the abundance of overall nematode genera composition in each habitat and region at the different depth intervals, suggesting the different patterns of genus abundance and spatial distribution.
Discussion
A cosmopolitan distribution has been proposed for some meiofaunal taxa (e.g. free-living marine nematodes), for which a transoceanic distribution has been observed. Our results support this hypothesis, as deep-sea environments provide relatively limited barriers to dispersal (van der Heijden et al. Citation2012). The large connectivity among macro-regions is invoked to explain the nematode cosmopolitanism (Strugnell et al. Citation2008; Bik et al. Citation2010; van der Heijden et al. Citation2012).
In contrast to previous large-scale analyses of deep-sea nematode biodiversity (Miljutin et al. Citation2010; Vanreusel et al. Citation2010), our study reveals that only 12 of the 41 families identified and 12 of the 199 genera identified showed a wide distribution in all investigated regions from the Atlantic Ocean to the Mediterranean. These results indicate that only ca 6% of nematode genera in deep-sea sediments show a high dispersal ability and a widespread distribution (Tietjen Citation1989; Bik et al. Citation2010; Zeppilli et al. Citation2011). The cosmopolitan genera include Halalaimus, Sabatieria, Pierrickia, Acantholaimus and Sphaerolaimus (all typically >5% of the total nematode abundance), which are known to be common along all the continental margins investigated (Vanreusel et al. Citation2010). Some of the cosmopolitan genera, such as Oxystomina, Pselionema and Campylaimus, are widespread but with very low abundance (typically <1% of the overall nematode abundance; Bianchelli et al. Citation2010). These genera are also characterised by a low number of species, as documented in deep-sea sediments at a global scale (Miljutin et al. Citation2010).
The analysis of nematode biodiversity in different macro-regions, habitats and depths also revealed the presence of a large amount of potential endemism, as evident from the high number of singletons (overall, 17 genera: 8% of the total). The number of endemic genera increases with increasing depth (from 13% singletons at 1000 m to 25% singletons at depths >4000 m),suggesting that depth could play a role in selecting specific nematode taxa and, consequently, in nematode biodiversity distribution at different spatial scales (Heip et al. Citation1985; Vincx et al. Citation1994; Moens et al. Citation2014). At the same time, we cannot discount the importance of the effect of sampling intensity (higher at shallower depths and lower at deeper depths) on the overall census of biodiversity.
Only eight families and 17 genera were associated with a single habitat type: 11 genera were exclusively encountered on continental slopes and six genera were exclusively encountered in canyons. The high number of singletons along slopes reveals the preeminent role of these habitats in contributing to the biodiversity of deeper basins and shelves through radiation and dispersal processes closely coupled with topographic and physical characteristics of the deep sea (Danovaro et al. Citation2009b). The fact that there are nematode genera exclusively associated with these habitats may be the result of two factors: (1) these specific deep-sea environments promote a higher speciation rate or the coexistence of more species (sensu Myers et al. Citation2000), and/or (2) the preference of some taxa for specific environmental features in the deep sea.
Submarine canyons, open slopes, deep-water coral systems, pockmarks, hydrothermal vents, and other complex topographic and hydrodynamic features can promote cosmopolitanism, rareness and endemism in deep-sea marine nematodes (Canals et al. Citation2009, Citation2013; De Leo et al. Citation2010; Danovaro et al. Citation2014). The low number of genera and singletons reported along canyons could be related to the lower diversity generally observed in this habitat in all regions except for the Catalan margins (where a large part of the data set came from), suggesting that the occurrence of multiple processes (episodic events, current fluxes, local hydrography and heterogeneity) can drive the overall diversity.
Approximately 10% of the nematode genera were rare and exclusively present in canyons, suggesting their ability to colonise these highly dynamic systems with few specimens. This pattern has been reported in other canyons throughout the European seas (Vetter & Dayton Citation1998; Curdia et al. Citation2004; Danovaro et al. Citation2009a; Duros et al. Citation2011; Romano et al. Citation2013; Leduc et al. Citation2014; Ramalho et al. Citation2014; Gunton et al. Citation2015; Gambi & Danovaro Citation2016). Although we cannot exclude the effect of a lower number of samples taken at deeper depths, our data, based on a standardised number of specimens per sample (n = 300), suggest that this pattern could indicate a specific feature of deep-sea ecosystems.
Deep-water coral ecosystems are hotspots of biodiversity, provide habitats and refuges for several deep-sea species and can contribute to shaping the biodiversity of the surrounding open slopes (Raes & Vanreusel Citation2006; Roberts et al. Citation2006; Bongiorni et al. Citation2010). We did not report any genera exclusively associated with deep-water corals, but four genera (Epsilonema, Glochinema, Gonionchus and Monoposthia) were found to be very rare and exclusively present in the Rockall bank corals and the adjacent slopes (North Atlantic). Among these genera, we find non-burrowing, interstitial or epifaunal forms belonging to epsilonematids that prefer the three-dimensional structure of deep-water corals (Vanreusel et al. Citation2010). Genera belonging to Epsilonematidae are characterised by the presence of unique locomotory structures based on the ambulatory setae on the ventral side of their posterior body that can facilitate their presence in these habitat types (Gourbault & Decraemer Citation1996; Decraemer et al. Citation1997).
Conclusions
Our large-scale investigation, covering a wide portion of the North Atlantic Ocean to the Mediterranean Sea, revealed that only approximately one-third of nematode families and 6% of their genera are widely distributed and can be considered potentially cosmopolitan. Conversely, 94% of the nematode genera were present in very low abundances in specific habitats or bathymetric ranges. Singleton nematode genera, which could indicate the presence of endemism, are common at all sampling sites and increased in importance with increasing water depth. We conclude that rareness and endemism may be a far more widespread feature than previously thought in deep-sea nematode assemblages.
Acknowledgements
This work has been conducted within the frame of the research activities financially supported by the Polytechnic University of Marche (Fondi di Ateneo).
Disclosure statement
No potential conflict of interest was reported by the authors.
Additional information
Funding
References
- Nemys. 2022. Nemys: World Database of Nematodes. https://nemys.ugent.be
- Anderson MT. 2017. Permutational multivariate analysis of variance (PERMANOVA). Wiley statsref: statistics reference online, 2014-2017. New York: John Wiley and Sons, Ltd. DOI:10.1002/9781118445112.stat07841.
- Anderson MJ, Robinson J. 2003. Generalized discriminant analysis based on distances. Australian New Zealand Journal of Statistics 45(3):301–318. DOI:10.1111/1467-842X.00285.
- Appeltans W, Ahyong ST, Anderson G, Angel MV, Artois T et al. 2012. The magnitude of global marine species diversity. Current Biology 22(23):2189–2202. DOI: 10.1016/j.cub.2012.09.036.
- Bhadury P, Austen MC, Bilton DT, Lambshead PJD, Rogers AD, Smerdon GR. 2008. Evaluation of combined morphological and molecular techniques for marine nematode (Terschellingia spp.) identification. Marine Biology 154(3):509–518. DOI:10.1007/s00227-008-0945-8.
- Bianchelli S, Gambi C, Zeppilli D, Danovaro R. 2010. Metazoan meiofauna in deep-sea canyons and adjacent open slopes: A large-scale comparison with focus on the rare taxa. Deep Sea Research Part I: Oceanographic Research Papers 57(3):420–433. DOI:10.1016/j.dsr.2009.12.001.
- Bik HM, Thomas WK, Lunt DH, Lambshead PJD. 2010. Low endemism, continued deep-shallow interchanges, and evidence for cosmopolitan distributions in free-living marine nematodes (order Enoplida). BMC Evolutionary Biology 10(1):389. DOI:10.1186/1471-2148-10-389.
- Bongiorni L, Mea M, Gambi C, Pusceddu A, Taviani M, Danovaro R. 2010. Deep-water scleractinian corals promote higher biodiversity in deep-sea meiofaunal assemblages along continental margins. Biological Conservation 143(7):1687–1700. DOI:10.1016/J.BIOCON.2010.04.009.
- Canals M, Company JB, Martín D, Sànchez-Vidal A, Ramírez-Llodra E. 2013. Integrated study of Mediterranean deep canyons: Novel results and future challenges. Progress in Oceanography 118:1–27. DOI: 10.1016/j.pocean.2013.09.004.
- Canals M, Danovaro R, Heussner S, Lykousis V, Puig P, Trincardi F, Calafat AM, Durrieude Madron X, Palanques A. 2009. Cascades in Mediterranean submarine grand canyons. Oceanography 22(1):26–43. DOI:10.5670/oceanog.2009.03.
- Clarke KR, Gorley RN. 2015. PRIMER V7: Getting started with PRIMER7. Plymouth, UK: PRIMER-E.
- Coomans A. 2000. Nematode systematics: Past, present and future. Nematology 2(1):3–7. DOI:10.1163/156854100508845.
- Costello MJ, Chaudhary C. 2017. Marine biodiversity, biogeography, deep-sea gradients, and conservation. Current Biology: CB 27(11):R511–R527. DOI:10.1016/j.cub.2017.04.060.
- Curdia J, Carvalho S, Ravara A, Gage JD, Rodrigues AM, Quintino V. 2004. Deep macrobenthic communities from Nazaré Submarine Canyon (NW Portugal). Scientia Marina 68(S1):171–180. DOI:10.3989/scimar.2004.68s1171.
- Danovaro R, Bianchelli S, Gambi C, Mea M, Zeppilli D. 2009a. a-, β-, γ-, δ- and ε-diversity of deep-sea nematodes in canyons and open slopes of Northeast Atlantic and Mediterranean margins. Marine Ecology Progress Series 396:197–209. DOI: 10.3354/meps08269.
- Danovaro R, Canals M, Gambi C, Heussner S, Lampadariou N, Vanreusel A. 2009b. Exploring benthic biodiversity patterns and hot spots on european margin slopes. Oceanography 22(1):16–25. https://doi.org/10.5670/oceanog.2009.02 .
- Danovaro R, Carugati L, Boldrin A, Calafat A, Canals M, Fabrese J, Finlay K, Heussner S, Miserocchi S, Sanchez-Vidal A. 2017. Deep-water zooplankton in the Mediterranean Sea: Results from a continuous, synchronous sampling over different regions using sediment traps. Deep Sea Research Part I: Oceanographic Research Papers 126:103–114. DOI: 10.1016/j.dsr.2017.06.002.
- Danovaro R, Fanelli E, Aguzzi J et al. 2020. Ecological variables for developing a global deep-ocean monitoring and conservation strategy. Nature Ecology & Evolution 4(2):181–192. DOI: 10.1038/s41559-019-1091-z.
- Danovaro R, Marrale D, Della Croce N, Dell’Anno A, Fabiano M. 1998. Heterotrophic nanoflagellates, bacteria, and labile organic compounds in continental shelf and deep-sea sediments of the eastern mediterranean. Microbial Ecology 35(3):244–255. DOI:10.1007/s002489900080.
- Danovaro R, Snelgrove PVR, Tyler P. 2014. Challenging the paradigms of deep-sea ecology. Trends in Ecology & Evolution 29(8):465–475 10.1016/j.tree.2014.06.002 PMID: 25001598
- De Leo FC, Smith CR, Rowden AA, Bowden DA, Clark MR. 2010. Submarine canyons: Hotspots of benthic biomass and productivity in the deep sea. Proceedings of the Royal Society B: Biological Sciences 277(1695):2783–2792. DOI:10.1098/rspb.2010.0462.
- Decraemer W, Gourbault N, Backeljau T. 1997. Marine nematodes of the family Draconematidae (Nemata): A synthesis with phylogenetic relationships. Hydrobiologia 357(1/3):185–202. DOI:10.1023/A:1003155424665.
- Derycke S, Remerie T, Backeljau T, Vierstraete A, Vanfleteren J, Vincx M, Moens T. 2008. Phylogeography of the Rhabditis (Pellioditis) marina species complex: Evidence for long-distance dispersal, and for range expansions and restricted gene flow in the northeast Atlantic. Molecular Ecology 17(14):3306–3322. DOI:10.1111/j.1365-294X.2008.03846.x.
- Duros P, Fontanier C, Metzger E, Pusceddu A, Cesbron F, de Stigter Hc, Bianchelli S, Danovaro R, Jorissen FJ. 2011. Live (stained) benthic foraminifera in the Whittard canyon, Celtic margin (NE Atlantic). Deep Sea Research Part I: Oceanographic Research Papers 58(2):128–146. DOI:10.1016/j.dsr.2010.11.008.
- Gambi C, Danovaro R. 2016. Biodiversity and life strategies of deep-sea meiofauna and nematode assemblages in the Whittard Canyon (Celtic margin, NE Atlantic Ocean). Deep Sea Research Part I: Oceanographic Research Papers 108:13–22. DOI: 10.1016/j.dsr.2015.12.001.
- Giere O. 2009. Meiobenthology. The Microscopic Motile Fauna of Aquatic Sediments. Berlin Heidelberg: Springer-Verlag. pp. 527.
- Gourbault N, Decraemer W. 1996. Marine nematodes of the family epsilonematidae: a synthesis with phylogenetic relationships. Nematologica 42(2):133–158. DOI:10.1163/004325996X00011.
- Guilini K, Soltwedel T, van Oevelen D, Vanreusel A. 2011. Deep-sea nematodes actively colonise sediments, irrespective of the presence of a pulse of organic matter: results from an in-situ experiment. PLoS ONE 6(4):e18912. https://doi.org/10.1371/journal.pone.0018912
- Gunton LM, Gooday AJ, Glover AG, Bett BJ. 2015. Macrofaunal abundance and community composition at lower bathyal depths in different branches of the Whittard Canyon and on the adjacent slope (3500 m; NE Atlantic). Deep Sea Research Part I: Oceanographic Research Papers 97:29–39. DOI: 10.1016/j.dsr.2014.11.010.
- Heip C, Vincx M, Vranken G. 1985. The ecology of marine nematodes. Oceanography and Marine Biology - an Annual Review 23:399–489.
- Leduc D, Rowden AA, Nodder SD, Berkenbusch K, Probert PK, Hadfield MG. 2014. Unusually high food availability in Kaikoura Canyon linked to distinct deep-sea nematode community. Deep Sea Research Part II: Topical Studies in Oceanography 104:310–318. DOI: 10.1016/j.dsr2.2013.06.003.
- Lee MR, Canales-Aguirre CB, Nuñez D, Pérez K, Hernández CE, Brante A. 2017. The identification of sympatric cryptic free-living nematode species in the Antarctic intertidal. PLoS ONE 12(10):e0186140. DOI:10.1371/journal.pone.0186140.
- Miljutin DM, Gad G, Miljutina MM, Mokievsky VO, Fonseca-Genevois V, Esteve AM. 2010. The state of knowledge on deep-sea nematode taxonomy: How many valid species are known down there? Marine Biodiversity 40(3):143–159. DOI:10.1007/s12526-010-0041-4.
- Moens T, Braeckman U, Derycke S, Fonseca G, Gallucci F, Gingold R. 2014. Ecology of free-living marine nematodes, and Schmidt-Rhaesa A et al.,editors. Handbook of Zoology. Hamburg, Germany: De Gruyter. pp.109–152
- Myers N, Mittermeier R, Mittermeier C et al. 2000. Biodiversity hotspots for conservation priorities. Nature 403(6772):853–858. DOI: 10.1038/35002501.
- Platt HM, Warwick RM. 1983. Free-living Marine Nematodes. Part I. In: British Enoploids. Synopses of the British Fauna, 307. Cambridge: Linnean Society of London and the Estuarine and Brackish-Water Sciences Association by Cambridge University Press.
- Platt HM, Warwick RM. 1988. Free-living marine nematodes. Part II British chromadorids. Synopses of the British Fauna, 502. Cambridge: Linnean Society of London and the Estuarine and Brackish-Water Sciences Association by Cambridge University Press.
- Raes M, Vanreusel A. 2006. Microhabitat type determines the composition of nematode communities associated with sediment-clogged cold-water coral framework in the Porcupine Seabight (NE Atlantic). Deep Sea Research Part I: Oceanographic Research Papers 53(12):1880–1894. DOI:10.1016/j.dsr.2006.08.012.
- Ramalho SP, Adão H, Kiriakoulakis K, Wolff GA, Vanreusel A, Ingels J. 2014. Temporal and spatial variation in the Nazaré Canyon (Western Iberian margin): Inter-annual and canyon heterogeneity effects on meiofauna biomass and diversity. Deep Sea Research Part I: Oceanographic Research Papers 83:102–114. DOI: 10.1016/j.dsr.2013.09.010.
- Roberts JM, Wheeler AJ, Freiwald A. 2006. Reefs of the deep: The biology and geology of cold-water coral ecosystems. Science 312(5773):543–547. DOI:10.1126/science.1119861.
- Romano C, Coenjaerts J, Flexas MM, Zúñiga D, Vanreusel A, Company JB, Martin D. 2013. Spatial and temporal variability of meiobenthic density in the Blanes submarine canyon (NW Mediterranean). Progress in Oceanography 118(118):144–158. DOI:10.1016/j.pocean.2013.07.026.
- Strugnell MJ, Rogers AD, Prodöhl PA, Collins MA, Allcock AL 2008. The thermohaline expressway: The Southern Ocean as a centre of origin for deep-sea octopuses. Cladistics 24(6):853–860. DOI:10.1111/j.1096-0031.2008.00234.x
- Tietjen JH. 1989. Ecology of deep-sea nematodes from the Puerto Rico trench area and Hatteras Abyssal plain. Deep Sea Research Part A. Oceanographic Research Papers 36(10):1579–1594. DOI:10.1016/0198-0149(89)90059-9.
- van der Heijden K, Petersen JM, Dubilier N, Borowski C. 2012. Genetic connectivity between north and south mid-atlantic ridge chemosynthetic bivalves and their symbionts. PLoS One 7(7):e39994. DOI:10.1371/journal.pone.0039994.
- Vanreusel A, Fonseca G, Danovaro R, da Silva Mc, Esteves AM, Ferrero T, Gad G, Galtsova V, Gambi C, Genevois V, Ingels J, Ingole B, Lampadariou N, Merckx B, Miljutin DM, Miljutina MM, Muthumbi A, Netto S, Portnova D, Radziejewska T, Raes M, Tchesunov A, Vanaverbeke J, Van Gaever S, Venekey V, Bezerra T, Flint H, Copley J, Pape E, Zeppilli D, Martinez P, Galeron J. 2010. The contribution of deep-sea macrohabitat heterogeneity to global nematode diversity. Marine Ecology 31(1):6–20. DOI:10.1111/j.1439-0485.2009.00352.x.
- Vetter EW, Dayton PK. 1998. Macrofaunal communities within and adjacent to a detritus-rich submarine canyon system. Deep Sea Research Part II: Topical Studies in Oceanography 45(1–3):25–54. DOI:10.1016/S0967-0645(97)00048-9.
- Vincx M, Bett BJ, Dinet A, Ferrero T, Gooday AJ, Lambshead PJD, Pfannkuche O, Soltwedel T, Vanreusel A. 1994. Meiobenthos of the deep NE Atlantic. Advances in Marine Biology 30:1–88.
- Warwick RM, Platt HM, Somerfield PJ 1998. Free-living marine nematodes. Part III. Monhysterids. In: Synopses of the British Fauna, vol. 53, 296. Field Studies Council, Shrewsbury.
- Zeppilli D, Vanreusel A, Danovaro R. 2011. Cosmopolitanism and Biogeography of the Genus Manganonema (Nematoda: Monhysterida) in the Deep Sea. Animals 1(3):291–305. DOI:10.3390/ani1030291.