Abstract
Forests are important habitats that harbor high biodiversity and have great economic value to humans. These ecosystems depend on saproxylic organisms, which play a crucial role in the decomposition of the main substrate of forests – wood. The highly important group of saproxylic organisms are beetles, with numerous species that are either of conservation concern (rare and threatened) or have economic value (“pests”). This review summarizes what is known about intra- and interspecific genetic information on species or populations of saproxylic beetles. A search of the literature yielded 110 articles published in 57 journals. The majority of molecular research has focused on “pests” (82 articles on 59 species), with fewer studies of the “non-pest” beetles (28 articles on 40 species, including 10 relicts of primeval forests). Most studies concerned Palearctic and Nearctic taxa, with only a few articles on beetles from (sub)tropical forests. Members of 11 families, 38 genera and 99 species were the subject of molecular studies. The majority of studies (71%) were conducted on beetles associated with conifers. Genetic metrics were found to have different overall values in “pests” and the “non-pest” species. Analyzes resulted in identification of units or either conservation (15 cases) or taxonomic (33) value, the discovery of hybridization (4), invasion routes, and common phylogeographic patterns with the localization of glacial refugial areas. This article is the first comprehensive summary of what is known about the history, present-day diversity, and relationships of highly important group of saproxylic beetles based on molecular studies. “Pests” found to be much wider studied than threatened taxa. A list of species that should be the subject of future molecular studies was provided along with a rationale. Finally, methodological and objective deficiencies in previous studies were characterized.
Introduction
Among forest-dwelling organisms, a remarkable high number of species is closely associated with woody parts of trees. Some of these species use living trees (Lieutier et al. Citation2004), but many of them are found only in dying trees or in the wood of dead trees (colloquially called “deadwood”). This group of organisms (hereafter referred to as saproxylic) (Siitonen Citation2001) has been neglected for many years in forest ecosystems. Their role in ecological processes in forests was finally noticed, leading to an increasing number of scientific studies focusing on various aspects of their biology, evolution, diversity and ecology (Kozák et al. Citation2021). Saproxylic organisms are crucial in natural forest ecosystems as they play many roles in different trophic levels (sapro-, xylo-, cambio- or mycetophages and predators) and are thus fundamental for forest functioning (Hjältén et al. Citation2012). In this respect, detritivores are particularly important as they depend on and are responsible for the decomposition of the most abundant substrate in this habitat, namely wood. Saproxylic organisms have received special attention from biologists and nature conservationists (Mason & Zapponi Citation2015), which has led to the protection of many rare, endangered or threatened species and the designation of numerous protected areas in forests of different areas and management regimes.
Division of saproxylic species into two opposing groups may be proposed. The first group includes rare, threatened, and often protected species, and is therefore of less concern to foresters, but may well conflict with forestry activities. Conservation of saproxylic organisms usually requires the designation of protected areas, or at least substantial changes in forest management practices that result in reduced logging and the preservation of large numbers of dying trees and large amounts of deadwood in forests (Davies et al. Citation2008). Such measures are usually at odds with the needs of timber production (Grove Citation2002). Furthermore, these conservation measures are not always properly implemented, which can be easily seen by the distribution of rare and threatened deadwood-related species, which are usually absent from managed forest stands and “pushed back” into protected areas. The second group of saproxylic organisms of great concern in forestry are species colloquially referred to as “pests” (De Groot et al. Citation2019). These species are generally considered harmful to trees, as their outbreaks lead to weakening of trees, development of diseases in trees (e.g. fungal infections), usually resulting in death of infested trees and/or loss of large areas of forest. This is one of the most serious problems in timber production and the reason for many studies aimed at finding methods to prevent or mitigate such outbreaks (Klapwijk et al. Citation2016). Forest management neglects the natural role of these common saproxylic species in forest ecosystems. These organisms are natural elements in the life of trees (Müller et al. Citation2008).
The best-known group of saproxylic organisms are the beetles (Coleoptera). Approximately 400,000 species are known in this insect order and 50–60% of them are thought to live in the trunks or roots of trees, with many species relying on dying trees or deadwood (Ślipiński et al. Citation2011; Gimmel & Ferro Citation2018). Saproxylic beetles are a focus of attention for biologists, conservationists and foresters due to their great diversity, abundance (in the case of common species) or rarity (in the case of threatened taxa), charismatic features (e.g. morphology, colors), and their role in forest ecosystems (either as flagship/umbrella/keystone species for forest ecosystems or as “pests” in timber production) (Dajoz Citation2000; Stokland et al. Citation2012). Saproxylic beetles are common in all types of forests and woodlands worldwide (Grove Citation2002; Gimmel & Ferro Citation2018). While wood-dwelling Coleoptera are best known from temperate and boreal forests, our knowledge from tropical forests is still unsatisfactory (Gimmel & Ferro Citation2018). Saproxylic beetles are known from 122 of the 187 beetle families and in 32 of them all or the majority of taxa are saproxylic (Gimmel & Ferro Citation2018). Among the saproxylic beetles, there are many rare and threatened taxa. In Europe, for example, 168 species are listed as saproxylic relicts of primeval forests (Cálix et al. Citation2018; Eckelt et al. Citation2018). There are also dozens of taxa of saproxylic beetles designated as “pests”, including many species of weevils, bark and ambrosia beetles, and some of jewel beetles and longhorn beetles.
Knowledge about saproxylic beetles is quite extensive thanks to the increasing number of studies (Horák et al. Citation2012). Many species have well-known distribution and population demography, reproductive biology, and ecology, including relationships with the environment and other organisms (such as fungi) (Evans et al. Citation2004; Schowalter Citation2012). Taxonomy and systematics of saproxylic beetles have been the subject of numerous studies, including recent revisions at various taxonomic levels (Knížek & Beaver Citation2007). Classification of these beetles has been additionally supported by phylogenetic and evolutionary biology studies, but mostly concerns higher units, e.g. elaboration of individual genera or families (De Santana Souza et al. Citation2020; Sun et al. Citation2020). The development of molecular tools and methods in recent decades has enabled a better understanding of the history of individual genera and species (particularly speciation, hybridization, and phylogeography), the diversity and connectivity of populations today (population genetics, demography, etc.) as well as reviewing the taxonomic status of known species, discovering new taxa (species delimitation, identification of Operational Taxonomic Units – OTU) or naming evolutionary units within species such as Evolutionary Significant Units (ESU) and Management Units (MU) (Blaxter et al. Citation2005; Allendorf et al. Citation2010; and other references in Supplementary Table S1 and in the next chapters). These findings have been sometimes focused on increasing basic knowledge about the species or group in question (Avise Citation2000; Kumar & Kumar Citation2018), but often had practical significance, e.g. identifying conservation priorities (in the case of discoveries of cryptic taxa, OTU, ESU, MU), or determining source areas for invasive species (especially those of economic importance). Moreover, the phylogeographic patterns revealed for saproxylic beetles follow (or not) some of the basic paradigms known for forest-dwelling taxa (e.g. Taberlet et al. Citation1998; Hewitt Citation2000). The available knowledge on the molecular diversity of saproxylic species is impressive but may also be highly unequal for particular taxa or groups (taxonomically, geographically, inhabiting particular forest types or tree species). Surprisingly, this topic has never been summarized before.
Considering the above reasons, we decided to conduct an intensive literature search for publications dealing with inter- and intraspecific studies of saproxylic beetles using molecular markers, focusing particularly on studies dealing with phylogeography, population genetics, landscape genetics and conservation genetics (or genomics).
The collected database of selected literature was processed to describe and summarize the knowledge related to the following issues:
Presentation of progress in intra- and interspecific genetic studies on saproxylic beetles (excluding deep phylogenies, see Material and methods), in particular, how many papers have been published over the years, in which journals, and for beetles from which parts of the world.
Taxonomic coverage of saproxylic beetles studied (how many species, genera, and families were subjected to molecular analyzes).
Ecological coverage of saproxylic beetles studied (what trophic associations, microhabitats, and host trees of species studied have been genetically examined to date).
Technical details of genetic studies on saproxylic beetles, including: sampling effort (number of sites sampled and individuals analyzed), selection of laboratory and analytical methods, and choice of genetic markers.
Use of genetic metrics and statistics for a description of the variability of examined saproxylic beetles, and comparison of available values between taxa considered as “pests” and “non-pest” species (common and endangered beetles), as well as between “pests” from Old and New World.
Elaboration of intra- and interspecific partitioning, distances and divergence dating for saproxylic beetles, and, where applicable, with comparison of New and Old World “pests”, and between common and endangered species.
Presentation of taxonomic decisions made for saproxylic beetles based on their genetics (except for deep phylogenies, see Material and methods), and listing of examples of hybridizing taxa.
Discussion of the phylogeography of saproxylic beetles, with emphasis on determining glacial refugia for which sufficient data are available.
This review also summarises genetic knowledge for selected taxa, and for a total of two defined groups (“pests” and invasive species, and primeval relicts and threatened species) based on data from the literature. We intentionally use the term “pest” in quotation marks, because species are considered pests only based on their classification in forestry.
Finally, we described information missing from previous genetic studies on saproxylic beetles, focusing on i) species for which molecular data are lacking but deserve scientific attention; ii) methods that need to be applied; and iii) scientific objectives for future research.
Material and methods
The scientific literature was searched using two databases: Web of Science; WoS (webofknowledge.com; Clarivate Analytics) and Scopus (scopus.com; Elsevier).
The following combination of keywords associated with the Boolean operators was implemented:(phylogeny OR phylogenetic OR phylogeography OR phylogeographic OR “population genetic” OR “conservation genetic” OR “landscape genetic” OR “population genomic” OR “conservation genomic” OR “landscape genomic”) AND (beetle OR Coleoptera) AND (saproxylic OR xylophagic OR saproxylophagic OR cambiophagic OR cambioxylophagic OR xylophagous OR saproxylophagous OR cambiophagous OR cambioxylophagous OR deadwood OR “dead wood” OR bark) AND (forest OR tree) AND NOT (fungi OR fungus OR fungal OR myceto OR yeast OR microbiome OR mycobiome OR microorganism OR nematode). The first set of keywords was linked to the methods of the studies, the second to the objects of the studies, and the third to the microhabitat (i.e. the substrate for saproxylic beetle development), the fourth with the general habitat, and the fifth was added to avoid entries related to fungi, bacteria, and nematodes. The search was conducted on 17/01/2021, therefore the publication date limit was 2020; however, some recently published papers could not be present in the databases at the time of the search.
The search yielded 1795 hits (Scopus) and 270 hits (WoS) results.
Each document was read critically for the information it contained on molecular studies of intra- or interspecific relationships in beetles related to “wood”, with particular reference to the study issues listed above. shows a flowchart for the systematic review following the PRISMA guidelines (Moher et al. Citation2009). Articles that had no relevance (e.g. all reports that did not focus on beetles, studies that did not use molecular = genetic data; studies on isozymes or allozymes) were excluded. This resulted in 152 (Scopus) and 132 (WoS) papers. Next, all publications dealing with i) higher-level phylogeny (we left only phylogenetic studies on species complexes or groups of closely related taxa, as such studies often contained data relevant to the objectives of this review); ii) barcoding studies on large data sets (exceptions were studies on small groups of closely related taxa as above); iii) genomics (presentation of genomes or mitogenomes); iv) marker development (mostly microsatellite loci) were excluded. Since an initial review of suitable articles revealed only two papers on predatory beetles (one on Cleridae and one on Cucujidae; all other papers found, i.e. on Carabidae or Staphylinidae, were not based on molecular data), we intentionally omitted these studies and narrowed the topic to taxa that feed on xylem, cambium, or phloem. Species associated with bracket fungi (e.g. Mycetophagidae) were also omitted because the search key excluded most papers on these taxa (only ambrosia beetles were included in the revision because they live in wood but feed on fungi that grow in the tree). Finally, 69 (Scopus) and 80 (WoS) articles were identified that met all search criteria and were appropriate for the purposes of this review. After removing duplicates (39), 110 publications remained for further review.
Figure 1. Prisma flow-diagram (see Moher et al. Citation2009) for literature search for the purposes of this study. Values indicate number of papers excluded at different assessment stages. Only articles retrieved from “Scopus” (Elsevier) and “Web of Science” (Clarivate Analytics) databases are presented.
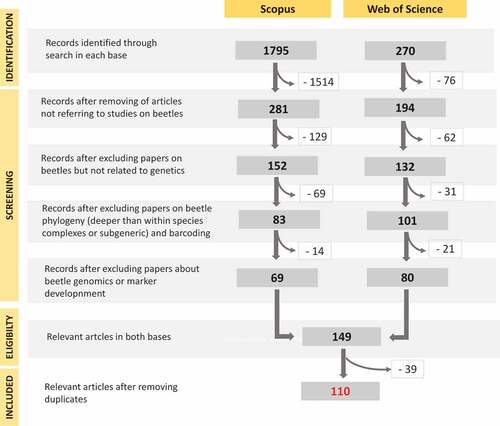
To facilitate description of the available data, analyzes, and discussion, the data set was divided into two groups: (i) “pests” (species considered harmful to trees, causing economic damage in production forests, invasive species, etc.); (ii) “non-pest” beetle species (those not referred to as “pests” in the publications and not considered to cause wood damage according to the literature and the authors’ expert knowledge) – the latter group was dominated by primeval relict or threatened taxa and other more common species. Although such classification of beetle species has neither a taxonomic nor ecological rationale (both groups include taxa from various families with different trophic associations and microhabitat preferences), it allowed us to present the available data in a simple design and to discuss the observed genetic patterns in terms of the economic importance of the “pests” and the conservation importance of the primeval relicts, threatened and protected species. In addition, for some analyzes (based on available genetic metrics), the “pests” were divided into two geographic groups: New World taxa (mostly Nearctic), and Old World taxa (mostly Palearctic). The remaining group of beetles was also divided into two subgroups based on their threat status, i.e. species with IUCN categories (see https://www.iucnredlist.org/). Thus, CR, EN, VU and NT were classified as “endangered”, while the species in the LC and DD categories and those not listed by the IUCN were classified as “common”.
We examined the collected data using the following criteria: (i) characteristics of publications [categorical], (ii) geographic distribution of studies and number of species studied from zoogeographic realms [categorical], (iii) trophic affinity and preferred microhabitats of the species studied [categorical], (iv) risk category and classification as a primeval relict according to the IUCN [binary], (v) classification as a “pest” [binary], (vi) taxonomy (number of studies conducted on beetles of specific families, genera and species) [numeric], (vii) sampling design (number of sites and individuals studied) [numeric], (viii) tree hosts (number of studies conducted on beetles associated with specific trees) [numeric], (ix) molecular marker characteristics (type and method of recovery) [categorical], (x) characteristics of molecular methods and software used (phylogenetic, delimitation, population genetic, and demographic) [categorical], (xi) basic molecular metrics and statistics (such as haplotype number and diversity, nucleotide diversity, allelic richness, heterozygosity, fixation indices, pairwise distances, isolation by distance, molecular variance, etc.) [numeric], (xii) number of distinct genetic clusters, evolutionary units, or phylogenetic lineages [numeric]. Statistical analyzes for the data collected in numerical values (e.g. Chi2 test for comparing the distribution of some traits or Kruskal-Wallis Z-test for comparing some variables between “pests” and “non-pest” beetles) were performed in R Core Team (Citation2020).
In addition, due to the nature of the data, the following characteristics were evaluated descriptively: (a) implications for conservation and management, (b) the phenomenon of hybridization, (c) invasion events, (d) taxonomic revisions, (e) phylogeographic history with the location of refugia and the distribution of distinct genetic units. Finally, for the part dealing with knowledge gaps and future challenges, data on beetle taxa characterized as primeval relicts or threatened species were taken from the following publications: Carpaneto et al. (Citation2010), Nieto and Alexander (Citation2010), Eckelt et al. (Citation2018), Cálix et al. (Citation2018), García et al. (Citation2018). In the case of “pests”, data on wood-dwelling species were taken from Lieutier et al. (Citation2004). This information was supplemented by the expert knowledge of the authors of this study.
Results and discussion
Characteristics of genetic studies on wood-dwelling beetles
Basic information
The final list of publications included 110 papers (see Supplementary Table S1). The oldest articles with relevant information were published in 1998 (Juan et al. Citation1998; Stauffer & Zuber Citation1998), and the number of articles published since then increased significantly from year to year (correlation Rho = 0.693; ). The majority of these articles (82%) were published after 2005. Studies on “pests” dominated over studies on “non-pest” beetles, except for the last three years (since 2018), when a similar number of articles were published for both of these groups.
Figure 2. Progress in number of research on saproxylic beetles – presented also separately for species considered as “pests” and “non-pest” taxa. Photographs of Osmoderma barnabita (left) and Hylobius abietis (right) were taken by J.M. Gutowski.
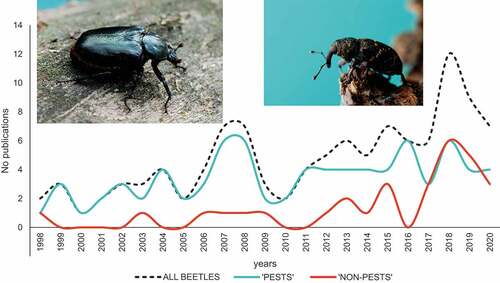
Selected studies were published in 57 scientific journals, most frequently in: Molecular Ecology (11 articles), PLoS ONE, Biological Journal of the Linnean Society and Agricultural and Forest Entomology (5 articles each) (Supplementary Figure S1).
A total of 82 articles were on beetles classified as “pests”. The “non-pest” species were the subject of studies in only 28 articles.
The objectives of the selected articles could be classified into seven categories. The majority of studies were conducted for population-genetic or landscape-genetic (40 cases), phylogeography (38), and phylogenetic-delimiting-barcoding (36) purposes. Fewer studies were conducted for invasion detection (14 cases), dispersal (11), conservation genetics (8), and reproductive mating kinship (5) (Supplementary Figure S2). Some works involved the resolving of multiple objectives.
Regarding the geographical localization of the studies performed and the number of species studied, most of them were conducted in the regions of Western Palearctic (73 articles with 57 taxa), Eastern Palearctic (43 and 42) and Nearctic (45 and 29). Significantly fewer studies were conducted and less taxa were examined in the Neotropical (14 and 12), Indomalayan (6 and 5), Afrotropical (2 and 2) and Australasian (2 and 2) regions, with no study conducted in Oceania ().
Taxonomy of examined beetles
The selected publications covered eleven beetle families. The vast majority of studies were conducted and taxa examined on Curculionidae (76 articles and 53 species), followed by Cerambycidae (17 and 19), Scarabaeidae (7 and 11), Buprestidae (2 and 2) and Lucanidae (2 and 2), Euchiridae (1 and 3), and Pythidae (1 and 3). Single species of Elateridae, Passalidae, Scraptiidae, Tenebrionidae were studied in single papers (Supplementary Figure S3).
Thirty-eight genera were studied in the selected articles (18 considered as “pests” and 20 “non-pest” species). Some studies were performed more than once on the same genera, such as Dendroctonus (22 papers and 10 species), Ips (15 and 7), Tomicus (12 and 7), Pissodes (7 and 5), Pityogenes (6 and 1), Xylosandrus (5 and 7), Osmoderma (5 and 5), Euwallacea (3 and 3), Cerambyx (3 and 2), Agrilus (2 and 2), Lucanus (2 and 2), Protaetia (2 and 2), Crypturgus (1 and 9), Cetonia (1 and 5), Anastrangalia (1 and 5), Propomacrus (1 and 3), Pytho (1 and 3), Aphanarthrum (1 and 2), and Morimus (1 and 2) (Supplementary Figure S3).
The most intensively studied species were pests: Dendroctonus ponderosae (11 papers), Ips typographus (8), Pityogenes chalcographus (7), Tomicus destruens and T. piniperda (each 7), and one endangered species: Osmoderma barnabita (5). Several species were studied in 2–3 papers, and the majority of “non-pests” was presented only in single articles (Supplementary Figure S3).
Trophic associations, microhabitats and host trees
One hundred articles dealt with species that feed on living trees (xylo- & cambiophages) – 82 of these were considered “pests” (59 species) and 18 were about “non-pest” beetles (24 species). Ten articles were about species that feed on dead trees (saprophages) – all taxa were not classified as “pests” (16 species).
Eighty-eight articles dealt with species living in fresh wood of dying and dead trees (81 “pests” and 7 “non-pest” species), 13 articles concerned species living in decomposed wood of dead trees (1 “pest” and 12 “non-pest” beetles), and 9 articles dealt with beetles living in wood mould (rotten holes) (all were not classified as “pests”).
The vast majority of studies (72%) involved beetles associated with conifers (), with pines Pinus spp. (ca. 49%) and spruces Picea spp. (ca. 19%) clearly dominating. Among deciduous trees, species associated with oaks Quercus spp. (ca. 5%), maples Acer spp. (ca. 4%) and beeches Fagus spp. (ca. 3%) were used most frequently. The differences between “pests” and “non-pest” beetles were significant (Chi2 = 1392.7, p < 0.001).
Figure 4. Number of studies on saproxylic beetles associated with particular tree hosts – presented separately for species considered as “pests” and “non-pest” taxa. Photographs of Cerambyx cerdo (left) (Photo. J.M. Gutowski), Elater ferrugineus (central) (Photo. J.M. Gutowski) and Ips acuminatus larval galleries on the trunk of pine (Photo. R. Plewa).
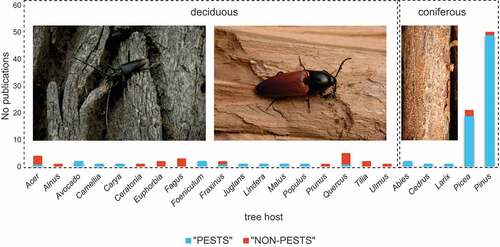
Sampling effort
The average number of populations (or sites sampled, as these two categories were listed in different papers, often interchangeably or without direct definition) was 10 (ranging from 1 to 121) per study and species studied (as some papers included studies on multiple taxa). This value was similar (Z = 0.50, p = 0.617) for “pests” (mean 12) and “non-pest” beetles (mean 9) (Supplementary Figure S4).
In contrast, the mean number of individuals examined was significantly different (Z = 2.62, p = 0.009) for “pests” (98) and “non-pest” beetles (36). The mean number of individuals examined per paper and species was 74 (from 1 to 4607) (Supplementary Figure S4).
Molecular methods and markers
There were 10 types of methods used to collect genetic data. The most commonly used method was Sanger sequencing (in 64% of studies), followed by microsatellite genotyping (17%), next-generation sequencing (NGS) with single nucleotide polymorphism (SNP) genotyping (6%) and Amplified Fragment Length Polymorphism Genotyping (5%). The remaining methods were used in individual studies (Supplementary Figure S5).
Considering only mitochondrial markers, fragments of the Cytochrome Oxidase (CO) subunit I gene were used most frequently (78% of cases), followed by CO subunit II (12%) and 16S (4%). Other mtDNA genes were used in individual studies. Except COI, COII and 16S, other genes were used only in studies on “pests”.
Regarding nuclear sequences, Internal Transcribed Spacers of rDNA (29%) and 28S of rDNA (25%) were most frequently used, followed by Elongation Factor 1-α gene (18%), and the remaining markers were used in individual studies. Most nuclear sequences were used in studies on “pests”, while only individual studies on the “non-pest” beetles used some of these markers (mostly rDNA).
The number of loci in microsatellite markers varied from 2 to 17 (mean 9), while the mean number of loci in SNPs was 1450 (min 89, max 19,904). Microsatellites were used in studies on different beetles, while SNPs were used only in studies on “pests”.
Among phylogenetic methods, Maximum Likelihood (27% of cases), Bayesian Inference (27%) and Maximum Parsimony (20%) were chosen with similar frequency, and the remaining methods were used in individual studies (Supplementary Figure S5). Phylogenies were most frequently reconstructed using Phylogenetic Analysis Using Parsimony (26%), Molecular Evolutionary Genetics Analysis (16%), PHYLogeny Inference Package (15%), MrBayes (13%), and Bayesian Evolutionary Analysis Sampling Trees (13%). The remaining tools were used occasionally (Supplementary Figure S5).
Delimitation methods were used infrequently (9 cases in 6 articles), with the following tools being used with similar frequency: Automatic Barcode Gap Discovery, Poisson Tree Processes and Generalized Mixed Yule Coalescent, but Bayesian Phylogenetics and phylogeography were used only once.
There were numerous population genetic methods used in conjunction with various software. These methods may be grouped as follows: molecular variance (used in 26% of studies), assignment tests (21% of studies), network reconstructions (20%), isolation by distance (Mantel test) (17%), multidimensional methods (9%), and nested clade analysis (6%) (Supplementary Figure S5).
Similarly, many methods and tools used in the reviewed studies for demographic analyzes could be categorized into the following groups: demographic tests (mostly Tajima and Fu tests) (used in 80% of articles), demographic Bayes methods (14%), and effective population size estimators (6%).
Genetic metrics variability
Mitochondrial haplotype diversity data were available from 43 studies. Haplotype diversity values were similar in “pests” (mean Hdiv = 0.86; 0.33–1.00; SD = 0.19) and for “non-pest” beetles (mean Hdiv = 0.95; 0.40–1.00; SD = 0.23), but overall differences were significant (Chi2 = 19.09, p = 0.024) (). The mean haplotype diversities were significantly different (Chi2 = 33.33, p < 0.001) between endangered (mean Hdiv = 0.96; 0.65–1.00; SD = 0.14) and common species (mean Hdiv = 0.52; 0.21–0.72; SD = 0.18). Also, “pests” from Old World (mean Hdiv = 0.84; 0.33–1.00; SD = 0.18) and New World (mean Hdiv = 0.96; 0.53–1.00; SD = 0.17) have significantly different haplotype diversities (Chi2 = 45.66, p < 0.001) (Supplementary Figure S6).
Figure 5. Visualization of variance of selected metrics (haplotype diversity, heterozygosity, fixation index, number of evolutionary units/genetic clusters, pairwise distance of mtDNA and divergence dating) available from research on saproxylic beetles – presented separately for species considered as “pests” and “non-pest” taxa.
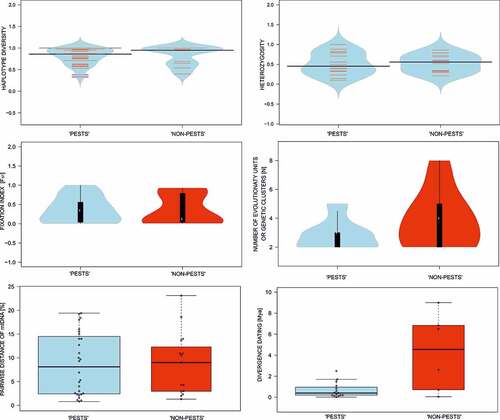
Data on heterozygosity were found in 26 articles. Mean heterozygosity was also similar in “pests” (mean He = 0.45; 0.09–1.00; SD = 0.29) and “non-pest” beetles (mean He = 0.56; 0.21–0.86; SD = 0.22), but again, overall differences were significant (Chi2 = 32.65, p < 0.001) (). The mean heterozygosity values were significantly different (Chi2 = 65.96, p < 0.001) between endangered (mean He = 0.52; 0.21–0.72; SD = 0.18) and common species (mean He = 0.79; 0.29–0.86; SD = 0.25). Also, “pests” from Old World (mean He = 0.48; 0.10–1.00; SD = 0.32) and New World (mean He = 0.45; 0.23–0.88; SD = 0.24) have significantly different haplotype diversities (Chi2 = 51.82, p < 0.001) (Supplementary Figure S6).
The fixation index (FST) was present in 46 publications. This index varied significantly (Chi2 = 239.87, p < 0.001) between “pests” (mean FST = 0.34; 0.00–1.00; SD = 0.33) and “non-pest” beetles (mean FST = 0.12; 0.01–0.93; SD = 0.40) (). The mean fixation indices were significantly different (Chi2 = 30.86, p < 0.001) between endangered (mean FST = 0.19; 0.01–0.92; SD = 0.36) and common species (mean FST = 0.03; 0.29–0.93; SD = 0.41). Also, “pests” from Old World (mean FST = 0.39; 0.00–1.00; SD = 0.33) and New World (mean FST = 0.14; 0.01–0.55; SD = 0.19) have significantly different haplotype diversities (Chi2 = 47.43, p < 0.001) (Supplementary Figure S6).
Intra- and inter-specific division, distances and divergence dating
Isolation by distance (IBD) was investigated in 28 studies which reported results for 20 taxa. Significant IBD was reported for the following taxa of “pests”: Agrilus planipennis, Dendroctonus rufipennis, D. approximatus, D. ponderosae, D. pseudotsugae, Ips typographus, I. sexdentatus, Pissodes nemorensis, P. strobi, Tomicus destruens, T. piniperda, T. yunnanensis (Stauffer et al. Citation1999; Laffin et al. Citation2004; Horn et al. Citation2006, Citation2009; Vasconcelos et al. Citation2006; Maroja et al. Citation2007; Mock et al. Citation2007; Sallé et al. Citation2007; Ruiz et al. Citation2009; James et al. Citation2011; Cullingham et al. Citation2012; Gayathri Samarasekera et al. Citation2012; Sánchez-Sánchez et al. Citation2012; Keever et al. Citation2013; Lü et al. Citation2014; Wondafrash et al. Citation2016; Avtzis et al. Citation2019). The Mantel test also proved significant in some “non-pest” beetle species: Cetonia aurata, Cerambyx welensii, Elater ferrugineus, Osmoderma barnabita, Protaetia marmorata, Rosalia alpina (Ahrens et al. Citation2013; Oleksa et al. Citation2013, Citation2015; Drag et al. Citation2015; Torres-Vila & Bonal Citation2019). IBD was found to be insignificant in studies on populations of: Ips typographus, Massicus raddei and Pityogenes chalcographus (all “pests”) (Gugerli et al. Citation2008; Bertheau et al. Citation2012; Némethy et al. Citation2018; Schebeck et al. Citation2018; Zhang et al. Citation2020).
Analysis of Molecular Variance (AMOVA) also found a significant and large (over 30%) proportion of variance between population groups of several “pests”: Agrilus planipennis, Dendroctonus valens, D. ponderosae, Ips typographus, Semanotus japonicus, Orthotomicus erosus, Pissodes nemorensis, Polygraphus proximus, Tomicus destruens, T. piniperda, T. yunnanensis and Xylosandrus germanus (Kerdelhué et al. Citation2002; Shoda et al. Citation2003; Horn et al. Citation2006; Mock et al. Citation2007; Cai et al. Citation2008; Ito et al. Citation2008; Keever et al. Citation2013; Lü et al. Citation2014; Mayer et al. Citation2014; Kononov et al. Citation2016; Wondafrash et al. Citation2016; Sarikaya & Şen Citation2017). For many “non-pest” species, AMOVA revealed low inter-group variance (e.g. Anaspis ruficollis; Schauer et al. Citation2018 and Acrocinus longimanus; Zeh et al. Citation2003).
In 65 articles, genetic data illustrated the existence of distinct genetic clusters (in the case of allelic markers) or phylogenetic lineages/evolutionary units (in the case of nucleotide markers). The total number of such distinguishable groups (regardless of their intra- or interspecific status) per taxon studied was similar (Z = −1.40, p = 0.161) for “pests” (mean N = 3.0; 2.0–5.0; SD = 1.0) and “non-pest” beetles (mean N = 4.0; 2.0–8.0; SD = 2.0) (). The mean number of clusters were insignificantly different (Z = 0.00, p = 1.00) between endangered (mean N = 4; 2–7; SD = 1.7) and common species (mean N = 3; 2–8; SD = 2.1). Also, “pests” from Old World (mean N = 3; 2–6; SD = 1.3) and New World (mean N = 3; 2–9; SD = 1.5) have significantly different haplotype diversities (Z = 0.57, p = 0.57) (Supplementary Figure S6).
Information on pairwise distances calculated on mitochondrial markers between different taxa was available in 43 articles. These distances differed significantly (Chi2 = 44.81, p = 0.004) between “pests” (mean d = 8.1; 0.8–19.4; SD = 6.5;) and “non-pest” beetles (mean d = 9.0; 1.3–23.1; SD = 6.6) (). The mean pairwise distances were significantly different (Chi2 = 62.50, p < 0.001) between endangered (mean d = 10.5; 3.0–23.1; SD = 7.2) and common species (mean d = 7.3; 1.3–16.4; SD = 5.6). Also, “pests” from Old World (mean d = 2.5; 0.0–19.4; SD = 7.4) and New World (mean d = 3.3; 0.0–16.0; SD = 5.8) have significantly different haplotype diversities (Chi2 = 35.81, p < 0.001) (Supplementary Figure S6).
These distances were next used to estimate divergence times in 21 articles. Mean divergence times were much lower for “pests” (mean D = 0.4 Mya; 0.0–2.5; SD = 0.7) than for “non-pest” beetles (mean D = 4.6 Mya; 0.1–9.0; SD = 3.7) (Chi2 = 57.33, p < 0.001) ().
Taxonomic revisions
A total of 34 taxonomic recommendations were proposed in 25 articles. Eight of them concerned the description of new taxa (Dendroctonus beckeri, Euwallacea fornicatior, E. whitfordiodendrus, E. kuroshio, Ips subelongatus, Tomicus armandii) (Stauffer et al. Citation2001; Cai et al. Citation2008; Li et al. Citation2010; Gomez et al. Citation2018), one designation of a new subspecies (Dendroctonus pseudotsugae barragani) (Ruiz et al. Citation2009), seven confirmed previous taxonomic revisions (Crypturgus subcribrosus distinct from C. cinereus; Monochamus sartor sartor distinct from M. sartor urussovii; Tomicus destruens as a valid species; distinctness of five species of Osmoderma) (Kerdelhué et al. Citation2002; Jordal & Knížek Citation2007; Audisio et al. Citation2008; Plewa et al. Citation2018), six delimited new taxa but without taxonomic decisions (new taxa distinct from: Dendroctonus brevicomis, D. frontalis, Euwallacea interjectus, Hegeter politus, Protaetia hypocrita, Odontotaenius disjunctus and Tomicus piniperda) (Juan et al. Citation1998; Kelley et al. Citation1999; Duan et al. Citation2004; Armendáriz-Toledano et al. Citation2014; Cognato et al. Citation2015; Vondráček et al. Citation2018; Garrick et al. Citation2019), eight synonymized extant taxa (Anastrangalia reyi and A. sequensi to be subspecies of A. dubia; Ips amitinus var. montana as a race of I. amitinus; rejection of the existence of five taxa related to Morimus asper; Propomacrus cypriacus reduction to P. bimucronatus cypriacus) (Stauffer & Zuber Citation1998; Solano et al. Citation2013; Sfenthourakis et al. Citation2017; Zamoroka et al. Citation2019), one rejected the distinctness of putative taxa in the Cetonia aurata complex (Ahrens et al. Citation2013) and two present uncertain taxonomic implications (doubts about the monophyly of Protaetia cuprea in relation to P. cuprina and uncertain species status of P. metallica) (Vondráček et al. Citation2018).
Hybridization
Individuals with mixed genotypes have been reported in natural populations of: Anastrangalia reyi and A. dubia in Europe (Zamoroka et al. Citation2019), Cerambyx cerdo and C. welensii (Torres-Vila & Bonal Citation2019) in Iberia, and Lucanus cervus and L. tetraodon in Italy (Solano et al. Citation2015). In addition, artificial interspecific crosses have been induced for Dendroctonus frontalis and Dendroctonus sp. nov. from Middle America (Armendáriz-Toledano et al. Citation2014).
Refugia
Phylogeographic analyzes indicating the localization of potential refugial areas (mostly glacial) were presented in 61 articles and concerned 47 beetle taxa.
Details of the phylogeographic patterns observed for all species studied are presented in Supplementary Table S2. In addition, important data on divergent lineages of some bark and ambrosia beetles are available in the barcoding study by Jordal and Kambestad (Citation2014) (not included in the review because it was identified as a large-scale barcoding study).
Several patterns of phylogeographic distribution of genetic lineages were observed for saproxylic beetles and are listed in .
Table I. Distribution of identified refugia for saproxylic beetles with lists of matching species
There are several areas in the world that could be identified as important refugia for saproxylic beetles, but unfortunately the available studies limit this list to the Palearctic and Nearctic regions. The best known glacial refugium in the Mediterranean Basin with several areas identified as genetic hotspots (Iberia, Apennines, Balkans, Anatolia, Maghreb) has been reported for 17 species, both relicts, common species and “pests”. Central European mountain systems (mainly Alps and Carpathians) proved to be refugia for at least four beetle taxa. About 20 species had distinct refugia over Eastern Europe and/or the southern Ural Mountains, Siberia, and East Asia (Far East Russia, Korea, East and South China). Another refugium for saproxylic beetles was noted in the Japanese archipelago, but the significance of this area requires further investigation, as data are available for only three taxa. In the New World, refugia were reported from eastern North America (Appalachians) (for four species), midwestern North America (Rocky Mountains) (for five taxa), the Pacific coast of North America (Cascade Mountains) (six species), and Middle America (Sierra Madres Occidental & Oriental) (two taxa) (Supplementary Figure S7). In addition, unique refugia were reported for only four species in both the Palearctic and Nearctic realms. These identified refugia, particularly in Europe, eastern Asia, and North America, fit very well with the general phylogeographic patterns known for the Palearctic and Nearctic species (Taberlet et al. Citation1998; Avise Citation2000; Hewitt Citation2000). Many of the saproxylic beetles show evidence of range contraction to southern refugia (such as the Mediterranean) during the Pleistocene. This is especially true for taxa associated with deciduous trees (e.g. Scarabaeidae, Lucanidae, Cerambycidae), whose distribution was restricted to southern areas at mid-elevations, but also for some beetles associated with conifers, especially pines (e.g. Scolytinae). Most boreal taxa of saproxylic beetles (especially Scolytinae and some Cerambycidae) were restricted to eastern refugia in Asia, from where they spread across the boreal zone into Eurasia. In addition, some of them survived the ice ages in local mountainous regions in Europe or Asia. In the case of the North American taxa, many species had refugia in the mountains of the western and eastern coastal areas and then spread in either a northeasterly or northwesterly direction. Notable is the lack of phylogeographic studies for subtropical and tropical saproxylic beetles, whose history was also influenced by glaciations, when thermophilic forests shrank, which must have consequences for the genetics of Neotropical, Afrotropical, Indomalayan and Australasian beetles.
Primeval forest relics, threatened species and conservation implications
Beetles considered to be primeval forest relicts (10 species) were studied in 18 articles.
Twenty-three publications included studies on threatened beetles. Of the taxa studied, one species had IUCN category CR (Propomacrus cypriacus: Sfenthourakis et al. Citation2017), three were EN (Osmoderma lassallei, O. italicum, O. cristinae: Audisio et al. Citation2008), four were VU (Cerambyx cerdo, Rosalia alpina, Morimus funereus and M. asper: Solano et al. Citation2013; Drag & Čížek, Citation2015; Drag et al. Citation2015, Citation2018; Melosik et al. Citation2018; Molfini et al. Citation2018; Torres-Vila & Bonal Citation2019), six were NT (Osmoderma eremita, O. barnabita, Lucanus cervus, Elater ferrugineus, Propomacrus bimucronatus and Cerambyx welensii: Audisio et al. Citation2008; Svensson et al. Citation2009; Oleksa et al. Citation2013, Citation2015; Solano et al. Citation2015; Landvik et al. Citation2017; Sfenthourakis et al. Citation2017; Cox et al. Citation2019; Torres-Vila & Bonal Citation2019; Melosik et al. Citation2020), and 12 species were in the LC category (Anastrangalia sequensi, A. sanguinea, Batocera lineolata, Lucanus tetraodon, Monochamus sartor, Protaetia marmorata, Pytho depressus, P. abieticola, Rhagium inquisitor, Prinobius myardi, Protaetia cuprina, Trichoferus campestris: Painter et al. Citation2007; Oleksa et al. Citation2013; Solano et al. Citation2015; Plewa et al. Citation2018; Vondráček et al. Citation2018; Torres-Vila & Bonal Citation2019; Zamoroka et al. Citation2019; Çakmak et al. Citation2020; Peng et al. Citation2020; Wu et al. Citation2020). One species (Pytho kolwensis: Painter et al. Citation2007) was assigned to the category DD.
Implications for conservation were presented in 13 articles for 9 taxa. For example, the probable extinction of an isolated population of Cerambyx cerdo in Poland within 19 years was reported (Melosik et al. Citation2018), but, at the same time, reintroductions proved effective in Czech Republic (Drag & Čížek Citation2015). Within the range of Rosalia alpina, distinct evolutionary units have been found in Greece and Italy, for which separate conservation measures have been recommended (Drag et al. Citation2018; Molfini et al. Citation2018). In the case of Morimus, the conservation status of M. funereus should be extended to the entire M. asper species (Solano et al. Citation2013). For Osmoderma barnabita, inbreeding in local populations has been reported (Oleksa et al. Citation2013), and the need for separate conservation of northern and central European populations has been highlighted (Landvik et al. Citation2017). Inbreeding in local populations has also been listed for Protaetia marmorata (Oleksa et al. Citation2013). Oleksa et al. (Citation2015) highlighted the importance of tree alleys for the dispersal of Elater ferrugineus. Evolutionary units of conservation value have been proposed for Lucanus cervus and L. tetraodon (Solano et al. Citation2015), with particular attention to the Greek population of the former species (Solano et al. Citation2015).
Endangered species (see Materials and methods for their assignment) were found to have significantly 0.7-fold lower haplotype diversities than common beetles (excluding “pests”), and the same pattern was observed for heterozygosity (0.65-fold lower) and fixation indices (0.3-fold lower). Contrary, pairwise distances occurred to be 1.8-fold higher among endangered species than within common taxa, and the number of identified genetic clusters or clades was 1.3-fold higher in endangered than common beetles. These differences in genetic metrics strongly suggest that populations of endangered saproxylic beetles have overall lower genetic diversities and are more structured genetically than common species. These general genetic patterns could have important implications for the proper conservation of rare and threatened saproxylic beetles, which are probably more vulnerable to environmental constraints.
“Pests” and invasive species
Beetle species of economic importance to forestry were studied in 80 articles containing data for 59 species (53 Curculionidae, 4 Cerambycidae and 2 Buprestidae). These studies (apart from invasion described below) investigated barcoding and species distinctiveness (Kohlmayr et al. Citation2002; Allender et al. Citation2008; Li et al. Citation2010; Cognato et al. Citation2019), intraspecific diversity and phylogeography (Kelley et al. Citation2000; Kerdelhué et al. Citation2002; Cognato et al. Citation2003; Ritzerow et al. Citation2004; Faccoli et al. Citation2005; Avtzis et al. Citation2006; Arthofer et al. Citation2007; Lakatos et al. Citation2007; Zhang et al. Citation2007; Anducho-Reyes et al. Citation2008; Gallego & Galián Citation2008; Ito et al. Citation2008; Ruiz et al. Citation2010; Avtzis & Aravanopoulos Citation2011, Citation2011; Schrey et al. Citation2011; Bertheau et al. Citation2013; Krascsenitsová et al. Citation2013; Janes et al. Citation2018), dispersion (Cognato et al. Citation1999; Janes et al. Citation2014; Mayer et al. Citation2015; Rugman-Jones et al. Citation2015; Sánchez-García et al. Citation2015; Dowle et al. Citation2017; Trevoy et al. Citation2018; Schebeck et al. Citation2019; Wittische et al. Citation2019) or reproduction biology (Lewis et al. Citation2001; Lewis Citation2002; Keller et al. Citation2011; Janes et al. Citation2016). The majority of these studies were undertaken for better understanding of outbreaks (which species cause damage in woods or what are source of their populations) or finding methods for preventing the spread of “pests”.
Comparison of genetic metrics found for Old World and New World “pest” saproxylic beetles revealed interesting differences, which, however, do not follow a single pattern. Available data show that Old World “pests” have overall 0.9-fold lower haplotype diversity than beetles from New World, but for heterozygosity, reverse patterns were observed (1.1-fold higher). Fixation indices were higher (2.7-fold) in Old World than New World beetles. Pairwise distances occurred to be lower (0.8-fold) in Old World than in New World. The mean number of genetic clusters or clades did not differ between “pests” from the Old and New World. These inconsistent differences could be partially explained by differences in data availability for “pest” saproxylic beetles and probably by various evolutionary histories of numerous taxa (particularly bark and ambrosia beetles) present in America or Eurasia (species of either restricted ranges or highly invasive taxa exist in both continents, having an impact on their genetic metrics). The most important topic regarding “pests” appeared to be their invasiveness (presented in 17 studies for 12 “pest” species and 2 “non-pest” beetles). East Asian populations were identified as the source of invasion into Europe of Polygraphus proximus, Xylosandrus germanus and Anoplophora glabripennis (Kononov et al. Citation2016; Javal et al. Citation2019; Dzurenko et al. Citation2021) and similar pattern was observed for North America as the main source of Euwallacea fornicatus, Agrilus planipennis, Trichoferus campestris, Allaeotes niger, Anoplophora glabripennis, Xylosandrus germanus, X. crassiusculus (Keever et al. Citation2013; Cognato et al. Citation2015; Landi et al. Citation2017; Storer et al. Citation2017; Stouthamer et al. Citation2017). Xylosandrus crassiusculus is reported to have invaded South America from North America (Landi et al. Citation2017). European populations of Pissodes castaneus have invaded South America and also Southern Africa (Zaleski et al. Citation2013; Pereyra et al. Citation2016; Wondafrash et al. Citation2016). North America was the source of invasion of Pissodes nemorensis to Africa (Wondafrash et al. Citation2016), and of Dendroctonus valens to Asia (Cognato et al. Citation2005; Cai et al. Citation2008; Taerum et al. Citation2016). Intracontinental invasions have been reported for Xylosandrus germanus from East Asia to Middle East (Dzurenko et al. Citation2021), Agrilus mali from eastern to western China (Bozorov et al. Citation2019), and Pissodes strobi from eastern to western North America (Lewis et al. Citation2001). In addition, Aphanarthrum glabrum has been reported as invasive in the Canary (Jordal et al. Citation2006). The weevil Allaeotes niger was introduced to Cuba from China but is not considered as “pest” (Grebennikov Citation2020). The graphical representation of the “invasion routes” is shown in Supplementary Figure S6.
Available information for selected taxa
The articles on saproxylic beetle taxa with available molecular data on their intra- or interspecific diversity and relationships are abundant, and it is therefore difficult to comprehensively characterize all species covered. On the other hand, many of these taxa have been studied only for specific objectives, on a very limited sample size, and with only simple methods, so that knowledge about many beetles is superficial. In the Supplementary File S1, we have selected some characteristic species from two groups: “pests” and “primeval relicts” together with threatened taxa, for which the available information is briefly summarized.
Missing data and understudied topics
Species missed in molecular studies
The numerous studies listed and characterized in this review represent only “the tip of the iceberg” of saproxylic beetle biodiversity worldwide, both in terms of species considered “pests” (at least in some areas), beetles known to be relicts of primeval forests and threatened and protected (globally or regionally) taxa. Between these two extremes are thousands of wood-dwelling beetles that are of neither economic nor conservation importance, but all of these taxa are natural elements of forest ecosystems that are critical to the proper functioning of ecological networks because of the role of saproxylic beetles in wood decomposition (Harmon et al. Citation1986; Speight Citation1989; Stokland et al. Citation2012). In addition, all these beetles are an important food source for numerous organisms (Scherzinger Citation1996; Gutowski et al. Citation2004) and often create microhabitats for other organisms (Dajoz Citation2000; Gutowski et al. Citation2004; Stokland et al. Citation2012).
It is not possible to summarize all taxa that have been overlooked in molecular studies to date. Below, we briefly list and characterize knowledge gaps in molecular studies of important saproxylic beetles of economic importance (“pests”, with special attention to invasive taxa) and of primeval relicts and/or beetles of highest conservation interest. As knowledge about these groups (especially about rare and threatened taxa) is mostly limited to the western Palearctic, we have focused on the European (west Palearctic) taxa.
“Pests”
In the Palearctic region, there are numerous saproxylic beetle species that are capable of building their populations to outbreak densities, resulting in damage to entire forest stands. Details of these beetles, which should be the object of future molecular studies are provided in Supplementary File S2. In summary, knowledge of the genetics of “pests”, particularly bark and ambrosia beetles, is quite impressive with respect to some genera that are of greatest economic importance to timber production worldwide (i.e. Ips, Pityogenes, Dendroctonus, Tomicus, Xylosandrus, Xyleborus and Euwallacea). However, some other genera of bark or ambrosia beetles have not yet been covered by molecular studies (e.g. Xyleborinus, Anisandrus, Trypodendron, Gnathotrichus, Platypus) Furthermore, for many other “pests” of conifers (e.g. Dryocoetes, Hylastes, Pityokteines) and deciduous trees (e.g. Scolytus, Hylesinus, Taphrorychus), intraspecific genetic studies are not available. The exception is studies aimed at developing barcodes for some of these taxa in large-scale studies of pests (e.g. Hulcr et al. Citation2015), or deep phylogenies (e.g. Cognato & Sperling Citation2000; Cognato & Sun Citation2007).
Primeval forest relics and threatened beetles
The current European Red List of saproxylic beetles includes 688 taxa (Cálix et al. Citation2018). Of these, 113 species have been classified as threatened on the European continent (IUCN categories CR, EN, and VU), another 69 species are near threatened (category NT), and knowledge about the conservation status of another 168 species is insufficient (category DD). Thus, about 50% of the red listed saproxylic beetles in Europe are endangered or about to be classified in this status. To date, 26 beetle species from the above-mentioned European Red List and 8 taxa considered threatened in Europe have been genetically studied (Supplementary Table S1), representing 3.8% and 1.1% of assessed taxa, respectively.
A valuable category of threatened saproxylic beetles are primeval forest relicts, whose current distribution in Europe is closely linked to remaining patches of natural/pristine forests. The list of primeval forest relict beetles in Central Europe includes 168 species from 38 families (Eckelt et al. Citation2018), of which, only 8 species have been genetically studied representing about 4.8% of this group (Supplementary Table S1).
In conclusion, despite the observed progress in the number of articles in the last decade (), the above data show that our knowledge of the intra- and interspecific genetics of endangered species, including primeval relicts, is still very incomplete, insufficiently explored, and in an initial phase.
As indicated by the attached data in Supplementary File S3, the need for research on the genetics of threatened and relict beetles is pressing. In Supplementary File S3 we provide a short list of beetles that have not yet been studied in this manner but are worth knowing about because they: i) belong to an unstudied family, ii) are protected by international initiatives for the conservation of continental biodiversity, iii) are associated with coniferous forests that dominate many areas and zones of the European continent, iv) belong to different ecological and feeding guilds (predators, mycophagous taxa, pyrophilous species), or v) are characterized by an unclear and unresolved taxonomic status.
Methodological limitations
The most important prerequisite for future studies on the genetics of saproxylic beetles is the introduction of next-generation sequencing (e.g. SNPs) or whole genomes. These methods allow for the simultaneous use of thousands of loci across the genome, greatly improving analysis capabilities. These modern methods should be used primarily on relict and endangered protected taxa, as none of them have yet been studied using these modern methods and tools.
Another necessary step would be the further development of barcode libraries for saproxylic beetles using the standard animal barcode – COI. This should be pursued to allow easy and rapid detection of saproxylic beetles in samples collected in the field and in environmental samples (bulk samples) collected from trees/wood (metabarcoding). Genomic data should be obtained for numerous species to facilitate genetic studies between and within populations. Genetic/genomic databases for saproxylic beetles should be developed and linked to taxonomy, species traits, and conservation or management requirements of barcoded/genotyped species.
Because of the rarity and low abundance of many saproxylic beetles, methods for non-invasive DNA extraction based on either live sampling (of beetle body parts), remains sampling (of insect remains), or environmental sampling (e.g. wood samples) should be implemented. Some of these methods have already been developed (see Garrick & Bouget Citation2018) and implemented (Redlarski et al. Citation2021).
Topics needed for future studies
There is still ongoing need for further studies on the phylogeography, population, and conservation genetics of selected beetle species characterized as relicts of primeval forests, and of these particularly endangered species that are highly threatened by forestry practices. In addition, molecular research continues on populations of as yet unstudied beetle species that are considered “pests” in managed forests and tree plantations, including tracking dispersal pathways for invasive species.
There is a great imbalance in terms of the geographic origin of the saproxylic beetles studied, with a lack of data from subtropical and tropical taxa and areas (especially “non-pest” taxa).
Surprisingly, there are no multi-species studies linking genetic polymorphism of saproxylic beetles (of both primeval relicts and “pests”) to quality and quantity of forest habitats and microhabitats, and to duration of forest protection or management intensity. These types of studies would improve understanding of the role of saproxylic beetles in forest ecosystem functioning and allow conservation priorities to be set, measures to protect rare and threatened taxa to be better implemented, or the mechanisms behind outbreaks of “pests” to be better understood. This research could be extended to other elements of forest ecosystems such as host trees, associated fungi, and predatory vertebrates to better understand the history of entire wood-dwelling communities and present-day interactions in forest ecosystems.
Conclusions
The present work is the first comprehensive summary of what is known, based on molecular studies, about the history, present-day diversity, and relationships of an extremely important group of organisms, namely saproxylic beetles. Species of economic importance to forestry (“pests”) have been the subject of numerous studies, and the information available for these species fills the body of knowledge relatively well, but many taxa have not yet been studied. On the other hand, only a small fraction of rare and threatened beetles have been adequately studied, and common species with neutral roles in forestry are still neglected in molecular studies, despite their critical importance to forest ecosystem functioning. The recommended directions for future molecular studies of saproxylic beetles would not only expand basic scientific knowledge, but would also be critical for the appropriate protection of rare and threatened taxa or for effective management of “pest” populations. In this regard, the database presented in this article (Supplementary Table S1) should be an excellent starting point for future scientific endeavors.
Supplemental Material
Download PDF (2.3 MB)Acknowledgements
We warmly thank Dominika Klimczyk for her assistance in the collection of articles and preparation of part of the preliminary database. We are grateful to Graham Holloway for the kind proofreading of the manuscript and valuable remarks.
Disclosure statement
No potential conflict of interest was reported by the author(s).
Authors have rights to all materials used in the article (all figures had been prepared originally for this article and all photographs used are property of one of the two Authors: Jerzy M. Gutowski and Radosław Plewa).
This study did not involve any experiments on animals or did not involve the use of human subjects. The research was carried out in accordance with accepted scientific standards.
Data availability statement
A list of the data sources is found in Supplementary Table S1 available at DOI https://doi.org/10.17605/OSF.IO/JK6V3.
Supplementary material
Supplemental data for this article can be accessed here.
Additional information
Funding
References
- Ahrens D, Fabrizi S, Šipek P, Lagod PK. 2013. Integrative analysis of DNA phylogeography and morphology of the European rose chafer (Cetonia aurata) to infer species taxonomy and patterns of postglacial colonisation in Europe. Molecular Phylogenetics and Evolution 69(1):83–94. DOI: 10.1016/j.ympev.2013.05.016.
- Allender CJ, Clancy KM, Degomez TE, Mcmillin JD, Woolbright SA, Keim P, Wagner DM. 2008. Lack of genetic differentiation in aggressive and secondary bark beetles (Coleoptera: Curculionidae, Scolytinae) from Arizona. Environmental Entomology 37(3):817–824. DOI: 10.1603/0046-225X(2008)37[817:LOGDIA]2.0.CO;2.
- Allendorf F, Hohenlohe P, Luikart G. 2010. Genomics and the future of conservation genetics. Nature Review Genetics 11:697–709. DOI: 10.1038/nrg2844.
- Anducho-Reyes M, Cognato A, Hayes J, Zuniga G. 2008. Phylogeography of the bark beetle Dendroctonus mexicanus Hopkins (Coleoptera: Curculionidae: Scolytinae). Molecular Phylogenetics and Evolution 49(3):930–940. DOI: 10.1016/j.ympev.2008.09.005.
- Armendáriz-Toledano FA, Mano JM-S, Victor J, Clarke SR. 2014. Two species within Dendroctonus frontalis (Coleoptera: Curculionidae): Evidence from morphological, karyological, molecular, and crossing studies. Annals of the Entomological Society of America 107(1):11–27. DOI: 10.1603/AN13047.
- Arthofer W, Avtzis DN, Stauffer C. 2007. Fast establishment of single-strand conformation polymorphism by targeted primer development in Pityogenes chalcographus (Coleoptera, Scolytinae). Electrophoresis 28(7):1046–1052. DOI: 10.1002/elps.200600430.
- Audisio P, Brustel H, Carpaneto GM, Coletti G, Mancini E, Trizzino M, Antonini G, De Biase A. 2008. Data on molecular taxonomy and genetic diversification of the European Hermit beetles, a species complex of endangered insects (Coleoptera: Scarabaeidae, Cetoniinae, Osmoderma). Journal of Zoological Systematics and Evolutionary Research 47(1):88–95. DOI: 10.1111/j.1439-0469.2008.00475.x.
- Avise JC. 2000. Phylogeography: The history and formation of species. Cambridge, MA: Harvard University Press.
- Avtzis DN, Aravanopoulos FA. 2011. Host tree and insect genetic diversity on the borderline of natural distribution: A case study of Picea abies and Pityogenes chalcographus (Coleoptera, Scolytinae) in Greece. Silva Fennica 45(1):157–164. DOI: 10.14214/sf.37.
- Avtzis DN, Arthofer W, Stauffer C. 2006. Identifying haplotypes of Pityogenes chalcographus (Col., Scolytidae) by Single Strand Conformation Polymorphism (SSCP). Mitteilungen der Deutschen Gesellschaft für Allgemeine und Angewandte Entomologie 15:173–176.
- Avtzis DN, Lakatos F, Gallego D, Pernek M, Faccoli M, Wegensteiner R, Stauffer C. 2019. Shallow genetic structure among the European populations of the six-toothed bark beetle Ips sexdentatus (Coleoptera, Curculionidae, Scolytinae). Forests 10(2):136. DOI: 10.3390/f10020136.
- Bertheau C, Bankhead-Dronnet S, Martin C, Lieutier F, Roux-Morabito G. 2012. Lack of genetic differentiation after host range extension argues for the generalist nature of Pityogenes chalcographus (Curculionidae: Scolytinae). Annals of Forest Science 69:313–323. DOI: 10.1007/s13595-011-0161-4.
- Bertheau C, Schuler H, Arthofer W, Avtzis DN. 2013. Divergent evolutionary histories of two sympatric spruce bark beetle species. Molecular Ecology 22(12):3318–3332. DOI: 10.1111/mec.12296.
- Blaxter M, Mann J, Chapman T, Thomas F, Whitton C, Floyd R, Abebe E. 2005. Defining operational taxonomic units using DNA barcode data. Philosophical Transactions of the Royal Society of London B Biological Sciences 360:935–1943. DOI: 10.1098/rstb.2005.1725.
- Bozorov TA, Luo Z, Li X, Zhang D. 2019. Agrilus Mali Matsumara (Coleoptera: Buprestidae), a new invasive pest of wild apple in western China: DNA barcoding and life cycle. Ecology and Evolution 9(3):1160–1172. DOI: 10.1002/ece3.4804.
- Cai Y-W, Cheng X-Y, Xu R-M, Duan D-H, Kirkendall LR. 2008. Genetic diversity and biogeography of red turpentine beetle Dendroctonus valens in its native and invasive regions. Insect Science 15(4):291–301. DOI: 10.1111/j.1744-7917.2008.00213.x.
- Çakmak YU, Soydabaş-Ayoub HK, Uçkan F. 2020. A preliminary phylogenetic analysis of ribbed-pine-borer (Rhagium inquisitor) based on mitochondrial COI sequences. Journal of Asia-Pacific Entomology 23(3):809–815. DOI: 10.1016/j.aspen.2020.06.008.
- Cálix M, Alexander KNA, Nieto A, Dodelin B, Soldati F, Telnov D, Vazquez-Albalate X, Aleksandrowicz O, Audisio P, Istrate P, Jansson N, Legakis A, Liberto A, Makris C, Merkl O, Mugerwa Pettersson R, Schlaghamersky J, Bologna MA, Brustel H, Buse J, Novák V, Purchart L. 2018. European Red List of saproxylic beetles. Brussels, Belgium: IUCN. Available: http://www.iucnredlist.org/initiatives/europe/publications.
- Carpaneto GM, Mazziotta A, Coletti G, Luiselli L, Audisio P. 2010. Conflict between insect conservation and public safety: The case study of a saproxylic beetle (Osmoderma eremita) in urban parks. Journal of Insect Conservation 14:555–565. DOI: 10.1007/s10841-010-9283-5.
- Cognato AI, Harlin AD, Fisher ML. 2003. Genetic structure among pinyon pine beetle populations (Scolytinae: Ips confusus). Environmental Entomology 32(5):1262–1270. DOI: 10.1603/0046-225X-32.5.1262.
- Cognato AI, Hoebeke ER, Kajimura H, Smith SM. 2015. History of the exotic ambrosia beetles Euwallacea interjectus and Euwallacea validus (Coleoptera: Curculionidae: Xyleborini) in the United States. Journal of Economic Entomology 108(3):1129–1135. DOI: 10.1093/jee/tov073.
- Cognato AI, Seybold SJ, Sperling FAH. 1999. Incomplete barriers to mitochondrial gene flow between pheromone races of the North American pine engraver, Ips pini (Say) (Coleoptera: Scolytidae). Proceedings of the Royal Society B. Biological Sciences 266(1431):1843–1850. DOI: 10.1098/rspb.1999.0855.
- Cognato AI, Smith SM, Li Y, Pham TH, Hulcr J. 2019. Genetic variability among Xyleborus glabratus populations native to Southeast Asia (Coleoptera: Curculionidae: Scolytinae: Xyleborini) and the description of two related species. Journal of Economic Entomology 112(3):1274–1284. DOI: 10.1093/jee/toz026.
- Cognato AI, Sperling FAH. 2000. Phylogeny of Ips DeGeer species (Coleoptera: Scolytidae) inferred from mitochondrial cytochrome oxidase I DNA sequence. Molecular Phylogenetics and Evolution 14(3):445–460. DOI: 10.1006/mpev.1999.0705.
- Cognato AI, Sun JH. 2007. DNA based cladograms augment the discovery of a new Ips species from China (Coleoptera: Curculionidae: Scolytinae). Cladistics 23(6):539–551. DOI: 10.1111/j.1096-0031.2007.00159.x.
- Cognato AI, Sun J-H, Anducho-Reyes MA, Owen DR. 2005. Genetic variation and origin of red turpentine beetle (Dendroctonus valens LeConte) introduced to the People’s Republic of China. Agricultural and Forest Entomology 7(1):87–94. DOI: 10.1111/j.1461-9555.2005.00243.x.
- Cox K, McKeown N, Antonini G, Harvey D, Solano E, Van Breusegem A, Thomaes A. 2019. Phylogeographic structure and ecological niche modelling reveal signals of isolation and postglacial colonisation in the European stag beetle. PLoS ONE 14(4):e0215860. DOI: 10.1371/journal.pone.0215860.
- Cullingham CI, Roe AD, Sperling FAH, Coltman DW. 2012. Phylogeographic insights into an irruptive pest outbreak. Ecology and Evolution 2(5):908–919. DOI: 10.1002/ece3.102.
- Dajoz R. 2000. Insects and forests. The role and diversity of insects in the forest environment. Andover, UK: Intercept Limited.
- Davies ZG, Tyler C, Stewart GB, Pullin AS. 2008. Are current management recommendations for conserving saproxylic invertebrates effective? Biodiversity Conservation 17:209–234. DOI: 10.1007/s10531-007-9242-y.
- De Groot M, Diaci J, Ogris N. 2019. Forest management history is an important factor in bark beetle outbreaks: Lessons for the future. Forest Ecology and Management 433:467–474. DOI: 10.1016/j.foreco.2018.11.025.
- De Santana Souza D, Marinoni L, Monné ML, Gómez-Zuritab J. 2020. Molecular phylogenetic assessment of the tribal classification of Lamiinae (Coleoptera: Cerambycidae). Molecular Phylogenetics and Evolution 145:106736. DOI: 10.1016/j.ympev.2020.106736.
- Dowle EJ, Bracewell RR, Pfrender ME, Mock KE, Bentz BJ, Ragland GJ. 2017. Reproductive isolation and environmental adaptation shape the phylogeography of mountain pine beetle (Dendroctonus ponderosae). Molecular Ecology 26(21):6071–6084. DOI: 10.1111/mec.14342.
- Drag L, Čížek L. 2015. Successful reintroduction of an endangered veteran tree specialist: Conservation and genetics of the Great Capricorn beetle (Cerambyx cerdo). Conservation Genetics 16:267–276. DOI: 10.1007/s10592-014-0656-2.
- Drag L, Hauck D, Bérces S, Michalcewicz J, Šerić Jelaska L, Aurenhammer S, Čížek L. 2015. Genetic differentiation of populations of the threatened saproxylic beetle Rosalia longicorn, Rosalia alpina (Coleoptera: Cerambycidae) in Central and South-East Europe. Biological Journal of the Linnean Society 116(4):911–925. DOI: 10.1111/bij.12624.
- Drag L, Hauck D, Rican O, Schmitt T, Shovkoon DF, Godunko RJ, Curletti G, Čížek L. 2018. Phylogeography of the endangered saproxylic beetle Rosalia longicorn, Rosalia alpina (Coleoptera, Cerambycidae), corresponds with its main host, the European beech (Fagus sylvatica, Fagaceae). Journal of Biogeography 45(12):2631–2644. DOI: 10.1111/jbi.13429.
- Duan Y, Kerdelhué C, Ye H, Lieutier F. 2004. Genetic study of the forest pest Tomicus piniperda (Col., Scolytinae) in Yunnan province (China) compared to Europe: New insights for the systematics and evolution of the genus Tomicus. Heredity 93:416–422. DOI: 10.1038/sj.hdy.6800518.
- Dzurenko M, Ranger CM, Hulcr J, Galko J, Kaňuch P. 2021. Origin of non-native Xylosandrus germanus, an invasive pest ambrosia beetle in Europe and North America. Journal of Pest Science 94:553–562. DOI: 10.1007/s10340-020-01283-x.
- Eckelt A, Müller J, Bense U, Brustel H, Bußler H, Chittaro Y, Čížek L, Frei A, Holzer E, Kadej M, Kahlen M, Köhler F, Möller G, Mühle H, Sanchez A, Schaffrath U, Schmidl J, Smolis A, Szallies A, Németh T, Wurst C, Thorn S, Haubo R, Christensen B, Seibold S. 2018. “Primeval forest relict beetles” of Central Europe: A set of 168 umbrella species for the protection of primeval forest remnants. Journal of Insect Conservation 22:15–28. DOI: 10.1007/s10841-017-0028-6.
- Evans HF, Moraal LG, Pajares JA. 2004. Biology, ecology and economic importance of Buprestidae and Cerambycidae. In: Lieuter F, Day KR, Battisti A, Grégoire J-C, Evans HF, editors. Bark and wood boring insects in living trees in Europe, A synthesis. Springer. pp. 447–474. DOI: 10.1007/978-1-4020-2241-8_20.
- Faccoli M, Piscedda A, Salvato P, Simonato M, Masutti L, Battisti A. 2005. Genetic structure and phylogeography of pine shoot beetle populations (Tomicus destruens and T. piniperda, Coleoptera Scolytidae) in Italy. Annals of Forest Science 62(4):361–368. DOI: 10.1051/forest:2005031.
- Gallego D, Galián J. 2008. Hierarchical structure of mitochondrial lineages of Tomicus destruens (Coleoptera, Scolytidae) related to environmental variables. Journal of Zoological Systematics and Evolutionary Research 46(4):331–339. DOI: 10.1111/j.1439-0469.2008.00472.x.
- García N, Bartolozzi C, Brustel L, Buse H, Norbiato J, Recalde M, Dodelin B, Alcázar E, Barrios V, Verdugo A, Audisio P, Micó E, Otero JC, Bahillo P, Viñolas A, Valladares L, Méndez M, El AS, Galante E. 2018. The conservation status and distribution of Mediterranean saproxylic beetles. IUCN Red List of Threatened Species - Regional Assessment 58. DOI: 10.2305/IUCN.CH.2018.RA.3.en.
- Garrick RC, Bouget C. 2018. Molecular tools for assessing saproxylic insect diversity. In: Ulyshen M, editor. Saproxylic insects. Zoological monographs, vol. 1. Cham: Springer. DOI: 10.1007/978-3-319-75937-1_25.
- Garrick R, Dickinson T, Reppel D, Yi R. 2019. Two divergent genetic lineages within the Horned Passalus beetle, Odontotaenius disjunctus (Coleoptera: Passalidae): An emerging model for insect behavior, physiology, and microbiome research. Insects 10(6):159. DOI: 10.3390/insects10060159.
- Gayathri Samarasekera GD, Bartell NV, Lindgren BS, Cooke JE, Davis CS, James PM, Coltman DW, Mock KE, Murray BW. 2012. Spatial genetic structure of the mountain pine beetle (Dendroctonus ponderosae) outbreak in western Canada: Historical patterns and contemporary dispersal. Molecular Ecology 21(12):2931–2948. DOI: 10.1111/j.1365-294X.2012.05587.x.
- Gimmel ML, Ferro ML. 2018. General overview of saproxylic Coleoptera. In: Ulyshen M, editor. Saproxylic insects. Zoological monographs, vol. 1. Cham: Springer. DOI: 10.1007/978-3-319-75937-1_2.
- Gomez DF, Skelton J, Steininger MS, Stouthamer R, Rugman-Jones P, Sittichaya W, Rabaglia RJ, Hulcr J. 2018. Species delineation within the Euwallacea fornicatus (Coleoptera: Curculionidae) complex revealed by morphometric and phylogenetic analyses. Insect Systematics and Diversity 2(6):2. DOI: 10.1093/isd/ixy018.
- Grebennikov VV. 2020. Allaeotes Niger, a weevil introduced to Cuba and the only known New World Stromboscerini (Coleoptera: Curculionidae: Dryophthorinae). Zootaxa 4803(3):495–504. DOI: 10.11646/zootaxa.4803.3.5.
- Grove SJ. 2002. Saproxylic insect ecology and the sustainable management of forests. Annual Review of Ecology and Systematics 33:1–23. DOI: 10.1146/annurev.ecolsys.33.010802.150507.
- Gugerli F, Gall R, Meier F, Wermelinger B. 2008. Pronounced fluctuations of spruce bark beetle (Scolytinae: Ips typographus) populations do not invoke genetic differentiation. Forest Ecology and Management 256(3):405–409. DOI: 10.1016/j.foreco.2008.04.038.
- Gutowski JM, Bobiec A, Pawlaczyk P, Zub K. 2004. Drugie życie drzewa. Warszawa, Hajnówka: WWF Polska.
- Harmon ME, Franklin JF, Swanson FJ, Sollins P, Gregory SV, Lattin JD, Anderson NH, Cline SP, Aumen NG, Sedell JR, Lienkaemper GW, Cromack K, Cummins KW. 1986. Ecology of coarse woody debris in temperate ecosystems. Advances in Ecological Research 34:59–234. DOI: 10.1016/S0065-2504(03)34002-4.
- Hewitt G. 2000. The genetic legacy of the Quaternary ice ages. Nature 405:907–913. DOI: 10.1038/35016000.
- Hjältén J, Stenbacka F, Pettersson RB, Gibb H, Johansson T, Danell K, Ball JP, Hilszczański J. 2012. Micro and macro-habitat associations in saproxylic beetles: Implications for biodiversity management. PLoS ONE 7(7):e41100. DOI: 10.1371/journal.pone.0041100.
- Horák J, Chumanová E, Hilszczański J. 2012. Saproxylic beetle thrives on the openness in management: A case study on the ecological requirements of Cucujus cinnaberinus from Central Europe. Insect Conservation and Diversity 5:403–413. DOI: 10.1111/j.1752-4598.2011.00173.x.
- Horn A, Roux-Morabito G, Lieutier F, Kerdelhue C. 2006. Phylogeographic structure and past history of the circum‐Mediterranean species Tomicus destruens Woll. (Coleoptera: Scolytinae). Molecular Ecology 15(6):1603–1615. DOI: 10.1111/j.1365-294X.2006.02872.x.
- Horn A, Stauffer C, Lieutier F, Kerdelhué C. 2009. Complex postglacial history of the temperate bark beetle Tomicus piniperda L. (Coleoptera, Scolytinae). Heredity 103:238–247. DOI: 10.1038/hdy.2009.48.
- Hulcr J, Atkinson TH, Cognato AI, Jordal BH, McKenna DD. 2015. Chapter 2 - Morphology, taxonomy, and phylogenetics of bark beetles. Bark beetles biology and ecology of native and invasive species. Academic Press. pp. 41–84. DOI: 10.1016/B978-0-12-417156-5.00002-2.
- Ito M, Kajimura H, Hamaguchi K, Araya K, Lakatos F. 2008. Genetic structure of Japanese populations of an ambrosia beetle, Xylosandrus germanus (Curculionidae: Scolytinae). Entomological Science 11(3):375–383. DOI: 10.1111/j.1479-8298.2008.00280.x.
- James PMA, Coltman DW, Murray BW, Hamelin RC, Sperling FAH. 2011. Spatial genetic structure of a symbiotic beetle-fungal system: Toward multi-taxa integrated landscape genetics. PLoS ONE 6(10):e25359. DOI: 10.1371/journal.pone.0025359.
- Janes JK, Li Y, Keeling CI, Yuen MMS, Boone CK, Cooke JEK, Bohlmann J, Huber DPW, Murray BW, Coltman DW, Sperling FAH. 2014. How the mountain pine beetle (Dendroctonus ponderosae) breached the Canadian Rocky Mountains. Molecular Biology and Evolution 31(7):1803–1815. DOI: 10.1093/molbev/msu135.
- Janes JK, Roe AD, Rice AV, Gorrell JC, Coltman DW, Langor DW, Sperling FAH. 2016. Polygamy and an absence of fine-scale structure in Dendroctonus ponderosae (Hopk.) (Coleoptera: Curcilionidae) confirmed using molecular markers. Heredity 116:68–74. DOI: 10.1038/hdy.2015.71.
- Janes JK, Worth JRP, Batista PD, Sperling FAH. 2018. Inferring ancestry and divergence events in a forest pest using low-density single-nucleotide polymorphisms. Insect Systematics and Diversity 2(6):3. DOI: 10.1093/isd/ixy019.
- Javal M, Lombaert E, Tsykun T, Courtin C, Kerdelhué C, Prospero S, Roques A, Roux G. 2019. Deciphering the worldwide invasion of the Asian long‐horned beetle: A recurrent invasion process from the native area together with a bridgehead effect. Molecular Ecology 28(5):951–967. DOI: 10.1111/mec.15030.
- Jordal BH, Emerson BC, Hewitt GM. 2006. Apparent ‘sympatric’ speciation in ecologically similar herbivorous beetles facilitated by multiple colonizations of an island. Molecular Ecology 15(10):2935–2947. DOI: 10.1111/j.1365-294X.2006.02993.x.
- Jordal BH, Kambestad M. 2014. DNA barcoding of bark and ambrosia beetles reveals excessive NUMTs and consistent east-west divergence across Palearctic forests. Molecular Ecology Resoirces 1:7–17. DOI: 10.1111/1755-0998.12150.
- Jordal BH, Knížek M. 2007. Resurrection of Crypturgus subcribrosus Eggers 1933 stat. n., and its close phylogenetic relationship to Nearctic Crypturgus (Coleoptera, Scolytinae). Zootaxa 1606:41–50. DOI: 10.11646/zootaxa.1606.1.3.
- Juan C, Ibrahim KM, Oromí P, Hewitt GM. 1998. The phylogeography of the darkling beetle, Hegeter politus, in the eastern Canary Islands. Proceedings of the Royal Society of London. Series B: Biological Sciences 265(1391):135–140. DOI: 10.1098/rspb.1998.0274.
- Keever CC, Nieman C, Ramsay L, Ritland CE, Bauer LS, Lyons DB, Cory JS. 2013. Microsatellite population genetics of the emerald ash borer (Agrilus planipennis Fairmaire): Comparisons between Asian and North American populations. Biological Invasions 15:1537–1559. DOI: 10.1007/s10530-012-0389-4.
- Keller L, Peer K, Bernasconi C, Taborsky M, Shuker DM. 2011. Inbreeding and selection on sex ratio in the bark beetle Xylosandrus germanus. BMC Evolutionary Biology 11:359. DOI: 10.1186/1471-2148-11-359.
- Kelley ST, Farrell BD, Mitton JB. 2000. Effects of specialization on genetic differentiation in sister species of bark beetles. Heredity 84(2):218–227. DOI: 10.1046/j.1365-2540.2000.00662.x.
- Kelley ST, Mitton JB, Paine TD. 1999. Strong differentiation in mitochondrial DNA of Dendroctonus brevicomis (Coleoptera: Scolytidae) on different subspecies of ponderosa pine. Annals of the Entomological Society of America 92(2):193–197. DOI: 10.1093/aesa/92.2.193.
- Kerdelhué C, Roux-Morabito G, Forichon J, Chambon J-M, Robert A, Lieutier F. 2002. Population genetic structure of Tomicus piniperda L. (Curculionidae: Scolytinae) on different pine species and validation of T. destruens (Woll.). Molecular Ecology 11(3):483–494. DOI: 10.1046/j.0962-1083.2002.01460.x.
- Klapwijk MJ, Bylund H, Schroeder M, Björkman C. 2016. Forest management and natural biocontrol of insect pests. Forestry 89:253–262. DOI: 10.1093/forestry/cpw019.
- Knížek M, Beaver R. 2007. Taxonomy and systematics of bark and ambrosia beetles. In: Lieutier F, et al., editors. Bark and wood boring insects in living trees in Europe, A synthesis. Germany: Springer. pp. 41–54.
- Kohlmayr B, Riegler M, Wegensteiner R, Stauffer C. 2002. Morphological and genetic identification of the three pine pests of the genus Tomicus (Coleoptera, Scolytidae) in Europe. Agricultural and Forest Entomology 4(2):151–157. DOI: 10.1046/j.1461-9563.2002.00139.x.
- Kononov A, Ustyantsev K, Blinov A, Fet V, Baranchikov YN. 2016. Genetic diversity of aboriginal and invasive populations of four-eyed fir bark beetle Polygraphus proximus Blandford (Coleoptera, Curculionidae, Scolytinae): Polygraphus proximus invasion. Agricultural and Forest Entomology 18(3):294–301. DOI: 10.1111/afe.12161.
- Kozák D, Svitok M, Wiezik M, Mikoláš M, Thorn S, Buechling A, Hofmeister J, Matula R, Trotsiuk V, Bače R, Begovič K, Čada V, Dušátko M, Frankovič M, Horák J, Janda P, Kameniar O, Nagel TA, Pettit JL, Pettit JM, Synek M, Wieziková A, Svoboda M. 2021. Historical disturbances determine current taxonomic, functional and phylogenetic diversity of saproxylic beetle communities in temperate primary forests. Ecosystems 24(10):37–55. DOI: 10.1007/s10021-020-00502-x.
- Krascsenitsová E, Kozánek M, Ferenčík J, Roller L, Stauffer C, Bertheau C. 2013. Impact of the Carpathians on the genetic structure of the spruce bark beetle Ips typographus. Journal of Pest Science 86:669–676. DOI: 10.1007/s10340-013-0508-8.
- Kumar R, Kumar V. 2018. A review of phylogeography: Biotic and abiotic factors. Geology, Ecology, and Landscapes 2(4):268–274. DOI: 10.1080/24749508.2018.1452486.
- Laffin RD, Langor DW, Sperling FAH. 2004. Population structure and gene flow in the white pine weevil, Pissodes strobi (Coleoptera: Curculionidae). Annals of the Entomological Society of America 97(5):949–956. DOI: 10.1603/0013-8746(2004)097[0949:PSAGFI]2.0.CO;2.
- Lakatos F, Grodzki W, Zhang Q-H, Stauffer C. 2007. Genetic comparison of Ips duplicatus (Sahlberg, 1836) (Coleoptera: Curculionidae, Scolytinae) populations from Europe and Asia. Journal of Forest Research 12:345–349. DOI: 10.1007/s10310-007-0025-9.
- Landi L, Gómez D, Braccini CL, Pereyra VA, Smith SM, Marvaldi AE. 2017. Morphological and molecular identification of the invasive Xylosandrus crassiusculus (Coleoptera: Curculionidae: Scolytinae) and its South American range extending into Argentina and Uruguay. Annals of the Entomological Society of America 110(3):344–349. DOI: 10.1093/aesa/sax032.
- Landvik M, Miraldo A, Niemelä P, Valainis U, Cibuļskis R, Roslin T. 2017. Evidence for geographic substructuring of mtDNA variation in the East European Hermit beetle (Osmoderma barnabita). Nature Conservation 19:171–189. DOI: 10.3897/natureconservation.19.12877.
- Lewis KG. 2002. Sexual reproduction in the white pine weevil (Pissodes strobi [Peck] [Coleoptera: Curculionidae]): Implications for population genetic diversity. Journal of Heredity 93(3):165–169. DOI: 10.1093/jhered/93.3.165.
- Lewis KG, Ritland K, El-Kassaby YA, McLean JA, Glaubitz J, Carlson JE. 2001. Randomly amplified polymorphic DNA reveals fine-scale genetic structure in Pissodes strobi (Coleoptera: Curculionidae). The Canadian Entomologist 133(2):229–238. DOI: 10.4039/Ent133229-2.
- Li X, Zhang Z, Wang H, Wu W, Cao P, Zhang P. 2010. Tomicus armandii Li & Zhang (Curculionidae, Scolytinae), a new pine shoot borer from China. Zootaxa 2572(1):57–64. DOI: 10.11646/zootaxa.2572.1.4.
- Lieutier F, Day KR, Battisti A, Grégoire J-C, Evans HF, editors. 2004. Bark and wood boring insects in living trees in Europe, a synthesis. Dordrecht: Kluwer Academic Publisher. DOI: 10.1007/978-1-4020-2241-8.
- Lü J, Hu S, Ma X, Chen J, Li Q, Ye H. 2014. Origin and expansion of the Yunnan shoot borer, Tomicus yunnanensis (Coleoptera: Scolytinae): A mixture of historical natural expansion and contemporary human-mediated relocation. PLoS ONE 9(11):e111940. DOI: 10.1371/journal.pone.0111940.
- Maroja LS, Bogdanowicz SM, Wallin KF, Raffa KF, Harrison RG. 2007. Phylogeography of spruce beetles (Dendroctonus rufipennis Kirby) (Curculionidae: Scolytinae) in North America. Molecular Ecology 16(12):2560–2573. DOI: 10.1111/j.1365-294X.2007.03320.x.
- Mason F, Zapponi L. 2015. The forest biodiversity artery: Towards forest management for saproxylic conservation. iForest - Biogeosciences and Forestry 9(2):205–216. DOI: 10.3832/ifor1657-008.
- Mayer F, Björklund N, Wallén J, Långström B, Cassel-Lundhagen A. 2014. Mitochondrial DNA haplotypes indicate two postglacial re-colonization routes of the spruce bark beetle Ips typographus through Northern Europe to Scandinavia. Journal of Zoological Systematics and Evolutionary Research 52(4):285–292. DOI: 10.1111/jzs.12063.
- Mayer F, Piel FB, Cassel-Lundhagen A, Kirichenko N, Grumiau L, Økland B, Bertheau C, Grégoire J-C, Mardulyn P. 2015. Comparative multilocus phylogeography of two Palaearctic spruce bark beetles: Influence of contrasting ecological strategies on genetic variation. Molecular Ecology 24(6):1292–1310. DOI: 10.1111/mec.13104.
- Melosik I, Baraniak E, Przewoźny M, Grzegorczyk T, Rzepka M. 2020. Does gene flow balance the effect of habitat fragmentation in a population of the hermit beetle Osmoderma barnabita? Insect Conservation and Diversity 13(4):360–373. DOI: 10.1111/icad.12413.
- Melosik I, Przewoźny M, Winnicka K, Baraniak E, Staszak J. 2018. Use of a genetically informed population viability analysis to evaluate management options for Polish populations of endangered beetle Cerambyx cerdo L. (1758) (Coleoptera, Cerambycidae). Journal of Insect Conservation 22:69–83. DOI: 10.1007/s10841-017-0039-3.
- Mock KE, Bentz BJ, O’neill EM, Chong JP, Orwin J, Pfrender ME. 2007. Landscape-scale genetic variation in a forest outbreak species, the mountain pine beetle (Dendroctonus ponderosae). Molecular Ecology 16(3):553–568. DOI: 10.1111/j.1365-294X.2006.03158.x.
- Moher D, Liberati A, Tetzlaff J, Altman DG, The PRISMA Group. 2009. Preferred reporting items for systematic reviews and meta-analyses: The PRISMA statement. PLoS Medicine 6(7):e1000097. DOI: 10.1136/bmj.b2535.
- Molfini M, Redolfi de Zan L, Campanaro A, Rossi de Gasperis S, Mosconi F, Chiari S, Cini A, Antonini G, Solano E, Audisio PA, Roversi PF, Sabbatini Peverieri G, Carpaneto GM, Mason F, Bologna MA, Mancini E. 2018. A first assessment of genetic variability in the longhorn beetle Rosalia alpina (Coleoptera: Cerambycidae) from the Italian Apennines. The European Zoological Journal 85(1):36–45. DOI: 10.1080/24750263.2018.1433243.
- Müller J, Bußler H, Goßner M, Rettelbach T, Duelli P. 2008. The European spruce bark beetle Ips typographus in a national park: From pest to keystone species. Biodiversity and Conservation 17:2979. DOI: 10.1080/24750263.2018.1433243.
- Némethy M, Mihálik D, Steifetten Ø, Rošteková V, Mrkvová M, Janiga M, Kraic J. 2018. Genetic differentiation between local populations of Ips typographus in the high Tatra Mountains range. Scandinavian Journal of Forest Research 33(3):215–221. DOI: 10.1080/02827581.2017.1368697.
- Nieto A, Alexander KNA, editors. 2010. European Red List of saproxylic beetles. Luxembourg: Publications Office of the European Union.
- Oleksa A, Chybicki IJ, Gawroński R, Glenn P, Svensson GP, Burczyk J. 2013. Isolation by distance in saproxylic beetles may increase with niche specialization. Journal of Insect Conservation 17:219–233. DOI: 10.1007/s10841-012-9499-7.
- Oleksa A, Chybicki IJ, Mattias CL, Svensson GP, Gawroński R. 2015. Rural avenues as dispersal corridors for the vulnerable saproxylic beetle Elater ferrugineus in a fragmented agricultural landscape. Journal of Insect Conservation 19:567–580. DOI: 10.1007/s10841-015-9778-1.
- Painter JN, Siitonen J, Hanski I. 2007. Phylogeographical patterns and genetic diversity in three species of Eurasian boreal forest beetles: Phylogeography of Eeurasian Pytho beetles. Biological Journal of the Linnean Society 91(2):267–279. DOI: 10.1111/j.1095-8312.2007.00797.x.
- Peng H, Zhang S, Geng X, Fang L, Zhang W, Shu J, Wang H. 2020. Population differentation of Batocera lineolate (Coleoptera: Cerambycidae) adults whose larvae fed on different host tree species. Scientia Silvae Sinicae 56:91–103. DOI: 10.11707/j.1001-7488.20200710.
- Pereyra VA, Gomez CA, La Manna L, Roux G, Lanteri AA, Vallejos NC, Marvaldi AE. 2016. Introduction and establishment of Pissodes castaneus (Coleoptera: Curculionidae) in the Andean Patagonia of Argentina. Journal of Economic Entomology 109(1):222–231. DOI: 10.1093/jee/tov304.
- Plewa R, Sikora K, Gutowski JM, Jaworski T, Tarwacki G, Tkaczyk M, Rossa R, Hilszczański J, Magoga G, Kajtoch Ł. 2018. Morphology, genetics and Wolbachia endosymbionts support distinctiveness of Monochamus sartor sartor and M. s. urussovii (Coleoptera: Cerambycidae). Arthropod Systematics & Phylogeny 76(1):123–135.
- R Core Team. 2020. R: A language and environment for statistical computing. Vienna, Austria: R Foundation for Statistical Computing. Available: http://www.r-project.org/index.html.
- Redlarski AJ, Klejdysz T, Kadej M, Meyza K, Vasilița C, Oleksa A. 2021. Body remains left by bird predators as a reliable source for population genetic studies in the great capricorn beetle Cerambyx cerdo, a veteran oak specialist. Insects 12(7):574. 23. DOI: 10.3390/insects12070574.
- Ritzerow S, Konrad H, Stauffer C. 2004. Phylogeography of the Eurasian pine shoot beetle Tomicus piniperda (Coleoptera: Scolytidae). European Journal of Entomology 101(1):13–19. DOI: 10.14411/eje.2004.003.
- Rugman-Jones PF, Seybold SJ, Graves AD, Stouthamer R. 2015. Phylogeography of the walnut twig beetle, Pityophthorus juglandis, the vector of thousand cankers disease in North American walnut trees. PLoS ONE 10(2):e0118264. DOI: 10.1371/journal.pone.0118264.
- Ruiz EA, Rinehart JE, Hayes JL, Zuñiga G. 2010. Historical demography and phylogeography of a specialist bark beetle, Dendroctonus pseudotsugae Hopkins (Curculionidae: Scolytinae). Environmental Entomology 39(5):1685–1697. DOI: 10.1603/en09339.
- Ruiz EA, Rinehart JE, Hayes JL, Zúñiga G. 2009. Effect of geographic isolation on genetic differentiation in Dendroctonus pseudotsugae (Coleoptera: Curculionidae). Hereditas 146(2):79–92. DOI: 10.1111/j.1601-5223.2009.02095.x.
- Sallé A, Arthofer W, Lieutier F, Stauffer C, Kerdelhué C. 2007. Phylogeography of a host‐specific insect: Genetic structure of Ips typographus in Europe does not reflect past fragmentation of its host. Biological Journal of the Linnean Society 90(2):239–246. DOI: 10.1111/j.1095-8312.2007.00720.x.
- Sánchez-García FJ, Galián J, Gallego D. 2015. Distribution of Tomicus destruens (Coleoptera: Scolytinae) mitochondrial lineages: Phylogeographic insights and niche modelling. Organisms Diversity & Evolution 15:101–113. DOI: 10.1007/s13127-014-0186-2.
- Sánchez-Sánchez H, Lopez-Barrera G, Penaloza-Ramirez JM, Rocha-Ramirez V, Oyama K. 2012. Phylogeography Reveals routes of colonization of the bark beetle Dendroctonus approximatus Dietz in Mexico. Journal of Heredity 103(5):638–650. DOI: 10.1093/jhered/ess043.
- Sarikaya O, Şen İ. 2017. Population genetic structure of Orthotomicus erosus (Wollaston, 1857) (Coleoptera: Curculionidae, Scolytinae) in pine forests of the Mediterranean region of Turkey. Applied Ecology and Environmental Research 15(4):915–928. DOI: 10.15666/aeer/1504_915928.
- Schauer B, Bong J, Popp C, Obermaier E, Feldhaar H. 2018. Dispersal limitation of saproxylic insects in a managed forest? A population genetics approach. Basic and Applied Ecology 32:26–38. DOI: 10.1016/j.baae.2018.01.005.
- Schebeck M, Dowle EJ, Schuler H, Avtzis DN, Bertheau C, Feder JL, Ragland GJ, Stauffer C. 2018. Pleistocene climate cycling and host plant association shaped the demographic history of the bark beetle Pityogenes chalcographus. Scientific Reports 8:14207. DOI: 10.1038/s41598-018-32617-6.
- Schebeck M, Schuler H, Einramhof B, Avtzis DN, Dowle EJ, Faccoli M, Battisti A, Ragland GJ, Stauffer C, Bertheau C. 2019. The Apennines as a cryptic Pleistocene refugium of the bark beetle Pityogenes chalcographus (Coleoptera: Curculionidae). Biological Journal of the Linnean Society 127(1):24–33. DOI: 10.1093/biolinnean/blz012.
- Scherzinger W. 1996. Naturschutz im Wald. Qualitätsziele einer dynamischen Waldentwicklung. Stuttgart: Ulmer Verlag. DOI: 10.1002/mmnz.19980740118.
- Schowalter TD. 2012. Ecology and management of bark beetles (Coleoptera: Curculionidae: Scolytinae) in southern pine forests. Journal of Integrated Pest Management 3(2):A1–A7. DOI: 10.1603/ipm11025.
- Schrey NM, Schrey AW, Heist EJ, Reeve JD. 2011. Genetic heterogeneity in a cyclical forest pest, the southern pine beetle, Dendroctonus frontalis, is differentiated into east and west groups in the southeastern United States. Journal of Insect Science 11(110):1–10. DOI: 10.1673/031.011.11001.
- Sfenthourakis S, Hadjiconstantis M, Makris C, Dimitriou AC. 2017. Revisiting the saproxylic beetle ‘Propomacrus cypriacus Alexis & Makris, 2002’ (Coleoptera: Euchiridae) using molecular, morphological and ecological data. Journal of Natural History 51(17–18):1021–1034. DOI: 10.1080/00222933.2017.1319521.
- Shoda E, Kubota K, Makihara H. 2003. Geographical structuring of mitochondrial DNA in Semanotus japonicus (Coleoptera: Cerambycidae). Applied Entomology and Zoology 38(3):339–345. DOI: 10.1303/aez.2003.339.
- Siitonen J. 2001. Forest management, coarse woody debris and saproxylic organisms: Fennoscandian boreal forest as an example. Ecological Bulletins 49:11–41.
- Ślipiński SA, Leschen RAB, Lawrence JF. 2011. Order Coleoptera Linnaeus, 1758. In: Zhang Z-Q, editor. Animal biodiversity: An outline of higher-level classification and survey of taxonomic richness. Zootaxa, vol. 3148, pp. 203–208. DOI: 10.11646/zootaxa.3148.1.39.
- Solano E, Mancini E, Ciucci P, Mason F, Audisio P, Antonini G. 2013. The EU protected taxon Morimus funereus Mulsant, 1862 (Coleoptera: Cerambycidae) and its western Palaearctic allies: Systematics and conservation outcomes. Conservation Genetics 14:683–694. DOI: 10.1007/s10592-013-0461-3.
- Solano E, Thomaes A, Cox K, Carpaneto GM, Cortellessa S, Baviera C, Bartolozzi L, Zilioli M, Casiraghi M, Audisio P, Antonini G. 2015. When morphological identification meets genetic data: The case of Lucanus cervus and L. tetraodon (Coleoptera, Lucanidae). Journal of Zoological Systematics and Evolutionary Research 54(3):197–205. DOI: 10.1111/jzs.12124.
- Speight MCD. 1989. Saproxylic invertebrates and their conservation. Nature and environment series 42. Strasbourg: Council of Europe.
- Stauffer C, Kirisits T, Nussbaumer C, Pavlin R, Wingfield MJ. 2001. Phylogenetic relationships between the European and Asian eight spined larch bark beetle populations (Coleoptera, Scolytidae) inferred from DNA sequences and fungal associates. European Journal of Entomology 98(1):99–105. DOI: 10.14411/eje.2001.014.
- Stauffer C, Lakatos F, Hewitt GM. 1999. Phylogeography and postglacial colonization routes of Ips typographus L. (Coleoptera, Scolytidae). Molecular Ecology 8(5):763–773. DOI: 10.1046/j.1365-294x.1999.00626.x.
- Stauffer C, Zuber M. 1998. Ips amitinus var. Montana (Coleoptera, Scolytidae) is synonymous to Ips amitinus: A morphological, behavioural and genetic re-examination. Biochemical Systematics and Ecology 26(2):171–183. DOI: 10.1016/s0305-1978(98)80004-5.
- Stokland JN, Siitonen J, Jonsson BG. 2012. Biodiversity in dead wood. Cambridge: Cambridge University Press. DOI: 10.33112/nm.8.1.27.
- Storer C, Payton A, McDaniel S, Jordal B, Hulcr J. 2017. Cryptic genetic variation in an inbreeding and cosmopolitan pest, Xylosandrus crassiusculus, revealed using ddRADseq. Ecology and Evolution 7(24):10974–10986. DOI: 10.1002/ece3.3625.
- Stouthamer R, Rugman-Jones P, Thu PQ, Eskalen A, Thibault T, Hulcr J, Wang L-J, Jordal BH, Chen C-Y, Cooperband M, Lin C-S, Kamata N, Lu -S-S, Masuya H, Mendel Z, Rabaglia R, Sanguansub S, Shih -H-H, Sittichaya W, Zong S. 2017. Tracing the origin of a cryptic invader: Phylogeography of the Euwallacea fornicatus (Coleoptera: Curculionidae: Scolytinae) species complex. Agricultural and Forest Entomology 19(4):366–375. DOI: 10.1111/afe.12215.
- Sun H, Zhao W, Lin R, Zhou Z, Huai W, Yao Y. 2020. The conserved mitochondrial genome of the jewel beetle (Coleoptera: Buprestidae) and its phylogenetic implications for the suborder Polyphaga. Genomics 112(5):3713–3721. DOI: 10.1016/j.ygeno.2020.04.026.
- Svensson GP, Oleksa A, Gawroński R, Lassance J-M, Larsson MC. 2009. Enantiomeric conservation of the male-produced sex pheromone facilitates monitoring of threatened European hermit beetles (Osmoderma spp.). Entomologia Experimentalis Et Applicata 133(3):276–282. DOI: 10.1111/j.1570-7458.2009.00923.x.
- Taberlet P, Fumagalli L, Wust-Saucy AG, Cossons JF. 1998. Comparative phylogeography and postglacial colonization routes in Europe. Molecular Ecology 7:453–464. DOI: 10.1046/j.1365-294x.1998.00289.x.
- Taerum SJ, Konec A, Beer ZWD, Cibria D, Wingfield MJ. 2016. Population genetics and symbiont assemblages support opposing invasion scenarios for the red turpentine beetle (Dendroctonus valens). Biological Journal of the Linnean Society 118(3):486–502. DOI: 10.1111/bij.12781.
- Torres-Vila LM, Bonal R. 2019. DNA barcoding of large oak-living cerambycids: Diagnostic tool, phylogenetic insights and natural hybridization between Cerambyx cerdo and Cerambyx welensii (Coleoptera: Cerambycidae). Bulletin of Entomological Research 109(5):583–594. DOI: 10.1017/s0007485318000925.
- Trevoy SAL, Janes JK, Sperling FAH. 2018. Where did mountain pine beetle populations in Jasper Park come from? Tracking beetles with genetics. The Forestry Chronicle 94(1):20–24. DOI: 10.5558/tfc2018-004.
- Vasconcelos T, Horn A, Lieutier F, Branco M, Kerdelhue C. 2006. Distribution and population genetic structure of the Mediterranean pine shoot beetle Tomicus destruens in the Iberian Peninsula and Southern France. Agricultural and Forest Entomology 8(2):103–111. DOI: 10.1111/j.1461-9563.2006.00292.x.
- Vondráček D, Fuchsová A, Ahrens D, Král D, Šípek P. 2018. Phylogeography and DNA-based species delimitation provide insight into the taxonomy of the polymorphic rose chafer Protaetia (Potosia) cuprea species complex (Coleoptera: Scarabaeidae: Cetoniinae) in the Western Palearctic. PLoS ONE 13(2):e0192349. DOI: 10.1371/journal.pone.0192349.
- Wittische J, Janes JK, James PMA. 2019. Modelling landscape genetic connectivity of the mountain pine beetle in western Canada. Canadian Journal of Forest Research 49(11):1339–1348. DOI: 10.1139/cjfr-2018-0417.
- Wondafrash M, Slippers B, Garnas J, Roux G, Foit J, Langor DW, Hurley BP. 2016. Identification and genetic diversity of two invasive Pissodes spp. Germar (Coleoptera: Curculionidae) in their introduced range in the southern hemisphere. Biological Invasions 18:2283–2297. DOI: 10.1007/s10530-016-1159-5.
- Wu Y, Krishnankutty SM, Vieira KA, Wang B, Nadel H, Myers SW, Ray AM. 2020. Invasion of Trichoferus campestris (Coleoptera: Cerambycidae) into the United States characterized by high levels of genetic diversity and recurrent introductions. Biological Invasions 22(4):1309–1323. DOI: 10.1007/s10530-019-02182-8.
- Zaleski SRM, Lazzari SMN, Lazzarotto CM, Panzavolta T, Iede ET, de Marques AF. 2013. Genetic structure of populations of Pissodes castaneus (De Geer) (Coleoptera, Curculionidae) using amplified fragment length polymorphism. Revista Brasileira de Entomologia 57(4):405–410. DOI: 10.1590/S0085-56262013005000036.
- Zamoroka AM, Semaniuk DV, Shparyk VY, Mykytyn TV, Skrypnyk SV. 2019. Taxonomic position of Anastrangalia reyi and A. sequensi (Coleoptera, Cerambycidae) based on molecular and morphological data. Vestnik Zoologii 53(3):209–226. DOI: 10.2478/vzoo-2019-0021.
- Zeh DW, Zeh JA, Bonilla MM. 2003. Phylogeography of the giant harlequin beetle (Acrocinus longimanus): Harlequin beetle phylogeography. Journal of Biogeography 30(5):747–753. DOI: 10.1046/j.1365-2699.2003.00880.x.
- Zhang H, Langor DW, Ye H, Li Z, Laffin RD. 2007. Genetic divergence among populations of Pissodes yunnanensis (Coleoptera: Curculionidae) in southwestern China. The Canadian Entomologist 139(3):308–318. DOI: 10.4039/n06-043.
- Zhang Y, Manzoor A, Wang X. 2020. Mitochondrial DNA analysis reveals spatial genetic structure and high genetic diversity of Massicus raddei (Blessig) (Coleoptera: Cerambycidae) in China. Ecology and Evolution 10(20):11657–11670. DOI: 10.1002/ece3.6799.