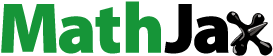
Abstract
Diclofenac (DCF), a non-steroidal anti-inflammatory drug (NSAID), is among the main pollutants of aquatic environments. Nevertheless, even if several authors evaluated its effects on marine organisms, no work has ever analysed its impact on the sea urchin Arbacia lixula. The purpose of this study was to analyse, for the first time, the impact of DCF at different concentrations (50 mg/l, 5 mg/l, 0.5 mg/l, 0.05 mg/l) on A. lixula embryos and gametes to indicate possible safe minimum levels of release of this drug in marine environments. Our results showed significant concentration-dependent effects on embryonic development (decrease in the number of developed embryos and increase in the quantity of degenerated eggs) and significant levels of bioaccumulation at DCF concentrations of 50 mg/l in both gametes and embryos. DCF showed a significant impact on the fertilisation of A. lixula due to its effects on gametes, confirming the possibility of environmental risk and highlighting the need to improve wastewater treatment and drug disposal processes.
Introduction
To date, pharmaceuticals compounds (PhACs) released in marine environment (e.g. antibiotic, anti inflammatory, antidepressants) are a threat for marine ecosystems (Branchet et al. Citation2021; Mezzelani & Regoli Citation2022). Among 70%–80% of this pollution is caused by humans, who expel PhACs as active metabolites via urine or faeces after ingestion (Zuccato et al. Citation2000). The remaining 20%–30% is caused by illegal industrial disposal (Heberer Citation2002; Bonnefille et al. Citation2018). Prior to its release into the aquatic environment, the waste passes through purification plants which are not yet efficient (Ojemaye & Petrik Citation2019; Angeles et al. Citation2020) at removing drug traces in surface water, ground water, and sediments (Zuccato et al. Citation2000; Farré et al. Citation2001; Bonnefille et al. Citation2018). These substances released in aquatic environments are foreign to the diets and metabolisms of marine organisms and can significantly affect marine communities and ecosystems (Desbiolles et al. Citation2018; Sehonova et al. Citation2018; Patel et al. Citation2019). One of the world’s most popular drugs is diclofenac (DCF; 2-[(2,6-dichlorophenyl) amino] phenylacetic acid) — the active ingredient in a number of non-steroidal anti-inflammatory medications (NSAIDs; Acuña et al. Citation2015; Fabbri & Franzellitti Citation2016). Its presence in seawater has been identified at concentrations ranging from 0.6 to 843.0 ng/L (Fent et al. Citation2006; Ankley et al. Citation2007; Gaw et al. Citation2014), and a number of scientific studies have also found it in drinking water, surface water, sewage, and aquatic organisms (Heberer & Feldmann Citation2005; Liu et al. Citation2015; Xie et al. Citation2015, Citation2017; Tran et al. Citation2018; Praveena et al. Citation2019). In detail, concentrations of DCF in water bodies vary from a few ng/l to μg/l (Zhang et al. Citation2008; Wang et al. Citation2010; Gonzalez-Rey et al. Citation2015; Xie et al. Citation2017; Nantaba et al. Citation2020), while concentrations in surface and wastewater range from a number of ng/L to a few mg/L (Vieno & Sillanpää Citation2014; Lonappan et al. Citation2016; Sousa et al. Citation2019; Sathishkumar et al. Citation2020). DCF is on the European Commission’s “Checklist” concerning the control of water bodies (European Union, EU Citation2015/495) and was added to the “EU Water Framework Directive” watch list in 2013 (EU, Citation2013).Due to its biologically and pharmacokinetically high activity, it can damage aquatic biota (Jobling et al. Citation2003); in fact, several authors showed delay in the development of fish Danio rerio (Van den Brandhof & Montforts Citation2010; Ribeiro et al. Citation2015; Zhang et al. Citation2020), damages in liver, kidneys, and gills of fish Oncorhynchus mykiss (Triebskorn et al. Citation2004; Gronër et al. Citation2017), teratogenesis, and embryotoxicity in amphibians Xenopus laevis and Lithobates catesbeianus (Cardoso-Vera et al. Citation2017). Although most scientific studies involved freshwater species (Aguirre-Martínez et al. Citation2013; Hughes et al. Citation2013), marine organisms, key sources of bioactive molecules (Lazzara et al. Citation2019; Inguglia et al. Citation2020; Luparello et al. Citation2020a, Citationb, Luparello Citation2021; Mauro et al. Citation2020a) and important environmental bioindicators (Cammilleri et al. Citation2019; Chiaramonte et al. Citation2020; Vazzana et al. Citation2020a, Citationb; Mauro et al. Citation2020b, Citation2021) can be adversely affected by drug pollution, and marine invertebrates in particular are among the organisms most threatened by drug pollution (Gagné et al. Citation2006; Lonappan et al. Citation2016). DCF is classified in European Union Directive 93/67/EEC as a very toxic substance to gametes of starfish Asterias rubens, sea urchin Psammechinus miliaris, and bloodworm Arenicola marina (EC50 = 100 and 1000 µg/l). In literature, several scientific studies highlighted that DCF can affect marine invertebrates at biochemical and physiological levels as in the case of mollusca Ruditapes philippinarum (Matozzo et al. Citation2012; Munari et al. Citation2018), crab Carcinus maenas (Eades & Waring Citation2010), mollusca Mytilus galloprovincialis and Mytilus edulis (Schmidt et al. Citation2011; Cunha et al. Citation2017; Mezzelani et al. Citation2018; Munari et al. Citation2018), Perna perna, Dreissena polymorpha, and planktonic crustacean Daphnia magna (Quinn et al. Citation2011; Cortez et al. Citation2012; Riva et al. Citation2012; Liu et al. Citation2017). Given the risk that DCF pollution can cause, it is now urgent to acquire further data regarding its effects on marine species to fully understand the toxicity and environmental risks. The aim of this study was to evaluate, for the first time, the effects of DCF at different concentrations on embryonic development and accumulation in embryos and gametes (eggs and spermatozoa) using A. lixula sea urchin as a model organism. It was decided to analyse the first phases of the life cycle because as well as being important for the survival of the species, these phases are particularly sensitive to environmental conditions and are used in the ecotoxicological approach to assess the quality of the environment (De Campos et al. Citation2022; Thompson et al. Citation2022; Venâncio et al. Citation2022). The objective of the study is to provide a better understanding of the effects of diclofenac in seawater to establish the potential toxicity and understand the relationship between concentrations and the effects on non-target organisms. The results obtained could help to fill the lack of information regarding the real effects (likely species-dependent) of DCF on marine species and to provide additional indications to establish limit values of the release of DCF into marine environments for the protection of biodiversity.
Materials and methods
Experimental animals
Sixty specimens of A. lixula were collected in spring from the coast of Terrasini (Palermo, Italy) and were maintained (while respecting the photo period) in three tanks at the same conditions (20 individuals per tank) containing aerated, filtered, and refrigerated seawater (16 ± 1°C) at the University of Palermo’s aquarium (Dept. STEBICEF). The sea water used was that of the sampling site, and one water change (one third of the volume) was made during the acclimatization period (lasted for a week). The animals were fed ab libitum with pelleted invertebrate food (Azoo, Taikong Corp. Taiwan) until 24 h before the start of the experiments.
Experimental samples
From 50 total individuals maintained in tanks, 9 females and 9 males were selected randomly and identified using gamete emissions to perform the experimental plan. Emissions (eggs and sperm) were induced from each individual using an intracoelomatic injection of 0.5 ml of KCl 0.5M into the peristomial membrane (His et al. Citation1999; Marin et al. Citation2000; Au et al. Citation2001; Volpi Ghirardini et al. Citation2005). The gametes’ sperm and eggs were then sampled separately after a few minutes and washed twice with a marine solution (MS) (CaCl2 12 mM, KCl 11 mM, MgCl2 26 mM, NaCl 0.45 M, Trizma Base 45 mM a pH 7.4). The quality of the gametes was evaluated using a 40X optical microscope, and only completely spherical/normal eggs and sperm with good/excellent motility were employed in the experiments (Fernandez & Beiras Citation2001; Bellas Citation2007).
The gametes were counted using a Neubauer chamber and the formula:
Diclofenac treatment
The DCF solutions used in the experiment were prepared by heat-dissolving the drug (99% purity, Sigma-Aldrich) in a MS. We first assessed the effects of different DCF concentrations on the embryonic development and, subsequently, the eggs and sperm of A. lixula to identify whether there was an impact on fertilisation.
Embryotoxicity were evaluated using eggs and sperm at a 1:8 ratio (1*106/ml eggs and 8*106/ml sperm). These were simultaneously combined on multiwells plates with different DCF solutions at final concentrations of 50 mg/L, 5 mg/L, 0.5 mg/L and 0.05 mg/L (10 mL for each well). The control sample was obtained by combining gametes in a MS. Two type of assessments were carried out to identify the impact of the different concentrations of DCF on early development. The former were performed using an inverted microscope to determine the number of normal embryos, degenerated eggs and unfertilised eggs 3 h after the start of their incubation with the drug. For all experimental concentrations and controls a total of 100 embryos/unfertilized eggs/degenerated eggs were counted and data were expressed as a percentage of each single category (embryos, unfertilized eggs and degenerated eggs). The second type of analyses were conducted to evaluate the levels of DCF accumulated by the embryos. In more detail, the samples were centrifuged at 2200 rpm for 10 min at 4°C and the pellets obtained were washed with a MS and stored at −80°C for the subsequent high-performance liquid chromatography (HPLC) analysis. The evaluations of the DCF-accumulation levels were conducted while respecting the equal weights of all the samples (240 mg). The assessments described were repeated nine times using nine different pairs of animals (nine female and nine male in total as reported above) ().
To evaluate the gametes, eggs and sperm were incubated for 3 h separately with each DCF solution using polystyrene well plates (as reported above). Egg/sperm samples of 1 ml were incubated with 10 ml of each DCF solution; 10 ml of a MS was used for the control. The quantitative analysis was performed using HPLC analysis as described above. The same final pellet weight (240 mg) was used for all the samples in this analysis (corresponding to 3*106 cells/ml for the eggs and 18*109 cells/ml for the sperm). To evaluate the gametes’ accumulation of DCF, this experiment was repeated nine times for the eggs and sperm separately using the same individuals used previously in fertilization analysis (18 individuals in total, nine for eggs and nine for spermatozoa) ().
HPLC analysis
Reagents and standards
Analytical grade (>99% purity) phosphoric acid, perchloric acid and the DCF standard were purchased from Sigma Aldrich (Amsterdam, Holland). All the samples were weighed and mixed with 20 μl of 85% phosphoric acid and 150 μl of 60% perchloric acid, and the solutions were centrifuged at 15,000 rpm for 10 min (room temperature). About 100 μl of supernatant were collected and placed on 150 μl vials for the HPLC analysis. This was conducted on an Agilent 1200 HPLC with an ultraviolet diode array detector (UV/DAD) using a Synergi 4 µm MAX-RP 80A HPLC column (250 x 4.6 mm; Phenomenex, Torrance, California, USA). The instrument conditions are set out in .
Table I. HPLC-UV/DAD instrument conditions
The method used employed an elution obtained after the HPLC column was conditioned in the mobile phase for 1 h. Acetonitrile acid (85:15, v/v) and phosphoric acid (0.01 M) were used as eluents, as per the gradients listed in . The method was validated for its linearity, detection capability (CCβ), trueness of recovery and repeatability according to the European Commission Decision 2002/657/EC. The linearity was calculated in a solution taking into account six concentration points (50–100-200-500-1000 µg/L), with r2 > 0.99. The recovery and repeatability were determined with blank samples spiked at 500 µg/Kg.
Table II. The gradients of the eluents after the HPLC column was conditioned in the mobile phase for 1 h
Statistical analysis
According to Helsel (Citation2005), the samples in which no DCF was detected by the method described above were treated as having half the detection capability (0.025 mg/L) for the purposes of the statistical analysis. The normal distribution of the dataset was tested using the Shapiro-Wilk test (p > 0.05). Wilcoxon rank-sum and t-tests were performed to evaluate differences in the levels of DCF present in the male and female gametic cells (including the controls). The Kruskal-Wallis test was employed to examine differences in the DCF accumulation level of control and treated sperm and egg samples and was also used to evaluate embryonic development (in terms of embryos and degenerated and unfertilised eggs). In particular, the test was employed to verify differences in DCF levels in the controls and the treated samples, as well as during the early stages of their development. An ANOVA test was carried out to identify differences in the amounts of DCF present in the controls and the treated embryos. All the statistical analyses were conducted using the R®3.0.3 software package.
Results
The results in relation to embryotoxicity test () revealed a reduction in successful fertilisation that depended on the concentration of DCF introduced during the incubation period: as the DCF level increased, there was a significant decrease in the number of correctly developed embryos (Kruskal-Wallis chi-squared = 17.318, df = 4, p = 0.002; ) and a significant increase in the number of degenerated eggs (Kruskal-Wallis chi-squared = 22.664, df = 4, p = 0.000; ). However, in comparison to control samples, only DCF concentrations of 50 mg/l led to significant reductions in the number of normal embryos. The number of unfertilised eggs rose as the DCF concentration increased, although these changes were not statistically significant (Kruskal-Wallis chi-squared = 9.126, df = 4, p = 0.058). In relation to the degenerated eggs, there was a significant increase in their number at DCF concentrations of 5 mg/L and 50 mg/L compared to the controls. This was also the case when the samples containing DCF concentrations of 0.05 mg/L and 5 mg/L were compared to those with DCF levels of 50 mg/L.
Figure 2. A, B and C: Box-plots obtained using the Kruskal-Wallis test on the developed embryos and the unfertilised and degenerated eggs (from left to right). The circles represent the outlier; a, b, c: microscopic photographs of the embryos and the unfertilised and degenerated eggs. Scale bar 50 µm. The asterisks represent statistical differences (** p < 0.01, ***p < 0.001).
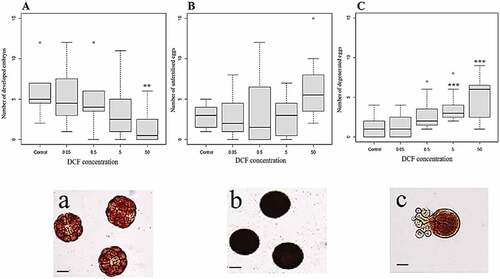
The levels of DCF present in the embryos were evaluated using an HPLC analysis () to verify the actual degree of accumulation. The ANOVA and Tukey post hoc tests confirmed that there was greater and significant accumulation of the drug respect to the control when the highest concentrations were introduced during the incubation period (F value = 7.357; df = 4; p = 0.001). The DCF accumulation was dose-dependent and directly correlated to the exposure concentration. This was significant when the different treatments were compared, in particular when comparing DCF levels of 0.05 (p < 0.01), 0.5 (p < 0.01) and 5 mg/L (p < 0.05) to those of 50 mg/L.
Figure 3. DCF accumulation in embryos. Values are expressed as the mean ± SD. The asterisks represent statistical differences (** p < 0.01).
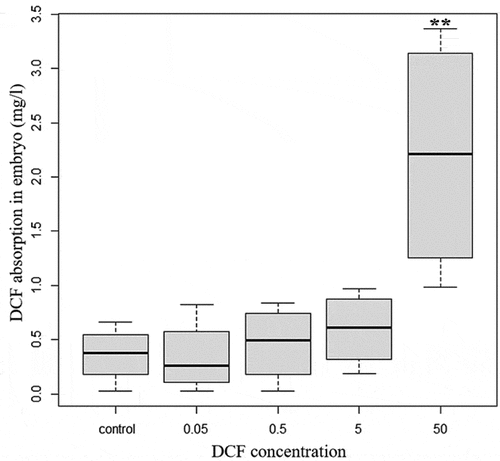
The HPLC analyses of the eggs () and sperm () incubated with different DCF concentrations revealed significant increases (p < 0.05) in accumulation compared to the controls (for eggs: Kruskal-Wallis chi-squared = 29.79, df = 4, p-value = 0.000; for sperm: Kruskal-Wallis chi-squared = 22.094, df = 4, p = 0.000): the accumulation were statistically significant when the eggs were incubated with 50 mg/L of DCF. Moreover, the increase in accumulation was dependent on the concentration of DCF introduced and was significant when levels of 0.05 and 0.5 mg/L were compared to one of 50 mg/L. sets out the mean values of DCF accumulation in egg and sperm samples.
Figure 4. DCF accumulation in A. lixula eggs (A) and sperm (B) measure by HPLC analysis. The circles represent the outlier. Significant accumulation were observed at concentrations over 50 mg/L. The asterisks represent statistical differences (***p < 0.001).
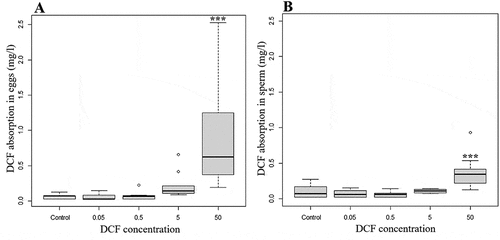
Table III. Concentrations of DCF absorbed in the sperm and egg samples, expressed as the mean ± standard deviation
Discussion
To date, among PhACs, Diclofenac (DCF) is one of the most commonly found NSAIDs worldwide (Bonnefille et al. Citation2018; Ajibola et al. Citation2021; Pap et al. Citation2021). In literature, it is known that DCF has negative effects on marine vertebrate and invertebrate organisms (Bonnefille et al. Citation2018). For this reason, it is important to fully understand its toxicity, environmental risks, and the relationship between its concentrations and effects. Echinoderms are important test species in marine ecotoxicology (Sugni et al. Citation2007) and in light of this, we studied the effects of DCF on the sea urchin A. lixula, focusing on the first phases of the life cycle and gametes, essential for the survival of the species and sensitive to environmental conditions (De Campos et al. Citation2022; Thompson et al. Citation2022; Venâncio et al. Citation2022). We also analysed their bioaccumulation capacity which can influence the biomagnification effect along the trophic chain. All these aspects are important to enlarge the diversity of animal model used for testing the toxicity of DCF. Although DCF concentrations found in seawater are generally below 1 μg/L, the European Union has included DCF in its first checklist of the Water Framework Directive (EU Citation2015/495, European Commission). This is because DCF often enters aquatic environments via Waste Water – treatment Plants (WWTP) inputs, and the extent of its degradation depends on the wastewater and the treatment technology used (Jiang et al. Citation2021; Kiejza et al. Citation2021). Furthermore, WWTP treatment of DCF is also dependent on sunlight, which promotes photolysis (Mestre & Carvalho Citation2019). For all these reasons, the distribution and real concentration of this drug in the aquatic environment is not yet clear. Four concentrations of DCF were used in this study, some even higher than the environmental concentrations.
These were chosen in agreement with other authors who examined the effects at concentrations ranging from a few ng/L to several mg/L, showing that marine organisms have different sensitivities, depending on the species, of the development stage and measured endpoints (reviewed by Bonnefille et al. Citation2018). Moreover, to date, the behaviour and distribution of this drug in aquatic environments are not yet clear, and the evaluation of maximum drug concentrations in an in vitro study could be useful to understand up to which concentration the negative effects are visible and important.
In this study, we demonstrated negative effects on embryos and gametes dependent on DCF concentration, causing a decrease in number of developed embryos and an increase in number of degenerated eggs as shown by other authors in mussels, crustacean, and echinoderms (Riberio et al. Citation2015; Liu et al. Citation2017; Balbi et al. Citation2018). Our results are in agreement with other authors which showed
important effects depending on pollutants (e.g alkylphenols, triorganotin compounds, bisphenol A, polystyrene microbeads, nanoparticles) concentration on A. lixula, P. lividus and Strongylocentrotus intermedius embryos and sperm highlighting closely concentration-dependent damages causing malformations and growth inhibition of embryos in the early life stages (Novelli et al. Citation2002, Citation2003; Arslan & Parlak Citation2007; Özlem & Hatice Citation2008; Messinetti et al. Citation2018; Pikula et al. Citation2020).
Moreover, also in oyster, genotoxic effects of herbicides depending on differences in pollutant sensitivity and affecting the sperm function and the fertilization were observed on embryos and spermatozoa (Akcha et al. Citation2012). The negative effects observed in this study on embryo development are likely due to drug bioaccumulation, which can cause changes in the expression of important genes (e.g., expression of vitellogenin the precursor of the common egg yolk protein vitellin) involved in the detoxification of the metabolism, growth, development, and reproduction (Dennery Citation2007; Liu et al. Citation2017). The observed effects on embryonic development could be due to a bioaccumulation of the drug in the gametes before fertilization. In fact, we showed that the bioaccumulation in gametes (eggs and spermatozoa) was significantly greater at the highest tested concentration. These data are very important because very few studies have analysed the bioaccumulation and have mainly been conducted on bivalves (Ericson et al. Citation2010; Bonnefille et al. Citation2017, Citation2018; Fu et al. Citation2020). Radenac et al. (Citation2001) evaluated the bioaccumulation and the frequencies of abnormalities of four metals in larvae of Paracentrotus lividus highliting in agreement with ours results that the concentrations bioaccumulated were directly dependent to the concentration exposure. In our study, the levels of DCF bioaccumulation at high exposure concentrations could have caused both the increase in the number of degenerate eggs and the decrease in the number of developed embryos as shown by Lewis and Galloway (Citation2009).
The observed increase, even if not significant, in the number of unfertilized eggs could also be due to the accumulation of the drug in the spermatozoa, which is capable of compromising their movement and the acrosome reaction as shown by several authors (Caldwell et al. Citation2004; Du et al. Citation2016; Zanuri et al. Citation2017; Fontes et al. Citation2018). In fact, as observed in the spermatozoa of marine invertebrate species, it is possible that the drug may cause an alteration in the functioning of the ion channels (Espinal-Enríquez et al. Citation2014), the stability of the lysosomal membrane (Fontes et al. Citation2018), the oxidative stress and polyspermia (Gonzalez-Rey & Bebianno Citation2012).
In conclusion, although the toxicity of DCF is well known (Lonappan et al. Citation2016) and the drug is on the EU’s Water Framework Directive’s Watch List of Priority Substances (European Commission Citation2013, Citation2015; Barbosa et al. Citation2016; Tiedeken et al. Citation2017), there are no restrictions on its emission into the sea. To date, the sea urchin embryotoxicity test (ASTM Citation2021: ASTM E1563 – 21 Standard Guide for Conducting Short-Term Chronic Toxicity Tests with Echinoid Embryos, ASTM International, West Conshohocken, PA, 2021) provides a good indication about the acceptability of pollutants dangerous concentrations. Our results represent the first indication of the negative effects of the acute exposure of DCF on sea urchin A. lixula, a marine invertebrate of ecological importance highlighting that analysing the early life stages is a promising approach for assessing the toxicity levels of pharmaceuticals, confirming that the presence of anthropogenic stressors, such as DCF, could compromise their developmental processes and therefore their survival (Hamdoun & Epel Citation2007). Understanding the effects on developing embryos and on the accumulation capacity of the drug by gametes can therefore provide predictive tools to identify potentially harmful compounds and provide indications on safe levels of release of this drug into the sea. All of this confirms the environmental risk of DCF and highlights the need to improve wastewater treatment and drug disposal processes.
Acknowledgements
Thanks go to Dr. Giampaolo Badalamenti for his collaboration in the management of the animals used in the experiments.
Disclosure statement
No potential conflict of interest was reported by the author(s).
Additional information
Funding
References
- Acuña V, Ginebreda A, Mor JR, Petrovic M, Sabater S, Sumpter J, Barceló D. 2015. Balancing the health benefits and environmental risks of pharmaceuticals: Diclofenac as an example. Environment International 85:327–333. DOI: 10.1016/j.envint.2015.09.023.
- Aguirre-Martínez GV, Del Valls TA, Martín-Día ML. 2013. Early responses measured in the brachyuran crab Carcinus maenas exposed to carbamazepine and novobiocin: Application of a 2-tier approach. Ecotoxicology and Environmental Safety 97:47–58. DOI: 10.1016/j.ecoenv.2013.07.002.
- Ajibola AS, Fawole ST, Ajibola FO, Adewuyi GO. 2021. Diclofenac and ibuprofen determination in sewage sludge using a QuEChERS approach: Occurrence and ecological risk assessment in three Nigerian wastewater treatment plants. Bulletin of Environmental Contamination and Toxicology 106(4):690–699. DOI: 10.1007/s00128-021-03139-1.
- Akcha F, Spagnol C, Rouxel J. 2012. Genotoxicity of diuron and glyphosate in oyster spermatozoa and embryos. Aquatic Toxicology 106:104–113. DOI: 10.1016/j.aquatox.2011.10.018.
- Angeles LF, Mullen RA, Huang IJ, Wilson C, Khunjar W, Sirotkin HI, McElroy AE, Aga DS. 2020. Assessing pharmaceutical removal and reduction in toxicity provided by advanced wastewater treatment systems. Environmental Science: Water Research & Technology 6:62–77.
- Ankley GT, Brooks BW, Huggett DB, Sumpter JP. 2007. Repeating history: Pharmaceuticals in the environment. Environmental Science & Technology 41:8211–8217. DOI: 10.1021/es072658j.
- Arslan OC, Parlak HATİCE. 2007. Embryotoxic effects of nonylphenol and octylphenol in sea urchin Arbacia lixula. Ecotoxicology 16(6):439–444. DOI: 10.1007/s10646-007-0147-z.
- ASTM 2021-ASTM E1563-21. Standard Guide for Conducting Short-Term Chronic Toxicity Tests with Echinoid Embryos. https://standards.globalspec.com/std/14376707/astm-e1563-21
- Au DWT, Lee CY, Chan KL, Wu RSS. 2001. Reproductive impairment of sea urchin upon chronic exposure to cadmium. Part I: Effects on gamete quality. Environmental Pollution 111:1–9. DOI: 10.1016/S0269-7491(00)00035-X.
- Balbi T, Montagna M, Fabbri R, Carbone C, Franzellitti S, Fabbri E, Canesi L. 2018. Diclofenac affects early embryo development in the marine bivalve Mytilus galloprovincialis. Science of the Total Environment 642:601–609. DOI: 10.1016/j.scitotenv.2018.06.125.
- Barbosa MO, Moreira NF, Riberio AR, Pereira MF, Silva AM. 2016. Occurrence and removal of organic micropollutants: An overview of the watch list of EU decision 2015/495. Water Research 94:257–279. DOI: 10.1016/j.watres.2016.02.047.
- Bellas J. 2007. Toxicity of the booster biocide Sea-Nine to the early developmental stages of the sea urchin Paracentrotus lividus. Aquatic Toxicology 83:52–61. DOI: 10.1016/j.aquatox.2007.03.011.
- Bonnefille B, Arpin-Pont L, Gomez E, Fenet H, Courant F. 2017. Metabolic profiling identification of metabolites formed in Mediterranean mussels (Mytilus galloprovincialis) after diclofenac exposure. Science of the Total Environment 583:257–268. DOI: 10.1016/j.scitotenv.2017.01.063.
- Bonnefille B, Gomez E, Courant F, Escande A, Fenet H. 2018. Diclofenac in the marine environment: A review of its occurrence and effects. Marine Pollution Bullettin 131:496–506. DOI: 10.1016/j.marpolbul.2018.04.053.
- Branchet P, Arpin-Pont L, Piram A, Boissery P, Wong-Wah-Chung P, Doumenq P. 2021. Pharmaceuticals in the marine environment: What are the present challenges in their monitoring? Science of the Total Environment 766:142644. DOI: 10.1016/j.scitotenv.2020.142644.
- Caldwell GS, Bentley MG, Olive PJW. 2004. First evidence of sperm motility inhibition by the diatom aldehyde 2E, 4E-decadienal. Marine Ecology Progress Series 273:97–108. DOI: 10.3354/meps273097.
- Cammilleri G, Galluzzo P, Pulvirenti A, Giangrosso IE, Lo Dico GM, Montana G, Lampiasi N, Mobilia MA, Lastra A, Vazzana M, Vella A, La Placa P, Macaluso A, Ferrantelli V. 2019. Toxic mineral elements in Mytilus galloprovincialis from Sicilian coasts (Southern Italy). Natural Product Research 34:177–182. DOI: 10.1080/14786419.2019.1610963.
- Cardoso-Vera JD, Islas-Flores H, SanJuan-Reyes N, Montero-Castro EI, Galar-Martínez M, García-Medina S, Elizalde-Velázquez A, Dublán-García O, Gómez-Oliván LM. 2017. Comparative study of diclofenac-induced embryotoxicity and teratogenesis in Xenopus laevis and Lithobates catesbeianus, using the frog embryo teratogenesis assay: Xenopus (FETAX). Science of Total Environment 574:467–475. DOI: 10.1016/j.scitotenv.2016.09.095.
- Chiaramonte M, Arizza V, La Rosa S, Queiroz V, Mauro M, Vazzana M, Inguglia L. 2020. Allograft Inflammatory factor AIF-1: Early immune response in the Mediterranean sea urchin. Paracentrotus lividus Zoology. 142:125815.
- Cortez FS, Pereira CDS, Aldo RSB, Cesar A, Choueri RB, Martini GD, Bohrer-Morel MB. 2012. Biological effects of environmentally relevant concentrations of the pharmaceutical Triclosan in the marine mussel Perna perna (Linnaeus, 1758). Environmental Pollution 168:145–150. DOI: 10.1016/j.envpol.2012.04.024.
- Cunha SC, Pena A, Fernandes JO. 2017. Mussels as bioindicators of diclofenac contamination in coastal environments. Environmental Pollution 225:354–360. DOI: 10.1016/j.envpol.2017.02.061.
- De Campos BG, Do Prado E Silva MBM, Avelelas F, Maia F, Loureiro S, Perina F, de Souza Abessa DM, Martins R. 2022. Toxicity of innovative antifouling additives on an early life stage of the oyster Crassostrea gigas: Short-and long-term exposure effects. Environmental Science and Pollution Research 29:1–14. DOI: 10.1007/s11356-021-17416-3.
- Dennery PA. 2007. Effects of Oxidative Stress on Embryonic Development. Birth Defects Research Part C: Embryo Today: Reviews 81:155–162. DOI: 10.1002/bdrc.20098.
- Desbiolles F, Malleret L, Tiliacos C, Wong-Wah-Chung P, Laffont-Schwob I. 2018. Occurrence and ecotoxicological assessment of pharmaceuticals: Is there a risk for the Mediterranean aquatic environment? Science of the Total Environment 639:1334–1348. DOI: 10.1016/j.scitotenv.2018.04.351.
- Du J, Mei C-F, Ying -G-G, Mei-Y X. 2016. Toxicity thresholds for diclofenac, Acetaminophen and ibuprofen in the water flea Daphnia magna. Bulletin of Environmental Contamination and Toxicology 97:84–90. DOI: 10.1007/s00128-016-1806-7.
- Eades C, Waring CP. 2010. The effects of diclofenac on the physiology of the green shore crab Carcinus maenas. Marine Environmental Research 69:S46–S48. DOI: 10.1016/j.marenvres.2009.11.001.
- Ericson H, Thorsen G, Kumblad L. 2010. Physiological effects of diclofenac, ibuprofen and propranolol on Baltic Sea blue mussels. Aquatic Toxicology 99:223–231. DOI: 10.1016/j.aquatox.2010.04.017.
- Espinal-Enríquez J, Darszon A, Guerrero A, Martínez-Mekler G. 2014. In Silico Determination of the effect of multi-target drugs on calcium dynamics signaling network underlying sea urchin spermatozoa motility. PlosOne 9(8). DOI: 10.1371/journal.pone.0104451.
- EU. 2013. Directive 2013/39/EU of the European Parliament and of the Council of 12 August 2013 amending Directives 2000/60/EC and 2008/105/EC as regards priority substances in the field of water policy. Official Journal of the European Union 226:1–17.
- European Commission. 2013. Directive 2013/39/EU of the European Parliament and of the Council amending Directives 2000/60/EC and 2008/105/EC as regards priority substances in the field of water policy. The Official Journal of the European Union L226:1–17.
- European Union. 2015. Commission Implementing Decision (EU) 2015/495 establishing a watch list of substances for Union-wide monitoring in the field of water policy pursuant to Directive 2008/105/EC of the European Parliament and of the Council. Official Journal of European Union L78:40
- Fabbri E, Franzellitti S. 2016. Human pharmaceuticals in the marine environment: Focus on exposure and biological effects in animal species. Environmental Toxicology and Chemistry 35:799–812. DOI: 10.1002/etc.3131.
- Farré M, Ferrer I, Ginebreda A, Figueras M, Olivella L, Tirapu L, Vilanova M, Barcelo D. 2001. Determination of drugs in surface water and wastewater samples by liquid chromatography-mass spectrometry: Methods and preliminary results including toxicity studies with Vibrio fischeri. Journal of Chromatography. A 938:187–197. DOI: 10.1016/S0021-9673(01)01154-2.
- Fent K, Weston AA, Caminada D. 2006. Ecotoxicology of human pharmaceuticals. Aquatic Toxicology 76(2):122–159. DOI: 10.1016/j.aquatox.2005.09.009.
- Fernandez N, Beiras R. 2001. Combined toxicity of dissolved mercury with copper, lead and cadmium on embryogenesis and early larval growth of the Paracentrotus lividus sea-urchin. Ecotoxicology 10:263–271. DOI: 10.1023/A:1016703116830.
- Fontes MK, Gusso-Choueri PK, Maranho LA, de Souza Abessa DM, Mazur WA, de Campos BG, Guimarães LL, de Toledo MS, Lebre D, Marques JR, Felicio AA, Cesar A, Almeida EA, Pereira CDS. 2018. A tiered approach to assess effects of diclofenac on the brown mussel Perna perna: A contribution to characterize the hazard. Water Research 132:361–370. DOI: 10.1016/j.watres.2017.12.077.
- Fu Q, Fedrizzi D, Kosfeld V, Schlechtriem C, Ganz V, Derrer S, Rentsch D, Hollender J. 2020. Biotransformation changes bioaccumulation and toxicity of diclofenac in aquatic organisms. Environmental Science & Technology 54(7):4400–4408. DOI: 10.1021/acs.est.9b07127.
- Gagné F, Blaise C, Fournier M, Hansen PD. 2006. Effects of selected pharmaceutical products on phagocytic activity in Elliptio ioassay mussels. Comparative Biochemistry and Physiology- Part C 143(2):179–186. DOI: 10.1016/j.cbpc.2006.01.008.
- Gaw S, Thomas KV, Hutchinson TH. 2014. Sources, impacts and trends of pharmaceuticals in the marine and coastal environment. Philosophical Transactions of the Royal Society of London B Biological Sciences 369:20130572.
- Gonzalez-Rey M, Bebianno MJ. 2012. Does non-steroidal anti-inflammatory (NSAID) ibuprofen induce antioxidant stress and endocrine disruption in mussel Mytilus galloprovincialis? Environmental Toxicology and Pharmacology 33:361–371. DOI: 10.1016/j.etap.2011.12.017.
- Gonzalez-Rey M, Tapie N, Le Menach K, Dévier MH, Budzinski H, Bebianno MJ. 2015. Occurrence of pharmaceutical compounds and pesticides in aquatic systems. Marine Pollution Bullettin 96(1–2):384–400. DOI: 10.1016/j.marpolbul.2015.04.029.
- Gröner F, Höhne C, Kleiner W, Kloas W. 2017. Chronic diclofenac exposure affects gill integrity and pituitary gene expression and displays estrogenic activity in Nile tilapia (Oreochromis niloticus). Chemosphere 166:473–481. DOI: 10.1016/j.chemosphere.2016.09.116.
- Hamdoun A, Epel D. 2007. Embryo stability and vulnerability in an always changing world. Proceedings of the National Academy of Sciences 104(6):1745–1750. DOI: 10.1073/pnas.0610108104.
- Heberer T. 2002. Occurrence, fate, and removal of pharmaceuticals residues in the aquatic environment: A review of recent research data. Toxicology Letters 131:5–17. DOI: 10.1016/S0378-4274(02)00041-3.
- Heberer T, Feldmann D. 2005. Contribution of effluents from hospitals and private households to the total loads of diclofenac and carbamazepine in municipal sewage effluents—ioassay versus measurements. Journal of Hazardous Materials 122:211–218. DOI: 10.1016/j.jhazmat.2005.03.007.
- Helsel DR. 2005. Nondetects and data analysis. Statistics for censored environmental data. Hoboken, New Jersey, U.S: Chapter in John Wiley & Sons. pp. 250.
- His E, Heyvang I, Geffard O, De Montaudouin X. 1999. A comparison between oyster (Crassostrea gigas) and sea urchin (Paracentrotus lividus) larval ioassay for toxicological studies. Water Research 33(7):1706–1718. DOI: 10.1016/S0043-1354(98)00381-9.
- Hughes SR, Kay P, Brown LE. 2013. Global synthesis and critical evaluation of pharmaceutical data sets collected from river systems. Environmental Science & Technology 47(2):661–677. DOI: 10.1021/es3030148.
- Inguglia L, Chiaramonte M, Di Stefano V, Schillaci D, Cammilleri G, Pantano L, Mauro M, Vazzana M, Ferrantelli V, Nicolosi R, Arizza V. 2020. Salmo salar fish waste oil: Fatty acids composition and antibacterial activity. PeerJ 8:e9299. DOI: 10.7717/peerj.9299.
- Jiang -Y-Y, Chen Z-W, Li -M-M, Xiang Q-H, Wang -X-X, Miao H-F, Ruan W-Q. 2021. Degradation of diclofenac sodium using Fenton-like technology based on nano-calcium peroxide. Science of the Total Environment 773:144801. DOI: 10.1016/j.scitotenv.2020.144801.
- Jobling S, Casey D, Rodgers-Gray T, Oehlmann J, Schulte-Oehlmann U, Pawlowski S, Baunbeck T, Turner AP, Tyler CR. 2003. Comparative responses of molluscs and fish to environmental estrogens and an estrogenic effluent. Aquatic Toxicology 65:205–220. DOI: 10.1016/S0166-445X(03)00134-6.
- Kiejza D, Kotowska U, Polińska W, Karpińska J. 2021. Peracids – New oxidants in advanced oxidation processes: The use of peracetic acid, peroxymonosulfate, and persulfate salts in the removal of organic micropollutants of emerging concern – A review. Science of the Total Environment 790:148195. DOI: 10.1016/j.scitotenv.2021.148195.
- Lazzara V, Arizza V, Luparello C, Mauro M, Vazzana M. 2019. Bright spots in the darkness of cancer: A review of starfishes-derived compounds and their anti-tumor action. Marine Drugs 17:617. DOI: 10.3390/md17110617.
- Lewis C, Galloway T. 2009. Reproductive consequences of paternal genotoxin exposure in marine invertebrates. Environmental Science & Technology 43:928–933. DOI: 10.1021/es802215d.
- Liu J, Lu G, Xie Z, Zhang Z, Li S, Yan Z. 2015. Occurrence, bioaccumulation and risk assessment of lipophilic pharmaceutically active compounds in the downstream rivers of sewage treatment plants. Science of the Total Environment 511:54–62. DOI: 10.1016/j.scitotenv.2014.12.033.
- Liu Y, Wang L, Pan B, Wang C, Bao S, Nie X. 2017. Toxic effects of diclofenac on life history parameters and the expression of detoxification-related genes in Daphnia magna. Aquatic Toxicology 183:104–113. DOI: 10.1016/j.aquatox.2016.12.020.
- Lonappan L, Brar SK, Das RK, Verma M, Surampalli RY. 2016. Diclofenac and its transformation products: Environmental occurrence and toxicity – A review. Environment International 96:127–138. DOI: 10.1016/j.envint.2016.09.014.
- Luparello C. 2021. Marine Animal-Derived compounds and autophagy modulation in breast cancer cells. Foundations 1:3–20. DOI: 10.3390/foundations1010002.
- Luparello C, Mauro M, Arizza V, Vazzana M. 2020b. Histone deacetylase inhibitors from marine invertebrates. Biology 9(2):429. DOI: 10.3390/biology9120429.
- Luparello C, Mauro M, Lazzara V, Vazzana M. 2020. Collective locomotion of Human cells, wound healing and their control by extracts and isolated compounds from marine invertebrates. Molecules 25(11):2471. DOI: 10.3390/molecules25112471.
- Marin MG, Moschino V, Cima F, Celli C. 2000. Embryotoxicity of butyltin compounds to the sea urchin Paracentrotus lividus. Marine Environmental Research 50(1–5):231–235. DOI: 10.1016/S0141-1136(00)00072-6.
- Matozzo V, Formenti A, Donadello G, Marin MG. 2012. A multi-biomarker approach to assess effects of Triclosan in the clam Ruditapes philippinarum. Marine Environmental Research 74:40–46. DOI: 10.1016/j.marenvres.2011.12.002.
- Mauro M, Lazzara V, Punginelli D, Arizza V, Vazzana M. 2020. Antitumoral compounds from vertebrate sister group: A review of Mediterranean ascidians. Developmental and Comparative Immunology 108:103669. DOI: 10.1016/j.dci.2020.103669.
- Mauro M, Pérez-Arjona I, Belda Perez EJ, Ceraulo M, Bou-Cabo M, Benson T, Espinosa V, Beltrame F, Mazzola S, Vazzana M, Buscaino G. 2020b. The effect of low frequency noise on the behaviour of juvenile Sparus aurata. The Journal of the Acoustical Society of America 147:3795. DOI: 10.1121/10.0001255.
- Mauro M, Queiroz V, Arizza V, Campobello D, Custódio MR, Chiaramonte M, Vazzana M. 2021. Humoral responses during wound healing in Holothuria tubulosa (Gmelin, 1788). Comparative Biochemistry and Physiology – Part B: Biochemistry & Molecular Biology 253:110550. DOI: 10.1016/j.cbpb.2020.110550.
- Messinetti S, Mercurio S, Parolini M, Sugni M, Pennati R. 2018. Effects of polystyrene microplastics on early stages of two marine invertebrates with different feeding strategies. Environmental Pollution 237:1080–1087. DOI: 10.1016/j.envpol.2017.11.030.
- Mestre AS, Carvalho AP. 2019. Photocatalytic degradation of pharmaceuticals carbamazepine, diclofenac, and sulfamethoxazole by semiconductor and carbon materials: A review. Molecules 24(20):3702. DOI: 10.3390/molecules24203702.
- Mezzelani M, Gorbi S, Fattorini D, d’Errico G, Consolandi G, Milan M, Bargelloni L, Regoli F. 2018. Long-term exposure of Mytilus galloprovincialis to diclofenac, Ibuprofen and Ketoprofen: Insights into bioavailability, biomarkers and transcriptomic changes. Chemosphere 198:238–248. DOI: 10.1016/j.chemosphere.2018.01.148.
- Mezzelani M, Regoli F. 2022. The biological effects of pharmaceuticals in the marine environment. Annual Review of Marine Science 14:105–128. DOI: 10.1146/annurev-marine-040821-075606.
- Munari M, Matozzo V, Gagne F, Chemello G, Riedl V, Finos L, Pastore P, Badocco D, Marin MG. 2018. Does exposure to reduced pH and diclofenac induce oxidative stress in marine bivalves? A comparative study with the mussel Mytilus galloprovincialis and the clam Ruditapes philippinarum. Environmental Pollution 240:925–937. DOI: 10.1016/j.envpol.2018.05.005.
- Nantaba F, Wasswa J, Kylin H, Palm W-U, Bouwman H, Kümmerer K. 2020. Occurrence, distribution, and ecotoxicological risk assessment of selected pharmaceutical compounds in water from Lake Victoria, Uganda. Chemosphere 239:124642. DOI: 10.1016/j.chemosphere.2019.124642.
- Novelli AA, Argese E, Tagliapietra D, Bettiol C, Ghirardini AV. 2002. Toxicity of tributyltin and triphenyltin to early life‐stages of Paracentrotus lividus (Echinodermata: Echinoidea). Environmental Toxicology and Chemistry: An International Journal 21(4):859–864.
- Novelli AA, Losso C, Ghetti PF, Ghirardini AV. 2003. Toxicity of heavy metals using sperm cell and embryo toxicity bioassays with Paracentrotus lividus (Echinodermata: Echinoidea): Comparisons with exposure concentrations in the lagoon of Venice, Italy. Environmental Toxicology and Chemistry: An International Journal 22(6):1295–1301. DOI: 10.1002/etc.5620220616.
- Ojemaye C:Y, Petrik L. 2019. Pharmaceuticals in the marine environment: A review. Environmental Reviews 27(2):151–165. DOI: 10.1139/er-2018-0054.
- Özlem ÇA, Hatice P. 2008. Effects of bisphenol A on the embryonic development of sea urchin (Paracentrotus lividus). Environmental Toxicology: An International Journal 23(3):387–392. DOI: 10.1002/tox.20349.
- Pap S, Taggart MA, Shearer L, Li Y, Radovic S, Sekulic MT. 2021. Removal behaviour of NSAIDs from wastewater using a P-functionalised microporous carbon. Chemosphere 264:128439. DOI: 10.1016/j.chemosphere.2020.128439.
- Patel M, Kumar R, Kishor K, Mlsna T, Pittman CU, Mohan D. 2019. Pharmaceuticals of emerging concern in aquatic systems: Chemistry, occurrence, effects, and removal methods. Chemical Reviews 119:3510–3673. DOI: 10.1021/acs.chemrev.8b00299.
- Pikula K, Zakharenko A, Chaika V, Em I, Nikitina A, Avtomonov E, Tregubenko A, Agoshkov A, Mishakov I, Kuznetsov V, Gusev A, Park S, Golokhvast K. 2020. Toxicity of carbon, silicon, and metal-based nanoparticles to sea urchin Strongylocentrotus intermedius. Nanomaterials 10(9):1825. DOI: 10.3390/nano10091825.
- Praveena SM, Mohd Rashid MZ, Mohd Nasir FA, Sze Yee W, Aris AZ. 2019. Occurrence and potential human health risk of pharmaceutical residues in drinking water from Putrajaya (Malaysia). Ecotoxology and Environmental Safety 180:549–556. DOI: 10.1016/j.ecoenv.2019.05.051.
- Quinn B, Schmidt W, O’Rourke K, Hernan R. 2011. Effects of the pharmaceuticals gemfibrozil and diclofenac on biomarker expression in the zebra mussel (Dreissena polymorpha) and their comparison with standardised toxicity tests. Chemosphere 84:657–663. DOI: 10.1016/j.chemosphere.2011.03.033.
- Radenac G, Fichet D, Miramand P. 2001. Bioaccumulation and toxicity of four dissolved metals in Paracentrotus lividus sea-urchin embryo. Marine Environmental Research 51(2):151–166. DOI: 10.1016/S0141-1136(00)00092-1.
- Ribeiro S, Torres T, Martins R, Santos MM. 2015. Toxicity screening of diclofenac, propranolol, sertraline and simvastatin using Danio rerio and Paracentrotus lividus embryo bioassays. Ecotoxology and Environmental Safety 114:67–74. DOI: 10.1016/j.ecoenv.2015.01.008.
- Riva C, Cristoni S, Binelli A. 2012. Effects of triclosan in the freshwater mussel Dreissena polymorpha: A proteomic investigation. Aquatic Toxicology 118:62–71. DOI: 10.1016/j.aquatox.2012.03.013.
- Sathishkumar P, Meena RAA, Palanisami T, Ashokkumar V, Palvannan T, Gu FL. 2020. Occurrence, interactive effects and ecological risk of diclofenac in environmental compartments and biota – A review. Science of the Total Environment 698:134057.
- Schmidt W, O’Rourke K, Hernan R, Quinn B. 2011. Effects of the pharmaceuticals gemfibrozil and diclofenac on the marine mussel (Mytilus spp) and their comparison with standardized toxicity tests. Marine Pollution Bulletin 62:1389–1395. DOI: 10.1016/j.marpolbul.2011.04.043.
- Sehonova P, Svobodova Z, Dolezelova P, Vosmerova P, Faggio C. 2018. Effects of waterborne antidepressants on non-target animals living in the aquatic environment: A review. Science of the Total Environment 631–632:789–794. DOI: 10.1016/j.scitotenv.2018.03.076.
- Sousa JCG, Ribeiro AR, Barbosa MO, Ribeiro C, Tiritan ME, Pereira MFR, Silva AMT. 2019. Monitoring of the 17 EU watch list contaminants of emerging concern in the Ave and the Sousa Rivers. Science of the Total Environment 649:1083–1095. DOI: 10.1016/j.scitotenv.2018.08.309.
- Sugni M, Mozzi D, Barbaglio A, Bonasoro F, Candia Carnevali MD. 2007. Endocrine disrupting compounds and echinoderms: New ecotoxicological sentinels for the marine ecosystem. Ecotoxicology 16:95–108. DOI: 10.1007/s10646-006-0119-8.
- Thompson WA, Shvartsburd Z, Vijayan MM. 2022. Sex-Specific and Long-Term Impacts of Early-Life Venlafaxine Exposure in Zebrafish. Biology 11(2):250. DOI: 10.3390/biology11020250.
- Tiedeken EJ, Tahar A, McHugh B, Rowan NJ. 2017. Monitoring, sources, receptors, and control measures for three European Union watch list substances of emerging concern in receiving waters – A 20 year systematic review. Science of the Total Environment 574:1140–1163.
- Tran NH, Reinhard M, Gin KYH. 2018. Occurrence and fate of emerging contaminants in municipal wastewater treatment plants from different geographical regions-a review. Water Research 133:182–207. DOI: 10.1016/j.watres.2017.12.029.
- Triebskorn R, Casper H, Heyd A, Eikemper R, Köhler H-R, Schwaiger J. 2004. Toxic effects of the non-steroidal anti-inflammatory drug diclofenac. Part I: Histopathological alterations and bioaccumulation in rainbow trout. Aquatic Toxicology 68(2):151–166. DOI: 10.1016/j.aquatox.2004.03.015.
- Van den Brandhof E-J, Montforts M. 2010. Fish embryotoxicity of carbamazepine, diclofenac and metoprolol. Ecotoxicology and Environmental Safety 73(8): 1862–6.
- Vazzana M, Maria Ceraulo Mauro M, Papale E, Dioguardi M, Mazzola S, Arizza V, Chiaramonte M, Buscaino G. 2020b. Effects of acoustic stimulation on biochemical parameters in the digestive gland of Mediterranean mussel Mytilus galloprovincialis (Lamark, 1819). The Journal of the Acoustical Society of America 147:4. DOI: 10.1121/10.0001034.
- Vazzana M, Mauro M, Ceraulo M, Dioguardi M, Papale E, Mazzola S, Arizza V, Beltrame F, Inguglia L, Buscaino G. 2020a. Underwater high frequency noise: Biological responses in sea urchin Arbacia lixula (Linnaeus, 1758). Comparative Biochemistry and Physiology – Part A: Molecular & Integrative Physiology 242:110650. DOI: 10.1016/j.cbpa.2020.110650.
- Venâncio C, Melnic I, Tamayo-Belda M, Oliveira M, Martins MA, Lopes I. 2022. Polymethylmethacrylate nanoplastics can cause developmental malformations in early life stages of Xenopus laevis. Science of the Total Environment 806(1):150491. DOI: 10.1016/j.scitotenv.2021.150491.
- Vieno N, Sillanpää M. 2014. Fate of diclofenac in municipal wastewater treatment plant - a review. Environment International 69:28–39. DOI: 10.1016/j.envint.2014.03.021.
- Volpi Ghirardini A, Arizzi Novelli A, Tagliapietra D. 2005. Sediment toxicity assessment in the Lagoon of Venice (Italy) using Paracentrotus lividus (Echinodermata: Echinoidea) fertilization and embryo bioassays. Environment International 3:1065–1077. DOI: 10.1016/j.envint.2005.05.017.
- Wang L, Ying GG, Zhao JL, Yang XB, Chen F, Tao R, Liu S, Zhou LJ. 2010. Occurrence and risk assessment of acidic pharmaceuticals in the Yellow River, Hai river and Liao river of north China. Science of the Total Environment 408:3139–3147. DOI: 10.1016/j.scitotenv.2010.04.047.
- Xie Z, Lu G, Liu J, Yan Z, Ma ZZ, Chen W. 2015. Occurrence, bioaccumulation, and trophic magnification of pharmaceutically active compounds in Taihu Lake, China. Chemosphere 138:140–147. DOI: 10.1016/j.chemosphere.2015.05.086.
- Xie Z, Lu G, Yan Z, Liu J, Wang P, Wang Y. 2017. Bioaccumulation and trophic transfer of pharmaceuticals in food webs from a large freshwater lake. Environmental Pollution 222:356–366. DOI: 10.1016/j.envpol.2016.12.026.
- Zanuri NBM, Bentley MG, Caldwell GS. 2017. Assessing the impact of diclofenac, ibuprofen and sildenafil citrate (Viagra®) on the fertilisation biology of broadcast spawning marine invertebrates. Marine Environmental Research 127:126–136. DOI: 10.1016/j.marenvres.2017.04.005.
- Zhang Y, Geissen SU, Gal C. 2008. Carbamazepine and diclofenac: Removal in wastewater treatment plants and occurrence in water bodies. Chemosphere 73(8):1151–1161. DOI: 10.1016/j.chemosphere.2008.07.086.
- Zhang K, Yuan G, Werdich AA, Zhao Y. 2020. Ibuprofen and diclofenac impair the cardiovascular development of zebrafish (Danio rerio) at low concentrations. Environmental Pollution 258:113613. DOI: 10.1016/j.envpol.2019.113613.
- Zuccato E, Calamari D, Natangelo M, Fanelli R. 2000. Presence of therapeutic drugs in the environment. The Lancet 355(9217):1789–1790. DOI: 10.1016/S0140-6736(00)02270-4.