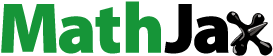
Abstract
Native to the Gulf of Mexico, Gulf wedge clam (Rangia cuneata) has been successfully invading Baltic Sea since first introduction with ballast waters in 2010. In this study, we report new occurrence sites in the Pomeranian Bay and in the brackish water of the Szczecin Lagoon. We aimed to describe the structure and stability of the new population of Gulf wedge clam using ecological indicators (i.e. condition index, length-at-age) and a molecular approach targeting first cytochrome oxidase subunit (COX1) fragments. The study showed an age structure dominated by adult clams (aged 4+), rather than the often reported juveniles. We found that the condition of the R. cuneata population in the Pomeranian Bay is characteristic of the expansion model after bottleneck. Genetic analyses indicated low nucleotide (π = 0.00387 ± 0.00012) and haplotype (h = 0.79 ± 0.018) diversity. A haplotype network inferred by a median-joining method showed lack of haplotype H6 (Chesapeake Bay, NW Atlantic) and numerous individuals with haplotype H1 (Gulf of Mexico). Demographic parameters (non-significant Tajima’s D = 2.058, Fu’s Fs = 2.298, Fu and Li’s D* = 1.195, and significant Fu and Li’s F test = 1.757) suggested that the population underwent a moderate population bottleneck, while analysis of mismatch distribution showed a bimodal shape which may imply population admixture as a consequence of “allelic surfing”. Moreover, we concluded that R. cuneata has successfully invaded the basin of the LNG terminal, although our study proved non-linear growth of this species.
1. Introduction
Native to the Gulf of Mexico Gulf wedge clam (Rangia cuneata, G.B. Sowerby I 1832) continues invasion around European waters, where presumably it has been introduced with ballast waters discharge (Verween et al. Citation2006). Kemp et al. (Citation2018) revealed that Gulf wedge clam has a high invader impact (RIP, Relative Impact Potential) and a propensity to drive ecosystem changes, thus causing threat to the native aquatic fauna, especially benthic species. As an invasive alien species, Gulf wedge clam has adapted very well to the environmental conditions prevailing in the European waters. The species inhabits shallow areas less than 6 m deep, prefers low salinity level (1 to 15‰), high turbidity and soft bottom composed of sand, silt, mud and vegetation (Guillen et al. Citation2016). However, Gulf wedge clams have been found as brackish water organisms that tolerate salinity between 0 and 33‰, and have been observed in freshwater bodies of Great Britain (Willing Citation2015). Tang et al. (Citation2019) reported appearance of Gulf wedge clam at the interface between freshwater and brackish water (1.02‰), but its population density was very low (3.5 ± 1.2 individuals per m2). Furthermore, Gulf wedge clams have developed the ability to survive approx. 2 weeks (at 22°C) under anaerobic conditions, which is a considerable advantage over other species (Hopkins et al. Citation1974). Expansion risk is also increased because they are less exposed to predatory attacks due to their shells being thicker and harder than those of native clams (Wrzecionkowski pers. comm.). Additionally, the r-type reproductive strategy and year-round spawn of Gulf wedge clams constitute a massive threat to the native benthic species (LaSalle & De la Cruz Citation1985), thus the species may have an immense potential to overpopulate local species such as Baltic clam (Macoma balthica), sand gaper (Mya arenaria), blue mussel (Mytilus edulis) or Common cockle (Cerastoderma edule). Therefore, to early detect further invasions, an eDNA-based tool has been developed to enable rapid management responses and tracking of the invasion dynamics (Ardura et al. Citation2015).
According to the available scientific literature, Gulf wedge clam reached Baltic Sea in 2010, when it was first discovered de visu in the eastern areas of the Vistula Lagoon in Russia (, Rudinskaya & Gusev Citation2012). Soon afterwards, it was found on the other side of the Vistula Lagoon (Poland), but its size range (30–40 mm) suggested introduction 2–3 years earlier, which is ca. same time as in Russia (Warzocha & Drgas Citation2013). Subsequently, the presence of this alien invasive species was reported in the port of Rotterdam (Gittenberger et al. Citation2015) and in the channel connecting the North Sea and the Baltic Sea, with entry in Brunsbüttel and around Lübeck (Wiese et al. Citation2016). Recently, Gulf wedge clam has invaded the Pärnu Bay in Estonia (Möller & Kotta Citation2017) and the Curonian Lagoon in Lithuania (Solovjova Citation2014; Solovjova et al. Citation2019). Despite many occurrence reports, little is known about the genetic diversity and origin of Gulf wedge clam in the Baltic Sea. Voroshilova et al. (Citation2018) revealed a high genetic diversity of Gulf wedge clam in the Vistula Lagoon (6 haplotypes in 45 samples) and predicted further invasive spread across the Baltic Sea coastal waters.
Table I. Chronology and sites of occurrence of wedge clam (Rangia cuneata) in the Baltic Sea
Therefore, the aim of this study was to estimate introduction time, based on the age and size of the collected clams, but also to assess the genetic stability of the Rangia cuneata population observed in a new site in the Polish part of the Pomeranian Bay. A further aim was to establish the possibility of further spread of R. cuneata along the Baltic Sea coastal waters, including neighbouring rivers and lakes in which individuals may inhabit brackish water niches and drive significant ecosystem changes.
2. Materials and methods
2.1. Observations and sample collection
First Gulf wedge clam observation in the Polish part of the Pomeranian Bay was incidental, on 8th of October 2018, a half of shell was found by a WWF Poland Blue Patrol volunteer on the beach in Świnoujście (). Thereafter, empty shells and live clams were found and collected inside the LNG terminal on 4th of February 2019. Since then, Gulf wedge clam observations were reported at 4 sites within a 15-km range from the first finding. To illustrate and summarise spread across the Baltic Sea (, ), and particularly in the Pomeranian Bay (), a map with occurrence sites was developed using the QGIS 3.12 software (QGIS Development Team, available from https://qgis.org/en/site/). In total, 405 live Gulf wedge clams were collected by hand and adjustable sampling rakes inside the LNG terminal in Świnoujście from the shoreline, secured in plastic bags and transported to laboratory for further analyses.
2.2. Size structure, morphology and condition
To determine the population structure, the length (the maximum distance along the anterior–posterior axis) and weight of all collected individuals were measured using an electronic calliper (±0.01 mm) and an electronic scale (±0.01 g), respectively. Simultaneously, the age of Gulf wedge clams was determined based on growth increments in accordance with other studies of annulus in freshwater bivalves (Aldridge Citation1999; Tang et al. Citation2019). Since Gulf wedge clam in the Pomeranian Bay is not exposed to tidal cycles, distinct increments on the shells were assumed as annual bands. Based on the obtained measurements, size-at-age was estimated, and all calculated data were visualised using ggplot2 (Wickham Citation2016). Flesh and shells from ten individuals (n = 10) were dried at 105°C until constant weight to asses dry weight. Subsequently, Condition Index (CI) was calculated with the following formula, according to Walne (Citation1976):
2.3. DNA extraction, PCR assay and genetic diversity assessment
From 98 randomly selected individuals, a piece of muscle tissue was collected, and total DNA extracted using the High Pure PCR Template Preparation Kit (Roche, Switzerland). Quality and quantity of DNA isolates were assessed by separation in 1.5% agarose gel and spectrophotometric measurements using a NanoDrop 2000 (Thermo Scientific, USA). Species-specific DNA barcodes were analysed based on amplification of the first cytochrome oxidase subunit (COX1) fragments, according to the method described by Machordom et al. (Citation2003). The following primers were used for amplification: LCO1490 5’-GGTCAACAAATCATAAAGATATTGG-3’ and HCO2198 5’-TCAGGGTGACCAAAAAATCA-3’. Amplifications were conducted on Mastercycler (Eppendorf, Germany) using the GoTaq PCR kit (Promega, USA), including 5 μl of Green GoTaq® Flexi Buffer, 2.5 μl of MgCl2 (25 mM Solution), 0.5 μl of PCR Nucleotide Mix (10 mM), 0.125 μl of GoTaq® DNA Polymerase (5 u/µl), 0.5 μM of each primer and 2 μl of DNA template in final volume of 25 μl. PCR products were assessed by 2% gel electrophoresis and bidirectionally sequenced by Genomed company (Warsaw). Raw sequences were processed, aligned and checked using the sequence editing software Geneious v.11.1.5 (created by Biomatters, available from http://www.geneious.com). Additionally, all sequences were translated into amino acids to confirm the accuracy of the sequences and to detect the presence of nuclear DNA pseudogenes, insertions, deletions or stop codons. Finally, all COX1 sequences were searched for matches against the GeneBank database using BLASTn to confirm their identity.
2.4. Phylogenetic analyses
Neighbour-joining (NJ) phylogenetic analyses were carried out using Geneious v.11.1.5 with evolutionary model parameters as estimated by the Akaike Information Criterion (AIC) of MEGA X (Kumar et al. Citation2018). Phylogenetic analyses incorporated published sequences from R. cuneata species, with Corbula amurensias (KJ522951), Coelomactra antiquata (HQ009290) and Acanthocardia echinate (MG935355) as outgroups. NJ analyses were then performed with a TN93 + I model for COX1 as selected by MEGAX (base frequencies A = 0.227, C = 0.134, G = 0.204, T = 0.434 and proportion of invariant sites: 0.37). Support for each node was assessed by bootstrapping, with heuristic analysis of 10,000 replicate datasets. DNA diversity metrics (number of variable sites – S, nucleotide diversity – π, haplotype diversity – h, neutrality tests – Tajima’s D, Fu’s Fs, Fu and Li’s D* and Fu and Li’s F, sum of squared differences – SSD, Ramos–Onsins and Rozas R2 statistic – R2, raggedness statistic – rg) and nucleotide site differences (mismatch distribution) were calculated with DnaSP v.6.12.03 (Rozas et al. Citation2017). The goodness of fit tests for a model of population expansion (sum of squared deviation, SSD; raggedness index, r; 10,000 replicates) were calculated in ARLEQUIN v.3.5.1.3 (Excoffier et al. Citation2005). The spatial distribution of haplotypes was constructed using a median joining (MJ) implemented in the NETWORK v.10.0.0.0 (Fluxus Technology Ltd., Suffolk, UK).
3. Results and discussion
3.1. Age structure, growth dynamics and condition index
The study presents first occurrence of Rangia cuneata in the Polish waters of the Pomeranian Bay and in the Szczecin Lagoon (), since presumably Gulf wedge clam was indirectly dragged with ballast waters to Europe, presumably as larvae (Verween et al. Citation2006). Such type of introduction is most probable for non-native clams spread worldwide (Drake & Lodge Citation2004; Endresen et al. Citation2004). However, despite the ongoing ballast water management programs, adult molluscs (different species sized 11–22 mm) have also been identified in ballast tanks (Briski et al. Citation2012).
In the study the age structure of the Gulf wedge clam population inside the LNG terminal ranged from 1+ to 8+ years. The population was dominated by adult clams (4+ years old – 52.59%), with a shell length of 36 to 38 mm (27.16%) and an average length of 34.53 ± 5.71 mm (). Moreover, analysis of length-at-age showed non-linear growth model (). The size and age structures of Gulf wedge clam revealed in our study were similar to the findings by Tang et al. (Citation2019) in saline water, and contrary to the often reported population structures of Gulf wedge clam dominated by juveniles (Janas et al. Citation2014; Solovjova et al. Citation2019). Hence, the structure of the Gulf wedge clam population is a result of abiotic and biotic factors which shape the image of the community. For instance, Poirrier and Caputo (Citation2015) showed that the presence of small individuals abruptly decreases when salinity stress occurs. Moreover, salinity and water temperature influence the spawning efficiency and survival of larvae (Cain Citation1973). The latter factor, that could influence the population structure, is associated with the susceptibility of smaller clams to predation (Ebersole & Kennedy Citation1994; Kornijów et al. Citation2018). The age structure did not clearly indicate the exact time of introduction. The highest number of Gulf wedge clams aged 4+ could indicate an estimated introduction of larvae 4 years back, c.a. 2014. However, first Q-flex class gas carriers with ballast tanks entered the LNG terminal in Świnoujście only 3 years earlier, on 11th December 2015, when “Al Nuaman” delivered gas from Qatar (Hajduk Citation2017). Furthermore, we found Gulf wedge clams aged even 8+, so it is uncertain whether Gulf wedge clam was introduced earlier or as an adult form with first LNG deliveries. Moreover, our results of the Gulf wedge clam growth dynamics are consistent with previous reports on non-linear growth model of Gulf wedge clam in brackish and saline water (Wolfe & Petteway Citation1968; Tang et al. Citation2019).
Figure 2. Age frequency distribution of live Rangia cuneata specimens collected from the Pomeranian Bay.
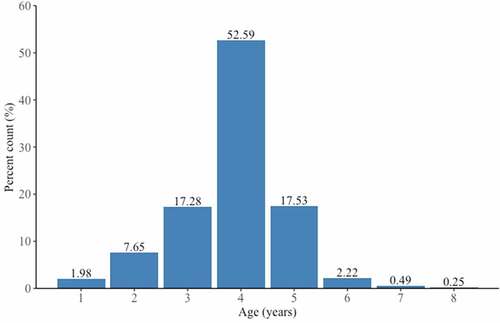
Figure 3. Length frequency distribution of live Rangia cuneata specimens collected from the Pomeranian Bay. Vertical dashed line represents mean shell length in the dataset.
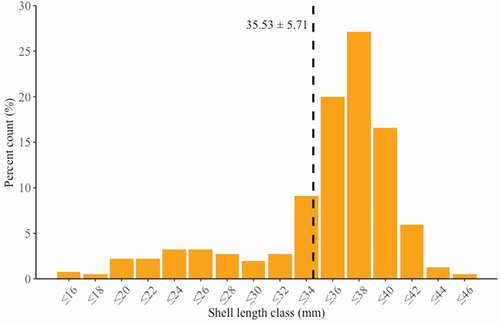
Figure 4. Length-at-age curve of Rangia cuneata collected from the Pomeranian Bay. Line represent fitted curve for the sampling site. Shaded areas represent 99% confidence intervals.
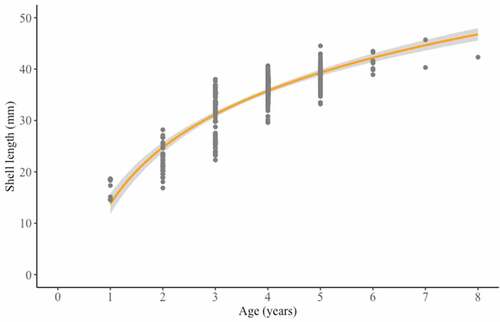
In our study CI of the Gulf wedge clam amounted to 4.39 ± 1.08. This result was comparable to the CI of Gulf wedge clam found in Lake Salvador (4.33 ± 0.52), where bottom sediments consisted mainly of sand (Wong et al. Citation2010). Such environmental conditions were also identified in our case, since the LNG terminal was built on a sandy coast, which is favourable for Gulf wedge clam (Tenore et al. Citation1968).
3.2. Population genetics and demographic parameters
In our study, alignment of 98 COX1 sequences was obtained and characterised by a low nucleotide diversity (π = 0.00387 ± 0.00012) and 7 parsimony informative sites. Within the set of the aligned sequences, seven haplotypes were identified, of which four had been previously described in the Vistula Lagoon of the Baltic Sea by Voroshilova et al. (Citation2018), i.e. H1 (GenBank accession no. MG267050), H2 (MG267051), H3 (MG267052) and H4 (MG267053) with the frequencies of 28%, 17%, 14% and 30%, respectively. Three other haplotypes were identified in our study for the first time, i.e. Pl_Hap_01 (MT304931), Pl_Hap_02 (MT304932) and Pl_Hap_03 (MT304933) with the frequencies of 5%, 3% and 3%, respectively. In a comparison of the haplotype diversity of the Gulf wedge clam populations from the Polish (h = 0.684 ± 0.096) and Russian (h = 0.787 ± 0.043) parts of the Vistula Lagoon with those in the Pomeranian Bay, a slightly higher value was observed (h = 0.79 ± 0.018). In our study, the low nucleotide diversity values and the high haplotype diversity suggested small differences between haplotypes. This was also confirmed by the haplotype network, which represents mostly single-nucleotide differences between the identified haplotypes (). This situation can be a signature of a rapid population expansion from a small effective (bottlenecked) population size as observed in the study of lagoon cockle (Cerastoderma glaucum) inside the Mar Menor coastal lagoon in Spain (Avise Citation2000; Vergara-Chen et al. Citation2013). Populations regarded as stable are usually characterised by the concurrent high haplotype and nucleotide diversity as shown for Mactra corallina from the Tunisian coast (Chetoui et al. Citation2016). Voroshilova et al. (Citation2018) showed also that Gulf wedge clams of haplotype H1 were the most frequent in the Vistula Lagoon and originated from the Gulf of Mexico. In our study, the two most observed haplotypes were H1 and H4, while haplotype H6 noted in the R. cuneata population from the Chesapeake Bay (Atlantic coast of the United States) was absent (). Haplotype H1 was most probably an ancestral haplotype. However, to decipher translocation routes and spatial distribution of R. cuneata individuals in the coastal areas of the Baltic Sea, detailed information concerning the genetic structure of the Gulf wedge clam populations inhabiting native ranges in the United States and in the established settlements across Europe is needed.
Figure 5. Haplotype network (A) and phylogenic neighbour-joining tree (B) of Rangia cuneata based on mitochondrial COI sequences.
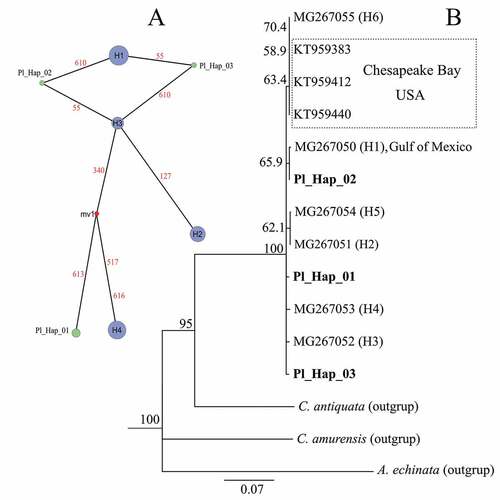
Molecular analysis showed large positive non-significant values of Tajima’s D (2.058), Fu’s Fs (2.298) and Fu and Li’s D* (1.195) neutrality tests, but also the positive significant value of Fu and Li’s F test (1.757, P < 0.05) for the collected Gulf wedge clams. Moreover, divergence based on the number of segregating sites amounted to θ = 0.00206. The Fs, D* and F indices suggest that the population underwent a moderate population bottleneck (population contraction) or its alleles are under balancing selection (Schneider and Excoffier Citation1999). Similarly, relatively low frequency of rare alleles and positive values of neutrality tests were observed for populations of Brachidontes pharaonis (Terranova et al. Citation2006) and Mytella charruana (Gillis et al. Citation2009) – two invasive mussel species that have successfully colonised areas outside their native range. In our study, divergence based on the number of segregating sites was lower than the average nucleotide diversity (π = 0.00387), which additionally indicates either balancing selection or admixture of two R. cuneata populations characterised by different genetic backgrounds (Rand Citation1996; Weigand & Leese Citation2018).
Analysis of mismatch distribution plot for the Gulf wedge clam population in the Pomeranian Bay showed a bimodal shape () with low fitness to the estimated allele frequency under a population expansion model (Harpending’s raggedness index: r = 0.05799, p = 0.35; Mean Absolute Error: 0.4522; SSD = 0.01792, p = 0.20). This pattern is indicative of populations at a demographic equilibrium, but also implies that they may have experienced secondary contact (population admixture) as described for the invasive marine mussel Mytella charruana (Tajima Citation1989; Harpending Citation1994; Rogers Citation1995; Gillis et al. Citation2009). Gulf wedge clam, as other numerous intertidal mollusc species (peppery furrow shell, Scrobicularia plantain; Atlantic surf clam, Spisula solidissima; dwarf surf clam, Mulinia lateralis), include in their life history planktonic larval stages of a considerable (7–20 days) duration (Mann et al. Citation1991; Santos et al. Citation2012). Planktonic development enables a successful spread of R. cuneata glochidia over further intertidal areas of the Baltic Sea by regional currents. This recurring stepwise mode of expansion ensures constant modifications (updates) of allelic/haplotypic richness in local populations which were established by variable numbers of founding individuals from potentially different source populations. This situation called “allelic surfing” encourages stochastic evolution through processes such as genetic drift, resulting in low genetic diversity as shown for the R. cuneata populations in the Pomeranian Bay (Riquet et al. Citation2013). Moreover, as described by Dlugosch and Parker (Citation2008), human-introduced populations often have discrete (but potentially numerous and repetitious) introductory sites, which results is an amalgamation of subpopulations.
3.3. Assessment of potential of R. cuneata range expansion along the Polish Baltic coast including neighbouring rivers and lakes
Gulf wedge clam is a typical estuarine brackish species characterised by numerous biological features predisposing it to constantly colonise new marine and brackish areas, but also to concomitantly expand its range of occurrence in neighbouring freshwater bodies. The population in the Pomeranian Bay has mainly two possible directions of expansion, i.e. upwards of the estuary of the Oder River and laterally along the southern coast of the Baltic Sea. The first direction allows a surge in the population size in the area in which environmental conditions are constantly shaped by high riverine nutrient load delivered by freshwater runoff from the Oder River catchment area, but also by the water exchange with the open sea (Friedland et al. Citation2019). The estuary of the Oder River, with the Szczecin Lagoon as a main part, has an optimal salinity level (1–3‰) and a high nutrient richness for the R. cuneata growth and development. Therefore, this species successively has increased the size of its geographical reach since the first occurrence in 2018 (). Similar observations were made from the monitoring of the R. cuneata spread in the area of the Trinity River delta and associated bayous and sub-bays (Guillen et al. Citation2016). The authors of the study also showed that at lower salinity (< 3‰), R. cuneata significantly increased its meat index (up to 30.3%) compared with that in high salinity conditions (~10‰, meat index ~12%). The observed discrepancy between the levels of this basic health index was attributed to the reduced stressful conditions observed in lower salinity conditions. In our opinion the rate and range of the Gulf wedge clam expansion in the estuary of the Oder River might also be strongly facilitated by the lack of natural predators, no harvesting for human consumption, and high nutrient load that subsequently influences primary production (Friedland et al. Citation2019). Eutrophication observed in the Szczecin Lagoon combined with an extremely high filtration rate suggests that R. cuneata will continue its successful colonisation of brackish recipient ecosystems (Tang et al. Citation2019) and may exert a negative impact (overperformance) on local clam populations, e.g., duck mussel, Anodonta anatina (Kemp et al. Citation2018). The lateral expansion (second direction) of Gulf wedge clam along the southern coast of the Baltic Sea might be additionally magnified by currents which may translocate planktonic larvae to coastal bays and lagoons that are suggested to have the most suitable conditions for colonisation (Solovjova et al. Citation2019). The Polish part of the Baltic Sea coast is scattered with 55 harbours/ports (e.g., Władysławowo, Gdynia) including those located at river openings (e.g., Mrzeżyno, Darłowo, Ustka), 9 main lakes (e.g., Jamno Lake, Resko Przymorskie Lake) that permanently or temporally exchange water with the open Baltic Sea, and several smaller rivers (Czerwona River, Piaśnica River) entering the Baltic Sea (Ecotec Research & Consulting Citation2006). These areas may shortly become new temporary “home” for further expansions of the species, which should be monitored in further studies.
Acknowledgements
This study was funded by the Department of Meat Sciences and the Department of Aquatic Bioengineering and Aquaculture of the ZUT in Szczecin. We would like to thank Prof. Małgorzata Sobczak for her assistance during R. cuneata sampling.
Disclosure statement
No potential conflict of interest was reported by the author(s).
References
- Aldridge DC. 1999. The morphology, growth and reproduction of unionidae (bivalvia) in a fenland waterway. Journal of Molluscan Studies 65(1):47–60. DOI:10.1093/mollus/65.1.47.
- Ardura A, Zaiko A, Martinez JL, Samulioviene A, Semenova A, Garcia-Vazquez E. 2015. eDNA and specific primers for early detection of invasive species – A case study on the bivalve Rangia cuneata, currently spreading in Europe. Marine Environmental Research 112:48–55. DOI:10.1016/j.marenvres.2015.09.013.
- Avise JC. 2000. Phylogeography: The history and formation of species. Cambridge: Harvard University Press. pp. 447.
- Bock G, Lieberum C, Schütt R, Wiese V. 2015. Erstfund der Brackwassermuschel Rangia cuneata in Deutschland (Bivalvia: Mactridae). Schr Malakozool Haus Nat Cisma 28:13–16.
- Briski E, Ghabooli S, Bailey SA, MacIsaac HJ. 2012. Invasion risk posed by macroinvertebrates transported in ships’ ballast tanks. Biological Invasions 14(9):1843–1850. DOI:10.1007/s10530-012-0194-0.
- Cain TD. 1973. The combined effects of temperature and salinity on embryos and larvae of the clam Rangia cuneata. Marine Biology 21(1):1–6. DOI:10.1007/BF00351185.
- Chetoui I, Denis F, Boussaid M, Telahigue K, El Cafsi M. 2016. Genetic diversity and phylogenetic analysis of two Tunisian bivalves (Mactridae) Mactra corallina (Linnaeus, 1758) and Eastonia rugosa (Helbling, 1799) based on COI gene sequences. Comptes Rendus Biologies 339(3–4):115–122. DOI:10.1016/j.crvi.2016.02.001.
- Dlugosch KM, Parker IM. 2008. Founding events in species invasions: Genetic variation, adaptive evolution, and the role of multiple introductions. Molecular Ecology 17(1):431–449. DOI:10.1111/j.1365-294X.2007.03538.x.
- Drake JM, Lodge DM. 2004. Global hot spots of biological invasions: Evaluating options for ballast–water management. Proceedings of the Royal Society of London. Series B: Biological Sciences 271(1539):575–580. DOI:10.1098/rspb.2003.2629.
- Ebersole EL, Kennedy VS. 1994. Size Selection of Atlantic Rangia Clams, Rangia cuneata, by Blue Crabs, Callinectes sapidus. Callinectes Sapidus. Estuaries 17(3):668–673. DOI:10.2307/1352414.
- Ecotec Research & Consulting (2006) An exhaustive analysis of employment trends in all sectors related to the sea or using sea resources. Country report-Poland. Birmingham, UK. Priestley House. https://ec.europa.eu/maritimeaffairs/sites/maritimeaffairs/files/docs/body/poland_employment_trends_en.pdf. Accessed 5 February 2020.
- Endresen Ø, Behrens HL, Brynestad S, Andersen AB, Skjong R. 2004. Challenges in global ballast water management. Marine Pollution Bulletin 48(7–8):615–623. DOI:10.1016/j.marpolbul.2004.01.016.
- Excoffier L, Laval G, Schneider S. 2005. Arlequin (version 3.0): An integrated software package for population genetics data analysis. Evolutionary Bioinformatics 1:47–50. DOI: 10.1177/117693430500100003.
- Florin AB (2017) Rangia cuneata introduction to Sweden/Baltic Sea. In: AquaNIS Editorial Board (Eds.), Information System on Aquatic Non-indigenous and Cryptogenic Species. World Wide Web Electronic Publication. www.corpi.ku.lt/databases/aquanis. Version 2.36+. Accessed 06 February 2020.
- Friedland R, Schernewski G, Gräwe U, Greipsland I, Palazzo D, Pastuszak M. 2019. Managing eutrophication in the szczecin (Oder) lagoon-development, present state and future perspectives. Frontiers in Marine Science 5:521. DOI: 10.3389/fmars.2018.00521.
- Gillis NK, Walters LJ, Fernandes FC, Hoffman EA. 2009. Higher genetic diversity in introduced than in native populations of the mussel Mytella charruana: Evidence of population admixture at introduction sites. Diversity and Distributions 15(5):784–795. DOI:10.1111/j.1472-4642.2009.00591.x.
- Gittenberger A, Rensing M, Gittenberger E. 2015. Rangia cuneata (Bivalvia, Mactridae) expanding its range in The Netherlands. Basteria 78(4–6):58–62.
- Guillen G, Gordon A, Oakley J, Mokrech M (2016) Trinity River delta and upper Trinity Bay rangia population assessment. Final Report 16-001. https://www.uhcl.edu/environmental-institute/research/publications/documents/16-001rangia-study-final-report.pdf. Accessed 5 February 2020.
- Hajduk J. 2017. An analysis of the LNG tanker Al Nuaman’s speeds during its first voyage to the LNG terminal in Świnoujście (route sections Arkona–Świnoujście–Arkona). Sci J Marit Univ Szczec 53(124):74–81. DOI:10.17402/247.
- Harpending HC. 1994. Signature of ancient population growth in a low-resolution mitochondrial DNA mismatch distribution. Human Biology 66(4):591–600.
- Hopkins SH, Anderson JW, Horvath K. 1974. Biology of the clam Rangia cuneata; what we know and what it means. Proc Natl Shellfish Ass 64:4.
- Janas U, Kendzierska H, Dąbrowska AH, Dziubińska A. 2014. Non-indigenous bivalve — The Atlantic rangia Rangia cuneata — In the Wisła Śmiała River (coastal waters of the Gulf of Gdańsk, the southern Baltic Sea). Oceanological and Hydrobiological Studies 43(4):427–430. DOI:10.2478/s13545-014-0158-3.
- Kemp JS, Tang F, Aldridge DC. 2018. Quantifying invader impact: Applying functional response metrics to a rapidly spreading non-native species. Freshwater Biology 63(12):1514–1522. DOI:10.1111/fwb.13180.
- Kornijów R, Pawlikowski K, Drgas A, Rolbiecki L, Rychter A. 2018. Mortality of post-settlement clams Rangia cuneata (Mactridae, Bivalvia) at an early stage of invasion in the Vistula Lagoon (South Baltic) due to biotic and abiotic factors. Hydrobiologia 811(1):207–219. DOI:10.1007/s10750-017-3489-4.
- Kumar S, Stecher G, Li M, Knyaz C, Tamura K. 2018. MEGA X: Molecular Evolutionary Genetics Analysis across Computing Platforms. Molecular Biology and Evolution 35(6):1547–1549. DOI:10.1093/molbev/msy096.
- LaSalle MW, De la Cruz AA (1985) Species profiles: Life histories and environmental requirements of coastal fishes and invertebrates (Gulf Of Mexico) – Common Rangia. United States Fish and Wildlife Service Office of Biological Services Report No. FWS/OBS-82/11.31, and United States Army Corps of Engineers Report No. TR EL-82- 4, Washington, D.C.
- Machordom A, Araujo R, Erpenbeck D, Ramos M-Á. 2003. Phylogeography and conservation genetics of endangered European Margaritiferidae (Bivalvia: Unionoidea). Biological Journal of the Linnean Society 78(2):235–252. DOI:10.1046/j.1095-8312.2003.00158.x.
- Mann R, Campos BM, Luckenbach MW. 1991. Swimming rate and responses of larvae of three mactrid bivalves to salinity discontinuities. Marine Ecology Progress Series 68:257–269. DOI: 10.3354/meps068257.
- Möller T, Kotta J. 2017. Rangia cuneata (G. B. Sowerby I, 1831) continues its invasion in the Baltic Sea: The first record in Pärnu Bay, Estonia. BioInvasions Records 6(2):167–172. DOI:10.3391/bir.2017.6.2.13.
- Poirrier MA, Caputo CE. 2015. Rangia cuneata clam decline in Lake Pontchartrain from 2001 to 2014 due to an El Niño Southern Oscillation shift coupled with a period of high hurricane intensity and frequency. Gulf Caribb Res 26(1):9–20. DOI:10.18785/gcr.2601.04.
- Rand DM. 1996. Neutrality tests of molecular markers and the connection between DNA polymorphism, demography, and conservation biology. Conservation Biology 10(2):665–671. DOI:10.1046/j.1523-1739.1996.10020665.x.
- Riquet F, Daguin‐Thiébaut C, Ballenghien M, Bierne N, Viard F. 2013. Contrasting patterns of genome-wide polymorphism in the native and invasive range of the marine molluscC repidula fornicata. Molecular Ecology 22(4):1003–1018. DOI:10.1111/mec.12161.
- Rogers AR. 1995. GENETIC EVIDENCE FOR A PLEISTOCENE POPULATION EXPLOSION. Evolution 49(4):608–615. DOI:10.1111/j.1558-5646.1995.tb02297.x.
- Rozas J, Ferrer-Mat A, Sánchez-De JC, Guirao-Rico S, Librado P, Ramos-Onsins SE, Sánchez-Gracia A. 2017. DnaSP 6: DNA Sequence Polymorphism Analysis of Large Data Sets. Molecular Biology and Evolution 34(12):3299–3302. DOI:10.1093/molbev/msx248.
- Rudinskaya LV, Gusev AA. 2012. Invasion of the North American wedge clam Rangia cuneata (G.B. Sowerby I, 1831) (Bivalvia: Mactridae) in the Vistula Lagoon of the Baltic Sea. Russian Journal of Biological Invasions 3(3):220–229. DOI:10.1134/S2075111712030071.
- Santos S, Cruzeiro C, Olsen JL, van der Veer HW, Luttikhuizen PC. 2012. Isolation by distance and low connectivity in the peppery furrow shell Scrobicularia plana (Bivalvia). Marine Ecology Progress Series 462:111–124. DOI: 10.3354/meps09834.
- Schneider S, Excoffier L. 1999. Estimation of demographic parameters from the distribution of pairwise differences when the mutation rates vary among sites: Application to human mitochondrial DNA. Genetics. 152(3):1079–1089. DOI:10.1093/genetics/152.3.1079
- Solovjova S (2014) Rangia cuneata introduction to Lithuania/Baltic Sea. In: AquaNIS. Editorial Board (Eds.), Information System on Aquatic Non-INDIGENOUS and Cryptogenic Species. World Wide Web Electronic Publication. www.corpi.ku.lt/databases/aquanis. Version 2.36+. Accessed 06 February 2020.
- Solovjova S, Samuilovienė A, Srėbalienė G, Minchin D, Olenin S. 2019. Limited success of the non-indigenous bivalve clam Rangia cuneata in the Lithuanian coastal waters of the Baltic Sea and the Curonian Lagoon. Oceanologia 61(3):341–349. DOI:10.1016/j.oceano.2019.01.005.
- Tajima F. 1989. Statistical method for testing the neutral mutation hypothesis by DNA polymorphism. Genetics 123(3):585–595. DOI:10.1093/genetics/123.3.585.
- Tang F, Kemp JS, Aldridge DC. 2019. Life on the edge: Compensatory growth and feeding rates at environmental extremes mediates potential ecosystem engineering by an invasive bivalve. The Science of the Total Environment 706:13574. DOI: 10.1016/j.scitotenv.2019.135741.
- Tenore KR, Horton DB, Duke TW. 1968. Effects of bottom substrate on the brackish water bivalve Rangia cuneata. Chesapeake Science 9(4):238–248. DOI:10.2307/1351314.
- Terranova MS, Lo Brutto S, Arculeo M, Mitton J. 2006. Population structure of Brachidontes pharaonis (P. Fisher, 1870) (Bivalvia, Mytilidae) in the Mediterranean Sea, and evolution of a novel mtDNA polymorphism. Marine Biology 150(1):89–101. DOI:10.1007/s00227-006-0330-4.
- Vergara-Chen C, Gonzalez-Wanguemert M, Marcos C, Perez-Ruzafa A. 2013. Small-scale genetic structure of Cerastoderma glaucum in a lagoonal environment: Potential significance of habitat discontinuity and unstable population dynamics. Journal of Molluscan Studies 79(3):230–240. DOI:10.1093/mollus/eyt015.
- Verween A, Kerckhof F, Vincx M, Degraer S. 2006. First European record of the invasive brackish water clam Rangia cuneata (G.B. Sowerby I, 1831) (Mollusca: Bivalvia). Aquatic Invasions 1(4):198–203. DOI:10.3391/ai.2006.1.4.1.
- Voroshilova IS, Ezhova EE, Pavlova VV. 2018. Genetic diversity of the first Baltic population of Rangia cuneata (Bivalvia: Mactridae). Russian Journal of Biological Invasions 9(2):114–118. DOI:10.1134/S2075111718020145.
- Walne PR. 1976. Factors affecting the relation between feeding and growth in bivalves. In: Devik O, editor. Harvesting polluted waters. Boston, MA: Springer. pp. 169–183.
- Warzocha J, Drgas A. 2013. The alien gulf wedge clam (Rangia cuneata G. B. Sowerby I, 1831) (Mollusca: Bivalia: Mactridae) in the Polish part of the Vistula Lagoon (SE. Baltic). Folia Malacologica 21(4):291–292. DOI:10.12657/folmal.021.030.
- Weigand H, Leese F. 2018. Detecting signatures of positive selection in non-model species using genomic data. Zool J Linnean Soc XX:1–56. DOI: 10.1093/zoolinnean/zly007.
- Wickham H (2016) ggplot2: Elegant Graphics for Data Analysis. Springer-Verlag, New York. 9783. 9783319242774. 319242774. https://ggplot2.tidyverse.org
- Wiese L, Niehus O, Faass B, Wiese V. 2016. Ein weiteres Vorkommen von Rangia cuneata in Deutschland (Bivalvia: Mactridae). in German. Schr Malakozool Haus Nat Cismar 29:53–60.
- Willing MJ. 2015. Two invasive bivalves, Rangia cuneata (B. Sowerby I, 1831) and Mytilopsis leucophaeata (Conrad, 1831), living in freshwater in Lincolnshire, Eastern England. J Conchol 42(2):189–192.
- Wolfe DA, Petteway EN. 1968. Growth of Rangia cuneata gray. Chesapeake Science 9(2):99–102. DOI:10.2307/1351251.
- Wong WH, Rabalais NN, Turner RE. 2010. Abundance and ecological significance of the clam Rangia cuneata (Sowerby, 1831) in the upper Barataria Estuary (Louisiana, USA). Hydrobiologia 651(1):305–315. DOI:10.1007/s10750-010-0310-z.