Abstract
The Atlantic Forest is home to several arboviruses potentially pathogenic to humans. Therefore, it is crucial to assess the effects of seasonality on mosquito populations circulating in this domain. We evaluated the influence of seasonal variation on the oviposition activity of epidemiologically important mosquito populations in an Environmental Protection Area in Rio de Janeiro, Brazil. Mosquito eggs were collected using ovitraps for 1 year. During the sampling period, 1,086 eggs were collected. Of these, 39 (3.6%) did not hatch, and 1,047 (96.4%) reached the adult stage. Aedes albopictus (44.8%), Ae. terrens (6.4%), and Haemagogus leucocelaenus (48.8%) eggs and adults were identified. The changes in this community over the seasons were also analyzed. Season influence on the collections was significant. The highest numbers of collected eggs were collected in the summer and autumn, with Hg. leucocelaenus dominant in the summer and Ae. albopictus in the autumn. These two seasons were more similar to each other in terms of the composition of the collected mosquito community, forming a separate cluster from winter and spring groups. Summer, autumn, and winter presented values of Dominance (D), Shannon Diversity (H), and Evenness (J) closer to each other than spring. Climatic factors recorded throughout the collection period were not associated with egg abundance, except for temperature, which was positively correlated with Ae. albopictus presence. Finally, seasonality seemed to influence the oviposition activity of the three species recorded. Summer and autumn were the most critical seasons due to Ae. albopictus and Hg. leucocelaenus circulation. These findings should be considered in prophylaxis and implementation of entomological control strategies in the study area.
Introduction
The Atlantic Forest has experienced severe human activity impacts associated with population densification of the Brazilian coast throughout history. Currently, the most extensive and preserved remnants of the Atlantic Forest are located within Conservation Units (Grelle et al. Citation2021). These preserved areas serve as refuges for fauna, including mosquito species (Evangelista et al. Citation2021).
Many studies have been focused on mosquitoes due to their epidemiological importance, associated with the circulation of various arboviruses in wild environments. Much of the research focuses on geographic distribution and the association of climatic and environmental aspects that directly influence the population dynamics of several Culicidae species (Asigau et al. Citation2017; Freire et al. Citation2021). The effect of seasonality on mosquito populations in the Atlantic Forest, as well as the dynamics of arboviruses transmitted by them, is an important public health issue (Possas et al. Citation2018).
There are approximately 3,600 species of mosquitoes described worldwide (Wilkerson et al. Citation2021), 530 of which have been reported in the Brazilian territory (Hutchings et al. Citation2020). The vast majority of these species are from the Atlantic Forest. Among these species, those belonging to the genera Aedes and Haemagogus are notable for carrying and transmitting yellow fever, Zika, and other arboviruses (Vanlandingham et al. Citation2015; Lourenço-de-Oliveira & Failloux Citation2017; Alencar et al. Citation2021).
Aedes albopictus (Skuse 1895) is an invasive species that has been adapted successfully even in degraded Atlantic Forest fragments, with the remarkable ability to move between the forest edge and urban environments (Santos et al. Citation2018). They are mosquitoes with diurnal behavior mainly concentrated in two activity peaks comprising morning and afternoon (Forattini Citation2002). Aedes terrens (Walker 1856) is very eclectic in the type of habitat and has been found in preserved forests to small forest fragments inserted in the urban environment (Stahlhöfer et al. Citation2021). This species is diurnal, and oviposits and feeds preferentially on the treetops (Guimarães et al. Citation1985). Haemagogus leucocelaenus (Dyar & Shannon 1924) is considered the most common species of Haemagogus in Brazil, as its broad phenotypic plasticity allows it to inhabit degraded wild environments (Marcondes & Alencar Citation2010). This culicid species also has acrodendrophilic habits (Pinto et al. Citation2009).
The use of ovitraps to collect mosquito eggs, especially those of Ae. albopictus, Ae. terrens and Hg. leucocelaenus has been shown to be effective in egg collection, when installed in wild environments (Maia et al. Citation2020). This collection methodology even proves to be important in studies on the detection of arboviruses in these mosquito species, as it allows eggs to be collected in nature and transported to the laboratory, where adults emerging from this material can be quickly identified and killed, which facilitates the preservation of viral genetic material (Alencar et al. Citation2021).
Therefore, understanding the biological responses and population dynamics of vector species such as Ae. albopictus, Ae. terrens and Hg. leucocelaenus to seasonal variations implies a better assessment of arbovirus transmission patterns. This study aimed to evaluate the influence of seasonal variation on the oviposition activity of epidemiologically important mosquito populations in the Environmental Protection Area of the São João River Basin, allowing us to establish which climatic seasons are more prone to the proliferation of these culicids in an area of the Atlantic Forest.
Table I. Abundance, relative frequency, dominance, diversity, and evenness in different seasons of mosquito species collected between July 2018 and June 2019 in the EPA of the São João River Basin, Rio de Janeiro, Brazil.
Materials and methods
Ethics statement
The collection, capture, and transport of zoological materials were authorized by Chico Mendes Institute for Biodiversity Conservation (ICMBio), Ministry of the Environment of Brazil, under license No. 44333-1. All members responsible for the collections received vaccination against yellow fever virus and were aware of the potential risks in the study area.
Study area
The study was conducted within the São João River Basin Environmental Protection Area (EPA), in Silva Jardim, state of Rio de Janeiro, Brazil. The EPA of the São João River Basin is a conservation unit with 150,374.61 hectares of Atlantic Forest. This conservation unit is located at Km 214 of BR 101 Highway in the city of Silva Jardim, Rio de Janeiro, Brazil (ICMBIO Citation2020). The São João River is the main watercourse of the region, significantly contributing to the water supply of the surrounding regions through the Juturnaíba Reservoir of Araruama, state of Rio de Janeiro. The vegetation is characterized by dense ombrophilous forests, also known as tropical rainforests. This ecosystem comprises evergreen foliage, canopies up to 50 m high, emerging trees up to 40 m tall, and dense shrub vegetation composed of ferns, bushes, and bromeliads (MMA Citation2008).
Collections and laboratory procedures
Between July 2018 and June 2019, monthly collections of mosquito eggs were carried out using ovitraps. Eighteen ovitraps were distributed using convenience sampling, a non-probabilistic sampling technique (Kenkel Citation1989), where traps were positioned at a minimum distance of 40 m from each other. Each ovitrap was placed at a height of 2.50 m affixed to trees located along a walking trail near the edge of the forest fragment used by visitors.
Eighteen ovitraps were installed at random intervals, with one trap placed at a height of 2.50 m per tree, between July 2018 and June 2019 ( and ).
Figure 1. Location of the eighteen sampling points in the Environmental Protection Area of São João Basin, Biological Reserve of the city of Silva Jardim, state of Rio de Janeiro, Brazil. Georeferenced positions for the ovitraps: Point 1. 22°37ʹ10.7″S, 42°18ʹ59.5″W; Point 2. 22°37ʹ10.7″S, 42°19ʹ01.9″W; Point 3. 22°37ʹ11.2″S, 42°19ʹ04.7″W; Point 4. 22°37ʹ12.7″S, 42°19ʹ06.2″W; Point 5. 22°37ʹ13.7″S, 42°19ʹ04.9″W; Point 6. 22°37ʹ15.2”S, 42°19ʹ03.0″W; Point 7. 22°37ʹ15.8″S, 42°19ʹ01.9″W; Point 8. 22 °37ʹ16.1”S, 42°18ʹ59.9″W; Point 9. 22°37ʹ17.1″S, 42°18ʹ58.3″W; Point 10. 22°37ʹ18.0”S, 42°18ʹ56.8″W; Point 11. 22°37ʹ18.0″S, 42°18ʹ54.0″W; Point 12. 22°37ʹ18.1”S, 42°18ʹ53.8″W; Point 13. 22°37ʹ18.1″S, 42°18’ 52.6″W; Point 14. 22°37ʹ13.8”S, 42°18ʹ43.2″W; Point 15.22°37ʹ13.5”S, 42°18ʹ43.3″W; Point 16.22°37ʹ15.0”S, 42°18ʹ42.8″W; Point 17. 22°37ʹ14.4″S, 42°18ʹ41.8″W; Point 18. 22°37ʹ15.2”S, 42°18ʹ40.8″W.
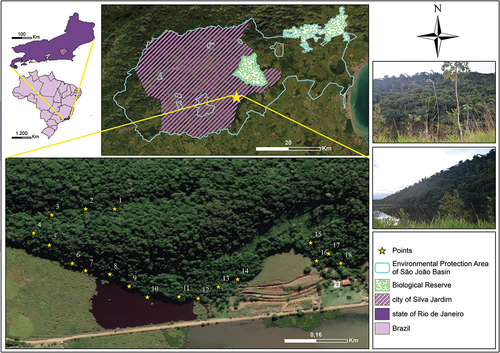
Ovitraps were used as a standard tool for the collection of Culicidae eggs. These traps consist of a 500 mL black-colored unlidded container resembling a plant vase. Each ovitrap contained four wooden oviposition paddles (2.5 × 14 cm), vertically held by a clip inside the trap. A total of 300 mL of natural water and approximately 100 g of leaf litter were added to each ovitrap in an effort to recreate a microecosystem resembling the natural environment. The ovitraps were inspected for the presence of eggs, and their aquatic content changed every 10 days throughout the collection period.
Once collected from the ovitraps, the paddles were packed in a polyethylene box and sent to the Diptera Laboratory of the Oswaldo Cruz Institute (FIOCRUZ). Paddles containing eggs were considered positive; these were sorted in the laboratory and subjected to egg counting. Then, these paddles were immersed in transparent trays containing dechlorinated water. Subsequently, the eggs were placed in a laboratory greenhouse under a controlled experimental environment and regulated thermoperiod (28°C ± 1°C, 75–90% relative air humidity, and a 12 h light/12 h dark cycle). These conditions allowed us to keep the specimens alive and perform species identification on adult forms after hatching, according to the methodology described by Alencar et al. (Citation2013).
Species identification was carried out by directly observing morphological characters under a stereomicroscope and using dichotomous keys developed by Arnell (Citation1973) and Forattini (Citation2002). Abbreviations of genus and subgenus names were based on Reinert’s proposal (Citation2009). After species identification, all specimens were listed in the FIOCRUZ Entomological Collection.
Data analysis
We used the Chi-square test to analyze the effect of seasonal variation on the abundance of mosquito species using the statistical software GraphPad Prism 8.01 for Windows. To analyze the effect of seasonal variation on the mosquito community, the Bray–Curtis similarity index was calculated, and values for Dominance (D), Shannon Diversity (H), and Pielou’s evenness (J) were obtained using the PAST 4.03 statistical software (Hammer et al. Citation2001). This software was also used to generate a canonical correspondence analysis (CCA), which was applied to verify the effect of the evaluated climatic variables (mean temperature and accumulated rainfall) on the sampled mosquito species. The level of significance used in the statistical tests was p < 0.05.
Mean temperatures and accumulated rainfall during the study period were obtained from the Weather Prevision Center and Climate Studies (CPTEC) of the National Institute for Space Research (INPE Citation2020).
Results
During the sampling period, 1,086 eggs were collected in the São João River Basin area. Of these, 39 (3.6%) did not hatch, and 1,047 (96.4%) evolved to the adult stage, corresponding to the following species: Ae. albopictus (Skuse, 1895) (469–44.8%), Ae. terrens (Walker, 1856) (67–6.4%) and Hg. leucocelaenus (Dyar & Shannon, 1924) (511–48.8%).
The three species of mosquitoes were present in the collections in all seasons. Haemagogus leucocelaenus was the most abundant species in the summer (57.5%) and winter (56.8%). In contrast, Ae. terrens was the most abundant species in the spring (42.3%) and Ae. albopictus was the most abundant in the autumn (55.6%) (). The influence of seasonality on the collections was significant by Chi-square analysis (χ2 = 222,9; d.f. = 6; p < 0.0001).
The highest number of eggs was obtained in the collections carried out in the autumn (466–44.5%) and summer (433–41.4%), followed by spring (104–9.9%) and winter (44–4.2%), respectively. Summer, autumn, and winter presented D, H, and J values closer to each other than when compared to spring (). The Bray-Curtis index indicated a high similarity between the composition and abundance of mosquito species collected in the autumn and summer (85%), whereas the cluster formed by species collected in the spring and winter had a similarity of approximately 60% ().
Figure 2. Cluster analysis (Bray-Curtis index) of mosquito samples collected in different seasons between July 2018 and June 2019 in the EPA of the São João River Basin, Rio de Janeiro, Brazil.
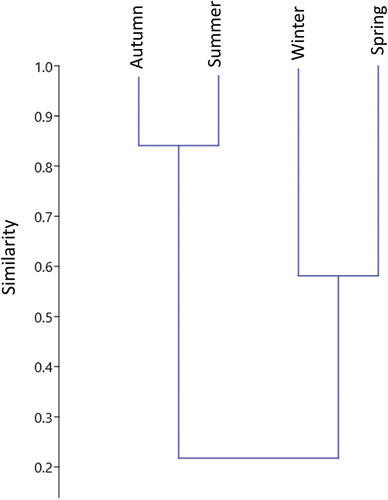
The CCA indicated significant positive correlations between Ae. albopictus with temperature (oC) (r = 0.59; p < 0.05), but not with precipitation (mm) (p > 0.05). The other species did not show a positive correlation with temperature or rainfall (p > 0.05) ().
Discussion
The three species of mosquitoes recorded in this study had already been captured by ovitraps in a wild environment, including in Atlantic Forest areas of the state of Rio de Janeiro (Alencar et al. Citation2016). When litter is added to its aquatic content, these traps simulate the conditions of breeding sites formed by tree holes (Alencar et al. Citation2004). Immature forms of Ae. terrens and Hg. leucocelaenus are commonly found in tree holes (Mangudo et al. Citation2018). Aedes albopictus has less frequent records of occurrence in these breeding sites than the other two, as it is a species whose occurrence in a wild environment is limited to areas most impacted by human activity (Pancetti et al. Citation2015).
Our results showed a greater abundance of Hg. leucocelaenus eggs, a species dominant in the summer and autumn. Studies involving the installation of ovitraps in fragments of the Atlantic Forest in Rio de Janeiro usually indicate dominance of this species (Alencar et al. Citation2016) often in all seasons of the year (Silva et al. Citation2018a). Aedes albopictus was the second most abundant species identified, dominant in the autumn. Silva et al. (Citation2018b) observed that Ae. albopictus was also the second most abundant species collected from ovitraps installed in forest areas. In a study using ovitraps to monitor Ae. albopictus in an urban area of the state of São Paulo, this species was present during the summer and autumn, with higher population numbers registered in April (Serpa et al. Citation2006). Autumn and summer seem to offer climatic conditions that favor the proliferation of Ae. albopictus and Hg. leucocelaenus. Aedes terrens was the least abundant species, whose presence was more prevalent in the spring and nearly absent in the winter. In a study developed by Silva et al. (Citation2021), Ae. terrens was also less frequent in ovitraps installed in a forest area of Rio de Janeiro than Hg. leucocelaenus and Ae. albopictus. Campos (Citation2016) observed in natural breeding sites formed by bamboo stumps that Ae. terrens only occurred in spring, being absent in other seasons.
The greater absolute abundance of eggs, as well as greater similarity in the composition of species recorded in the autumn and summer ( and ), indicates that these seasons possibly offer climatic conditions that favor the proliferation of mosquitoes, especially of Ae. albopictus and Hg. leucocelaenus. Although several studies suggest that the structure and complexity of the environment have an important effect on the diversity and composition of mosquito species (Catenacci et al. Citation2018), variations in climatic conditions are also an important element (Wilke et al. Citation2017). As reported in other studies, the increase in rainfall and temperature in the summer allows an increase in mosquito populations due to the formation of new breeding sites and the intensification of the activity of these insects (Chadee & Tikasingh Citation1991; Muller & Marcondes Citation2007; Medeiros-Sousa et al. Citation2015). In the present observations and as previously reported, autumn proved to be very similar to summer, which can be explained by the delay in climate change influenced by seasonality that affects mosquito communities. Probably, the weather conditions generated in the summer were still influencing the mosquito records carried out throughout the autumn (Couto-Lima et al. Citation2020). Couto-Lima observed a significant effect of temperature and rainfall on the oviposition of sylvatic mosquitoes after four weeks. The same pattern was observed for Ae. aegypti in an urban environment, where the egg density was affected by the variables temperature and rainfall after three and 4 weeks of their records (Honório et al. Citation2009), indicating that, possibly, climatic variables recorded in a season can influence the abundance of mosquito eggs in the following season.
The abundances of Hg. leucocelaenus, Ae. albopictus and Ae. terrens showed similar values in the spring when compared to other seasons. This implied higher values of H and J and lower values of D. The high abundance of Hg. leucocelaenus in summer, of Ae. albopictus in the autumn and the reduced abundance in the winter influenced the results with lower values of H and J, and higher values of D at these stations (). In contrast to the present study, Guedes and Navarro-Silva (Citation2014) collected adult mosquitoes in an area of Atlantic Forest in the state of Paraná and observed lower values of evenness and diversity and greater dominance during the spring. This pattern was also observed by Cardo et al. (Citation2012), who studied immature forms of mosquitoes in different breeding sites in a wild area of Argentina.
In this study, no significant correlation was found between the abundance of eggs of the three species and climatic factors, except for Ae. albopictus, which showed a positive correlation with temperature (). This result corroborates the study by Silva et al. (Citation2021) conducted in a region near the São João River EPA using ovitraps and showing a positive correlation between Ae. albopictus egg abundance and temperature. In the present study, water was initially added to the ovitraps, and these were revised every 10 days when the paddles were changed; if necessary, more water was added during inspections. By these means, eventually, in drier and hotter periods, the traps continued to have water available for female mosquitoes’ oviposition. This methodology may have influenced the lack of a significant correlation between rainfall and egg abundance.
In conclusion, seasonality seemed to influence the oviposition activity of the three species recorded. Summer and autumn can be considered the most critical seasons for Ae. albopictus and Hg. leucocelaenus, which should be considered in any prophylaxis and entomological control strategies implemented in the São João River Basin Environmental Protection Area.
Disclosure statement
No potential conflict of interest was reported by the author(s).
Additional information
Funding
References
- Alencar J, Gil-Santana HR, Lopes CM, Santos JS, Guimarães AE. 2004. Utilização de armadilha “ovitrampa” para monitoramento de Haemagogus janthinomys (Diptera: Culicidae) em área de Mata Atlântica. Entomologia y Vectores 2:369–374.
- Alencar J, Morone F, De Mello CF, Dégallier N, Lucio PS, de Serra-Freire NM, Guimarães AE. 2013. Flight height preference for oviposition of mosquito (Diptera: Culicidae) vectors of sylvatic yellow fever virus near the hydroelectric reservoir of Simplício. Journal of Medical Entomology 50:791–795. DOI: 10.1603/ME12120.
- Alencar J, de Mello CF, Barbosa LS, Gil-Santana HR, Maia DA, Marcondes CB, Silva JS. 2016. Diversity of yellow fever mosquito vectors in the Atlantic Forest of Rio de Janeiro, Brazil. Revista da Sociedade Brasileira de Medicina Tropical 49(3):351–356. DOI: 10.1590/0037-8682-0438-2015.
- Alencar J, Mello CF, Marcondes CB, Guimarães AE, Toma HK, Bastos AQ, Silva SOF, Machado SL. 2021. Natural infection and vertical transmission of Zika virus in sylvatic mosquitoes Aedes albopictus and Haemagogus leucocelaenus from Rio de Janeiro, Brazil. Tropical Medicine and Infectious Disease 6(2):99. DOI: 10.3390/tropicalmed6020099.
- Arnell JH. 1973. Mosquito studies (Diptera, Culicidae) XXXII. A revision of the genus Haemagogus. Contributions of the American Entomological Institute 10:1–174.
- Asigau S, Hartman DA, Higaschiguchi JM, Parker PG. 2017. The distribution of mosquitoes across an altitudinal gradient in the Galapagos Islands. Journal of Vector Ecology 42:243–253. DOI: 10.1111/jvec.12264.
- Campos RE. 2016. Phytotelmata colonization in bamboo (Guadua sp.) culms in northeast Argentina. Journal of Natural History 50(15–16):923–941. DOI: 10.1080/00222933.2015.1091096.
- Cardo MV, Vezzani D, Carbajo AE. 2012. Immature mosquitoes from groundwater habitats in a temperate wetland of Argentina: Environmental associations and seasonal variation of community attributes. Journal of the American Mosquito Control Association 28(3):151–159. DOI: 10.2987/12-6225R.1.
- Catenacci LS, Nunes-Neto J, Deem SL, Palmer JL, Travassos-da-Rosa ES, Tello JS. 2018. Diversity patterns of hematophagous insects in Atlantic forest fragments and human-modified areas of southern Bahia, Brazil. Journal of Vector Ecology 43(2):293–304. DOI: 10.1111/jvec.12313.
- Chadee DD, Tikasingh ES. 1991. Seasonal incidence and diel oviposition periodicity of Haemagogus mosquitoes (Diptera: Culicidae) in Trinidad, W.I. Part III. Haemagogus celeste (Dyar and Nunez Tovar) and Haemagogus leucocelaenus (Dyar and Shannon). Annals of Tropical Medicine and Parasitology 85(5):543–550. DOI: 10.1080/00034983.1991.11812607.
- Couto-Lima DC, Anderazzi CS, Leite PJ, Bersot MIL, Alencar J, Lourenço-de-Oliveira R. 2020. Seasonal population dynamics of the primary yellow fever vector Haemagogus leucocelaenus (Dyar & Shannon) (Diptera: Culicidae) is mainly influenced by temperature in the Atlantic Forest, southeast Brazil. Memórias do Instituto Oswaldo Cruz 115:e200218. DOI: 10.1590/0074-02760200218.
- Evangelista E, Medeiros-Sousa AR, Ceretti-Junior W, Oliveira-Christe R, Wilk-da-Silva R, Amrc D, Vendrami DP, Carvalho GC, Mucci LF, Marrelli MT. 2021. Relationship between vertical stratification and feeding habits of mosquito (Diptera: Culicidae) assemblages collected in conservation units in the green belt of the city of São Paulo, Brazil. Acta Tropica 221(2021):106009. DOI: 10.1016/j.actatropica.2021.106009.
- Forattini OP. 2002. Culicidologia Médica. Vol 2: Identificação, biologia, epidemiologia. São Paulo: Editora da Universidade de São Paulo.
- Freire RCM, Barbosa TM, Jales JT, Ximenes MFFM, Corte RL, Gama RA. 2021. Ecological aspects of mosquitoes (Diptera: Culicidae) in a fragment of seasonal dry tropical forest (Caatinga) in Brazil. Journal of Arid Environments 190:104528.
- Grelle CEV, Bayma AP, Paixão LR, Egler M, Senta MM, Jenkins CN, Uezu A, Pellin A, Martensen AC, Shirai H, and Soares N. 2021. Conservation initiatives in the Brazilian Atlantic Forest. In: Marques MCM, Grelle CEV, editors. The Atlantic Forest: History, biodiversity, threats and opportunities of the megadiverse forest Switzerland: Springer. pp. 421–449.
- Guedes MLP, Navarro-Silva MA. 2014. Mosquito community composition in dynamic landscapes from the Atlantic Forest biome (Diptera, Culicidae). Revista Brasileira de Entomologia 58(1):88–94. DOI: 10.1590/S0085-56262014000100014.
- Guimarães AE, Arlé M, Machado RNM. 1985. Mosquitos no Parque Nacional da Serra dos Órgãos, Estado do Rio de Janeiro, Brasil. II- Distribuição vertical. Memórias do Instituto Oswaldo Cruz 80:171–185. DOI: 10.1590/S0074-02761985000200008.
- Hammer Ø, Harper DAT, Ryan PD. 2001. PAST: Paleontological statistics software package for education and data analysis. Palaeontologia Electronica 4:1–9.
- Honório NA, Codeço CT, Alves FC, Mafm M, Lourenço-de-Oliveira R. 2009. Temporal distribution of Aedes aegypti in different districts of Rio De Janeiro, Brazil, measured by two types of traps. Journal of Medical Entomology 46(5):1001–1014. DOI: 10.1603/033.046.0505.
- Hutchings RW, Sallum MAM, Hutchings RSG. 2020. Culicidae in Catálogo Taxonômico da Fauna do Brasil. Available: http://fauna.jbrj.gov.br/fauna/faunadobrasil/798. Accessed Sep 2021 10.
- ICMBIO. 2020. APA da Bacia do Rio São João/Mico-Leão-Dourado. Available: https://www.icmbio.gov.br/portal/unidadesdeconservacao/biomas-brasileiros/mata-atlantica/unidades-de-conservacao-mata-atlantica/2169-apa-da-bacia-do-rio-sao-joaomico-leao-dourado. Accessed Jun 2020.
- INPE. Instituto Nacional de Pesquisas Espaciais. Centro de Previsão de Tempo e Estudo Climáticos. Avaliable: http://sinda.crn.inpe.br/PCD/SITE/novo/site/historico/index.phphttp://sinda.crn.inpe.br/PCD/SITE/novo/site/historico/index.php. Accessed June 2020.
- Kenkel NC, Juhász-Nagy PJ. 1989. On sampling procedures in population and community ecology. Vegetatio 83:195–207. DOI: 10.1007/BF00031692.
- Lourenço-de-Oliveira R, Failloux AB. 2017. Lessons learned on Zika virus vectors. PLOS Neglected Tropical Diseases 11(6):e0005511. DOI: 10.1371/journal.pntd.0005511.
- Maia DA, Bastos AQ, Leite PJ, Gil-Santana HR, Silva JS, Alencar J. 2020. Comparative analysis between sampling methods for immature mosquitoes in an Atlantic Forest fragment in Brazil. Journal of the American Mosquito Control Association 36(4):245–248. DOI: 10.2987/20-6947.1.
- Mangudo C, Aparicio JP, Rossi GC, Gleiser RM. 2018. Tree hole mosquito species composition and relative abundances differ between urban and adjacent forest habitats in northwestern Argentina. Bulletin of Entomological Research 108:203–212. DOI: 10.1017/S0007485317000700.
- Marcondes CB, Alencar J. 2010. Revisão de mosquitos Haemagogus Williston (Diptera: Culicidae) do Brasil. Review Biomedical 21:221–238.
- Medeiros-Sousa AR, Ceretti-Júnior W, Carvalho GC, Nardi MS, Araujo AB, Vendrami DP, Marrelli MT. 2015. Diversity and abundance of mosquitoes (Diptera: Culicidae) in an urban park: Larval habitats and temporal variation. Acta Tropica 150:200–209. DOI: 10.1016/j.actatropica.2015.08.002.
- MMA. Ministério do Meio Ambiente. 2008. Plano de Manejo da Área de Proteção Ambiental da Bacia do Rio São João/Mico Leão Dourado.
- Muller GA, Marcondes CB. 2007. Immature mosquitoes (Diptera: Culicidae) on the bromeliad Nidularium innocentii in ombrophilous dense forest of Santa Catarina Island, Florianópolis, Santa Catarina State, southern Brazil. Biotemas 20(2):27–31.
- Pancetti FGM, Honório NA, Urbinatti PR, Lima-Camara TN. 2015. Twenty-eight years of Aedes albopictus in Brazil: A rationale to maintain active entomological and epidemiological surveillance. Revista da Sociedade Brasileira de Medicina Tropical 48(1):87–89. DOI: 10.1590/0037-8682-0155-2014.
- Pinto CS, Confalonieri UEC, Mascarenhas BM. 2009. Ecology of Haemagogus sp. and Sabethes sp. (Diptera: Culicidae) in relation to the microclimates of the Caxiuanã National Forest, Pará, Brazil. Memórias do Instituto Oswaldo Cruz 104(4):592–598. DOI: 10.1590/S0074-02762009000400010.
- Possas C, Lourenço-de-Oliveira R, Tauil PL, Pinheiro FP, Pissinatti A, Cunha RV, Freire M, Martins RM, Homma A. 2018. Yellow fever outbreak in Brazil: The puzzle of rapid viral spread and challenges for immunization. Memorias do Instituo Oswaldo Cruz 113(10):e180278.
- Reinert JF. 2009. List of abbreviations for currently valid generic-level taxa in family Culicidae (Diptera). European Mosquito Bulletin 27:68–76. PMID: 83370280.
- Santos TP, Roiz D, Abreu FVS, Luz SLB, Santalucia M, Jioll D, Msam N, Simard F, Lourenço-de-Oliveira R, Paupy C. 2018. Potential of Aedes albopictus as a bridge vector for enzootic pathogens at the urban-forest interface in Brazil. Emerging Microbes & Infections 7:191.
- Serpa LLN, Costa KVRM, Voltolini JC, Kakitani I. 2006. Variação sazonal de Aedes aegypti e Aedes albopictus no município de Potim, São Paulo. Revista de Saúde Pública 40(6):1101–1105. DOI: 10.1590/S0034-89102006005000008.
- Silva SOF, Mello CF, Figueiró R, Maia DA, Alencar J. 2018a. Distribution of the mosquito communities (Diptera: Culicidae) in oviposition traps introduced into the Atlantic Forest in the State of Rio de Janeiro, Brazil. Vector-Borne and Zoonotic Diseases 18(4):214–221. DOI: 10.1089/vbz.2017.2222.
- Silva SOF, Mello CF, Gleiser RM, Oliveira AA, Maia DA, Alencar J. 2018b. Evaluation of multiple immersion effects on eggs from Haemagogus leucocelaenus, Haemagogus janthinomys, and Aedes albopictus (Diptera: Culicidae). Under Experimental Conditions. Journal of Medical Entomology 55(5):1093–1097.
- Silva SOF, Mello CF, Figueiró R, Docile T, Serdeiro M, Fagundes D, Alencar J. 2021. Oviposition behavior of wild yellow fever vector mosquitoes (Diptera: Culicidae) in an Atlantic Forest fragment, Rio de Janeiro state, Brazil. Scientific Reports 11:6081. DOI: 10.1038/s41598-021-85752-y.
- Stahlhöfer BD, Borsekowsky AF, Müller GA. 2021. Mosquitoes (Diptera: Culicidae) in oviposition traps set in forest fragments of a semideciduous seasonal forest, Atlantic Forest domain, in the state of Rio Grande do Sul, Brazil. Revista Chilena de Entomología 47(2):375–383. DOI: 10.35249/rche.47.2.21.20.
- Vanlandingham D, Higgs S. 2015. Chikungunya virus and its mosquito vectors. Vector Borne and Zoonotic Diseases (Larchmont, NY) 15(2):1–10. DOI: 10.1089/vbz.2015.15.1.intro.
- Wilke ABB, Medeiros-Sousa AR, Ceretti-Junior W, Marrelli MT. 2017. Mosquito populations dynamics associated with climate variations. Acta Tropica 166(2017):343–350. DOI: 10.1016/j.actatropica.2016.10.025.
- Wilkerson RC, Linton Y-M, and Strickman D. 2021. Mosquitoes of the world. Baltimore, MD: Johns Hopkins University Press.