Abstract
Nosemosis is one of the most widespread honeybee diseases. Its epidemical state can be determined as panzootic. The infectious agents are the microsporidia Nosema apis and N. ceranae. Numerous substances and preparations were tested in order to find a way to combat this disease. However, methodology used in artificial infection experiments is not unique; concentrations of N. ceranae spores in inoculum vary as well as the age of honey bees when they are infected. In addition, the disease itself is still relatively poorly understood. This makes the interpretation of such research difficult. The aim of this study is to investigate the effect of bee age and inoculum concentration on the development of N. ceranae infection. Honeybee workers were collectively infected at the age of 2 and 10 days post-emergence with concentrations of 104, 5 × 104, and 105 spores/bee. While the results indicate a significant effect of both tested factors on the development of N. ceranae, the relationship is not simple, and age alters the pattern of nosemosis development in response to the given concentrations.
Highlights
The course and the effect of Nosema ceranae infection differed depending on bee age at the moment of infection.
The development of the infection differed depending on concentration of N. ceranae spores in inoculum.
Concentration of N. ceranae spores had an effect on mortality and syrup intake in 10-day-old bees.
Introduction
Nosemosis is a widespread honeybee disease caused by the microsporidians Nosema apis (Zander 1909) and N. ceranae (Fries et al. Citation1996). The microsporidia are obligate intracellular eukaryotic parasites, currently classified as fungi. They have secondarily lost their mitochondria and peroxisomes and do not have the classic Golgi apparatus, making their development dependent on the resources of the host cell (Franzen et al. Citation2004). Nosema spores infect bee midgut epithelial cells. They enter the intestinal lumen along with food. The spore injects sporoplasm containing the genetic material directly to the cytoplasm of the host cell. The spores multiply through merogony and sporogony, and the descendant spores leave the cell to enter the lumen of the intestine. There, they infect other epithelial cells or leave the body with the faeces, ready to infect a new host. The same cell may also be reinfected. Repeated infection of epithelial cells may lead to epithelial necrosis and the inability to rebuild the peritrophic membrane of the midgut (Higes et al. Citation2010; Ptaszyńska Citation2013; Tomaszewska & Chorbiński Citation2013; Martín-Hernández et al. Citation2018). The development cycle of N. ceranae lasts less than three days and is not dependent on temperature (Higes et al. Citation2010; Tomaszewska & Chorbiński Citation2013).
Nosema apis infection in western honeybee was described more than 100 years ago; it causes disease called “type A nosemosis”. Infection dynamics is seasonal, with peaks in spring and autumn (Copley et al. Citation2012). The infection in acute form causes diarrhea, brown faecal marks can be visible on combs and on bee entrance; sick bee workers are trembling and have dilated abdomens; lots of weak and dead bees are in the hive vicinity. The disease also causes weakness of the colony and decrease in brood production (Higes et al. Citation2010). In contrast, N. ceranae infection (called “type C nosemosis”) has been present in the western honeybee only for several decades, but it spread very widely and nowadays is very common. It usually does not show any visible symptoms. The infection may intensify at any time of the year, resulting in the weakening or death of the entire colony (albeit some authors observed seasonality in N. ceranae infection; viz. Traver et al. Citation2012). Heavily-infected collecting bees likely do not return to the hive and die far away (Higes et al. Citation2010; Tomaszewska & Chorbiński Citation2013). The colonies are weaker, smaller and produce less honey and brood than healthy colonies. These are non-specific symptoms that make it difficult to detect the infection (Roman Citation2006; Higes et al. Citation2010; Tomaszewska & Chorbiński Citation2013). The death of bees occurs as a result of energetic stress caused by the degeneration of the intestinal epithelium, which results in a lower absorption of nutrients and lower secretion of digestive enzymes. It has been shown that the level of protein is lower in the bodies of diseased bees, which leads to the atrophy of the pharyngeal glands. As a result of infection, the fatty acid profile of the haemolymph changes. N. ceranae can cause anatomical changes in the structure of the bee’s ovaries, rendering it sterile (Ptaszyńska Citation2013). Recent studies have shown that N. ceranae disrupts the iron balance in the bees, leading to its deficiency. In addition to low iron levels, infected bees showed increased expression of transferrins, i.e. iron-binding transport proteins, which can be explained by their capture by the parasite from the host organism (Rodríguez-García et al. Citation2021). Nosemosis is recognized as one of the causes of the colony collapse disorder (CCD) (Ellis et al. Citation2010). In the acute course of the disease, diarrhoea occurs, and the sweet faeces are eagerly licked by bees, what leads to infection. Sick feeder bees can infect the fed larvae (Ptaszyńska Citation2013). Bees become infected through trophallaxis (Smith Citation2012). The disease can spread between colonies by robbery. Beekeeping equipment that has not been properly cleaned and disinfected can also be a vector of the parasite (Tomaszewska & Chorbiński Citation2013). It has been shown that Nosema spp. spores occur in bee products and remain viable for a long time (MacInnis et al. Citation2020). The antibiotic fumagillin, produced by the fungus Aspergillus fumigatus, was used to treat nosemosis. However, due to its entry into bee products and a toxic effect on humans, it was prohibited in the European Union. The antibiotic is still used, inter alia, in the United States, but spore resistance to the drug has appeared (Huang et al. Citation2013). There is currently no other effective drug to combat the disease. Infection is prevented mainly by disinfecting the hives and apiary equipment or by avoiding merging sick families with healthy ones. Supporting bees also involves maintaining the colony in a good condition and preventing hunger by enabling access to appropriate food sources and feeding the colony when needed (Formato et al. Citation2022). Beekeepers can also use commercial supplements, often consisting of a composition of various plant extracts, which also help to reduce the level of infection and improve the condition of bee colonies. However, these products do not completely eliminate the pathogen (Chioveanu et al. Citation2004; Gajger et al. Citation2009; Shumkova et al. Citation2021). Therefore, many studies have recently focused on finding substances with the therapeutic potential to fight the disease. Numerous plant extracts were tested for their potential in nosemosis inhibition (Pohorecka Citation2004; Porrini et al. Citation2011b; Chen et al. Citation2015; Kim et al. Citation2016, Citation2017; Bravo et al. Citation2017; Moradi Citation2017; Xu et al. Citation2017; Arismendi et al. Citation2018; Lee et al. Citation2018; Nanetti et al. Citation2021), as well as single components (Maistrello et al. Citation2008; Costa et al. Citation2010; Strachecka et al. Citation2014a, Citation2014b, Citation2015; Ptaszyńska et al. Citation2018; Borges et al. Citation2020; Buczek et al. Citation2020). The natural microbiome facilitates the digestion of food, takes part in the detoxification of harmful substances, provides valuable nutrients and participates in the immune response and protects against pathogens and parasites. For that reason various probiotics and prebiotics have also been investigated (Engel & Moran Citation2013; Andrearczyk et al. Citation2014; Audisio et al. Citation2015; Baffoni et al. Citation2016; Corby-Harris et al. Citation2016; Ptaszyńska et al. Citation2016a, Citation2016b; El Khoury et al. Citation2018). In many cases, a positive effect on the infected honeybees was observed, but an effective cure is still being sought.
It was investigated that various factors can affect nosemosis development, such as the morphotype of Nosema, honeybee breed (Porrini et al. Citation2020), climate, time of research (Copley et al. Citation2012; Gisder et al. Citation2017; Emsen et al. Citation2020), method of inoculation (Milbrath et al. Citation2013; Urbieta-Magro et al. Citation2019). Also the age of bee and concentration of infective inoculum are significant factors determining the final rate of infection (Porrini et al. Citation2011a; Urbieta-Magro et al. Citation2019). These factors were evaluated alone. The aim of this study was to investigate effect of these factors in combinations, mainly the rate of disease development. Two age variants were chosen for research: 2 days and 10 days post-emergence, as they represent different physiological stages of honeybee life. According to the standard methodology, it is recommended that bees are infected no earlier than at the age of a few days old (2–5) because of possible damage through handling (Amdam et al. Citation2005; Fries et al. Citation2013). An inoculum concentration of 104 spores/bee was established as causing a 100% bee infection rate after inoculation (Forsgren & Fries Citation2010), which is why it was used in our study as the lowest spore dosage.
Materials and methods
Experimental design
In order to test the impact of host age and inoculum concentration on infection development, two host age variants, 2 days and 10 days, and three infecting spore concentrations: 104, 5 × 104 and 105 spores/bee were used. There were two control groups, one for each age variant. Therefore, 8 groups took part in the experiment: 6 treatment groups (2 × 3 variants) and 2 controls for the host age variants. Each group consisted of 4–6 replications (cages).
Research material
Colonies were checked for nosemosis infection with haemocytometry (the bees were collected from peripheral combs; n = 30 bees were tested from each colony). From Nosema-free colonies, frames with sealed worker brood aged 21 days were taken to the laboratory and kept in an incubator at 34.5 ± 0.5°C and 80% humidity. Newly-emerged worker honeybees were transferred to cages, 150 individuals per cage. Cages were kept in an incubator at 30.5 ± 0.5°C. The bees were fed ad libitum with 1:1 (v:w) sucrose syrup. Syrup intake and mortality were recorded daily, sugar syrup was replaced to fresh and dead bees were removed.
Spore preparation
Spore suspensions were freshly prepared. Abdomens of bees from naturally-infected colonies were macerated with a mortar and pestle, then suspended in 0.6% saline. To isolate spores, the suspension was centrifuged for 5 min in 5,000 G (centrifugation method of purification, from standard methodology; Fries et al. Citation2013). The supernatant was replaced with fresh saline three times. After the last centrifugation, the supernatant was replaced with 1:1 (v:w) sucrose syrup, and spore concentration was examined using haemocytometry. Next, the infecting solutions were diluted to obtain the final concentrations of 104, 5 × 104 and 105 spores per 10 µL. The identity of the spores was confirmed with PCR.
Genetic identification of Nosema ceranae
For DNA analysis, the spores obtained from the intestines of the 10 infected bees were resuspended in 10 ml of distilled water (approx. 1000 µl/bee). 50 µL of the spore suspension was incubated in the presence of 120 µL of the TNES buffer (100 mM Tris-HCl pH 8.0, 5 mM EDTA, 0.3% SDS, 200 mM NaCl) with 8 µL of proteinase K (10 mg/ml) for 2 hours at 56°C with shaking. The undigested debris was removed using centrifugation, and the DNA from the supernatant was precipitated by adding an equal volume of 100% isopropanol. The DNA was washed twice in 70% ethanol and resuspended in 50 µL of nuclease-free water. PCR amplification using species-specific primers complementary to the rRNA genes of Nosema species and a PCR Mix Plus kit containing PCR anti-inhibitors (A&A Biotechnology) was performed in the following conditions: 94°C, 3 min initial denaturation and 35 cycles (94°C for 30 sec, 52°C for 30 sec and 72°C for 30 sec). The following primer pairs were used: N. ceranae, forward: 5’- CGGATAAAAGAGTCCGTTACC, reverse: 5’- TGAGCAGGGTTCTAGGGAT (Chen et al. Citation2008); N. apis, forward: 5’- CCATTGCCGGATAAGAGAGT, reverse: 5’- CCACCAAAAACTCCCAAGAG (Chen et al. Citation2009). The amplification products were analysed with gel electrophoresis using 2% agarose gel.
Inoculation and cage trials
Bees were starved for 2 h before infection and inoculated collectively by administering the infecting solution to each cage in a volume of 10 µL/bee (to calculate exact volume of infecting solution per cage, all days before inoculation the mortality was recorded; the number of individuals at the beginning minus the sum of dead ones determined the final volume). After the infecting suspension was completely consumed, the bees were fed sucrose syrup ad libitum. Since this time syrup intake and mortality were recorded daily to collect data to comparison. The syrup was also replaced with a fresh one, and dead bees were removed from cages. The experiment lasted seven days.
Infection level examination
The presence of N. ceranae spores was examined using haemocytometry. Abdomens of 10 randomly chosen bees from one cage were macerated with a mortar and pestle and suspended in 10 mL distilled water. After thorough mixing, a small amount of suspension was applied to a Thoma counting chamber. Spores were counted under a light microscope with 400x magnification on 16 large squares (volume: 0.064 mm3). For each group, 18–20 samples (10 bees per sample) were analysed.
Statistical analysis
Statistical analysis and plots were performed using R, version 3.4.4, with RStudio (R Core Team Citation2018). The significance level α = 0.05 was used in the statistical tests. The Shapiro-Wilk test was used to check data distribution normality. The Kruskal-Wallis test with Holm’s correction for multiple comparisons using the “agricolae” package was used to check the significance of differences between the groups. Survival analysis was carried out using the “survival” and “survminer” packages and on the basis of the original code, transforming laboratory data into the form required by these packages. The charts were made using the “ggplot2” package and the “Inkscape” vector graphics program to improve the readability of the charts.
Results
Syrup intake
The mean sugar syrup intake per bee since the time of infection to the end of the experiment is presented in . All groups inoculated 2 days after emergence had similar syrup intake levels, including the control group. In the case of bees inoculated 10 days after emergence, 2 infected groups had significantly higher syrup intake levels than the corresponding control (Kruskal-Wallis test with Holm’s correction for multiple comparisons, α = 0.05).
Figure 1. Mean daily syrup intake per bee since inoculation over 7 days of the experiment duration. Error bars present SD. Groups are marked under the x-axis, the dose is an inoculum concentration (spores/bee), 0 means control group. Treatments with the same lowercase above the error bars are not significantly different (Kruskal–Wallis test with Holm’s correction for multiple comparisons, α = 0.05).
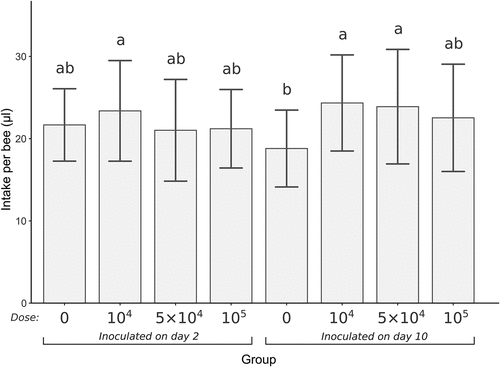
Mortality
Mean daily survival is presented in . Within the groups inoculated on the 2nd day post-emergence showed similar survival to control (), log-rank test: χ2 = 4.7, df = 3, p-value = 0.2). While there were no statistically significant differences, the highest mortality was observed in the group inoculated with the lowest spore concentration (104 spores/bee).
Figure 2. Percent of mean daily survival from inoculation to the end of the experiment. (a) bees inoculated on 2nd day post-emergence; (b) bees inoculated on 10th day post-emergence. Concentration of 0 spores/bee means control groups. Asterisks indicate significant differences compared to control (log-rank test, α = 0.05).
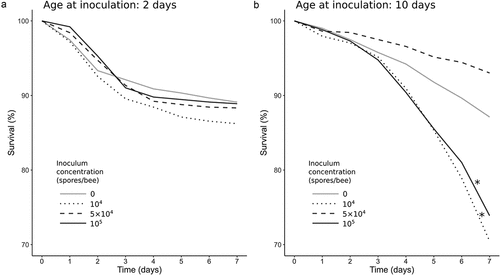
Among the bees inoculated at the age of 10 days, inoculum concentration impacted survival (), global log-rank test: χ2 = 88.3, df = 3, p-value = 2.4 × 10−19). Compared to control, two spore dosage variants caused increased mortality: the lowest dosage, 104 spores/bee (log-rank test: χ2 = 20.4, df = 1, p-value = 6 × 10−6) and the highest dosage, 105 spores/bee (log-rank test: χ2 = 13.4, df = 1, p-value = 3 × 10−4). For the survival data of both groups, the Cox proportional hazard model was demonstrated to be suitable (concordance index: 0.63); the hazard ratios in comparison to control were 2.35 and 2.00; p-values: 1.2 × 10−10 and 3.2 × 10−7, respectively. Inoculation with the 5 × 104 spores dosage, which is between the previously described inoculum concentrations, did not affect the bees’ survival (log-rank test: χ2 = 2.3, df = 1, p-value = 0.1).
Infection level
The infection level at the end of the experiment is shown on . In both controls, infection level was very low (in the 2-day age variant, mean spore load was 1.7 × 103 ± 5.1 × 103 SD; in the 10-day age variant, all counts equalled 0), which proves that infection in the treatment groups is the effect of artificial inoculation. There is a clearly-visible different infection development between the age groups in response to the administered infecting spore dosages. Within the groups infected at the age of 2 days, the highest level of infestation occurred with the lowest and the highest inoculum concentration (104 and 105 spores/bee, respectively). The middle inoculum concentration (5 × 104 spores/bee) resulted in a much lower infection level in this age group. This dependency was entirely different for the group infected at the age of 10 days, where the level of infection increases with an increase of the administered spore dosage. PCR analysis confirmed that the spores isolated from the bees at the end of the experiment were N. ceranae, rather than N. apis (not shown).
Figure 3. Distribution of N. ceranae spore counts after 7 days post-inoculation according to experimental variant. In the boxplots, the central line shows the median, box – interquartile range, whiskers – 95% confidence interval, and circles – outliers. The concentration of 0 spores/bee means control groups, which are presented on the left of each age variant. Treatments with the same lowercase above the boxplots are not significantly different (Kruskal–Wallis test with Holm’s correction for multiple comparisons, α = 0.05).
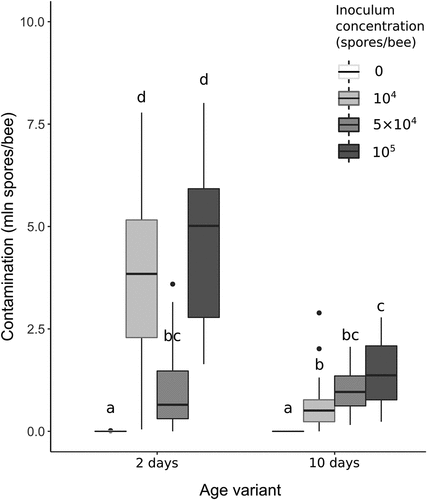
The bees inoculated on the 2nd day post-emergence showed considerable differences in contamination level, while among the bees inoculated on the 10th day, clearly noticeable differences in mortality were observed. Interestingly, in both cases, the smallest changes were caused by the medium inoculum concentration.
Discussion
Our results confirm that both studied factors, host age and inoculum concentration, have a significant impact on the development of Nosema ceranae infection. The investigation of variables combining their effects gives new insight into the dynamics of nosemosis development and the host response to the infection.
Syrup intake
In this study, syrup intake was not altered in groups inoculated on the 2nd day. Among the groups infected on the 10th day, syrup intake increased, but only in two dosage groups (the highest inoculum concentration, 105 spores/bee, did not affect syrup intake). Therefore, both factors, age and inoculum concentration, were demonstrated to impact syrup consumption. Consumption rate did not increase with an increase in the inoculum concentration in any age group. A different relationship was observed in other studies. Inoculation of honeybees on the 5th day post-emergence with N. ceranae spores resulted in increased syrup intake. The dose-effect was relevant: an inoculum concentration of 105 spores/bee caused a higher syrup consumption rate than the lower spore dosages (103, 104 and 5 × 104 spores/bee) (Martín-Hernández et al. Citation2011). Vidau et al. (Citation2011), after infecting bees at the age of 5 days with 1.25 × 105 N. ceranae spores/bee, observed a higher syrup intake than in control. Nosema infection causes energetic stress in the host’s body, which manifests in increased appetite and hunger levels (Mayack & Naug Citation2009). As the microsporidia lack mitochondria, they demand energy from the host’s internal environment (Germot et al. Citation1997). When a spore inoculates the host cell, sporogony and merogony begin. Through these two processes, it was observed that the parasites are surrounded by the host’s mitochondria, which deliver ATP (Higes et al. Citation2007; Tsaousis et al. Citation2008; Hacker et al. Citation2014; Han et al. Citation2019, Citation2020). Direct energy absorption by the parasites is not the only reason behind the host’s energy decline. Invasion also causes damage in the midgut epithelium, impairing nutrient absorption (Higes et al. Citation2007, Citation2008; Kurze et al. Citation2018). Infection activates the immune response, which is also expensive energy-wise (Schmid-Hempel Citation2005; Goblirsch et al. Citation2013). Interestingly, in our study, the consumption of the syrup matched neither the inoculum concentration nor the final infection rate. In the most infected groups, syrup intake did not increase.
Mortality
Increased mortality in a nosemosis infection is also a result of energetic stress (Higes et al. Citation2007; Mayack & Naug Citation2009). In our study, mortality in response to the inoculum concentration differed between age groups. For bees infected at 2 days of age, mortality was similar in all infected groups and control. Interestingly, the highest mortality occurred in the group infected with the lowest spore dosage (104 spores/bee). Greater differences occurred in the group infected at the age of 10 days. In this case, the groups infected with the lowest and the highest dosage (104 and 105 spores/bee, respectively) showed significantly higher mortality than control. The middle dosage (5 × 104 spores/bee) caused an unexpectedly high survival level. The effect of the inoculum concentration on bee survival was investigated before in bees infected on the 5th day post-emergence with dosages of 103, 104, 5 × 104 and 105 N. ceranae spores/bee. Mortality rates were related to the inoculum concentration: higher spore dosages resulted in higher mortality. On the 7th day post-infection, the highest cumulative mortality occurred in the group administered with 105 spores/bee dosage, reaching 93%. N. apis infection was investigated according to the same procedure, and a similar positive relationship between inoculum concentration and mortality was observed, but in general, mortality rates were lower than in the N. ceranae infection, with the highest value reaching 31% (Martín-Hernández et al. Citation2011). Higes et al. (Citation2007) infected 5-day-old bees with a inoculum concentration of 1.25 × 105 N. ceranae spores/bee, and after 7 days, an equally high mortality was observed (94%). In our study, neither group demonstrated such a high mortality, as the highest mean values did not exceed 30%, which is closer to N. apis rather than N. ceranae infection based on the studies described above. The dependency between inoculum concentration and mortality in the bees infected 10 days post-emergence was also much different from these studies, in which the bees were inoculated 5 days post-emergence. Much lower mortality rates were observed when younger bees were inoculated. The effect of the administered spore dosage on bee survival was studied by Eiri et al. (Citation2015) on bees infected in the larval stage with dosages of 104 and 4 × 104 spores/bee. There were no significant differences in survival between the groups after 30 days of testing post-emergence. Milbrath et al. (Citation2015) infected bees immediately after emergence. They were infected individually with an inoculum concentration of 3 × 104 spores/bee with N. ceranae, N. apis, or a mixture of spores of these two species in various proportions. Mortality for the first 10 days was low in all groups (less than 10% – cumulative value). Later groups infected with a mixture of spores showed an increase in mortality compared to control, which did not occur in either group infected with the spores of only one species. Porrini et al. (Citation2020) demonstrated over a 21-day period that survival depends on bee age at infection time. The bees were individually infected with a dosage of 2.5 × 104 N. ceranae spores/bee. Bees infected 3 days post-emergence had significantly higher survival compared to those infected 5 days post-emergence. In our study, the young bees, infected at the age of 2 days, were also characterised by high survival, even after administration of high inoculum concentration.
Infection level
Our studies have shown that both age and inoculum concentration are factors affecting the development of nosemosis in honey bees, albeit the relationship between these factors and the level of infection is not linear. The age of the bees at the time of infection may change the disease development pattern in response to the administered spore dosages. The significant effect of age on the development of infection with N. ceranae spores is confirmed by studies conducted in Spain on the Spanish honey bee (Apis mellifera iberiensis) (Urbieta-Magro et al. Citation2019). Bees of different ages were infected with a concentration of 1.14 × 105 spores/bee using two methods: individually and collectively. The level of infection was assessed genetically 7 days after infection, which means that both the vegetative and the spore forms of Nosema were tested. The research covered many different age groups (0–15 days after emergence). It was shown that the infected bees developed the highest level of infection within 48 hours post-emergence. In the remaining age groups (2–15 days after emergence), for bees which were older at the time of infection lower infection development was observed. This tendency was evident in both individually and collectively inoculated bees, but when infected individually, the level of infection was significantly higher in all age groups. In our research, the highest spore dosage had a similar concentration (105 spores/bee) to the dosage used in the experiment conducted by Urbieta-Magro et al. (Citation2019) and resulted in a similar effect: the level of infection in young bees was significantly higher than in those infected at 10 days of age.
On the other hand, a completely different relationship between the level of infection and the age of bees was observed when bees at 3 and 5 days of age were individually infected with a concentration of 2.5 × 104 spores/bee with N. ceranae spores obtained from three different regions of Argentina (Porrini et al. Citation2020). It was found that the contamination with N. ceranae spores reached a higher level in bees infected 5 days post-emergence. Porrini et al. (Citation2011a) investigated the effect of the concentration of N. ceranae spores and diet on the development of nosemosis infection. Bees at the age of 7 days were individually infected with the following concentrations: 4.6 × 104, 2.3 × 105 and 1.15 × 106 spores/bee. It was observed that in bees fed with pure sugar syrup, the level of infection depended on the infecting concentration, and with an increasing concentration, the spore load in the midgut increased. In bees fed with syrup enriched with a composition of vitamins and amino acids and in those fed with a syrup with added bee bread, a higher dosage also caused a higher level of infection, but the spore load was much higher. Thus, it has been shown that the infecting concentration and diet are factors significantly affecting the level of nosemosis infection in honey bees. Due to methodological differences, it is difficult to directly compare the level of bee infestation with Nosema spores in individual groups with the results of our research (primarily concentration, method of infection, age of bees and the time of assessing the level of infection). It can be noted, however, that the investigations showed a similar trend of the concentration-effect on nosemosis development in bees infected on the 7th day post-emergence to that observed in bees infected on the 10th day post-emergence in our study. Part of nosemosis research focuses not on the level of the infection itself, but on the concentration infecting 50% (ID50) and 100% (ID100) of the bees through individual inoculation. In this respect, the age of the bees also proved to be an important factor. A study conducted by Huang et al. (Citation2015) observed a high level of these indicators in newly-emerged bees (> 24 h) compared to older ones (5 and 14 days old). A comparison between these results and those obtained in a study by Urbieta-Magro et al. (Citation2019) leads to interesting conclusion: to infect 100% of newly-emerged bees, a very high concentration of spores (5 × 105) is required (Huang et al. Citation2015), but it is also in this age group that the average level of infection after the administration of a concentration of 1.14 × 105 spores proved to be significantly higher (together with 1-day-old bees) compared to the older age groups (2–15 days) (Urbieta-Magro et al. Citation2019). Thus, bees on the first day after emergence were the most resistant to infection, but if the infection did occur, it developed very intensely. Nosema bombi is a microsporidian parasite specific to the bumblebee host. The effect of the inoculum dosage and host age on two bumblebee species (Bombus terrestris and B. lucorum) was investigated. Age demonstrated to be a crucial factor to the occurrence of infection, with bees inoculated at the age of 2 days being twice as susceptible as at the age of 10 days. concentration effect also was important: higher dosages caused a considerable increase in infection prevalence (Rutrecht et al. Citation2007).
Summary
Our research has shown that both the age of the bees and the concentration of administered N. ceranae spores are factors influencing the development of nosemosis. Furthermore, these factors strongly affected the outcome of the disease, specifically, mortality and syrup intake. Interestingly, alterations in the magnitude of the tested indicators (i.e. syrup intake, mortality and final infection level) were not related to each other. The alterations did not directly intensify as concentration increased. Also the age of bees was a factor affecting infection. The experimental design involving a simultaneous investigation of the impact of these two factors across multiple variants led to unexpected results and gives new insight into nosemosis dependencies.
Disclosure statement
No potential conflict of interest was reported by the author(s).
Additional information
Funding
References
- Amdam GV, Aase ALTO, Seehuus S-C, Kim Fondrk M, Norberg K, Hartfelder K. 2005. Social reversal of immunosenescence in honey bee workers. Experimental Gerontology 40(12):939–947. DOI: 10.1016/j.exger.2005.08.004.
- Andrearczyk S, Kadhim M, Knaga S. 2014. Influence of a probiotic on the mortality, sugar syrup ingestion and infection of honeybees with Nosema spp. under laboratory assessment. Med Weter 70(12):762–765.
- Arismendi N, Vargas M, López MD, Barría Y, Zapata N. 2018. Promising antimicrobial activity against the honey bee parasite Nosema ceranae by methanolic extracts from Chilean native plants and propolis. Journal of Apicultural Research 57(4):522–535. DOI: 10.1080/00218839.2018.1453006.
- Audisio MC, Sabaté DC, Benítez-Ahrendts MR. 2015. Effect of Lactobacillus johnsonii CRL1647 on different parameters of honeybee colonies and bacterial populations of the bee gut. Beneficial Microbes 6(5):687–695. DOI: 10.3920/bm2014.0155.
- Baffoni L, Gaggìa F, Alberoni D, Cabbri R, Nanetti A, Biavati B, Di Gioia D. 2016. Effect of dietary supplementation of Bifidobacterium and Lactobacillus strains in Apis mellifera L. against Nosema ceranae. Beneficial Microbes 7(1):45–51. DOI: 10.3920/bm2015.0085.
- Borges D, Guzman-Novoa E, Goodwin PH. 2020. Control of the microsporidian parasite Nosema ceranae in honey bees (Apis mellifera) using nutraceutical and immuno-stimulatory compounds. PloS one 15(1):e0227484. DOI: 10.1371/journal.pone.0227484.
- Bravo J, Carbonell V, Sepulveda B, Delporte C, Valdovinos CE, Martin-Hernandez R, Higes M. 2017. Antifungal activity of the essential oil obtained from Cryptocarya alba against infection in honey bees by Nosema ceranae. Journal of Invertebrate Pathology 149:141–147. DOI: 10.1016/j.jip.2017.08.012.
- Buczek K, Deryło K, Kutyła M, Rybicka-Jasińska K, Gryko D, Borsuk G, Rodzik B, Trytek M. 2020. Impact of protoporphyrin lysine derivatives on the ability of Nosema ceranae spores to infect honeybees. Insects 11(8):504. DOI: 10.3390/insects11080504.
- Chen Y, Evans JD, Smith IB, Pettis JS. 2008. Nosema ceranae is a long-present and wide-spread microsporidian infection of the European honey bee (Apis mellifera) in the United States. Journal of Invertebrate Pathology 97(2):186–188. DOI: 10.1016/j.jip.2007.07.010.
- Chen Y, Evans JD, Zhou L, Boncristiani H, Kimura K, Xiao T, Litkowski AM, Pettis JS. 2009. Asymmetrical coexistence of Nosema ceranae and Nosema apis in honey bees. Journal of Invertebrate Pathology 101(3):204–209. DOI: 10.1016/j.jip.2009.05.012.
- Chen -X-X, Wang S, Gong H-R, Hu F-L, Zheng H-Q. 2015. Screening of natural herbs against Nosema ceranae in honeybees (Apis mellifera L.). In: Kwon HW, editor. 44th APIMONDIA international apicultural congress abstract book. Tae Hyoung Korea Inc. 208 pp.
- Chioveanu G, Ionescu D, Mardare A. 2004. Control of nosemosis - the treatment with “Protofil. Apiacta 39:31–38.
- Copley T, Chen H, Giovenazzo P, Houle É, Jabaji S. 2012. Prevalence and seasonality of Nosema species in Québec honey bees. The Canadian Entomologist 144(4):577–588. DOI: 10.4039/tce.2012.46.
- Corby-Harris V, Snyder L, Meador CAD, Naldo R, Mott B, Anderson KE. 2016. Parasaccharibacter apium, gen. nov., sp. nov., improves honey bee (Hymenoptera: Apidae) resistance to Nosema. Journal of Economic Entomology 109(2):537–543. DOI: 10.1093/jee/tow012.
- Costa C, Lodesani M, Maistrello L. 2010. Effect of thymol and resveratrol administered with candy or syrup on the development of Nosema ceranae and on the longevity of honeybees (Apis mellifera L.) in laboratory conditions. Apidologie 41(2):141–150. DOI: 10.1051/apido/2009070.
- Eiri DM, Suwannapong G, Endler M, Nieh JC. 2015. Nosema ceranae can infect honey bee larvae and reduces subsequent adult longevity. PloS one 10(5):e0126330. DOI: 10.1371/journal.pone.0126330.
- El Khoury S, Rousseau A, Lecoeur A, Cheaib B, Bouslama S, Mercier P, Demey V, Castex M, Giovenazzo P, Derôme N. 2018. Deleterious interaction between honeybees (Apis mellifera) and its microsporidian intracellular parasite Nosema ceranae was mitigated by administrating either endogenous or allochthonous gut microbiota strains. Frontiers in Ecology and Evolution 6:58. DOI: 10.3389/fevo.2018.00058.
- Ellis JD, Evans JD, Pettis J. 2010. Colony losses, managed colony population decline, and colony collapse disorder in the United States. Journal of Apicultural Research 49(1):134–136. DOI: 10.3896/IBRA.1.49.1.30.
- Emsen B, De La Mora A, Lacey B, Eccles L, Kelly PG, Medina-Flores CA, Petukhova T, Morfin N, Guzman-Novoa E. 2020. Seasonality of Nosema ceranae infections and their relationship with honey bee populations, food stores, and survivorship in a North American region. Veterinary Sciences 7(3):131. DOI: 10.3390/vetsci7030131.
- Engel P, Moran NA. 2013. The gut microbiota of insects – Diversity in structure and function. FEMS Microbiology Reviews 37(5):699–735. DOI: 10.1111/1574-6976.12025.
- Formato G, Rivera-Gomis J, Bubnic J, Martín-Hernández R, Milito M, Croppi S, Higes M. 2022. Nosemosis prevention and control. Applied Sciences 12(2):783. DOI: 10.3390/app12020783.
- Forsgren E, Fries I. 2010. Comparative virulence of Nosema ceranae and Nosema apis in individual European honey bees. Veterinary Parasitology 49(3–4):212–217. DOI: 10.1016/j.vetpar.2010.02.010.
- Franzen C. 2004. Microsporidia: How can they invade other cells? Trends in Parasitology 20(6):275–279. DOI: 10.1016/j.pt.2004.04.009.
- Fries I, Chauzat MP, Chen YP, Doublet V, Genersch E, Gisder S, Higes M, McMahon D, Martín-Hernández R, Natsopoulou M, Paxton R, Tanner G, Webster T, Williams G. 2013. Standard methods for Nosema research. Journal of Apicultural Research 52(1):1–28. DOI: 10.3896/IBRA.1.52.1.14.
- Fries I, Feng F, da Silva A, Slemenda SB, Pieniazek NJ. 1996. Nosema ceranae n. sp. (Microspora, Nosematidae), morphological and molecular characterization of a microsporidian parasite of the Asian honey bee apis cerana (Hymenoptera, Apidae). European Journal of Protistology 32(3):356–365. DOI: 10.1016/S0932-4739(96)80059-9.
- Gajger IT, Petriniec Z, Pinter L, Kozarić Z. 2009. Experimental treatment of Nosema disease with “Nozevit” phyto-pharmacological preparation. American Bee Journal 149(5):485–490.
- Germot A, Philippe H, Le Guyader H. 1997. Evidence for loss of mitochondria in microsporidia from a mitochondrial-type HSP70 in Nosema locustae. Molecular and Biochemical Parasitology 87(2):159–168. DOI: 10.1016/s0166-6851(97)00064-9.
- Gisder S, Schüler V, Horchler LL, Groth D, Genersch E. 2017. Long-term temporal trends of Nosema spp. infection prevalence in Northeast Germany: Continuous spread of Nosema ceranae, an emerging pathogen of honey bees (Apis mellifera), but no general replacement of Nosema apis. Frontiers in Cellular and Infection Microbiology 7:301. DOI: 10.3389/fcimb.2017.00301.
- Goblirsch M, Huang ZY, Spivak M. 2013. Physiological and behavioral changes in honey bees (Apis mellifera) induced by Nosema ceranae infection. PloS one 8(3):e58165. DOI: 10.1371/journal.pone.0058165.
- Hacker C, Howell M, Bhella D, Lucocq J. 2014. Strategies for maximizing ATP supply in the microsporidian E ncephalitozoon cuniculi: Direct binding of mitochondria to the parasitophorous vacuole and clustering of the mitochondrial porin VDAC. Cellular Microbiology 16(4):565–579. DOI: 10.1111/cmi.12240.
- Han B, Ma Y, Tu V, Tomita T, Mayoral J, Williams T, Horta A, Huang H, Weiss LM. 2019. Microsporidia interact with host cell mitochondria via voltage-dependent anion channels using sporoplasm surface protein 1. MBio 10(4):e01944–19. DOI: 10.1128/mBio.01944-19.
- Han B, Takvorian PM, Weiss LM. 2020. Invasion of host cells by microsporidia. Frontiers in Microbiology 11:172. DOI: 10.3389/fmicb.2020.00172.
- Higes M, Garcia-Palencia P, Martin-Hernandez R, Meana A. 2007. Experimental infection of Apis mellifera honeybees with Nosema ceranae (Microsporidia). Journal of Invertebrate Pathology 94(3):211–217. DOI: 10.1016/j.jip.2006.11.001.
- Higes M, Martín-Hernández R, Botías C, Bailón EG, González-Porto AV, Barrios L, Del Nozal MJ, Bernal JL, Jiménez JJ, Palencia PG, Meana A. 2008. How natural infection by Nosema ceranae causes honeybee colony collapse. Environmental Microbiology 10(10):2659–2669. DOI: 10.1111/j.1462-2920.2008.01687.x.
- Higes M, Martín-Hernández R, Meana A. 2010. Nosema ceranae in Europe: An emergent type C nosemosis. Apidologie 41(3):375–392. DOI: 10.1051/apido/2010019.
- Huang WF, Solter L, Aronstein K, Huang Z. 2015. Infectivity and virulence of Nosema ceranae and Nosema apis in commercially available North American honey bees. Journal of Invertebrate Pathology 124:107–113. DOI: 10.1016/j.jip.2014.10.006.
- Huang WF, Solter LF, Yau PM, Imai BS. 2013. Nosema ceranae escapes fumagillin control in honey bees. PLoS Pathogens 9(3):e1003185. DOI: 10.1371/journal.ppat.1003185.
- Kim HK, Lee MY, Lee ML, Choi YS, Byeon KH. 2017. Control of honeybee intracellular gut parasites, nosemosis. In: Kaptan B, Dayan E, editors. 45th APIMONDIA international apicultural congress abstract book. Istanbul. 53 pp.
- Kim JH, Park JK, Lee JK. 2016. Evaluation of antimicrosporidian activity of plant extracts on Nosema ceranae. Journal of Apicultural Science 60(2):167–178. DOI: 10.1515/jas-2016-0027.
- Kurze C, Le Conte Y, Kryger P, Lewkowski O, Müller T, Moritz RFA. 2018. Infection dynamics of Nosema ceranae in honey bee midgut and host cell apoptosis. Journal of Invertebrate Pathology 154:1–4. DOI: 10.1016/j.jip.2018.03.008.
- Lee JK, Kim JH, Jo M, Rangachari B, Park JK. 2018. Anti-nosemosis activity of aster scaber and artemisia dubia aqueous extracts. Journal of Apicultural Science 62(1):27–38. DOI: 10.2478/jas-2018-0003.
- MacInnis CI, Keddie BA, Pernal SF. 2020. Nosema ceranae (Microspora: Nosematidae): A sweet surprise? Investigating the viability and infectivity of N. ceranae spores maintained in honey and on beeswax. Journal of Economic Entomology 113(5):2069–2078. DOI: 10.1093/jee/toaa170.
- Maistrello L, Lodesani M, Costa C, Leonardi F, Marani G, Caldon M, Mutinelli M, Granato A. 2008. Screening of natural compounds for the control of Nosema disease in honeybees (Apis mellifera). Apidologie 39(4):436–445. DOI: 10.1051/apido:2008022.
- Martín-Hernández R, Bartolomé C, Chejanovsky N, Le Conte Y, Dalmon A, Dussaubat C, García-Palencia P, Meana A, Pinto MA, Soroker V, Higes M. 2018. Nosema ceranae in Apis mellifera: A 12 years postdetection perspective. Environmental Microbiology 20(4):1302–1329. DOI: 10.1111/1462-2920.14103.
- Martín-Hernández R, Botías C, Barrios L, Martínez-Salvador A, Meana A, Mayack C, Higes M. 2011. Comparison of the energetic stress associated with experimental Nosema ceranae and Nosema apis infection of honeybees (Apis mellifera). Parasitology Research 109(3):605–612. DOI: 10.1007/s00436-011-2292-9.
- Mayack C, Naug D. 2009. Energetic stress in the honeybee Apis mellifera from Nosema ceranae infection. Journal of Invertebrate Pathology 100(3):185–188. DOI: 10.1016/j.jip.2008.12.001.
- Milbrath MO, van Tran T, Huang W-F, Solter LF, Tarpy DR, Lawrence F, Huang ZY. 2015. Comparative virulence and competition between Nosema apis and Nosema ceranae in honey bees (Apis mellifera). Journal of Invertebrate Pathology 125:9–15. DOI: 10.1016/j.jip.2014.12.006.
- Milbrath MO, Xie X, Huang ZY. 2013. Nosema ceranae induced mortality in honey bees (Apis mellifera) depends on infection methods. Journal of Invertebrate Pathology 114(1):42–44. DOI: 10.1016/j.jip.2013.05.006.
- Moradi M. 2017. Use of thymus vulgaris ethanolic extract for Nosema apis control in honey bee. In: Kaptan B, Dayan E, editors. 45th APIMONDIA international apicultural congress abstract BOOK. Istanbul. 52 pp.
- Nanetti A, Ugolini L, Cilia G, Pagnotta E, Malaguti L, Cardaio I, Matteo R, Lazzeri L. 2021. Seed meals from brassica nigra and eruca sativa control artificial Nosema ceranae infections in Apis mellifera. Microorganisms 9(5):949. DOI: 10.3390/microorganisms9050949.
- Pohorecka K. 2004. Laboratory studies on the effect of standardized artemisia absinthium L. extract on Nosema apis infection in the worker Apis mellifera. Journal of Apicultural Science 48(2):131–136.
- Porrini MP, Fernandez N, Garrido P, Medici S, Gende LB, Medici SC, Eguaras MJ. 2011b. In vivo evaluation of antiparasitic activity of plant extracts on Nosema ceranae (Microsporidia). Apidologie 42(6):700–707. DOI: 10.1007/s13592-011-0076-y.
- Porrini LP, Porrini MP, Garrido MP, Müller F, Arrascaeta L, Iriarte PJF, Eguaras MJ. 2020. Infectivity and virulence of Nosema ceranae (Microsporidia) isolates obtained from various Apis mellifera morphotypes. Entomologia Experimentalis Et Applicata 168(4):286–294. DOI: 10.1111/eea.12902.
- Porrini M, Sarlo E, Medici S, Garrido P, Porrini D, Damiani N, Eguaras M. 2011a. Nosema ceranae development in Apis mellifera: Influence of diet and infective inoculum. Journal of Apicultural Research 50(1):35–41. DOI: 10.3896/IBRA.1.50.1.04.
- Ptaszyńska A. 2013. Wybrane aspekty budowy, taksonomii oraz biologii rozwoju mikrosporydiów z rodzaju Nosema (Selected aspects of the structure, development, taxonomy and biology of microsporidian parasites belonging to the genus Nosema). Med Weter 69(12):716–725.
- Ptaszyńska A, Borsuk G, Mułenko W, Wilk J. 2016b. Impact of vertebrate probiotics on honeybee yeast microbiota and on the course of nosemosis. Med Weter 72(2):430–434. DOI: 10.21521/mw.5534.
- Ptaszyńska A, Borsuk G, Zdybicka-Barabas A, Cytryńska M, Małek W. 2016a. Are commercial probiotics and prebiotics effective in the treatment and prevention of honeybee nosemosis C? Parasitology Research 115(1):397–406. DOI: 10.1007/s00436-015-4761-z.
- Ptaszyńska A, Trytek M, Borsuk G, Buczek K, Rybicka-Jasińska K, Gryko D. 2018. Porphyrins inactivate Nosema spp. microsporidia. Scientific Reports 8(1):1–11. DOI: 10.1038/s41598-018-23678-8.
- R Core Team. 2018. R: A language and environment for statistical computing. Vienna, Austria: R Foundation for Statistical Computing. https://www.R-project.org/
- Rodríguez-García C, Heerman MC, Cook SC, Evans JD, DeGrandi-Hoffman G, Olubukola B, Zhang Y, Huang S, Hamilton M, Chen YP. 2021. Transferrin-mediated iron sequestration suggests a novel therapeutic strategy for controlling Nosema disease in the honey bee, Apis mellifera. PLOS Pathogens 17(2):e1009270. DOI: 10.1371/journal.ppat.1009270.
- Roman A. 2006. Podstawy pszczelarstwa. Wrocław: Wydawnictwo Akademii Rolniczej we Wrocławiu.
- Rutrecht S, Klee J, Brown M. 2007. Horizontal transmission success of Nosema bombi to its adult bumble bee hosts: Effects of dosage, spore source and host age. Parasitology 134(12):1719–1726. DOI: 10.1017/S0031182007003162.
- Schmid-Hempel P. 2005. Evolutionary ecology of insect immune defenses. Annual Review of Entomology 50(1):529–551. DOI: 10.1146/annurev.ento.50.071803.130420.
- Shumkova R, Balkanska R, Hristov P. 2021. The herbal supplements NOZEMAT HERB® and NOZEMAT HERB PLUS®: An alternative therapy for N. ceranae infection and its effects on honey bee strength and production traits. Pathogens 10(2):234. DOI: 10.3390/pathogens10020234.
- Smith ML. 2012. The honey bee parasite Nosema ceranae: Transmissible via food exchange? PLoS one 7(8):e43319. DOI: 10.1371/journal.pone.0043319.
- Strachecka A, Krauze M, Olszewski K, Borsuk G, Paleolog J, Merska-Kazanowska M, Chobotow J, Bajda M, Grzywnowicz K. 2014b. Unexpectedly strong effect of caffeine on the vitality of western honeybees (Apis mellifera). Biochemistry (Moscow) 79(11):1464–1475. DOI: 10.1134/s0006297914110066.
- Strachecka A, Olszewski K, Paleolog J. 2015. Curcumin stimulates biochemical mechanisms of Apis mellifera resistance and extends the apian life-span. Journal of Apicultural Science 59(1):129–141. DOI: 10.1515/jas-2015-0014.
- Strachecka A, Olszewski K, Paleolog J, Borsuk G, Bajda M, Krauze M, Merska-Kazanowska M, Chobotow J. 2014a. Coenzyme Q10 treatments influence the lifespan and key biochemical resistance systems in the honeybee (Apis mellifera). Archives of Insect Biochemistry and Physiology 86(3):165–179. DOI: 10.1002/arch.21159.
- Tomaszewska B, Chorbiński P. 2013. Choroby owadów użytkowych. Wrocław: Wydawnictwo Uniwersytetu Przyrodniczego we Wrocławiu.
- Traver BE, Williams MR, Fell RD. 2012. Comparison of within hive sampling and seasonal activity of Nosema ceranae in honey bee colonies. Journal of Invertebrate Pathology 109(2):187–193. DOI: 10.1016/j.jip.2011.11.001.
- Tsaousis AD, Kunji ER, Goldberg AV, Lucocq JM, Hirt RP, Embley TM. 2008. A novel route for ATP acquisition by the remnant mitochondria of Encephalitozoon cuniculi. Nature 453(7194):553–556. DOI: 10.1038/nature06903.
- Urbieta-Magro A, Higes M, Meana A, Barrios L, Martín-Hernández R. 2019. Age and method of inoculation influence the infection of worker honey bees (Apis mellifera) by Nosema ceranae. Insects 10(12):417. DOI: 10.3390/insects10120417.
- Vidau C, Diogon M, Aufauvre J, Fontbonne R, Viguès B, Brunet J-L, Texier C, Biron DG, Blot N, El Alaoui H, Belzunces LP, Delbac F. 2011. Exposure to sublethal doses of fipronil and thiacloprid highly increases mortality of honeybees previously infected by Nosema ceranae. PLoS one 6(6):e21550. DOI: 10.1371/journal.pone.0021550.
- Xu Y, Chen X, Wang S, Hu F, Zheng H. 2017. Therapeutic potentials of herbal extracts in controling nosemosis in honey bees (Apis mellifera L.). In: Kaptan B, Dayan E, editors. 45th APIMONDIA international apicultural congress abstract book. Istanbul. 59 pp.