Abstract
The skeleton of most Porifera species consists of siliceous spicules that, after the sponge death, persist for a long time in the sediment. The reduced suspension occurring within the meadows of the seagrass Posidonia oceanica makes these habitats a stable deposit for sponge spicules. Spicules trapped into the network of rhizomes progressively buried, named “matte”, represent a sedimentary sequence interpretable on a temporal scale. By means of a vertical corer we collected samples of sediment at 0.5, 1, 1.5 and 2 m depth along the matte profile in order to quantify the amount of biogenic silica used to assess past dynamics of the sponge community in four P. oceanica meadows of the Ligurian sea. The content in biogenic silica was determined by spectrophotometric analysis of the sediments. While at Prelo Bay the values are constant in the core sample, a general decreasing trend with depth was observed in the meadows of Punta Pedale, while in the site of Punta Manara the major amount of silica was found deeper in the matte. The temporal variations of sponge abundance were probably due to historical anthropogenic factors. In the meadow of Bergeggi, BSi concentrations, significantly lower compared to all other sites, were likely caused from inputs from the close Savona harbor and strong bottom currents avoiding spicule deposition.
Introduction
In the last decades, a series of mass mortality events of structuring benthic organisms (especially sponges, bryozoans and gorgonians) led to a substantial simplification of the superficial benthic communities of the Mediterranean Sea (Coma et al. Citation2009; Bianchi et al. Citation2019).
Long-term monitoring and comparative observations of biodiversity through time (on ten-years to millennia time scale) are essential to understand and interpret possible changes occurred in the benthic marine communities related to past and recent climate vicissitudes, or induced by anthropogenic pressures (Cerrano et al. Citation2000; Roberts Citation2009; Lejeusne et al. Citation2010; Bianchi et al. Citation2012; Boero et al. Citation2015; Di Camillo & Cerrano Citation2015; Longobardi et al. Citation2017; Montefalcone et al. Citation2018; Costa et al. Citation2018b, Citation2019).
The Mediterranean Sea is recognised as a biodiversity hotspot (Bianchi & Morri Citation2000; Coll et al. Citation2010) also thanks to the presence of key species as the endemic seagrass Posidonia oceanica (Linnaeus) Delile, 1813, able to create extensive meadows from the surface down to 40 m depth that are considered one of the most important marine ecosystems in coastal waters (Boudouresque et al. Citation2006). Posidonia oceanica meadows are indeed identified as a priority habitat under the Habitat Directive (92/43/EEC). The high level of productivity they support and their capacity to create a three-dimensional environment, where an otherwise poor and less structured habitat would exist, ensures an extensive array of biodiverse associated flora and fauna (Unsworth et al. Citation2019). The effect of plant or algal canopies on sediment deposition and resuspension results in wave energy dissipation and in the reduction of water flow inside the meadow, where fragile erected organisms can grow partly sheltered (Costa et al. Citation2018a), under dim light conditions typical of deeper environments (e.g., coralligenous assemblages). In the last decades, P. oceanica meadows experienced a general decline throughout the Mediterranean basin, essentially due to multiple stressors linked to anthropogenic activities and climate change (Marbà et al. Citation2014; Oprandi et al. Citation2020); this process inevitably involved associated fauna and surrounding habitats that depend on the several ecosystem services provided by P. oceanica. Among the main zoological taxon characterizing coastal marine habitats, sponges are a key component of benthic communities. They are highly biodiverse and may play significant ecological roles such as bentho-pelagic coupling, food source, and provision of habitat for other organisms in these systems (Bell Citation2008).
Although previous studies reported no evidence regarding a characteristic Porifera population associated to P. oceanica meadows (Pansini & Pronzato Citation1985; Padiglia et al. Citation2018), it should be taken into account that the wide extent and the hiding ability of this habitat made it hard getting relevant information through traditional investigations. Bertolino et al. (Citation2012) by using an alternative method which implies retrospective analysis on sponge spicules proved that P. oceanica meadows represent an ideal environment where population dynamics of such abundant and ubiquitous taxa can be investigated. P. oceanica beds are characterized by the so-called “matte”, a root-rhizome layer consisting of lignified roots and rhizomes admixed with sediment that can be up to several meters thick (Romero et al. Citation1994). The matte works as a sediment trap whose content reflects a rather precisely temporal sequence of the entrapment. Most of the Demospongiae consist of siliceous spicules that, after the sponge death, persist for a long period in the sediments, since they are protected from dissolution and degradation. Consequently, the entrapped sediments, including spicules, can be a powerful proxy to reconstruct the abundance and diversity of sponge assemblages. Several studies on other valuable Mediterranean habitats (i.e., coralligenous) have recently proved that siliceous spicules represent an effective tool to reconstruct ancient sponges’ assemblages over millennia time scale and can be used as indicators of climate change (Bertolino et al. Citation2014, Citation2017a; b, Citation2019). Regarding P. oceanica meadows, spicules abundance and diversity can be interpreted on centenary temporal scale thanks to the plant ability to face sedimentation through the continuous vertical growth of its rhizomes (orthotropic growth), which have on average a growth rate comprised between 4 and 9 mm y−1 in undisturbed sites, and between 2 and 4 mm y−1 in polluted sites (Marbà et al. Citation2006).
Although an adequate knowledge of species diversity is needed in order to identify conservation priorities, sponges’ diversity assessment through the identification of spicules type is renowned as a time-consuming practice. Large-scale studies require faster ways to recognize species temporal and spatial patterns. In this regard, quantitative analysis of biogenic silica (BSi) by spectrophotometric techniques reported comparable results with the qualitative ones (Bertolino et al. Citation2012). As sponges are sessile organisms exhibiting a relatively low potential of dispersion their abundance possibly reflects local conditions as well as likely perturbations in the environment, both natural and anthropogenic.
This work aims at evaluating past dynamics of sponge assemblages in four P. oceanica meadows of the Ligurian Sea (NW Mediterranean) by quantifying the biogenic silica corresponding to the sponge spicules trapped into a 2 meters thick matte, and interpretating differences in the layers on a temporal scale, according to natural events and/or anthropogenic disturbances.
Materials and methods
Study area
Four P. oceanica meadows have been investigated along the Ligurian Riviera (NW Italy). Three meadows (Prelo, Punta Pedale and Punta Manara) were located on the east coast within a 9 Nm radius area, and one (Bergeggi) on the west coast, about 35 Nm apart from the eastern ones ().
Prelo (44°20ʹ11.99”N, 9°13ʹ37.56”E) is a small cove surrounded by rocks that opens up in the western side of the Tigullio Gulf (Ligurian Sea). It hosts a valuable P. oceanica meadow that has long been investigated since the 1960s. The meadow stretches up to only 15 m depth but in its shallower part it creates a récif-frangeant where plant leaves can reach the sea surface thanks to the sheltered conditions of the bay (Diviacco & Coppo Citation2006). P. oceanica typically grows on matte which in some areas results uncovered leaving a thick terrace visible, up to 1.5 m high (Bertolino et al. Citation2012). The meadow suffered from several anthropogenic impacts in the past, including anchoring and the construction of a small jetty that altered local hydrodynamics with consequences on P. oceanica cover (Lasagna et al. Citation2006).
Punta Pedale meadow stretches along the coast for about 1300 linear meters inside a large bay (44°18ʹ58.40”N, 9°13ʹ24.25”E) located 2 km to the south of Prelo and partly included in the zone C of the Portofino promontory Marine Protected Area. Similarly to Prelo, the offshore development of the meadow does not exceed 15 m depth due to the influence of the Entella River on the east, that caused a reduction in the coastal waters clarity (Morri et al. Citation1986). P. oceanica grows mainly on matte but its cover looks patchy, especially at shallow depths, approaching the tip of Pedale. The area has indeed undergone the dumping of earthy materials in the ‘70 that likely caused the regression of the meadow (Pansini & Pronzato Citation1975).
The Promontory of Punta Manara (44°15ʹ3.20”N, 9°24ʹ47.41”E) represents the easternmost site of the study area and it is characterized by a rocky cliff at whose base, on the eastern side, a P. oceanica meadow develops up to about 25 m depth. P. oceanica settles mainly on rocks near the coast then continues on matte and sand. As a result of multiple stressors, the meadow shows a jagged outline the closer it approaches the village of Riva Trigoso. The decline of the meadow can be ascribed to the landfill of digging materials and to the vicinity of shipbuilding activities, operating in the area since the late 1800s.
The Bergeggi meadow (44°13ʹ21.65”N, 8°26ʹ28.28”E) on the western Ligurian coast is included in the Marine Protected Area of Begeggi Island. Between the tip of Maiolo and the island of Bergeggi, the meadow reaches the maximum extent of about 400 m. The lower limit is located at 21 m depth but a regression of about 40 m is documented (Vetere & Pessani Citation1989). P. oceanica grows mainly on matte with a lower cover near the coast then the meadow becomes more dense and uniform towards the open sea. Massive beach nourishments interested the coastline over the years, badly affecting the meadow, which in some areas has been replaced by the other Mediterranean seagrass Cymodocea nodosa.
Sample collection and Si analysis
Field activity was carried out in summer 2019 by scuba diving. In each meadow sediment samples were collected at 10 m depth, where P. oceanica showed the higher cover and possibly in the presence of the thickest matte layer. By using a vertical corer manually operated, four samples were collected along the matte profile, at 0.5 m (A), 1 m (B), 1.5 m (C) and 2 m (D) depth respectively (). The sampling involved performing three replicates in each meadow, 10 m apart from each other ().
The biogenic silica present into sediment was treated following Bertolino et al. (Citation2012). The silica was extracted with the chemical method proposed by DeMaster (Citation1981) and Mortlock and Froelich (Citation1989) and measured by spectrophotometric analysis (Grasshoff et al. Citation1983). The procedure consists in adding 5 ml of H2O2 (10%) to 200 mg of dry sediment and, after 30 minutes, 5 ml of HCL (10%) were added to this initial compound. After being centrifuged (5000 rpm × 5 min) and dried, the samples (100 mg in duplicate) were resuspended in 50 ml of a NaOH solution (1 M) and immersed in water at 85°C, shaking them during alkaline extraction (Klein Citation2008). After 10 minutes of centrifuged at 4000 rpm, the supernatant obtained was used to determine the dissolved biogenic silica of the sponge spicules. With the molybdate blue spectrophotometric method at 810 nm (Grasshoff et al. Citation1983) silica concentration was measured, and a linear regression was plotted running through the values corresponding to 2, 3 and 5 h of extraction. The y-intercept of the regression line corresponded to the concentration of biogenic silica in the sample (DeMaster Citation1981).
The amount of biogenic silica in the core samples at the different depths was assumed as directly related to the sponge abundance in the surrounding area of the meadow during the past deposition periods of silicious spicules as suggested by Bertolino et al. (Citation2012).
Data analysis
Quantitative data of biogenic silica from Prelo and Manara, that had not a normal distribution, were analysed through the non-parametric Kruskal-Wallis test to detect significant differences between depth layers, while data from Punta Pedale and Bergeggi, that showed a normal distribution, were analysed through the parametric one-way analysis of variance (ANOVA). Four separated analyses were computed for the investigated meadows using PAST® (PAleontological STatistics) since the goal was not about investigating differences between meadows but rather differences between depths that were time related and then dependent on the own history of each meadow.
Results
In the meadow of Prelo, a matte thickness of only 1.5 m did not allow the deeper layer D (2 m) to be sampled. The three sampled layers (A, B, and C) showed very similar amounts of biogenic silica (i.e., 1989.7 µM Si·g−1, 1806.4 µM Si·g−1 and 2240.0 µM Si·g−1 respectively) and no significant differences (, ).
In the meadow of Punta Pedale, the highest amounts of biogenic silica were found at 0.5 m and 1.5 m depth, respectively layers A (3132.2 µM Si·g−1) and C (3554.9 µM Si·g−1), while layers B (2255.9 µM Si·g−1) and D (2158.5 µM Si·g−1) had similar lower values. A significant decrease of biogenic silica (ρ < 0.05) was detected between the shallower layer A and the deeper layer D.
Figure 3. Average values of biogenic silica (µMSi·g−1) along the matte profile in the four investigated P. oceanica meadows. * p < 0.05.
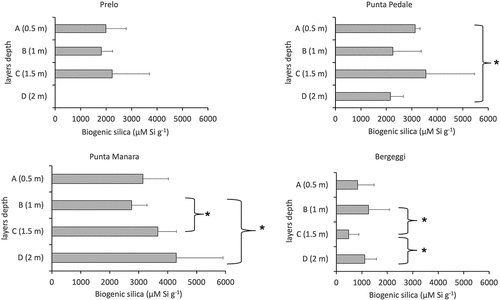
Table I. Results of significant Kruskal-Wallis test and one-way ANOVA on the biogenic silica content of sediments. * p < 0.05.
Overall, the highest total amount of biogenic silica was detected in the matte of Punta Manara meadow. Starting from the layer B (1 m), an increasing trend with depth can be recognized. Biogenic silica grew significantly (ρ < 0.05) from B (2755.3 µM Si·g−1) to C (3659.2 µM Si·g−1) and from B to D (4295.1 µM Si·g−1) (, ).
Oppositely to Punta Manara, the meadow of Bergeggi, showed the lowest silica values on record in the study area, averagely 2 to 4 times lower than others. Biogenic silica significantly decreased (ρ < 0.05) between 1 m (layer B, 1264.5 µM Si·g−1) and 1.5 m depth (layer C, 4796.4 µM Si·g−1), then significantly increased again from 1.5 m depth to 2 m depth (layer D, 1114.5 µM Si·g−1) (; ).
Discussion
The ability of Posidonia oceanica meadows to act as spicule traps, already suggested by Bertolino et al. (Citation2012), is confirmed by this study conducted on four meadows along the Ligurian Coast. The use of chemical analysis of BSi is a faster and easier method than the direct evaluation of the number and type of spicule fragments. Indeed, this approach does not require special recognition skills and the lab procedure can be easily learned and replicated. It can be used as a method to routinely assess the dynamics of sponge communities on a large number of meadows at a century scale. This statement is also supported by the fact that, by studying the fine fraction of sediments from different stations in the Ligurian Sea, Bertolino et al. (Citation2012) observed that sponge spicules represent almost the total BSi, while other siliceous remains, such as tests of diatoms and silicoflagellates, constitute a negligible fraction.
This study is based on the obvious hypothesis that the amount of BSi in a matte layer is directly related to the sponge biomass living in that period. Considering the assumption that Posidonia mattes regularly grow with an estimated rate between 4 and 9 mm y−1 in undisturbed sites, and between 2 and 4 mm y−1 in polluted sites (Marbà et al. Citation2006) and that the considered meadows are settled in places characterised by slightly stressed conditions, we can state that our study covers a time-span extending from recent to about 400 Years Before Present (YBP).
The first evidence arising from this study is that BSi, putatively related to sponge spicules, is present in all the surveyed meadows and levels. These spicules may have two different origins: (i) from sponge communities settled on the Posidonia rhizomes; (ii) from sponge communities settled far from the meadows and that have been transported by the current. Bavestrello et al. (Citation1996) provided data about the number of spicules present in the sediments outside meadows in a station very close to the meadow of Prelo Bay, which was studied by Bertolino et al. (Citation2012). The comparison of data (906 ± 188 vs 4240 ± 800 spicules g−1 of sediment) indicates a number of spicules four times higher inside than outside the meadow. Although this cannot be considered definitive, it suggests that the spicules present in the Posidonia matte are very likely to be largely supplied by sponges living on the P. oceanica rhizomes.
A second goal of this study is the clear difference among the tested meadows: the lowest amount of BSi was recorded in Bergeggi (~90 mM g−1) followed by Prelo (~200 mM g−1), Punta Pedale (~280 mM g−1) and Punta Manara (~340 mM g−1). Unfortunately, we don’t have a current estimation of the biomass of sponges inside the different meadows. Nevertheless, these data strongly suggest that meadows and related communities have likely experienced the same fate over time, as the Ecological Status of the investigated meadows (Oprandi et al. Citation2021) is in agreement with the amount of BSi (). The classification of the Ecological Status of the four investigated meadows likely displayed not only the actual status of the meadows themselves but, more generally their level of stress over time. The meadow of Punta Manara is protected from any river input by the promontory; on the contrary, those of Prelo and Punta Pedale receive the input of the Entella River (Corradi et al. Citation1980) and the Bergeggi meadow is very close to the Savona harbour that has been in operation since the mid-19th century. Moreover, the low amount of BSi found in the Bergeggi matte could also be invoked by hydrological conditions, as the strong bottom current that is generated at the channel between Punta del Maiolo and the island of Bergeggi could reduce the spicule sedimentation (Ferrari et al. Citation2014).
Figure 4. Average values of BSi in the four layers of each meadow plotted vs the Ecologica status index of the meadow (from Oprandi et al. Citation2021).
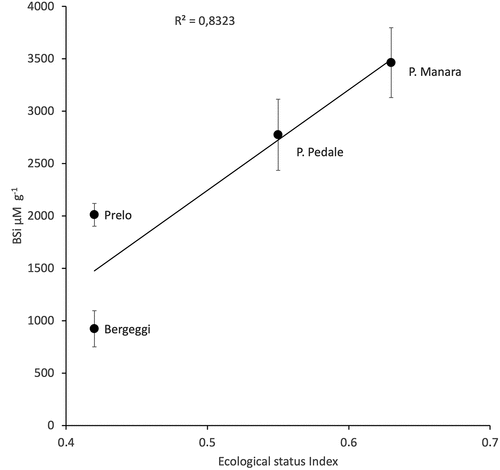
Finally, the variability of biogenic silica in the various layers of the four studied localities is probably related to the anthropogenic impacts that have exponentially increased in the last 200 years (Jiang et al. Citation2012) together with the natural variation related to global changes. In the Prelo meadow values are constant and in the same order of magnitude of those recorded during the survey of 2012 (Bertolino et al. Citation2012). In that survey some differences among layers were recorded and attributed to anthropogenic impact. The uniformity of the data observed during this study was probably related to a coarser pattern in the sample collection than in 2012 (every 50 cm instead of every 20 cm). At Punta Pedale, the minimum value of silica in the deepest layer could depend on the impact of the construction of the coastal road at the beginning of the twentieth century. The values of BSi progressively increased since that time. A recent increase in the abundance of sponge population in relation to the warming of the water was recorded in localities very close to this meadow (Bertolino et al. Citation2016). On the contrary, in recent times, the development of shipyards close to Punta Manara could have caused a progressive decrease in the sponge populations of this localities.
Although we are far from understanding a general pattern of the temporal variation of BSi stored in the sediment, which is in turn related to sponge abundance, at basin scale, the method here presented seems to be a potential powerful tool. Further large-scale studies coupled with the estimation of sponge biomass will be the baseline for the description of the variation at century level.
Ethical approval
All applicable national and institutional guidelines for animal testing, animal care, and use of animals were followed by the authors.
Sampling and field studies
All necessary permits for sampling and observational field studies have been obtained by the authors from the competent authorities and are mentioned in the text.
Acknowledgements
The authors wish to dedicate this work to the loving memory of their dear colleague and friend Prof. Riccardo Cattaneo-Vietti, who passed away in 2021 due to Covid-19.
Disclosure statement
No potential conflict of interest was reported by the author(s).
References
- Bavestrello G, Cattaneo‐vietti R, Cerrano C, Cerutti S, Sará M. 1996. Contribution of sponge spicules to the composition of biogenic silica in the Ligurian Sea. Marine Ecology 17(1–3):41–50. DOI: 10.1111/j.1439-0485.1996.tb00488.x.
- Bell JJ. 2008. The functional roles of marine sponges. Estuarine, Coastal and Shelf Science 79(3):341–353. DOI: 10.1016/j.ecss.2008.05.002.
- Bertolino M, Betti F, Bo M, Cattaneo-Vietti R, Pansini M, Romero J, Bavestrello G. 2016. Changes and stability of a Mediterranean hard bottom benthic community over 25 years. Journal of the Marine Biological Association of the United Kingdom 96(2):341–350. DOI: 10.1017/S0025315415001186.
- Bertolino M, Calcinai B, Cappellacci S, Cerrano C, Lafratta A, Pansini M, Penna A, Bavestrello G. 2012. Posidonia oceanica meadows as sponge spicule traps. Italian Journal of Zoology 79(2):231–238. DOI: 10.1080/11250003.2011.614641.
- Bertolino M, Calcinai B, Cattaneo-Vietti R, Cerrano C, Lafratta A, Pansini M, Pica D, Bavestrello G. 2014. Stability of the sponge assemblage of Mediterranean coralligenous concretions along a millennial time span. Marine Ecology 35:149–158. DOI: 10.1111/maec.12063.
- Bertolino M, Cattaneo-Vietti R, Costa G, Pansini M, Fraschetti S, Bavestrello G. 2017a. Have climate changes driven the diversity of a Mediterranean coralligenous sponge assemblage on a millennial timescale? Palaeogeography, Palaeoclimatology, Palaeoecology 487:355–363. DOI: 10.1016/j.palaeo.2017.09.020.
- Bertolino M, Costa G, Carella M, Cattaneo-Vietti R, Cerrano C, Pansini M, Quarta G, Calcagnile L, Bavestrello G. 2017b. The dynamics of a Mediterranean coralligenous sponge assemblage at decennial and millennial temporal scales. PLoS ONE 12:e0177945. DOI: 10.1371/journal.pone.0177945.
- Bertolino M, Costa G, Cattaneo-Vietti R, Pansini M, Quarta G, Calcagnile L, Bavestrello G. 2019. Ancient and recent sponge assemblages from the Tyrrhenian coralligenous over millennia (Mediterranean Sea). Facies 65(3):1–12. DOI: 10.1007/s10347-019-0573-4.
- Bianchi CN, Azzola A, Bertolino M, Betti F, Bo M, Cattaneo-Vietti R, Cocito S, Montefalcone M, Morri C, Opradi A, Peirano A, Bavestrello G. 2019. Consequences of the marine climate and ecosystem shift of the 1980-90s on the Ligurian Sea biodiversity (NW Mediterranean). The European Zoological Journal 86(1):458–487. DOI: 10.1080/24750263.2019.1687765.
- Bianchi CN, Morri C. 2000. Marine biodiversity of the Mediterranean Sea: Situation, problems and prospects for future research. Marine Pollution Bulletin 40:367–376. DOI: 10.1016/S0025-326X(00)00027-8.
- Bianchi CN, Morri C, Chiantore M, Montefalcone M, Parravicini V, Rovere A. 2012. Mediterranean Sea biodiversity between the legacy from the past and a future of change. In: Stambler N, editor. Life in the Mediterranean Sea: A look at habitat changes. New York: Nova Science Publishers. pp. 1–55.
- Boero F, Kraberg AC, Krause G, Wiltshire KH. 2015. Time is an affliction: Why ecology cannot be as predictive as physics and why it needs time series. Journal of Sea Research 101:12–18. DOI: 10.1016/j.seares.2014.07.008.
- Boudouresque CF, Charbonnel E, Coppo S, Le Direach L, Ruitton S. 2006. Les méthodes de surveillance des herbiers à Posidonia oceanica. In: Boudouresque CF, Bernard G, Bonhomme P, Charbonnel E, Diviacco G, Meinesz A, Pergent G, Pergent-Martini C, Ruitton S, Tunesi L, editors. Préservation et conservation des herbiers à Posidonia oceanica. RAMOGE Publication: Monaco. pp. 132–140.
- Cerrano C, Bavestrello G, Bianchi CN, Cattaneo-Vietti R, Bava S, Morganti C, Morri C, Picco P, Sara G, Schiaparelli S, Siccardi S, Sponga F. 2000. A catastrophic mass-mortality episode of gorgonians and other organisms in the Ligurian Sea (North-Western Mediterranean), summer 1999. Ecology Letters 3:284–293. DOI: 10.1046/j.1461-0248.2000.00152.
- Coll M, Piroddi C, Steenbeek J, Kaschner K, Ben Rais Lasram F, Aguzzi J, Ballesteros E, Bianchi CN, Corbera J, Dailianis T, Danovaro R, Estrada M, Froglia C, Galil BS, Gasol JM, Gertwagen R, Gil J, Guilhaumon F, Kesner-Reyes K, Kitsos MS, Koukouras A, Lampadariou N, Laxamana E, de la Cuadra Cm L-F, Lotze HK, Martin D, Mouillot D, Oro D, Raicevich S, Rius-Barile J, Saiz-Salinas JI, San Vicente C, Somot S, Templado J, Turon X, Vafidis D, Villanueva R, Voultsiadou E. 2010. The biodiversity of the Mediterranean Sea: Estimates, patterns, and threats. PLoS One 5. DOI: 10.1371/journal.pone.0011842.
- Coma R, Ribes M, Serrano E, Jimeneza E, Salat J, Pascual J 2009. Global warming enhanced stratification and mass mortality events in the Mediterranean. Proceedings of the National Academy of Sciences, United States of America 106:6176–6181. DOI: 10.1073/pnas.0805801106.
- Corradi N, Fanucci F, Firpo M, Piccazzo M, Traverso M. 1980. L’olocene della piattaforma continentale ligure da Portofino alla Spezia. Istituto Idrografico Marina Genova, F.C 1099:1–13.
- Costa G, Bavestrello G, Micaroni V, Pansini M, Strano F, Bertolino M. 2019. Sponge community variation along the Apulian coasts (Otranto Strait) over a pluri-decennial time span. Does water warming drive a sponge diversity increasing in the Mediterranean Sea? Journal of the Marine Biological Association of the United Kingdom 99(7):1519–1534. DOI: 10.1017/S0025315419000651.
- Costa G, Bertolino M, Pinna S, Bonaviri C, Padiglia A, Zinni M, Pronzato R, Manconi R. 2018a. Mediterranean sponges from shallow subtidal rocky reefs: Cystoseira canopy vs barren grounds. Estuarine, Coastal and Shelf Science 207:293–302. DOI: 10.1016/j.ecss.2018.04.002.
- Costa G, Betti F, Nepote E, Cattaneo-Vietti R, Pansini M, Bavestrello G, Bertolino M. 2018b. Sponge community variations within two semi-submerged caves of the Ligurian Sea (Mediterranean Sea) over a half-century time span. European Journal of Zoology 85:382–392. DOI: 10.1080/24750263.2018.1525439.
- DeMaster DJ. 1981. The supply and accumulation of silica in the marine environment. Geochimica Et Cosmochimica Acta 45:1715–1732. DOI: 10.1016/0016-7037(81)90006-5.
- Di Camillo CG, Cerrano C. 2015. Mass mortality events in the NW Adriatic Sea: Phase shift from slow- to fast-growing organisms. PLoS ONE 10:e0126689. DOI: 10.1371/journal.pone.0126689.
- Diviacco G, Coppo S. 2006. Atlante degli habitat marini della Liguria: Descrizione e cartografia delle praterie di Posidonia oceanica e dei principali popolamenti marini costieri. Regione Liguria: Genova. pp. 205.
- Ferrari M, Cabella R, Berriolo G, Montefalcone M. 2014. Gravel sediment bypass between contiguous littoral cells in the NW Mediterranean Sea. Journal of Coastal Research 30(1):183–191. DOI: 10.2112/JCOASTRES-D-12-00241.1.
- Grasshoff K, Ehrhardt M, Kremling K. 1983. Methods of seawater analysis. 2nd ed. Weinheim: Verlag Chemie. pp. 419.
- Jiang ZB, Chen QZ, Zeng JN, Zeng JN, Liao YB, Shou L, Liu JJ. 2012. Phytoplankton community distribution in relation to environmental parameters in three aquaculture systems in a Chinese subtropical eutrophic bay. Marine Ecology Progress Series 446:73e89. DOI: 10.3354/meps09499.
- Klein AW 2008. Analysis of biogenic silica from marine sediment in Maxwell Bay, Antarctica: A look at holocene climate history. Unpublished senior thesis, Middlebury College, Middlebury, Vermont.
- Lasagna R, Montefalcone M, Bianchi CN, Morri C, Albertelli G. 2006. Morphology of a Posidonia oceanica meadow under altered sedimentary budget. Biologia Marina Mediterranea 13:245–249.
- Lejeusne C, Chevaldonné P, Pergent-Martini C, Boudouresque CF, Pérez T. 2010. Climate change effects on a miniature ocean: The highly diverse, highly impacted Mediterranean Sea. Trends in Ecology & Evolution 25(4):250–260. DOI: 10.1016/j.tree.2009.10.009.
- Longobardi L, Bavestrello G, Betti F, Cattaneo-Vietti R. 2017. Long-term changes in a Ligurian infralittoral community (Mediterranean Sea): A warning signal? Regional Studies in Marine Science 14:15–26. DOI: 10.1016/j.rsma.2017.03.011.
- Marbà N, Díaz-Almela E, Duarte CM. 2014. Mediterranean seagrass (Posidonia oceanica) loss between 1842 and 2009. Biological Conservation 176:183–190. DOI: 10.1016/j.biocon.2014.05.024.
- Marbà N, Santiago R, Díaz-Almela E, Álvarez E, Duarte CM. 2006. Seagrass (Posidonia oceanica) vertical growth as an early indicator of fish farm-derived stress. Estuarine, Coastal and Shelf Science 67(3):475–483. DOI: 10.1016/j.ecss.2005.11.034.
- Montefalcone M, De Falco G, Nepote E, Canessa M, Bertolino M, Bavestrello G, Morri C, Bianchi CN. 2018. Thirty-year ecosystem trajectories in a submerged marine cave under changing pressure regime. Marine Environment Research 137:98–110. DOI: 10.1016/j.marenvres.2018.02.022.
- Morri C, Bianchi CN, Damiani V, Peirano A, Romeo G, Tunesi L. 1986. The marine environment between Punta della Chiappa and Sestri Levante (Ligurian Sea): Ecotipological outline and proposal of a biocenotic map. Bollettino Dei Musei E Degli Istituti Biologici dell’Università Di Genova 52:213–231.
- Mortlock RA, Froelich PN. 1989. A simple method for the rapid determination of biogenic opal in pelagic marine sediments. Deep Sea Research Part A. Oceanographic Research Papers 36:1415–1426. DOI: 10.1016/0198-0149(89)90092-7.
- Oprandi A, Bianchi CN, Karayali O, Morri C, Rigo I, Montefalcone M. 2021. RESQUE: A novel comprehensive approach to compare the performance of different indices in evaluating seagrass health. Ecological Indicators 131:108118. DOI: 10.1016/j.ecolind.2021.108118.
- Oprandi A, Mucerino L, De Leo F, Bianchi CN, Morri C, Azzola A, Benelli F, Besio G, Ferrari M, Montefalcone M. 2020. Effects of a severe storm on seagrass meadows. Science of the Total Environment 748:141373. DOI: 10.1016/j.scitotenv.2020.141373.
- Padiglia A, Cadeddu B, Ledda FD, Bertolino M, Costa G, Pronzato R, Manconi R. 2018. Biodiversity assessment in Western Mediterranean marine protected areas (MPAs): Porifera of Posidonia oceanica meadows (Asinara island MPA) and marine caves (Capo Caccia–Isola Piana MPA) of Sardinia. The European Zoological Journal 85(1):409–422. DOI: 10.1080/24750263.2018.1525440.
- Pansini M, Pronzato R. 1975. Analisi preliminari sulla distribuzionc dei Poriferi in aree sottoposte a differenti tipi di inquinamento. Bollettino dei Musei e degli Istituti Biologici dell’Università di Genova 43:21–32.
- Pansini M, Pronzato R. 1985. Distribution and ecology of epiphytic Porifera in two Posidonia oceanica (L.) Delile Meadows of the Ligurian and Tyrrhenian Sea. Marine Ecology 6(1):1–11. DOI: 10.1111/j.1439-0485.1985.tb00316.x.
- Roberts C. 2009. The Unnatural History of the Sea. Washington, DC: Island Press.
- Romero J, Pérez M, Mateo MA, Sala E. 1994. The belowground organs of the Mediterranean seagrass Posidonia oceanica as a biogeochemical sink. Aquatic Botany 47(1):13–19. DOI: 10.1016/0304-3770(94)90044-2.
- Unsworth RK, McKenzie LJ, Collier CJ, Cullen-Unsworth LC, Duarte CM, Eklöf JS, Jones BL, Nordlund LM. 2019. Global challenges for seagrass conservation. Ambio 48(8):801–815. DOI: 10.1007/s13280-018-1115-y.
- Vetere M, Pessani D. 1989. Morfologia e fruttificazione della prateria di Posidonia oceanica di Bergeggi (Liguria). Oebalia 15(1):351–354.