Abstract
Plastic pollution in the environment has become a much-discussed issue worldwide. In recent decades, the contamination of all environments has become increasingly evident, in particular, that of water is highly concerning. Ingestion by different animal species under natural conditions has also been demonstrated. Among these is the Talitrid Amphipod Cryptorchestia garbinii, which lives on the banks of the internal waterways and lakes’ shorelines. As detritivores species, it is very exposed to microplastics that can be ingested, probably mistaking them for food. Aiming to highlight the microplastic ingestion and the role of this species as an entry point for the food web, we analyzed 80 specimens from 4 sites along the shores of Garda Lake, one of the first lakes in Italy to be studied for this type of contamination. The microplastics ingested were observed and quantified through the Nile Red staining method. We were able to verify the presence of ingested microplastics in all the samples analyzed and, therefore, in the food web. This species could serve as valuable natural models of plastic exposure. Microplastic sentinel species can be used as a proxy for environmental exposure and ecosystem monitoring tools to quantify and assess the impacts of microplastic contamination.
Introduction
Plastic production has increased every year since 1941, when R. Whinfield and J.T. Dickson developed the thermoplastic resin, polyethylene terephthalate PET (da Silva et al. Citation2020). In 2020 only about 33% of plastic produced was recycled in Europe; the remainder was stored in landfills or was sent to energy recovery (Plastics Europe, Citation2021). At the end of the cycle, plastic waste, if improperly handled, may contribute to the input and dispersion of microplastics into the environment and to the generation of contaminated environmental matrices (de Souza Machado et al. Citation2018; Kallenbach et al. Citation2022). Eighty percent of the plastics produced globally are of petrochemical origin and, therefore, not biodegradable but only recyclable (Urbanek et al. Citation2018). This plastic produced and accumulated in the environment will be fragmented into increasingly smaller particles. Any plastic particle < 5 mm in diameter is commonly defined as “microplastic” (GESAMP; 2015; Lambert & Wagner Citation2018). The microplastics (MPs) can be classified based on their origin as “primary” MPs, produced, or directly dispersed in the environment with these dimensions, and “secondary” MPs are the result of the degradation from macroplastics (Boucher & Friot Citation2017), IUCN report to its slow rate of degradation in nature (Shahnawaz et al. Citation2019). Since the beginning of this century, plastic has been considered an emerging pollutant due to almost all latitudes of the Earth, from tropical to arctic areas (Iannilli et al. Citation2019; Schwarzer et al. Citation2022), and in all waters of the Earth, both marine and freshwaters (Van Cauwenberghe et al. Citation2013; Faure et al. Citation2015; Imhof et al. Citation2018; Sighicelli et al. Citation2018). The distribution is influenced by various factors such as wind, geostrophic circulation, turbulence, and oceanographic current. The plastics’ properties (durability, lightness, composition, shape, size) can cause their transport and aggregation to influence the distribution (Li et al. Citation2018). In recent decades, a lot of research has been done to evaluate plastic pollution in freshwaters, through the analysis of sediments and internal freshwater ecosystems, including Lake Garda (Imhof et al. Citation2013, Citation2018; Faure et al. Citation2015). This lake accumulates a significant presence of microplastic contaminations (Nel et al. Citation2018), even if the lack of standardized collection and analysis methods makes comparisons with others hard to do. Garda is the largest lake in Italy; it is located in an industrialized and urbanized region and is an important tourist site from everywhere in Europe, therefore greatly subject to environmental pollution (Imhof et al. Citation2013; Sighicelli et al. Citation2018).
MPs pollution can be monitored by direct collection in the water bodies. Still, it is essential to consider if this contamination is transferred to the biota and, consequently, to the food web. For this reason, it is imperative to develop bioindicators that can indicate this (Fossi et al. Citation2018; Corami et al. Citation2022). The talitrid amphipod Cryptorchestia garbinii Ruffo et al. (Citation2014) is a semi-terrestrial species that live on the banks of rivers and lakes in central-southern Europe and is a detritivorous, polyphagous, and scavenger species (Ruffo et al. Citation2014). It is discontinuously distributed along the Garda shores, and it has been used as a crustacean model in studies on cellular differentiation and expression of genes as other amphipod species (Davolos et al. Citation2015, Citation2018). Iannilli et al. (Citation2020) demonstrated that it could ingest small plastic fragments dispersed in the environment together with organic debris in three volcanic lakes in Central Italy: Albano, Bracciano, and Vico. We aimed to use this species as a model to monitor if MPs’ contamination from 4 sites at Garda Lake entered the food web, analyzing MPs ingested by the Nile Red method. These amphipods are key species in the energy flow of inland shores. Feeding both on terrestrial and aquatic material, they are affected by the contamination of both ecosystems. C. garbinii constitute an important food source for other invertebrates, fishes and birds (Griffiths et al. Citation1983). They can therefore transfer the contamination of microplastics from the aquatic to the terrestrial trophic chain.
Material and methods
Sites
Lake Garda is the largest lake in Italy, has an area of about 370 km2, is 65 m above the sea level, with maximum fluctuations of about 1 m, and is surrounded by three regions: Lombardy, Veneto, Trentino-Alto-Adige. It has a maximum depth of 346 m, while the average is 135 m. The main tributary is the Sarca river, whose sources are in the Alps above, while the river Mincio, South of the lake, is the emissary. The inflows of waters is regulated through dams and locks. The flora is particular and diversified between shore and hill vegetation due to the characteristics of the lake itself and the surrounding area; the fauna of the lake is rich even if the endemic species are at risk of extinction due to overfishing, poor water quality, and the arrival of invasive species (Turri Citation1978). Several alien species were introduced in the last decades, enough to be considered a freshwater hotspot of xenodiversity in Europe (Lopez-Soriano et al. Citation2018).
The waters are used in agriculture, industry and fishery. They are also used, after treatment, for drinking purposes. Moreover, it is an important resource for recreation and tourism from Italy and Europe. The southern part is large and shallow (less than 50 m) and interested by the presence of most of the tourist sites and recreational places which can also have a direct influence on water quality of the Lake.
Sampling sites are showed in where we also reported data about population density in the main locations on the Lake.
Cryptorchestia garbinii
Sample collection
In this work, the semi-terrestrial amphipod species Cryptorchestia garbinii (Ruffo et al. Citation2014) was used as a model organism to study the ingestion of microplastics in the natural environment (). Eighty specimens were collected along the shores of Lake Garda at four different sites (10 male and 10 female each site): Bardolino on the East shore, Lugana (Sirmione) on the South coast, Riva del Garda on the North coast, and finally Toscolano-Maderno on the West bank of the lake. C. garbinii specimens were collected in October 2019, using an aspirator or by hand, among the shore sediments near the water line and fixed in 75% ethanol in 50 mL Falcon tubes.
Sample treatment
The protocol used aimed to highlight the microplastics ingested by Nile red staining, it is an easy, fast, and low-cost method for identifying and quantifying microplastics in environmental matrices. Nile red is a solvatochromic dye which stains hydrophobic plastics emitting higher fluorescence at low wavelengths (Shim et al. Citation2016; Erni-Cassola et al. Citation2017).
In the present work we treated the samples as described in Iannilli et al. (Citation2019). After identification and dissection, the digestive tubes were extracted, and placed in Eppendorf, in pairs with separate sexes, with H2O2 30% added and maintained for 48 hours, at a constant temperature of 60°C to obtain the degradation of the organic matter. After that, the samples were filtered using a glass Buchner filtering kit on black polycarbonate membranes (Cyclopore Track Etched Membrane, 0.2 UM, 47 mm, WhatmanTM) to make the microplastics stand out better against the dark background. The filters were washed with n-hexane and then stained with few drops of Nile red working solution on the surface. Nile red was dissolved in acetone and diluted in n-hexane (5 mg/L NR working solution) according to the protocol by Shim et al. (Citation2016) and Iannilli et al. (Citation2019) to obtain a work staining solution. The stained filters were observed under a microscope (Nikon Eclipse Ci-L) in green fluorescence (ex. 450–490; em. 505–520 nm) to highlight microplastic particles. Four negative control samples were processed with the same protocol to check for any MPs contamination during the analysis. In this work, the 40 filters obtained (each with two specimens’ digestive tubes) and negative control samples were observed. Because the morphological identification can help in understanding the origin and fate of plastic pollution, the microplastic particles found were observed, measured, and photographed with the camera Nikon DS-Ri2 and the Software NIS-elements BR4.50. We used the Aspect Ratio (AR) calculation (a ratio between the width and the particle’s height) to distinguish fibers from fragments.
Statistical analysis
Data analysis (t-test for equal means) was performed using the software Past, Paleontological Statistics Version 4.08 to determine the level of significance among sites.
Results and discussion
The Nile Red staining applied to the material ingested by Cryptorchestia garbinii made it possible to highlight the microplastic particles ingested through the observation under a microscope in green fluorescence (ex. 450–490 nm; em. 515–565 nm) ().
Figure 3. Microscope images of microplastics on a filter stained with Nile red, in green fluorescence (ex. 450–490; em 515–565 nm) (left) and brightfield (right), with measurements.
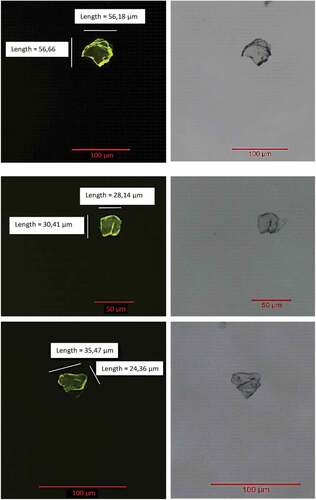
The use of Nile red (NR) fluorescent staining allows the visualization of MPs in environmental matrices using fluorescence microscopy, while this method does not provide the chemical identification of the MPs as are able to do other techniques, like FTIR and Raman (Erni-Cassola et al. Citation2017). However, although NR interaction with different polymers varies leading to a different fluorescence, methods for polymer classification based on NR have only recently been proposed (Meyers et al. Citation2022).
The MPs ingestion was demonstrated, in the last years, in a wide range of taxa, including crustaceans. The extent of adverse effects on biota is not fully understood, although it is known that they negatively affect many species (Jamieson et al. Citation2019). Many invertebrates feed on collecting, sorting, and digesting the organic particulates; since this plastic debris occupies the same size range, a wide range of invertebrates can ingest them by confusing them with food. Ingestion and accumulation within organisms cause physical and mechanical damage, obstructions of the gastrointestinal tract, and consequent pseudo satiety, resulting in reduced food intake and, therefore, can lead to the individual’s death. Moreover, other chemical compounds used as additives easily leach out from degraded plastic objects and exhibit adverse biological effects (Ficociello et al. Citation2021; Cosentino et al. Citation2022). Several studies have been carried out on the ingestion of microplastics by Amphipods, both in laboratory conditions (Blarer & Burkhardt-Holm Citation2016; Redondo-Hasselerharm et al. Citation2018a; Haegerbaeumer et al. Citation2019) and under natural conditions (Iannilli et al. Citation2018, Citation2019, Citation2020). Other benthic macroinvertebrates taxa, such as Annelids, Isopods, and the Bivalves (Redondo-Hasselerharm et al. Citation2018a), Trichoptera (Windsor et al. Citation2019), Ephemeroptera (Windsor et al. Citation2019), Chironomids (Nel et al. Citation2018; Lin et al. Citation2021) and Oligochaetes (Silva et al. Citation2021) can ingest microplastics. Microplastics have been demonstrated in all groups analyzed, thus highlighting a potential risk in the freshwater ecosystems.
In this work, a total of 80 specimens of C. garbinii were processed, 20 for each sampling site, of which 10 were females and ten males. Based on microscopic observation, all the samples analyzed contained microplastics belonging, for the most part, to the category of fragments. We can’t infer if this is related to the feeding behaviour. The most likely reason is that fragments are the most present category in Garda waters’ plastic litter, as demonstrated by Sighicelli et al. (Citation2018), though they referred to larger particles.
The difference in the number of microplastics found in the digestive tracts of male and female individuals is not statistically significant, so the data were therefore treated as a whole. The size ranges between 9.09 and 706.16 μm (length) (), and more than 87% of them are small microplastics (SMPs, <100 µm), and 70% are smaller than 50 μm. The size of the microplastics found is in line with the study conducted by Iannilli et al. in 2020 on the lakes of Lazio (); the mean size is about 60 µm (length) and 30 µm (width). The small size of the particles makes them particularly dangerous for biota because they reach cellular size. All microplastics can potentially cause sublethal effects themselves still, to date, little investigated, however, smaller particles may have more significant consequences within organisms because they may end up in the tissues or even inside the cells (Prinz & Korez Citation2020). It has also been shown that microplastic pollution increases with decreasing size of plastic fragments (Enders et al. Citation2015; Imhof et al. Citation2016).
Table I. Microplastic particles size measured in μm as length (the longest dimension) and width (perpendicular to the length).
In all four sites, we found 85 MPs with fiber shape (length>3*width), about 14.8% of the total (). The fibers percentage ranges from 12% at Toscolano Maderno to 19% at Bardolino (). Corami et al. (Citation2020) attribute the origin of the fibers in waters mainly to the washing of clothing and the lack of or poor wastewater filtration. Many fibers are too small to be removed by urban sewage systems and, as a result, they will be flushed into the lake. The more significant accumulation of fibers in one site rather than another can be attributed not only to the greater volumes of wastewater treated (depending on the population density and, in this case, also for tourism) but also to the type of plant and its purification capacity. Koutnik et al. (Citation2021) observed that the microplastic shape could affect their environmental diffusion, influencing transport. The fibers resulted in abundant sediment in coastal areas, indicating that they are easily transported from the source. Fibers are unexpectedly concentrated in glaciers, probably because they can be suspended and transported by wind (Koutnik et al. Citation2021).
Figure 5. Images of fiber observed on a filter stained with Nile red, in green fluorescence (ex. 450–490; em 515–565 nm) (left) and brightfield (right).
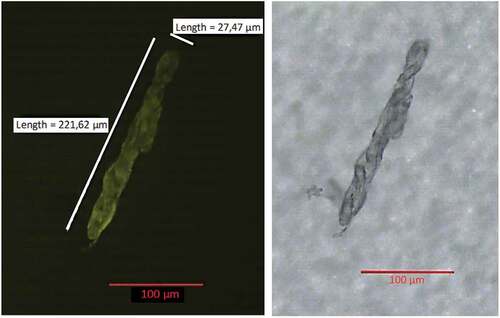
We observed a mean value of 7.13 (±2.11) microplastics in each specimen analyzed, while in the negative procedural control tests, no microplastic particles were found, hence, contamination was efficiently minimized by the procedures. This value is slightly higher than the MP averages found in C. garbinii in the lakes of Lazio (Italy) conducted with the same methods (lake of Albano: 2.2–1.8 MP/ind, lake Bracciano: 5–4.6 MP/ind) (Iannilli et al. Citation2020). In particular, the average MPs values found in the four sites are: Bardolino 8,5 (±1.1) particles/ind; Lugana 9.3 (±1) particles/ind; Riva 5.4 (±1.6) particles/ind; while in Toscolano-Maderno 5.5 (±1) particles/ind. These differences are statistically significant, as shown in .
Figure 7. Average number of MPs ingested per specimen (± standard error) by sampling site. Different letters indicate significant differences according to t test (P ≤ 0.05).
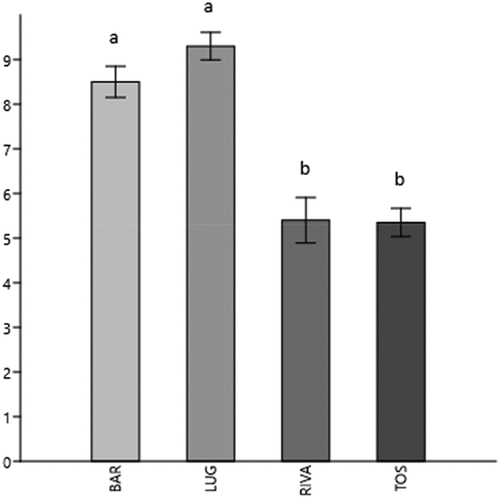
The MPs accumulation in this species is certainly in proportion with the abundance present in water and sediment, but these organisms can ingest the MPs in a different way depending on the type of sediment. There may be a connection between the sediment structure such as the granules’ size or distribution and the quantity or size of the MPs ingested by C. garbinii which individuals mistake for food. Fine sediment with high organic content, for example, favour the accumulation of microplastic particles. In contrast, large sediment grain sizes make the MPs adhere less and accumulate more in the interstices, leading to deeper penetration into larger interstitial spaces of the sediment (Imhof et al. Citation2018). Zbyszewski et al. (Citation2014) and Ballent et al. (Citation2016) observed the same in California lakes. A positive relationship between the type of sediment and the abundance of MP was also observed in our study. In the sampling sites where there is a small layer of large grains and then a layer of clay below with organic material deposited by the waves, more particles of MP were found in the organisms because the place where animals live coincides with the accumulation site of the MP deposited; therefore, these will be bioavailable to be mistaken for food and ingested. While in the places where the sediments were thicker and with a greater thickness in depth, the MPs deposited by the water will create a concentration gradient of the particles downwards, and in this case, C. garbinii living within a few centimetres from the surface will have less likelihood of encountering and ingesting the plastic fragments.
In the area of Bardolino and Lugana the microplastic particles seems to be more available to ingestion by C. garbinii. From we can observe that in the southeastern part of the lake we have the most residential population density. This factor is often recognized as positively related with microplastic pollution, in particular, high concentrations of fibers characterize the waters of densely populated areas, in consequence of the laundering of synthetic materials (Talbot & Chang Citation2022). Moreover, currents and wind could accumulate a larger amount of plastic litter in the south eastern part of the lake. There is the “peler” wind that blows from north to south of the lake, and in particular, Lovato & Pecenik (Citation2012) highlighted that the wind named “ora sul Garda” has the effect of accumulation and retention of buoyant litter in the area of Bardolino.
According to the study by Imhof et al. in 2018 on sediments, the shore area where most of the MP fragments are found is near the water (water line and drift line), which coincides with the living area of C. garbinii. Moreover, the MPs found have a size compatible with the MPs ingested. Among these, the water line has a lower MPs accumulation because of the action of the waves, which continuously have the effect of depositing and removing. At the same time, the drift line has the greatest MP deposit and is also the deposit of organic matter, which is the food source for C. garbinii (Imhof et al. Citation2018).
Conclusion
It is important to identify sentinel species to monitor the distribution and impact of MPs in our environment. C. garbinii, considered a model organism for biological investigations, can also be considered a suitable bioindicator of MP contamination and an entry point of MPs into the food web. The presence of microplastics in all the specimens tested at all sites indicates that this emerging pollutant penetrates the food web, becoming available for higher trophic levels, potentially up to humans.
Disclosure statement
The authors declare that they have no known competing financial interests or personal relationships that could have appeared to influence the work reported in this paper.
References
- Ballent A, Corcoran PL, Madden O, Helm PA, Longstaffe FJ. 2016. Sources and sinks of microplastics in Canadian Lake Ontario nearshore, tributary and beach sediments. Marine Pollution Bulletin 110(1):383–395. DOI: 10.1016/j.marpolbul.2016.06.037.
- Blarer P, Burkhardt-Holm P. 2016. Microplastics affect assimilation efficiency in the freshwater amphipod Gammarus fossarum. Environmental Science and Pollution Research 23(23):23522–23532. DOI: 10.1007/s11356-016-7584-2.
- Boucher J, Friot D. 2017. Rep. primary microplastics in the Oceans: A global evaluation of sources. Vol. 43. Gland, Switzerland: The International Union for Conservation of Nature (IUCN).
- Corami F, Rosso B, Bravo B, Gambaro A, Barbante C. 2020. A novel method for purification, quantitative analysis and characterization of microplastic fibers using micro-FTIR. Chemosphere 238:124564. DOI: 10.1016/j.chemosphere.2019.124564.
- Corami F, Rosso B, Iannilli V, Ciadamidaro S, Bravo B, Barbante C. 2022. Occurrence and characterization of small microplastics (<100 μm), additives, and plasticizers in Larvae of simuliidae. pp. 1–16.
- Cosentino S, Aureli F, Iannilli V. 2022. Bisphenols A and its analogues induce genotoxic damage in marine and freshwater amphipods. Environmental Advances 7:100183. DOI: 10.1016/j.envadv.2022.100183.
- da Silva FZ, Bastos IC, Hirata DB. 2020. Effects of temperature and concentration on the determination of PET viscosity. Journal of the Brazilian Chemical Society 31:1891–1897. DOI: 10.21577/0103-5053.20200084.
- Davolos D, Chimenti C, Ronci L, Setini A, Iannilli V, Pietrangeli B, De Matthaeis E. 2015. An integrated study on Gammarus elvirae (Crustacea, Amphipoda): Perspectives for toxicology of arsenic-contaminated freshwater. Environmental Science and Pollution Research 22(20):15563–15570. DOI: 10.1007/s11356-015-4727-9.
- Davolos D, Vonk R, Latella L, De Matthaeis E. 2018. The name of a model species: The case of Orchestia cavimana (Crustacea: Amhipoda: Talitridae). The European Zoological Journal 85(1):229–231. DOI: 10.1080/24750263.2018.1473513.
- de Souza Machado AA, Kloas W, Zarfl C, Hempel S, Rillig MC. 2018. Microplastics as an emerging threat to terrestrial ecosystems. Global Change Biology 24(4):1405–1416. DOI: 10.1111/gcb.14020.
- Enders K, Lenz R, Stedmon CA, Nielsen TG. 2015. Abundance, size and polymer composition of marine microplastics ≥ 10 μm in the Atlantic Ocean and their modelled vertical distribution. Marine Pollution Bulletin 100(1):70–81. DOI: 10.1016/j.marpolbul.2015.09.027.
- Erni-Cassola G, Gibson MI, Thompson RC, Christie-Oleza JA. 2017. Lost, but found with Nile Red: A novel method for detecting and quantifying small microplastics (1 mm to 20 μm) in Environmental Samples. Environmental Science & Technology 51(23):13641–13648. DOI: 10.1021/acs.est.7b04512.
- Faure F, Saini C, Potter G, Galgani F, de Alencastro LF, Hagmann P. 2015. An evaluation of surface micro- and mesoplastic pollution in pelagic ecosystems of the Western Mediterranean Sea. Environmental Science and Pollution Research 22(16):12190–12197. DOI: 10.1007/s11356-015-4453-3.
- Ficociello G, Gerardi V, Uccelletti D, Setini A. 2021. Molecular and cellular responses to short exposure to bisphenols A, F, and S and eluates of microplastics in C. elegans. Environmental Science and Pollution Research 28(1):805–818. DOI: 10.1007/s11356-020-10498-5.
- Fossi MC, Peda C, Compa M, Tsangaris C, Alomar C, Claro F, Baini M. 2018. Bioindicators for monitoring marine litter ingestion and its impacts on Mediterranean biodiversity. Environmental Pollution 237:1023–1040. DOI: 10.1016/j.envpol.2017.11.019.
- Griffiths CL, Stenton-Dozey JME, Koop K. 1983. Kelp wrack and the flow of energy through a sandy beach ecosystem. In: McLachlan A, Erasmus T, editors. Sandy beaches as ecosystems. The Hague: Junk Publications. pp. 547–556.
- Haegerbaeumer A, Mueller M, Fueser H, Traunspurger W. 2019. Impacts of micro- and nano-sized plastic particles on benthic invertebrates: A literature review and gap analysis. 7. DOI: 10.3389/fenvs.2019.00017.
- Iannilli V, Corami F, Grasso P, Lecce F, Buttinelli M, Setini A. 2020. Plastic abundance and seasonal variation on the shorelines of three volcanic lakes in Central Italy: Can amphipods help detect contamination? Environmental Science and Pollution Research 27(13):14711–14722. DOI: 10.1007/s11356-020-07954-7.
- Iannilli V, Di Gennaro A, Lecce F, Sighicelli M, Falconieri M, Pietrelli L, Battisti C. 2018. Microplastics in Talitrus saltator (Crustacea, Amphipoda): New evidence of ingestion from natural contexts. Environmental Science and Pollution Research 25(28):28725–28729. DOI: 10.1007/s11356-018-2932-z.
- Iannilli V, Pasquali V, Setini A, Corami F. 2019. First evidence of microplastics ingestion in benthic amphipods from Svalbard. Environmental Research 179:108811. DOI: 10.1016/j.envres.2019.108811.
- Imhof HK, Ivleva NP, Schmid J, Niessner R, Laforsch C. 2013. Contamination of beach sediments of a subalpine lake with microplastic particles. Current Biology 23(19):R867–R868. DOI: 10.1016/j.cub.2013.09.001.
- Imhof HK, Laforsch C, Wiesheu AC, Schmid J, Anger PM, Niessner R et al. 2016. Pigments and plastic in limnetic ecosystems: A qualitative and quantitative study on microparticles of different size classes. Water Research 98:64–74. DOI: 10.1016/j.watres.2016.03.015.
- Imhof HK, Wiesheu AC, Anger PM, Niessner R, Ivleva NP, Laforsch C. 2018. Variation in plastic abundance at different lake beach zones - a case study. Science of Total Environment 613:530–537. DOI: 10.1016/j.scitotenv.2017.08.300.
- Jamieson AJ, Brooks LSR, Reid WDK, Piertney SB, Narayanaswamy BE, Linley TD. 2019. Microplastics and synthetic particles ingested by deep-sea amphipods in six of the deepest marine ecosystems on Earth. Royal Society Open Science 6(2):180667. DOI: 10.1098/rsos.180667.
- Kallenbach EMF, Rødland ES, Buenaventura NT, Hurley R. 2022. Microplastics in terrestrial and freshwater environments. In: Bank MS, editor. Microplastic in the environment: Pattern and process. environmental contamination remediation and management. Cham: Springer. pp. 87–130. DOI: 10.1007/978-3-030-78627-4_4.
- Koutnik VS, Leonard J, Alkidim S, DePrima FJ, Ravi S, Hoek EMV, Mohanty SK. 2021. Distribution of microplastics in soil and freshwater environments: Global analysis and framework for transport modeling. Environmental Pollution 274:116552. DOI: 10.1016/j.envpol.2021.116552.
- Lambert S, Wagner M. 2018. Microplastics are contaminants of emerging concern in freshwater environments: An overview. In: Wagner M, Lambert S, editors. Freshwater microplastics. Emerging environmental contaminants? Cham: Springer. Vol. 58, pp.1–23.
- Li J, Liu H, Paul Chen J. 2018. Microplastics in freshwater systems: A review on occurrence, environmental effects, and methods for microplastics detection. Water Research 137:362–374. DOI: 10.1016/j.watres.2017.12.056.
- Lin C-T, Chiu M-C, Kuo M-H. 2021. 123AD. Effects of anthropogenic activities on microplastics in deposit-feeders (Diptera: Chironomidae) in an urban river of Taiwan. Scientific Reports 11:400. DOI: 10.1038/s41598-020-79881-z.
- Lopez-Soriano J, Quiñonero-Salgado S, Cappelletti C, Faccenda F, Ciutti F. 2018. Unraveling the complexity of Corbicula clams invasion in Lake Garda (Italy). Advances in Oceanography and Limnology 9(2). DOI: 10.4081/aiol.2018.7857.
- Lovato T, and Pecenik G 2012. 19 - Three-dimensional modeling of pollutant dispersion in Lake Garda (North Italy). In: Ferenc J, and Sven Erik J (editors), Developments in Environ- mental Modelling. Elsevier. Vol. 25, pp. 319–330. DOI: 10.1016/B978-0-444-59396-2.00019-5.
- Meyers N, Catarino AI, Declercq AM, Brenan A, Devriese L, Vandegehuchte M, De Witte B, Janssen C, Everaert G. 2022. Microplastic detection and identification by Nile red staining: Towards a semi-automated, cost- and time-effective technique. Science of the Total Environment 823:153441. DOI: 10.1016/j.scitotenv.2022.153441.
- Nel HA, Dalu T, Wasserman RJ. 2018. Sinks and sources: Assessing microplastic abundance in river sediment and deposit feeders in an austral temperate urban river system. Science of Total Environment 612:950–956, PlascticEurope-Association of Plastics Manufactures, 2020. Plastics – the Facts 2020. PlasticEurope 1–64. DOI: 10.1016/j.scitotenv.2017.08.298.
- Plastics Europe 2021. Plastics – the Facts 2021: An analysis of European plastics production, demand and waste data. https://plasticseurope.org/wp-content/uploads/2021/12/Plastics-the-Facts-2021-web-final.pdf
- Prinz N, Korez Š. 2020. Understanding how microplastics affect marine biota on the cellular level is important for assessing ecosystem function: A review. In: Jungblut S, Liebich V, Bode-Dalby M, editors. YOUMARES 9 - The Oceans: Our research, our future. Cham: Springer. pp. 101–120. DOI: 10.1007/978-3-030-20389-4_6.
- Redondo-Hasselerharm PE, De Ruijter VN, Mintenig SM, Verschoor A, Koelmans AA. 2018a. Ingestion and chronic effects of car tire tread particles on freshwater benthic macroinvertebrates. Environmental Science & Technology 52(23):13986–13994. DOI: 10.1021/acs.est.8b05035.
- Ruffo S, Tarocco M, Latella L. 2014. Cryptorchestia garbinii n. sp. (Amphipoda: Talitridae) from Lake Garda (Northern Italy), previously referred to as Orchestia cavimana Heller, 1865, and notes on the distribution of the two species. Italian Journal of Zoology 81(1):91–98. DOI: 10.1080/11250003.2014.891662.
- Schwarzer M, Brehm J, Vollmer M, Jasinski J, Xu C, Zainuddin S, Fröhlich T, Schott M, Greiner A, Scheibel T, Laforsch C. 2022. Shape, size, and polymer dependent effects of microplastics on Daphnia magna. Journal of Hazardous Materials 426. DOI: 10.1016/j.jhazmat.2021.128136.
- Shahnawaz M, Sangale MK, Ade AB. 2019. Case studies and recent update of plastic waste degradation. In: Bioremediation technology for plastic waste. Singapore: Springer Nature. pp. 31–43. DOI: 10.1007/978-981-13-7492-0_4.
- Shim WJ, Song YK, Hong SH, Jang M. 2016. Identification and quantification of microplastics using Nile Red staining. Marine Pollution Bulletin 113(1–2):469–476. DOI: 10.1016/j.marpolbul.2016.10.049.
- Sighicelli M, Pietrelli L, Lecce F, Iannilli V, Falconieri M, Coscia L, Di Vito S, Nuglio S, Zampetti G. 2018. Microplastic pollution in the surface waters of Italian Subalpine Lakes. Environmental Pollution 236:645–651. DOI: 10.1016/j.envpol.2018.02.008.
- Silva CJ, Patrício Silva AL, Campos D, Soares AM, Pestana JL, Gravato C. 2021. Lumbriculus variegatus (Oligochaeta) exposed to polyethylene microplastics: Biochemical, physiological and reproductive responses. Ecotoxicology and Environmental Safety 207. DOI: 10.1016/j.ecoenv.2020.111375.
- Talbot R, Chang H. 2022. Microplastics in freshwater: A global review of factors affecting spatial and temporal variations. Environmental Pollution 292:118393. DOI: 10.1016/j.envpol.2021.118393.
- Turri E. 1978. Il Lago di Garda, Novara, Istituto geografico De Agostini, pp. 8–10.
- Urbanek AK, Rymowicz W, Mirończuk AM. 2018. Degradation of plastics and plastic-degrading bacteria in cold marine habitats. Applied Microbiology and Biotechnology 102(18):7669–7678. DOI: 10.1007/s00253-018-9195-y.
- Van Cauwenberghe L, Vanreusel A, Mees J, Janssen CR. 2013. Microplastic pollution in deep-sea sediments. Environmental Pollution 182:495–499. DOI: 10.1016/j.envpol.2013.08.013.
- Windsor FM, Tilley RM, Tyler CR, Ormerod SJ. 2019. Microplastic ingestion by riverine macroinvertebrates. Science of the Total Environment 646:68–74. DOI: 10.1016/j.scitotenv.2018.07.271.
- Zbyszewski M, Corcoran PL, Hockin A. 2014. Comparison of the distribution and degradation of plastic debris along shorelines of the Great Lakes, North America. Journal of Great Lakes Research 40(2):288–299. DOI: 10.1016/j.jglr.2014.02.012.