Abstract
The invasive tiger mosquito Aedes albopictus is a serious nuisance for people due to its biting and potential for disease transmission, even in Italian mountain regions. In this work, we examined its occurrence dynamic from the first invasion in two Alpine cities, Rovereto and Trento, only 25 km apart. Mosquito’s distribution was assessed using ovitraps, from mid-May to end-October, from its first reported occurrence, i.e. 2001 in Rovereto and 2010 in Trento. In both cities, ovitraps were located in seven habitat types: gathering places, residential areas, urban parks, car parks, near watercourses, industrial areas and croplands. The annual average temperature and precipitation were similar in the two cities from 2001 to 2020. We had hypothesized that the seasonal and annual differences in egg number between both cities would be limited. Significant linear and positive relationship was found between the average number of eggs and air temperature in both the cities, but with a steeper slope in Trento. In addition, the average number of eggs per ovitrap was higher in Trento than in Rovereto (average 50.3 vs 14.2). None of the considered risk factors (climate, habitat type) explained such difference in abundance between cities. Indeed, based on the temperature, the expectation was to find fewer eggs in Trento being cooler than Rovereto during 2010–2020 (the average in the period of activated traps was, respectively, 19.2 vs 19.7°C). Thus, we argue that other anthropogenic factors, such as different control activities (adulticide treatments were carried out solely in Rovereto), might explain the difference in egg abundance found between cities. A specific experimental design to evaluate treatment effect might validate our hypothesis (e.g. apply adulticides during the surveillance period, with treatments applied at regular distances from the ovitraps and with a specific periodicity to compare adult and egg abundance).
Highlights
Ae. albopictus invaded two alpine cities 25 km apart with a lag of 12 yrs
Temporal trend of egg abundance was positively correlated with temperature in both cities
Ae. albopictus was more abundant in gathering places in urban areas
The colder city (Trento) was the more infested
Control activities carried out in Rovereto might explain difference in abundance between the two cities
1. Introduction
Understanding the dynamics of an invasive alien species is crucial in invasion biology. Aedes (Stegomyia) albopictus (Skuse, 1897) (Diptera: Culicidae), commonly known as the “Asian tiger mosquito”, is listed as one of the top 100 invasive species by the Invasive Species Specialist Group (http://www.iucngisd.org/gisd/species.php?sc=109, accessed on June 2022) and its involvement in disease transmission makes its surveillance and control of paramount importance. It is a highly invasive mosquito originally indigenous to South-East Asia, islands of the Western Pacific and Indian Ocean. In the last decades, Ae. albopictus has expanded its range to Africa, Europe, and the Americas via human activities and active transportation (Gratz Citation2004). The successful invasion of Ae. albopictus is due to a number of factors including its wide range of breeding sites, its feeding behaviour (Ae. albopictus can feed on a wide range of hosts), its competitiveness, its long-range transport through globalization, and lack of surveillance and control activities immediately following its introduction (Paupy et al. Citation2009).
Ae. albopictus is currently considered a serious biting nuisance for humans in several European countries, such as Italy (Scholte & Schaffner Citation2007; Genchi et al. Citation2009), southern France (Vazeille et al. Citation2008) and Spain, where it significantly reduces the quality of life of residents in infested areas (Aranda et al. Citation2006). Additionally, the tiger mosquito is a competent vector of at least 22 arboviruses (Gratz Citation2004) including Chikungunya, Dengue and Zika (Knudsen et al. Citation1996; Aranda et al. Citation2006; Fontenille et al. Citation2007). In Italy, two outbreaks of Chikungunya occurred in Ravenna in 2007 (Angelini et al. Citation2007; Rezza et al. Citation2007) and in the Lazio region in 2017 (Venturi et al. Citation2017), autochthonous Dengue cases were reported in Vicenza Province during 2020 (Lazzarini et al. Citation2020; Barzon et al. Citation2021) and autochthonous transmission of Zika in Florence in 2014 (Venturi et al. Citation2016).
This explains the considerable attention devoted to this species by the scientific community. Indeed, about 6000 scientific papers are indexed by Google Scholar with “Aedes albopictus” in the title, keywords or text; output of 02 August 2022. In Europe, this mosquito species was first detected in Albania in 1979, but it was suspected to be present from 1976 (Adhami & Murati Citation1987). Although Ae. albopictus became established in Albania, there were no reports in any other European countries until 1990, when it was found in Italy. Since 1990s, it has rapidly expanded its range across several countries, it is currently distributed over the Mediterranean regions and it is now spreading northward (https://www.ecdc.europa.eu/en/disease-vectors/facts/mosquito-factsheets/aedes-albopictus).
Asian tiger mosquito in Italy occurred in 1990 in the harbour of Genoa (Region of Liguria). The mosquito was likely introduced on a ship carrying tyres from the United States (Sabatini et al. Citation1990). This was the second official record of Ae. albopictus in Europe.
After only a couple of years, numerous foci of introduction were discovered in the province of Padua (Region of Veneto). Then, it invaded all Italian regions during the subsequent years (Valerio et al. Citation2010). Today, Ae. albopictus is established over most of Italy in urban and suburban areas below 600 m above sea level, with the exception of particularly dry areas in the South (Romi Citation2001; Fontenille et al. Citation2007; Romi et al. Citation2008). Italy is now one of the most heavily infested countries in Europe, with the highest abundance reported in Veneto and Friuli-Venezia-Giulia, large parts of Lombardy and Emilia-Romagna, and coastal areas of central Italy (https://www.ecdc.europa.eu/en/publications-data/aedes-albopictus-current-known-distribution-march-2021; accession date June 2022). In the Trentino Province, a mountain region located in the Rhaetian Alps, Ae. albopictus was detected for the first time in 1996 in the city of Rovereto (Ferrarese Citation2004), in a used tyre depot (as frequently occurs, Paupy et al. Citation2009). Since 2003, it has rapidly spread to the municipalities of Arco and Riva del Garda (Ferrarese Citation2005).
In 2008, it was first reported in Trento, the provincial capital, about 25 km away from Rovereto. Ae. albopictus has rapidly established itself over nearby hill regions through passive transportation of adults (car, trucks and trains). Today, there are stable populations in Valsugana, Valle dei Laghi and throughout the Adige Valley, up to 700 m a.s.l. (Roiz et al. Citation2011; Lencioni et al. Citation2018). This rapid spread to cold mountainous regions is related to the capacity of Ae. albopictus to lay cold-tolerant diapausing eggs allowing its survival in winter in temperate climates (Hanson & Craig Citation1995). Winters are becoming milder because of global climate change (Delatte et al. Citation2009; Roiz et al. Citation2011; Caminade et al. Citation2012; Reinhold et al. Citation2018).
Temperature is the key environmental factor affecting Ae. albopictus survival, distribution, abundance and seasonal range (Alto & Juliano Citation2001; Brugueras et al. Citation2020). Higher temperatures tend to accelerate larval development, increasing (i) the number of adults, (ii) the autumnal development of immatures and (iii) consequently the rates of egg overwintering (Medlock et al. Citation2006). Various temperature thresholds have been used to simulate the establishment of this species in new geographic locations. An average January temperature >0°C permits diapausing eggs to overwinter (Knudsen et al. Citation1996; Medlock et al. Citation2006) Average annual temperatures >11°C are required for adult survival and activity (Kobayashi et al. Citation2002). A maximum summer temperature of 25‒30°C is required for optimum development (Medlock et al. Citation2015). However, there are reports of established populations in areas with lower average temperatures (5‒28°C) (Severini et al. Citation2008) and diapausing eggs are able to survive cold spells of −10°C (Thomas et al. Citation2012). There is very limited adult activity below 9°C, but adults do seek warmer microclimates indoors, entering private houses, and feeding during the night (while generally they bite at dawn and dusk), so continuing their activity throughout winter (Romi et al. Citation2008).
Apart from temperature as the limiting factor, low precipitation, especially in summer, may limit the availability of breeding places, as the species requires small aquatic habitats as breeding sites (Knudsen et al. Citation1996; Eritja et al. Citation2005; Valdez et al. Citation2017). At least 500 mm of annual rainfall is required for the maintenance of aquatic habitats suitable for Ae. albopictus establishment, or more than of 60 days of precipitation/year (Eritja et al. Citation2005). However, there are records for areas with lower rainfall (290 mm annually) (Benedict et al. Citation2007; Severini et al. Citation2008). The impact of other climatic factors such as air humidity and winds has not been studied in great detail to date (Brugueras et al. Citation2020). Here, we compare the distribution and abundance of Ae. albopictus in two neighbouring cities in the Trentino Province: Rovereto and Trento. In both the cities, active surveillance has been conducted using oviposition traps (ovitraps) with a standardized method. Eggs were collected weekly from May to October 2001 and 2010, respectively.
This study aims to (1) analyse temporal trends in egg abundance in Trento and Rovereto during the last two decades and (2) investigate the relationship between ovitrap data with climatic factors such as air temperature and precipitation with egg abundance to interpret any differences between the two cities. Considering the vicinity of the two cities (only 25 km apart), and the similar climate conditions, we hypothesized limited difference in seasonal and annual occurrence trends of Ae. albopictus.
2. Materials and methods
2.1. Study area
Trento (194 m a.s.l.) and Rovereto (204 m a.s.l.) are the two main cities of the Autonomous Province of Trento (Trentino, north-eastern Italy), in the Rhaetian Alps (). Both cities are surrounded by mountains, forests, agricultural (mostly vineyards and apple orchards) and industrial areas and are crossed by the River Adige, Italy’s second longest river (410 km). Trento is the capital city of the Province, and with 118,879 inhabitants is the most densely populated city of Trentino, followed by Rovereto (39,954 inhabitants) (ISTAT data http://dati.istat.it/Index.aspx?QueryId=18552&lang=en#, accessed on 20 December 2021). Rovereto is located 25 km south of Trento (). According to the Köppen climate classification, Trento has a humid sub-tropical-continental climate (Cfa-Dfa), while Rovereto has a warm temperate climate (Cfb), due to the vicinity to the Garda Lake, which is the largest lake in Italy. Considering the period 2001–2020, the coldest month was January in both cities, with a monthly average temperature of 1.7°C in Trento and 1.8°C in Rovereto, and the warmest was July, with a monthly average temperature of 22.9°C in Trento and 23.7°C in Rovereto (, Table S1).
Table I. Air temperature (°C) and total rainfall (mm) in Trento and Rovereto in 2001–2009 and 2010–2020. “Summer” refers to the period from 21 June to 21 September; “active traps” to the period from May to October.
Figure 1. Study area. White circles = ovitrap sites in Trento and Rovereto (Trentino Province, north-eastern Italy). Trento: 46°04′N, 11°07′E; 194 m a.s.l.; Rovereto: 45°53′00″N, 11°02′03″E; 204 m a.s.l.
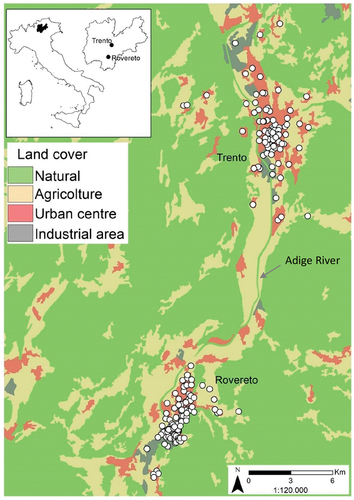
On average, the annual temperature was comparable in the two cities (12.5°C in Trento and 12.6°C in Rovereto). However, if we consider the two decades separately, temperatures increased more rapidly in Rovereto than in Trento from 2001 to 2009 to 2010–2020 (+1°C in Rovereto and +0.6°C in Trento, see ). During 2010–2020, when the mosquito was present in both cities, the temperature was always higher in Rovereto than in Trento, i.e. +0.4°C in January and +0.7°C during summer (). Total annual rainfall was comparable in both cities during the two decades, exceeding 1000 mm between 2010 and 2020 ().
In both cities, total rainfall slightly increased from the first to the second decade (+9% in Trento and +14% in Rovereto).
Climate data were downloaded from the Meteotrentino website (http://www.meteotrentino.it, accessed on 25 March 2022), as daily average air temperature and daily total precipitation. Data on Trento referred to the Trento “Laste” meteorological station (46°04ʹ18.7'' N, 11°08ʹ08.4'' E), and to “Rovereto” meteorological station (45°53ʹ46.8'' N 11°02ʹ38.4'' E) for Rovereto.
2.2. Monitoring protocol
Abundance of Ae. albopictus has been monitored with ovitraps in Rovereto and Trento since 2001 and 2010, respectively, from mid-May to end-October.
The ovitrap is a small black plastic container (height = 12 cm, diameter = 8 cm) with a hole 1 cm from the edge to prevent overfilling, mimicking the preferred natural and artificial breeding sites for the species, i.e., tree-holes, rock-holes and small man-made containers. The container is filled for two-thirds with water and contains a wood or masonite rough paddle (width = 3 cm; thickness = 0.3 cm; length = 13 cm) for females to lay eggs for adult females to lay eggs on. Mosquito presence and abundance is indirectly estimated by counting eggs laid on the paddle. In ovitraps, a larvicide based on Diflubenzuron® 2% (TARVIS Xzapi Expert© or DEVICE® TB-2) was added to the water to prevent the pots from acting as additional breeding sites for the mosquito. Paddles were removed and replaced weekly; the eggs were then collected and observed under the stereomicroscope (Leica MS5) at a magnification of 50x for identification and egg counting. The morphological identification of eggs was based on the observation of the ornamentation of the exocorion (Schaffner et al. Citation2014: ; Bova et al. Citation2016: ).
Figure 2. (a) Boxplot of the average number of eggs in the two cities, period from May to September, 2010 to 2020. The number of eggs is significantly different because the two boxes do not overlap. Box represents the first interquartile range, the line is the median, the whiskers are the third interquartile range. (b) Trends of the average number of eggs in Rovereto (from 2001 to 2020) and Trento (2010–2020).
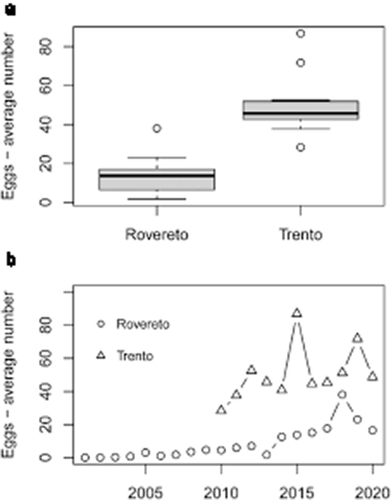
Ovitraps were deployed according to the known breeding site preferences and host presence of Ae. albopictus, considering that land use/land cover, type of urbanization and human population density affect both its distribution and density (Richards et al. Citation2006). Natural habitats consist in phytotelms (water bodies held by terrestrial plants, e.g. tree holes) and rock pools (Delatte et al. Citation2009), but Ae. albopictus has the ability to breed in natural and artificial habitats, some of which include tyres, barrels, rainwater gulley, catch basins and watering troughs (Hawley Citation1988). Monitoring sites were classified according to the presence of anthropogenic or natural factors. Ovitraps were placed in seven habitat types: (1) gathering places such as schools, hospitals, community centres, residential home for the elderly; (2) residential areas, with homes, apartments, or any place where people live (including private gardens); (3) urban parks, located in gardens, green areas and public parks; (4) car parks, located in a public parking area; (5) watercourses, located in the immediate vicinity of a watercourse; (6) industrial areas; (7) croplands, including vegetable gardens and crop fields.
In Trento, the monitoring was performed in the urban, suburban and non-urban areas, between the localities of Mattarello and Gardolo. Overall, the monitored area had an extension of 42.3 km2, at an altitude ranging between 200 and 300 m a.s.l., with a resident population of 82,895 inhabitants. The ovitraps were placed at 84 sites (listed in Table S2), distributed over eight districts: Gardolo, Ravina-Romagnano, Argentario, Mattarello, Villazzano, Oltrefersina, S. Giuseppe- S. Chiara, Historical Centre-Piedicastello. The ovitraps were placed, over 2010–2020, in urban area (64), 17 in suburban and 3 in non-urban areas. More specifically, 30 traps were placed in gathering places, 22 in urban parks, 10 in car parks, 9 close to watercourses and 6 in residential areas, 5 in industrial areas and 2 in croplands (, Table S2). In Rovereto, monitoring was carried out in urban, suburban and non-urban areas, including nine districts: Borgo Sacco, Lizzana, Lizzanella, Marco, Moietto, Mori stazione, Noriglio, San Giorgio and Sant’Ilario. This whole area had an extension of 51 Km2, an average altitude of 204 m a.s.l., and a total population of 40,332 inhabitants. Overall, from 2000 to 2020 ovitraps were placed in 109 sites, of which 66 in the urban area, 27 in suburban and 16 in non-urban areas. Specifically, 31 were places in residential area, 27 in gathering places, 19 in industrial areas, 14 in car parks, 9 in croplands, 8 in urban parks, 1 close to watercourse (, Table S2).
Table II. Number of activated ovitraps across the years classified according to the features of the neighbouring areas.
The number of ovitraps varied in the two cities across the years. The number of ovitraps ranged between 30 and 53 in Trento (average 41), and from 41 to 80 in Rovereto (average 75) (Table S3). The number of cumulative weeks with active ovitraps was on average 888 in Trento and 1777 in Rovereto considering the period 2010–2020. This implied that the average number of weeks each year was 21.7 in Trento (= 888/41 traps) and 23.7 (= 1777/75 traps) in Rovereto.
Since 1997 in Rovereto and 2010 in Trento, the municipal area has been treated with larvicide by private companies commissioned by the municipal administration, at a frequency of two to 4 weeks from May to October for a total of six to ten treatments/year. Overall, from 1,000 to 3,000 manholes and drains in public areas were treated each year. Larvicide products based on Diflubenzuron® 2% were used until 2016, they were then replaced by the microbiological larvicide (VectoMax® FG) which is based on Bacillus thuringiensis var. israelensis and Bacillus sphaericus in granular form since 2017, in both cities. In addition, adulticide treatments have only been carried out in Rovereto with pyrethroid insecticides, by spraying the vegetation up to a maximum height of 3–4 m in the absence of people, pets and wind (https://www.comune.rovereto.tn.it/Entra-in-Comune/Servizi-erogati/Cerca-per-argomento/Ambiente-animali-e-rifiuti/Zanzara-Tigre/(view)/6309, accessed on June 2022). Adulticide treatments were carried out intensively in the first 10 years, with a total of 10 complete cycles of treatments/year, In the last decade, adulticide treatments have been used only in the weeks of greatest nuisance.
2.3. Data analysis
The data were quality controlled to remove inaccurate records due to ovitrap overturns and missing ovitrap data (partial or total). This was possible because the original dataset contained detailed notes about any issue related to the ovitraps.
To test the statistical significant difference between the annual average number of eggs in Trento and Rovereto, we used the Student’s t-test for paired samples.
A non-parametric test, the Kruskal–Wallis rank sum test, was used to compare egg abundance in the different locations of ovitraps. A Wilcox post hoc test with Bonferroni correction for pairwise comparisons was then used if the overall test was significant. Non-parametric methods were preferred as the sample sizes was different between groups. The relationship between the number of eggs and the temperature was studied using linear and non-linear equations (polynomial regression), testing the fit improvement with the Fisher F-test against the reduced model. In order to calculate the delay between the summer temperature peak and the peak of eggs, we used a hierarchical set of models known as Huisman-Olff-Fresco (HOF) models (Huisman et al. Citation1993). Model comparison was performed using information criteria and a bootstrap approach (Jansen & Oksanen Citation2013). Because the skewed curve (model V) was by far the most selected by the automatic procedure, we decided to apply that model for all the years both in Trento and Rovereto. In order to test the correlations between temperature and eggs, and between rainfall and eggs, we used Pearson correlation. Cross-correlation was used to test if the effect of rainfall was delayed with respect to the egg number. The tested lags were 1, 2, 3, 4 weeks, and we used the ccf function available in R. All numerical analyses were conducted using the R software version 4.0.3 (R Core Team Citation2020) with the packages tidyverse, visreg and eHOF.
3. Results and discussion
We hypothesized limited difference in seasonal and annual occurrence trends of Ae. albopictus.
Conversely to our hypothesis, we observed a significant difference in the average annual egg abundance in the two cities (Paired t-test = 7.50, df = 10, P < 0.0001 ()) despite their geographical proximity and similarity concerning the environmental factors (). The difference in egg abundance was tested considering the period 2010–2020 (): in Trento the average was 50.3 eggs/trap, whereas in Rovereto, it was 8.5. To explain this difference, we explored the relationships between egg abundance, habitats and climate variables (air temperature and precipitation).
3.1. Habitat types surrounding the ovitraps and egg abundance
The features of the neighbouring areas where ovitraps were placed affected the average number of eggs counted in both cities (). The Kruskal–Wallis test performed among the seven groups of ovitraps was significant in Rovereto (chi-squared = 13.8, P = 0.017) and nearly so in Trento (chi-squared = 11.2, P = 0.083). This result precluded the post hoc comparison for Trento traps. In Rovereto, several locations like car parks and gathering places had a larger number of eggs than industrial areas (car park vs industrial park, pairwise Wilcox test and Bonferroni correction, P = 0.006; gathering place vs industrial park, P = 0.013). This is not surprising given that Ae albopictus preferentially feeds on humans and consequently tends to be more abundant in places where people gather (Paupy et al. Citation2009).
Figure 3. Egg abundance in the different habitat categories where ovitraps were placed in Rovereto (a) and Trento (b). Box represents the first interquartile range, the line is the median, the whiskers are the third interquartile range. * = p < 0.01, ** = p < 0.001.
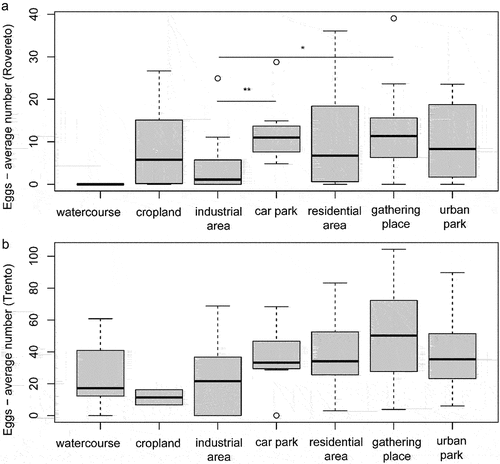
In Trento, a higher abundance of eggs was also evident in croplands, as expected, since conversion of natural habitats to agricultural land increases mosquito larval habitats, increasing the abundance of Ae. albopictus (McClure et al. Citation2018). Anyway, differences were not significant, probably because several traps belonged to mixed categories (e.g. croplands close to urban parks), and because the available data were unbalanced among categories ().
3.2. Climate variables and egg occurrence
The relationship between number of eggs (averages among ovitraps) and temperature was linear and positive in both the cities () but with different slopes. In Trento, the slope was steeper than in Rovereto (4.82 vs 1.14, ), indicating a more rapid proliferation. This result cannot be explained in terms of temperature, because in the period of activated ovitraps (2010–2020) Trento was even slightly cooler than Rovereto (respectively, 19.2 vs 19.7°C, ). Several field studies on Ae. albopictus in Japan, the United States, and Italy have shown a positive correlation between average weekly/seasonal temperature and temporal pattern of abundance estimable by the weekly increment in female abundance or number of deposited eggs or egg hatching rate (Toma & Miyagi Citation1990; Toma et al. Citation2003; Richards et al. Citation2006; Higa et al. Citation2007; Roiz et al. Citation2010). We found that the female mosquitoes start laying eggs when the temperature is 12.5–13.5°C (). This is in agreement with Roiz et al. (Citation2010) who stated that the temperature threshold necessary to initiate activity in adult female is 13°C. However, we noted that in several cases few eggs were also present at lower temperature, indicating that after years of invasion, Ae. albopictus can successfully adapt to colder conditions than previously thought. This capability to develop at a lower temperature, increases both the length of the breeding season and potentially the areas suitable to colonise at higher altitude.
Table III. Results of the linear model between number of eggs and temperature in the two cities. SE = standard error, df = degrees of freedom.
Figure 5. Average yearly temperatures (daily averages in the period of activated traps) in the two cities.
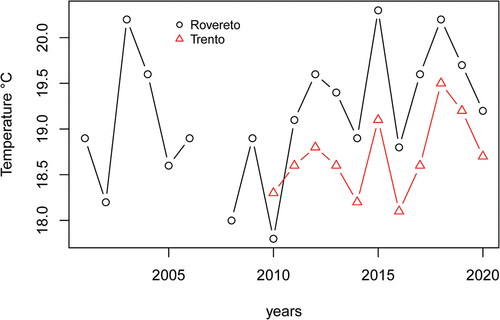
Figure 6. Number of eggs (median across ovitraps) and temperature measured in the two cities. The female adults start laying eggs when temperature is 12.5–13.5°C, with few cases when it is colder.
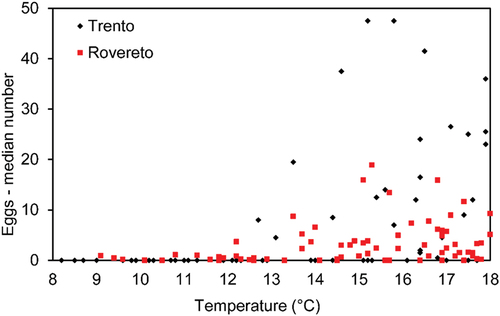
Generally, the peak in the number of eggs occurred on average 4.3–4.4 weeks after the peak of temperature, both in Trento and Rovereto (, ; Figure S1). The lag between eggs and temperature was different among years and even between cities in the same year, but the long-term averages were similar. The delay of 4.3–4.4 weeks is comparable with estimates (CitationRoiz et al. Citation2010) for host-seeking female abundance: it was positively affected by the accumulated temperatures over the period 3 and 4 weeks before the sampling week, possibly as a consequence of the positive effect of accumulated temperatures on larval density. Rainfall was not a limiting factor in both the cities as the average yearly rainfall was about 1000 mm from 2010 to 2020 (, Table S1) (Eritja et al. Citation2005). Non-significant correlation was found between the number of eggs and rainfall both in Trento (R = −0.104, P = 0.101) and Rovereto (R = −0.010, P = 0.916). We also considered a time-lagged cross-correlation between the increase of eggs and precipitation to test for possible delay. As shown in , the average number of eggs responded negatively to the precipitation with a delay of 1 week in Trento. In Rovereto, the relationship was similar but not significant. A negative correlation between precipitation and mosquito abundance, probably causing egg washout processes, was observed also by other authors (Roiz et al. Citation2010; Cunze et al. Citation2016). Rainfall needs to be sufficient during summer months to maintain egg-hatching sites (Medlock et al. Citation2015), but several authors (Roiz et al. Citation2010; Cunze et al. Citation2016) reported that periods of high precipitation reduce short-term abundance of mosquito females. Specifically, Roiz et al. (Citation2010) highlighted that accumulated precipitation over 1–4 weeks before sampling is negatively correlated with host-seeking female abundance.
Table IV. Week numbers when the peak of temperature and eggs occurred in the years. For example, in 2010 the peak of temperature occurred at the 27th week whereas that of eggs at 35th week; the lag between the peak of temperature and eggs was 8.1 weeks.
Figure 7. Visualisation of the delay between temperature peak and eggs peak in a typical year (2019, Trento). Data for all the years and in both cities are presented in . Relationship between week number and (a) average daily temperature and (b) average number of eggs among 2019’s ovitraps. Hierarchical logistic regression model using a skewed response curve (model V of the HOF models).
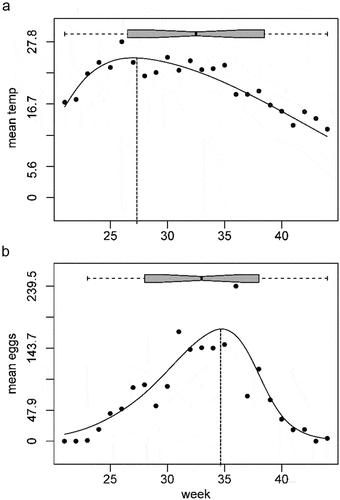
4. Conclusions
The differences in egg abundance between the two cities could not be explained either by temperature or by precipitation or habitat type of ovitrap location. Therefore, we argue that anthropogenic factors such as different control activities including occasional adulticide treatments performed only in Rovereto might explain the difference in egg abundance between Trento and Rovereto. Adulticides have only been used in Rovereto from the mosquito first invasion in the urbanized area, intensively in the first decade and more sporadically in the second one. This practice may have contributed to keeping the mosquito populations down since the beginning of its occurrence, as highlighted by other authors who experimentally demonstrated the detrimental effect of pyrethroids on mosquito abundance (e.g., Farajollahi et al. Citation2012; Baldacchino et al. Citation2015; Marini et al. Citation2015; Pichler et al. Citation2021). Data for Trento look very similar to datasets referred to other Italian regions infested by Ae. albopictus (e.g. Emilia Romagna: https://www.zanzaratigreonline.it/it). Egg abundance data is far lower for Rovereto, which strengthens the argument about vector control playing an important role in that city. However, we cannot statistically evaluate the possible effect of larvicide/adulticide treatments or good practices adopted by citizens due to the lack of quantitative data. The two municipalities did not adopt a specific experimental design during the surveillance period, being treatments applied at different distances from the ovitraps and without a specific periodicity. This hindered our ability to understand the impact of control activities on egg abundance even though we offered indirect evidence. These results encourage sound experimental studies to evaluate both larvicide and adulticide effects, as an integral part of the surveillance activity to improve the risk assessment of mosquito-borne diseases. For example, applying larvicide/adulticide at a defined distance from the traps and with a specific periodicity, makes it possible to assess the effect of the treatment (e.g. Milam et al. Citation2000; Holcomb et al. Citation2021).
Another intriguing considering is related to the presence of potential animal hosts other than humans in both cities. Specifically, Ae albopictus in the wild will mainly feed on rodents (Goodman et al. Citation2018). Mice and rats colonize areas in the proximity of the Adige River crossing the two cities, and periodically they infest some urban and suburban districts, among others the Albere district in Trento, including the cemetery, frequently infested by Rattus norvegicus (Berkenhout). We cannot demonstrate that the higher abundance of Ae. albopictus in Trento is due to a higher abundance of rodents, but we pose an important question about the role of rodents in supporting a mosquito population more consistent in Trento than in Rovereto.
Author contributions
Valeria Lencioni: conceptualization, supervision, project administration, funding acquisition, methodology, writing-reviewing and editing of the manuscript. Federica Bertola: investigation, data curation. Alessandra Franceschini: investigation, data curation. Uberto Ferrarese: investigation, data curation, scientific consultancy. Fabiana Zandonai: investigation, data computing, reviewing of the manuscript. Gionata Stancher: data computing, reviewing of the manuscript, supervision (for Rovereto dataset). Daniel Spitale: data computing, statistical analysis, writing-reviewing and editing of the manuscript.
Institutional review board statement
The study did not require ethical approval.
Acknowledgements
The Authors thank the Municipality of Trento – Environmental Service (Tiziana Friz, Luisella Codolo, Lino Nardelli, Lorenza Forti), the Municipality of Rovereto – Environment Office (Pino Bertolini, Christian Roverato, Emiliano Boschetti, Ezio Bernardinelli), the Provincial Agency for Sanitary Services (APSS, Franco Guizzardi) and the Provincial Service for employment support and environmental enhancement (SOVA, Innocenzo Coppola), for their collaboration in setting up the monitoring plan and the prevention activities. We also thank Franco Finotti, director of the Rovereto Museum Foundation from 1986 to 2017, and all technicians and researchers who contributed to the field work and egg counting: Elisa Riservato, Luca Toldo, Valentina Lai, Sonia Endrizzi, Davide Frizzera, Alfredo Maule, Daniele Debiasi and Francesca Paoli (for her support in drawing ), Alessandra Rosà, Daniela Bona, Osvaldo Maffei, Giovanni D’Alessandro, Alen Angeli. Thanks are also due to Claudia Beretta for having re-read the manuscript critically and to Janet Kilkenny for the English language revision.
Disclosure statement
No potential conflict of interest was reported by the authors.
Data availability statement
The data presented in this study (if not included in the Supplementary Material) are available on request from the corresponding author. The data of egg abundance are of jointed property with funders.
Additional information
Funding
References
- Adhami J, Murati N. 1987. The presence of the mosquito Aedes albopictus in Albania. Revista Mjekesore 1:13–16.
- Alto BW, Juliano SA. 2001. Precipitation and temperature effects on populations of Aedes albopictus (Diptera: Culicidae): Implications for range expansion. Journal of Medical Entomology 38(5):646–656. DOI: 10.1603/0022-2585-38.5.646.
- Angelini R, Finarelli AC, Angelini P, Po C, Petropulacos K, Macini P, Fiorentini C, Fortuna C, Venturi G, Romi R, et al. 2007. An outbreak of chikungunya fever in the province of Ravenna, Italy. Eurosurveillance 12(36): 3260. DOI:10.2807/esw.12.36.03260-en.
- Aranda C, Eritja R, Roiz D. 2006. First record and establishment of the mosquito Aedes albopictus in Spain. Medical and Veterinary Entomology 20(1):150–152. DOI: 10.1111/j.1365-2915.2006.00605.x.
- Baldacchino F, Caputo B, Chandre F, Drago A, Della Torre A, Montarsi F, Rizzoli A. 2015. Control methods against invasive Aedes mosquitoes in Europe: A review. Pest Management Science 71:1471–1485. DOI: 10.1002/ps.4044.
- Barzon L, Gobbi F, Capelli G, Montarsi F, Martini S, Riccetti S, et al. 2021. Autochthonous dengue outbreak in Italy 2020: Clinical, virological and entomological findings. Journal of Travel Medicine 28:taab130. DOI: 10.1093/jtm/taab130.
- Benedict MQ, Levine RS, Hawley WA, Lounibos LP. 2007. Spread of the tiger: Global risk of invasion by the mosquito Aedes albopictus. Vector-Borne and Zoonotic Diseases 7(1):76–85. DOI: 10.1089/vbz.2006.0562.
- Bova J, Paulson S, Paulson G. 2016. Morphological differentiation of the eggs of North American container-inhabiting Aedes mosquitoes. Journal of the American Mosquito Control Association 32(3):244–246. DOI: 10.2987/15-6535.1.
- Brugueras S, Fernández-Martínez B, Martínez-de la Puente J, Figuerola J, Montalvo Porro T, Rius C, Larrauri A, Gómez-Barroso D. 2020. Environmental drivers, climate change and emergent diseases transmitted by mosquitoes and their vectors in Southern Europe: A systematic review. Environmental Research 191:110038. DOI: 10.1016/j.envres.2020.110038.
- Caminade C, Medlock JM, Ducheyne E, McIntyre KM, Leach S, Baylis M, Morse AP. 2012. Suitability of European climate for the Asian tiger mosquito Aedes albopictus: Recent trends and future scenarios. Journal of the Royal Society Interface 9:2708–2717. DOI: 10.1098/rsif.2012.0138.
- Cunze S, Kochmann J, Koch LK, Klimpel S. 2016. Aedes albopictus and its environmental limits in Europe. PLoS One 11(9):e0162116. DOI: 10.1371/journal.pone.0162116.
- Delatte H, Gimonneau G, Triboire A, Fontenille D. 2009. Influence of temperature on immature development, survival, longevity, fecundity, and gonotrophic cycles of Aedes albopictus, vector of chikungunya and dengue in the Indian Ocean. Journal of Medical Entomology 46:33–41. DOI: 10.1603/033.046.0105.
- Eritja R, Escosa R, Lucientes J, Marques E, Roiz D, et al. 2005. Worldwide invasion of vector mosquitoes: Present European distribution and challenges for Spain. Biological Invasions 7:87–97. DOI: 10.1007/s10530-004-9637-6.
- Farajollahi A, Healy SP, Unlu I, Gaugler R, Fonseca DM. 2012. Effectiveness of ultra-low volume night time applications of an adulticide against Diurnal Aedes albopictus, a critical vector of Dengue and Chikungunya viruses. PLoS One 7(11):e49181. DOI: 10.1371/journal.pone.0049181.
- Ferrarese U. 2004. Monitoraggio di Aedes albopictus (Skuse) (Diptera, Culicidae) attorno a un focolaio nel comune di Rove-reto (Trento). Annali del Museo Civico di Rovereto 19:281–285.
- Ferrarese U. 2005. Nuovi dati sulla diffusione di Aedes albopictus (Skuse) (Diptera, Culicidae) nel comune di Rovereto (Trento). Annali del Museo Civico di Rovereto 20:349–356.
- Fontenille D, Failloux AB, Romi R. 2007. Should we expect chikungunya and dengue in Southern Europe? In: Takken W, Knols BG, editors. Emerging pests and Vector-Borne diseases in Europe. Nageningen, The Netherlands: Wageningen Academic Publishers. pp. 169–184.
- Genchi C, Rinaldi L, Mortarino M, Genchi M, Cringoli G. 2009. Climate and Dirofilaria infection in Europe. Veterinary Parasitology 163(4):286–292. DOI: 10.1016/j.vetpar.2009.03.026.
- Goodman H, Egizi A, Fonseca DM, Leisnham PT, LaDeau SL. 2018. Primary blood-hosts of mosquitoes are influenced by social and ecological conditions in a complex urban landscape. Parasites & Vectors 11(1):1–10. DOI: 10.1186/s13071-018-2779-7.
- Gratz NG. 2004. Critical review of the vector status of Aedes albopictus. Medical and Veterinary Entomology 18(3):215–227. DOI: 10.1111/j.0269-283X.2004.00513.x.
- Hanson S, Craig G. 1995. Relationship between cold hardiness and supercooling point in Aedes albopictus eggs. Journal of the American Mosquito Control Association 11:35–38.
- Hawley W. 1988. The biology of Aedes albopictus. Journal of the American Mosquito Control Association 1:1–39.
- Higa Y, Toma T, Araki Y, Onodera I, et al. 2007. Seasonal changes in oviposition activity, hatching and embryonation rates of eggs of Aedes albopictus (Diptera: Culicidae) on three islands of the Ryukyu Archipelago, Japan. Medical Entomology and Zoology 58:1–10. DOI: 10.7601/mez.58.1_1.
- Holcomb KM, Reiner RC, Barker CM. 2021. Spatio-temporal impacts of aerial adulticide applications on populations of West Nile virus vector mosquitoes. Parasites & Vectors 14(1):1–15. DOI: 10.1186/s13071-021-04616-6.
- Huisman J, Olff H, Fresco LFM. 1993. A hierarchical set of models for species response analysis. Journal of Vegetation Science 4(1):37–46. DOI: 10.2307/3235732.
- Jansen F, Oksanen J. 2013. How to model species responses along ecological gradients–Huisman–Olff–Fresco models re-visited. Journal of Vegetation Science 24(6):1108–1117. DOI: 10.1111/jvs.12050.
- Knudsen AB, Romi R, Majori G. 1996. Occurrence and spread in Italy of Aedes albopictus, with implications for its introduction into other parts of Europe. Journal of the American Mosquito Control Association 12:177–183.
- Kobayashi M, Nihei N, Kurihara T. 2002. Analysis of Northern distribution of Aedes albopictus (Diptera: Culicidae) in Japan by geographical information system. Journal of Medical Entomology 39:4–11. DOI: 10.1603/0022-2585-39.1.4.
- Lazzarini L, Barzon L, Foglia F, Manfrin V, Pacenti M, Pavan G, Rassu M, Montarsi F, Martini S, Zanella F, et al. 2020. First autochthonous dengue outbreak in Italy, August 2020. Eurosurveillance 1:8–11.
- Lencioni V, Franceschini A, Paoli F. 2018. Monitoraggio della zanzara tigre Aedes albopictus (Skuse) nel Comune di Trento: Analisi dei dati mediante l’Indice di Intensità di Uova. Studi Trentini di Scienze Naturali 96:143–153.
- Marini L, Baseggio A, Drago A, Martini S, Manella P, Romi R, Mazzon L. 2015. Efficacy of two common methods of application of residual insecticide for controlling the Asian tiger mosquito, Aedes albopictus (Skuse), in urban areas. PLoS One 10(8):e0134831. DOI: 10.1371/journal.pone.0134831.
- McClure KM, Lawrence C, Kilpatrick AM. 2018. Land use and larval habitat increase Aedes albopictus (Diptera: Culicidae) and Culex quinquefasciatus (Diptera: Culicidae) abundance in lowland Hawaii. Journal of Medical Entomology 55(6):1509–1516. DOI: 10.1093/jme/tjy117.
- Medlock JM, Avenell D, Barrass I, Leach S. 2006. Analysis of the potential for survival and seasonal activity of Aedes al-bopictus (Diptera: Culicidae) in the United Kingdom. Journal of Vector Ecology 31:292–304. DOI: 10.3376/1081-1710(2006)31[292:AOTPFS]2.0.CO;2.
- Medlock JM, Hansford KM, Versteirt V, Cull B, Kampen H, Fontenille D, et al. 2015. An entomological review of invasive mosquitoes in Europe. Bulletin of Entomological Research 105(6):637–663. DOI: 10.1017/S0007485315000103.
- Milam CD, Farris JL, Wilhide JD. 2000. Evaluating mosquito control pesticides for effect on target and nontarget organisms. Archives of Environmental Contamination and Toxicology 39(3):324–328. DOI: 10.1007/s002440010111.
- Paupy C, Delatte H, Bagny L, Corbel V, Fontenille D. 2009. Aedes albopictus, an arbovirus vector: From the darkness to the light. Microbes and Infection 11(14–15):1177–1185. DOI: 10.1016/j.micinf.2009.05.005.
- Pichler V, Mancini E, Micocci M, Calzetta M, Arnoldi D, Rizzoli A, et al. 2021. A novel Allele specific polymerase chain reaction (AS-PCR) assay to detect the V1016G knockdown resistance mutation confirms its widespread presence in Aedes albopictus populations from Italy. Insects 12:79. DOI: 10.3390/insects12010079.
- R Core Team. 2020. R: A language and environment for statistical computing. Vienna, Austria: R Foundation for Statistical Computing. Available: https://www.R-project.org/.
- Reinhold JM, Lazzari CR, Lahondère C. 2018. Effects of the environmental temperature on Aedes aegypti and Aedes albopictus mosquitoes: A review. Insects 9(4):158. DOI: 10.3390/insects9040158.
- Rezza G, Nicoletti L, Angelini R, Romi R, Finarelli A, Panning M, et al. 2007. Infection with chikungunya virus in Italy: An outbreak in a temperate region. Lancet 370:1840–1846. DOI: 10.1016/S0140-6736(07)61779-6.
- Richards SL, Apperson CS, Ghosh SK, Cheshire HM, Zeichner BC. 2006. Spatial analysis of Aedes albopictus (Diptera: Culicidae) oviposition in suburban neighbourhoods of a piedmont community in North Carolina. Journal of Medical Entomology 43:976–989. DOI: 10.1093/jmedent/43.5.976.
- Roiz D, Neteler M, Castellani C, Arnoldi D, Rizzoli A. 2011. Climatic factors driving invasion of the tiger mosquito (Aedes albopictus) into new areas of Trentino, Northern Italy. PLoS One 6(4):e14800. DOI: 10.1371/journal.pone.0014800.
- Roiz D, Rosa R, Arnoldi D, Rizzoli A. 2010. Effects of temperature and rainfall on the activity and dynamics of host-seeking Aedes albopictus females in Northern Italy. Vector-Borne and Zoonotic Diseases 10:811–816. DOI: 10.1089/vbz.2009.0098.
- Romi R. 2001. Aedes albopictus in Italia: un problema sanitario sottovalutato. Annali dell’Istituto Superiore Di Sanita 37(2):241–247.
- Romi R, Toma L, Severini F, Di Luca M. 2008. Twenty years of the presence of Aedes albopictus in Italy – From the annoying pest mosquito to the real disease vector. European Infectious Diseases 2:98–101.
- Sabatini A, Raineri V, Trovato G, Coluzzi M. 1990. Aedes albopictus in Italia e possibile diffusione delle specie nell’area mediterranea. Parassitologia 32:301–304.
- Schaffner F, Kaufmann C, Pflüger V, Mathis A. 2014. Rapid protein profiling facilitates surveillance of invasive mosquito species. Parasites & Vectors 7(1):1–7. DOI: 10.1186/1756-3305-7-142.
- Scholte EJ, Schaffner F. 2007. Waiting for the tiger: Establishment and spread of the Aedes albopictus mosquito in Europe. In: Takken W, Knols BGJ, editors. Emerging pests and vector-borne diseases in Europe. Vol. 1. Wageningen, The Netherlands: Wageningen Academic Publishers. pp. 241–260.
- Severini F, Di Luca M, Toma L, Romi R. 2008. Aedes albopictus in Rome: Results and perspectives after 10 years of moni-toring. Parassitologia 50(1–2):121–123.
- Thomas SM, Obermayr U, Fischer D, Kreyling J, Beierkuhnlein C. 2012. Low-temperature threshold for egg survival of a post-diapause and non-diapause European aedine strain, Aedes albopictus (Diptera: Culicidae). Parasites & Vectors 5:100. DOI: 10.1186/1756-3305-5-100.
- Toma T, Miyagi I. 1990. Seasonal changes in the hatchability of Aedes albopictus (Diptera: Culicidae) eggs in Okinawajima, Ryukyu Archipelago, Japan. Medical Entomology and Zoology 41:195–204. DOI: 10.7601/mez.41.195.
- Toma L, Severini F, Di Luca M, Bella A, et al. 2003. Seasonal patterns of oviposition and egg hatching rate of Aedes al-bopictus in Rome. Journal of the American Mosquito Control Association 19:19–22.
- Valdez LD, Sibona GJ, Diaz LA, Contigiani MS, Condat CA. 2017. Effects of rainfall on Culex mosquito population dynamics. Journal of Theoretical Biology 421:28–38. DOI: 10.1016/j.jtbi.2017.03.024.
- Valerio L, Marini F, Bongiorno G, Facchinelli L, Pombi M, Caputo B, et al. 2010. Host-feeding patterns of Aedes albopictus (Diptera: Culicidae) in urban and rural contexts within Rome province, Italy. Vector-Borne and Zoonotic Diseases 10(3):291–294. DOI: 10.1089/vbz.2009.0007.
- Vazeille M, Jeannin C, Martin E, Schaffner F, Failloux AB. 2008. Chikungunya: A risk for Mediterranean countries? Acta Tropica 105(2):200–202. DOI: 10.1016/j.actatropica.2007.09.009.
- Venturi G, Di Luca M, Fortuna C, Remoli ME, Riccardo F, Severini F, Toma L, Del Manso M, Benedetti E, Caporali MG, et al. 2017. Detection of a chikungunya outbreak in Central Italy, August to September 2017. Eurosurveillance 22:39. DOI: 10.2807/1560-7917.ES.2017.22.39.17-00646.
- Venturi G, Zammarchi L, Fortuna C, Remoli ME, Benedetti E, Fiorentini C, Trotta M, Rizzo C, Mantella A, Rezza G, Bartoloni A. 2016. An autochthonous case of Zika due to possible sexual transmission, Florence, Italy, 2014. Eurosurveillance 21(8):30148.