Abstract
This research was carried out in north-eastern Poland, where Whooper Swan Cygnus cygnus is a sparse breeder. Whooper Swan is timid and exhibits a tendency to hide in emergent vegetation. A drone was used to improve the effectiveness of research into its breeding success and offspring productivity. The breeding density of Whooper Swan in the study area in 2022 was 10 pairs/100 km2. The number of breeding birds detected at the beginning of the breeding season did not differ according to the method used (drone vs ground). The breeding productivity of the sample of swans studied (N = 36) was 2.19 cygnets per breeding pair using the ground method, but 3.71 per pair with the drone, a significant difference (p-value of the Wilcoxon test = 0.0148). The traditional method showed that 50% of the pairs had bred successfully, whereas with the drone this figure was 79%. The birds either did not react to the drone’s presence or else moved slowly away. The drone study of Whooper Swan breeding productivity was considerably faster (9 min per site vs 1–2 h ground survey), more precise, and less invasive for the birds than a traditional ground survey.
Introduction
Whooper Swan Cygnus cygnus is a monotypic species that breeds in the Palearctic, from Iceland, across the boreal and subarctic part of Eurasia, to as far east as Kamchatka (Carboneras & Kirwan Citation2020). During the past 40 years it has been expanding into new regions in central Europe (Sikora et al. Citation2012; Lehikoinen et al. Citation2020). Because of climate change, most species in the Northern Hemisphere are shifting their ranges northwards, so in fact Whooper Swan is an example of the opposite process, though not an isolated one (Marchowski et al. Citation2022). The number of Whooper Swans in Poland in 2021 was estimated at 290–330 breeding pairs, with around 30% of this population in the north-eastern part of the country (Sikora & Wieloch Citation2022)), and the highest local density (up to 10 pairs/100 km2) near the border with the Kaliningrad District of Russia. It is interesting that although this is a northern species faced by global warming, one would expect these birds to withdraw northwards (Valiela & Bowen Citation2003; McDonald et al. Citation2012). This opens up new opportunities for research on this species and its breeding interactions with a competing sympatric species – Mute Swan Cygnus olor (Bałdyga et al. Citation2003; Kampe-Persson & Boiko Citation2011; Butkauskas et al. Citation2012; Sikora et al. Citation2012). Whooper Swan has become a regular breeder in north-eastern Poland (Wieloch et al. Citation2014), which is why this region was selected for the present study. There, Whooper Swan inhabits mainly small water bodies (Sikora et al. Citation2012; Chylarecki et al. Citation2015), often those formed by the activities of European beaver Castor fiber, as is the case in Latvia and Lithuania (Boiko et al. Citation2014). Since these sites are usually covered with dense vegetation, access to them on foot is difficult. The methods for studying Whooper Swan productivity require two checks during the breeding season (Chylarecki et al. Citation2015). However, avifaunistic studies using drones for other species prompted us to conduct comparative studies of the standard method (Chylarecki et al. Citation2015) with the method using the drone.
In recent years, the study of nature using unmanned aerial vehicles, i.e. drones, has been developing rapidly (Barnas et al. Citation2020). Previous studies have involved counting birds using drones during both the breeding season (Chabot et al. Citation2015; Ratcliffe et al. Citation2015; Corregidor-Castro et al. Citation2021) and the non-breeding period (Hodgson et al. Citation2018; Jarrett et al. Citation2020). A few authors have described the use of drones for more detailed studies of aspects like breeding success (Junda et al. Citation2015; Weissensteiner et al. Citation2015; Sardà-Palomera et al. Citation2017; Valle & Scarton Citation2021). To date, however, there are no reports of drones being used to investigate the breeding productivity of swans.
The aim of this research was to assess the breeding density and to compare the traditional ground survey with a drone survey with respect to their effectiveness, their invasiveness regarding birds and the time required to carry out the study of Whooper Swan breeding productivity.
Methods
Study site and field method
The study site is in north-eastern Poland, near the border with the Kaliningrad District of Russia (). This lowland area in the Górowo Heights mesoregion has a varied, undulating landscape (max. elevation 216 m a.s.l.). The network of watercourses is diverse, but the density of lakes is relatively low. This is compensated by the numerous European beaver ponds. The landscape, little changed by humans, consists predominantly of forests, and extensively farmed agricultural land. There is only one small town and the human population density in these rural areas is just a dozen or so people per km2, 67% of the study area is situated in the Ostoja Warmińska Natura 2000 Special Protected Area.
The planned study consisted of three basic elements: 1) assessment of breeding density, 2) assessment of young productivity and 3) comparison of methods for assessing young productivity using the standard ground method and the drone method.
Assessment of breeding density took place within the designated core study area (402 km2, – bordered in red), where effort was made to detect all breeding pairs present. The other two elements of studies 2 and 3 were conducted in the study area (~1700 km2 – bordered in green). Whooper Swan was searched for in its breeding habitats, the particular focus being on water bodies from a few to several dozen hectares in area. All the breeding sites known from previous years were inspected (Monitoring of Birds of Poland – Chief Inspectorate of Environmental Protection, http://monitoringptakow.gios.gov.pl/PM-GIS/), and other potential breeding locations were investigated using both ground and drone surveys (N = 38). The 11 breeding pairs of Whooper Swan from the core area were not included in the drone study and were inspected only from the ground, either because drone flights could not be carried out in the state border zone, or the birds were detected outside the indicated inspection periods. The main study was done in 2022, the preliminary one in 2021. Some of the data collected in 2021 were used to analyse the birds’ responses to the drone – see below for details in the drone study section of Methods.
Ground study
Ground inspections covered an entire water body or a group of these. Observations were made by walking around the water body and stopping at vantage points from which the area could be scanned with a spotting scope and/or binoculars. The first ground survey was carried out between 11 and 30 April 2022, the second one between 23 July and 2 August 2022; both took place during the periods recommended for monitoring breeding populations of this species in Poland (Chylarecki et al. Citation2015). During the breeding season, Whooper Swan is secretive and very alert; moreover, despite its large size and contrasting plumage, it is easily overlooked (). In spring, when the vegetation was still sparse, ground observations were not difficult, and the adult birds were readily detectable. During the second visit in summer, however, the luxuriant plant growth on the banks of the water bodies and the emergent vegetation all but prevented adults with cygnets from being spotted, even from higher vantage points.
Figure 2. A Whooper Swan Cygnus cygnus adult with six cygnets at one of the study sites within the study area. 2 August 2022. Photo by Dominik Marchowski.
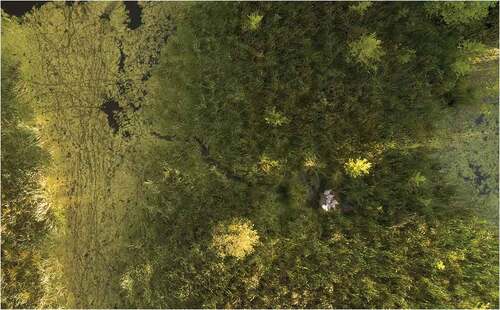
The first visit to a site involved ascertaining that the birds were indeed present there and assigning the observations to a specific breeding category (Bibby et al. Citation2000). The second visit involved evaluating the breeding success, if such was the case, and counting the number of cygnets (brood productivity). The breeding categories are as used in the European Breeding Bird Atlas (BBC2 – Keller et al. Citation2020). The pairs found during the second inspections were taken into consideration for calculating the reproduction parameters.
Drone study
During both the first (April) and second (July-August) inspection, each site was also inspected using a drone independently by a second observer (N = 38).
A DJI Phantom drone (version 4 Pro V2.0) was used for the fieldwork. This is a remotely controlled quadcopter device with a total weight of 1,388 grams, equipped with a camera capable of taking both still photographs (max quality: 5,472 x 3,648 pixels) and videos (max quality: 4,096 x 2,160 pixels), with the option of continuous tracking of unrecorded images transmitted to the display coupled with the remote control. Each photo records the geographic location and altitude in the metadata.
A distance usually of several hundred metres but never less than 100 m separated the observer from the surveyed sites. The drone flight height was set at about 60 m, the same as recommended for bird counts from aircraft (Laursen et al. Citation2008). From this height, the study site was scanned for birds. If a group of birds or a single individual were spotted on the remote-control display, a photo was taken, and the birds were approached in order to identify the species (Whooper vs Mute Swan). Down to a height of 30 m, the birds were approached diagonally. If on reaching 30 m, the birds were still not responding to the drone, and the species was still not known, the drone was moved over the birds to identify the species. The distance was shortened but the height maintained, after which the drone was lowered, usually to about 10–20 m. If the birds could not be seen on the tablet display, the area was scanned from a height of 30–45 m and photos taken to cover the entire site. The birds’ reactions to the drone were classified as follows: 1 – no reaction, 2 – slowly moving away, 3 – panic escape. The duration of each drone mission was measured, and the bird detection time was recorded on the drone control screen. The response of birds to the drone was examined during the second inspection in August – 13 drone missions in 2021 and 38 in 2022.
Comparison of methods
During the April visit, the presence or absence of birds at each site was compared. Then, the number of birds observed, and the breeding categories determined from two types of inspections were compared. During the second visit (July-August), the number of young birds (cygnets) detected by drone and by ground observation was determined at the 38 sites.
Paired Samples Wilcoxon Tests in the R environment (R Development Core Team Citation2021) were carried out. During the first inspection at the beginning of the breeding season (April), the first set of data concerned the number of birds that were detected by the traditional ground method, the second set of data was the number of birds detected using a drone. During the second inspection (July-August), the first set of data were the results of breeding productivity (the number of cygnets per breeding pair) obtained during the ground surveys, while the second set of data were obtained using the drone (N = 38). The result was considered statistically significant if the p-value of the Wilcoxon test was less than 0.05.
Results
Breeding density – core study area
The combined assessment of the two methods in 2022 showed 29 breeding pairs in the core area and an additional 11 pairs identified through the ground survey only (refer to ). The breeding density, which is the first study element, was calculated as follows: The total number of Whooper Swan pairs in the 402 km2 core area was 40, giving a density of 10 pairs per 100 km2.
Breeding productivity and method comparison – study area
During the first, traditional ground survey in April, swans were found on 31 out of the 38 selected potential sites (82%), whereas the drone inspection revealed 32 sites with swans. The difference was not significant ().
Table I. The number of sites occupied by Whooper Swans (Cygnus cygnus) assessed through ground observation and drone surveys in the spring (first survey) and summer (second survey) with a sample size of 38 in the survey area.
The differences between the methods were also not significant when the breeding category was assessed; the vast majority were assigned to the highest category – confirmed breeding ().
During the second ground survey (July-August) of 16 sites (41%), adults with cygnets were detected, and the total number of cygnets was 70 (average 2.19 young per pair that bred). However, during the second drone survey at 22 sites (68%), adults with cygnets were found, the total number of cygnets being 104 (average 3.71 young per pair that bred, ). The difference in the number of cygnets as revealed by the two test methods was statistically significant (p value of Wilcoxon test = 0.0148), ().
Figure 3. Productivity of Whooper Swan Cygnus cygnus assessed using the traditional ground observation and drone survey (N = 36). Dots – all data; rhombus – mean; whiskers – standard error.
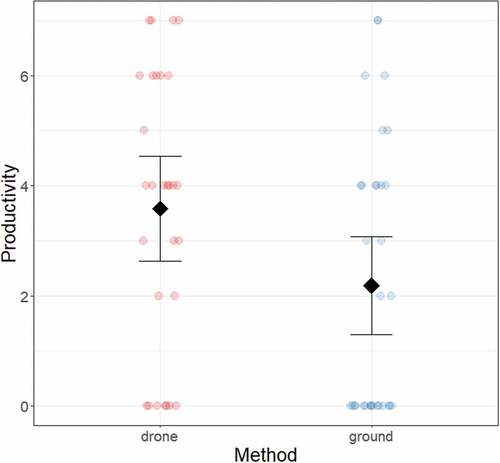
Table II. Reproduction in Whooper Swan on the Górowo Heights in 2022 as shown by the standard ground and drone methods for two surveys separately (spring, summer) and for both surveys combined.
In three cases during the second inspection in July-August, the birds detected using the traditional ground method were not discovered with the drone, whereas in 11 cases of sites where no birds were detected using the ground method, they were found using the drone. Thus, by combining all the birds detected using both methods, we obtain 24 pairs that achieved breeding success, a total of 112 cygnets and an average of 3.11 young per pair (). However, the drone and the combined survey results were not statistically different (p value of Wilcoxon test = 0.265).
Analysis of drone missions, birds’ reactions to the drone
The swans’ reactions to the drone in 52 missions in 2021 and 2022 during the second inspection period in August were as follows: 27 missions (52%) – no reaction; 24 missions (46%) – slow movement away; 1 mission (2%) – panic escape.
During the 38 drone missions of the first inspection in 2022 at 32 sites, birds were detected on the drone control screen; double-checking on the large computer screen revealed no additional birds. During the 38 drone missions of the second inspection in 2022 at 25 sites, birds were detected on the tablet screen during the mission, and analysis of photos on the large computer screen of two additional sites yielded further birds.
The average flight time of the drone during a single mission during the second survey in 2022 (summer) was 9 minutes (2–20 min-max, N = 38).
Discussion
The study area is an optimal habitat for Whooper Swan, and thus has one of the highest breeding densities in Europe (9–10 pairs/100 km2, BirdLife International Citation2015; Sikora et al. Citation2022, see Results – 10 p/100 km2).
We found that using a drone to study Whooper Swan breeding productivity was effective and yielded significantly more reliable research results. Moreover, this approach was minimally invasive, as the birds usually ignored the drone or, if they did react to it, moved slowly away from it (see Results – Analysis of drone missions). We do not know how the stress caused by the drone affects the physiology of birds (Marchowski Citation2021), but a preliminary study of anserine birds, specifically Common Eider Somateria mollissima, indicates that the physiological response to well-planned drone research is negligible (Geldart et al. Citation2022).
It’s important to note that drones are not a foolproof tool. During the summer inspection (second survey), three sites were missed using the drone compared to the traditional ground method. However, during the spring inspection (first survey), the results from ground and drone surveys were not significantly different (refer to ). To achieve the most accurate results, it’s recommended to use a combination of ground counts and drone surveys when necessary, or to conduct additional drone surveys at sites where no birds were detected during the initial visit.
The use of a drone for conducting site inspections resulted in a significant time-saving compared to the standard ground method. The average duration of a single drone mission was just nine minutes, not including the time needed to analyze the photos and prepare the drone for take-off (5–10 minutes). In comparison, ground inspections took around 1–2 hours on average and up to several hours for difficult-to-reach sites, as reported by Chylarecki et al. (Citation2015). The presence of an observer on the ground often made the swans skittish and they would fly off and hide among the vegetation, whereas the use of a drone minimizes this effect and leads to higher quality results.
To date, the use of drones for studying the breeding biology of swans has not been described in the scientific literature. However, drones have been used for research related to the conservation of swan species, such as the Black-necked Swan Cygnus melancoryphus in Chile (Jaramillo et al. Citation2018) and Mute Swan in Denmark (Clausen et al. Citation2020). Drones have also been used for more advanced studies of avian biology in birds of prey (Junda et al. Citation2015), ducks (Pöysä et al. Citation2018), Pied Avocet Recurvirostra avosetta (Scarton & Valle Citation2020), and Sandwich Tern Thalasseus sandvicensis (Valle & Scarton Citation2021).
Results indicating that drone chick counts were more accurate were also obtained by Valle and Scarton (Citation2021) for Sandwich Tern, and our research corroborates this in the case of Whooper Swan. Initial research on the breeding success of birds using drones has also been described by a few other authors (Junda et al. Citation2015; Weissensteiner et al. Citation2015; Pöysä et al. Citation2018), but the development of this field is still in its early stages.
In conclusion, the study of Whooper Swan biology using a drone is faster, more precise, and less invasive than the traditional ground survey. Both Whooper Swan and Mute Swan are large, contrastingly coloured birds, and one might think they would be plainly visible in the field. But differences in their behaviour mean that different field techniques are required for detecting them. This could lead to systematically repeated errors, causing the breeding success and productivity of Whooper Swan to be underestimated. The use of drones is especially important in the case of Whooper Swan, as it is more timid and more often nests in hard-to-reach habitats than Mute Swan, which is not very skittish and nests in more open habitats.
Drones have potential to be utilized in similar studies of other waterbird species inhabiting overgrown and inaccessible areas, and in environments with sparse low vegetation. For instance, the Greylag Goose Anser anser, which nests in dense reeds, has not yet been studied using drones for its breeding population. Initial research suggests that the drone can be a highly effective tool for studying the breeding populations of the Great Egret Ardea alba (Zbytyt, Citation2018), indicating that the device may also be beneficial for other species from this group, such as the Purple Heron Ardea purpurea or other species that nest in reeds or similar vegetation, such as the Crane Grus grus, Marsh Harrier Circus aeruginosus, and Montagu’s Harrier Circus pygargus. The drone has also proven to be an accurate and minimally invasive tool for conducting surveys of breeding colonies of gulls, terns, and other seabirds (Ratcliffe et al. Citation2015; Rush et al. Citation2018; Marchowski Citation2021).
Field experience, knowledge of the terrain and the biology of the species are essential. This is important both during traditional ground inspections and when using a drone. Less experienced researchers may be less effective at spotting birds on sites.
Data availability
The authors confirm that the data supporting the findings of this study are available at:
Marchowski et al. (Citation2022), “The use of drones to study the breeding productivity of Whooper Swan Cygnus cygnus. Dataset”, Mendeley Data, V1, DOI: 10.17632/57x4kkgmw6.1
Disclosure statement
No potential conflict of interest was reported by the author(s).
References
- Bałdyga T, Wieloch M, Czyż S 2003. Second mixed brood of Whooper Swan Cygnus cygnus and Mute Swan C. olor in Poland. Not. Orn. 44: 270–273. (Polish with English summary).
- Barnas AF, Chabot D, Hodgson AJ, David W, Johnston DW, Bird DM, Ellis-Felege SN. 2020. A standardized protocol for reporting methods when using drones for wildlife research. Journal of Unmanned Vehicle Systems 8:89–98. DOI: 10.1139/juvs-2019-0011.
- Bibby CJ, Burgess ND, Hill DA, Mustoe S. 2000. Bird census techniques. London, San Diego, New York, Boston, Sydney, Tokyo, Toronto: Academia Press.
- BirdLife International. 2015. European red list of birds. Luxembourg: Office for Official Publications of the European Communities.
- Boiko D, Kampe-Persson H, Morkunas J. 2014. Breeding Whooper Swans Cygnus cygnus in the Baltic states, 1973–2013: A result of re-colonisation. Wildfowl 64:207–216.
- Butkauskas D, Švažas S, Tubelytė V, Morkūnas J, Sruoga A, Boiko D, Paulauskas A, Stanevičius V, Baublys V. 2012. Coexistence and population genetic structure of the whooper swan Cygnus cygnus and mute swan Cygnus olor in Lithuania and Latvia. Open Life Sciences 7(5):886–894. DOI: 10.2478/s11535-012-0065-9.
- Carboneras C, Kirwan GM. 2020. Whooper Swan (Cygnus cygnus), version 1.0. In: Del Hoyo J, Elliott A, Sargatal J, Christie DA, de Juana E, editors. In birds of the world. Ithaca, NY, USA: Cornell Lab of Ornithology. DOI: 10.2173/bow.whoswa.01.
- Chabot D, Craik SR, Bird DM. 2015. Population census of a large common tern colony with a small, unmanned aircraft. PLoS ONE 10(4):e0122588. DOI: 10.1371/journal.pone.0122588.
- Chylarecki P, Sikora A, Cenian Z, Chodkiewicz T (eds.) 2015. Monitoring ptaków lęgowych. Poradnik metodyczny. [Monitoring breeding birds – a methodological handbook] Wydanie 2. GIOŚ, Warszawa.
- Clausen KK, Holm TE, Pedersen CL, Jacobsen EM, Bregnballe T. 2020. Sharing waters: The impact of recreational kayaking on moulting mute swans Cygnus olor. Journal of Ornithology 161:469–479. DOI: 10.1007/s10336-020-01746-z.
- Corregidor-Castro A, Holm TE, Bregnballe T. 2021. Counting breeding gulls with unmanned aerial vehicles: Camera quality and flying height affects precision of a semi-automatic counting method. Ornis Fennica 98:33–45.
- Geldart EA, Barnas AF, Semeniuk CAD, Gilchrist HG, Harris CM, Love OP. 2022. A colonial-nesting seabird shows no heart-rate response to drone-based population surveys. Scientific Reports 12:18804. DOI: 10.1038/s41598-022-22492-7.
- Hodgson JC, Mott R, Baylis SM, Pham TT, Wotherspoon S, Kilpatrick AD, Segaran RR, Reid I, Terauds A, Koh LP. 2018. Drones count wildlife more accurately and precisely than humans. Methods in Ecology and Evolution 9:1160–1167. DOI: 10.1111/2041-210X.12974.
- Jaramillo E, Lagos NA, Labra FA, Paredes E, Acuña E, Daniel Melnick D, Manzano M, Velásquez C, Duarte C. 2018. Recovery of black-necked swans, macrophytes and water quality in a Ramsar wetland of southern Chile: Assessing resilience following sudden anthropogenic disturbances. Science of the Total Environment 628/629:291–301. DOI: 10.1016/j.scitotenv.2018.01.333.
- Jarrett D, Calladine J, Cotton A, Wilson MW, Humphreys E 2020. Behavioural responses of non-breeding waterbirds to drone approach are associated with flock size and habitat, Bird Study, DOI: 10.1080/00063657.2020.1808587.
- Junda J, Greene E, Bird DM. 2015. Proper flight technique for using a small rotary-winged drone aircraft to safely, quickly, and accurately survey raptor nests. Journal of Unmanned Vehicle Systems 3:222–236. DOI: 10.1139/juvs-2015-0003.
- Kampe-Persson H, Boiko D. 2011. Occurrence of swan hybrids around the Baltic Sea – An outcome of range expansions? Ornis Svecica 21:45–54. DOI: 10.34080/os.v21.22609.
- Keller V, Herrando S, Voříšek P, Franch M, Kipson M, Milanesi P, Martí D, Anton M, Klvaňová A, Kalyakin MV, Bauer H-G, Foppen RPB. 2020. European breeding bird Atlas 2: Distribution, abundance and change. Barcelona: European Bird Census Council & Lynx Edicions.
- Laursen K, Frikke J, Kahlert J. 2008. Accuracy of ‘total counts’ of waterbirds from aircraft in coastal waters. Wildlife Biology 14(2):165–175. DOI: 10.2981/0909-6396(2008)14[165:AOTCOW]2.0.CO;2.
- Lehikoinen A et al. 2020. Cygnus cygnus Whooper Swan.In: Keller V, Herrando S, Vorisek P, editors. European breeding bird Atlas 2: Distribution, abundance and change Barcelona: European Bird Census Council & Lynx Edicions. pp. 102–103.
- Marchowski D. 2021. Drones, automatic counting tools, and artificial neural networks in wildlife population censusing. Ecology and Evolution 11:16214–16227. DOI: 10.1002/ece3.8302.
- Marchowski D, Mohr A, Ławicki Ł, Jankowiak Ł. 2022. Warmer winters increase the breeding success of the Goosander in the Pomeranian Lake District in Poland. Ardea 110:1–15. DOI: 10.5253/arde.v110i1.a3.
- McDonald KW, McClure CJW, Rolek BW, Hill GE. 2012. Diversity of birds in eastern North America shifts north with global warming. Ecology and Evolution 2(12):3052–3060. DOI: 10.1002/ece3.410.
- Pöysä H, Kotilainen J, Väänänen VM, Kunnasranta M. 2018. Estimating production in ducks: A comparison between ground surveys and unmanned aircraft surveys. European Journal of Wildlife Research 64:74. DOI: 10.1007/s10344-018-1238-2.
- Ratcliffe N, Guihen D, Robst J, Crofts S, Stanworth A, Enderlein P. 2015. A protocol for the aerial survey of penguin colonies using UAVs. Journal of Unmanned Vehicle Systems 3(3):95–101. DOI: 10.1139/juvs-2015-0006.
- R Development Core Team. 2021. R: A Language and Environment for Statistical Computing.” Vienna, Austria: R Foundation for Statistical Computing. http://www.r-project.org/. Accessed Jan 2023 10.
- Rush GP, Clarke LE, Stone M, Wood MJ. 2018. Can drones count gulls? Minimal disturbance and semiautomated image processing with an unmanned aerial vehicle for colony-nesting seabirds. Ecology and Evolution 8(24):12322–12334. DOI: 10.1002/ece3.4495.
- Sardà-Palomera F, Bota G, Padilla N, Brotons L, Sardà F. 2017. Unmanned aircraft systems to unravel spatial and temporal factors affecting dynamics of colony formation and nesting success in birds. Journal of Avian Biology 48:1273–1280. DOI: 10.1111/jav.01535.
- Scarton F, Valle RG. 2020. Could we assess the hatching success of Pied Avocets (Recurvirostra avosetta) by drone monitoring? A pilot study. Lavori Soc Ven Sci Nat 45:135–138.
- Sikora A, Marchowski D, Półtorak W. 2022. Use of beaver ponds by breeding Whooper Swans Cygnus cygnus and Mute Swans C. olor at Górowo Heights (north-eastern Poland). Ornis Polonica 63:199–214. (Polish with English summary).
- Sikora A, Wieloch M 2022. Whooper swan monitoring. Chief Inspectorate of Environmental Protection. http://monitoringptakow.gios.gov.pl/PM-GIS/.
- Sikora A, Wieloch M, Chylarecki P. 2012. Breeding population of the Whooper Swan Cygnus cygnus in Poland. Ornis Polonica 53:69–85. (Polish with English summary).
- Valiela I, Bowen JB. 2003. Shifts in winter distribution in birds: Effects of global warming and local habitat change. AMBIO: A Journal of the Human Environment 32(7):476–480. DOI: 10.1579/0044-7447-32.7.476.
- Valle RG, Scarton F. 2021. Drone-conducted counts as a tool for the rapid assessment of productivity of Sandwich Tern (Thalasseus sandvicensis). Journal of Ornithology 126:621–628. DOI: 10.1007/s10336-020-01854-w.
- Weissensteiner MH, Poelstra JW, Wolf JBW. 2015. Low-budget ready-to-fly unmanned aerial vehicles: An effective tool for evaluating the nesting status of canopy-breeding bird species. Journal of Avian Biology 46:425–430. DOI: 10.1111/jav.00619.
- Wieloch M, Sikora A, Rohde Z, Neubauer G 2014. Expansion of breeding population of the Whooper Swan in Poland. 5th International Swan Symposium, Easton, Maryland, USA, 2-6 February 2014. Poster.
- Zbyryt A. 2018. The impact of drones on birds and their application in ornithological research and conservation. Ornis Polonica 59:56–70.