Abstract
In wildlife captive management, contraception is highly desirable due to inbreeding risk and needed control of intra- and inter-specific aggressions. Deer, for which surgical castration can cause abnormal growth of the antlers, are suitable candidates for pharmacological castration. The aim of this study was to evaluate the effect of pharmacological castration on the physiological patterns and volatile chemical profiles of two deer species. We placed a subcutaneous implant of deslorelin acetate (4.7 mg) on four male red deer (Cervus elaphus) and two male fallow deer (Dama dama) (N = 6) living together in a mixed-sex and -species group housed at a wildlife rescue centre in Tuscany, Italy. We combined hormone measurements and chemical investigation of metatarsal scent-gland odour secretions to test whether pharmacological castration will influence the volatile chemical profile, potentially modifying the olfactory communication during the rutting season. We used radioimmunoassay technique to determine faecal and plasma testosterone as well as faecal cortisol levels, and solid-phase microextraction coupled to gas chromatography-mass spectrometry to analyse the scent-gland odour secretions. We found that deer testosterone levels decreased after the subcutaneous deposition of the implant. However, castrated red deer exhibited more variability in testosterone concentrations throughout the study period compared to fallow deer, whose testosterone levels remained low after the implant. We detected a total of 124 (red deer) and 88 (fallow deer) volatile compounds in male scent-gland odour secretions, including naturally occurring ketones, alcohols, aldehydes, terpenes, carboxylic acids, esters, volatile fatty acids, and hydrocarbons. Odour richness, but not total abundance or ratios of compounds, changed with testosterone levels in red deer. In conclusion, our preliminary findings suggest that deslorelin acetate, causing a decrease of testosterone level and impacting the chemical profile of odour secretions, could work as a non-invasive contraception approach in human-managed populations of deer.
1 Introduction
The control of reproduction in ungulates is a matter of increasing interest in both free-ranging and captive settings (Barrell et al. Citation2009; Fagerstone et al. Citation2010; Massei and Cowan Citation2014). Reproductive activity is driven by sexual hormones, whose concentration can be reduced by the use of gonadotropin-releasing hormone (GnRH) agonists. Specifically, even if initially GnRH agonists bind to the GnRH receptors in the hypophysis and stimulate the production of FSH and LH, causing a subsequent increase of the concentration of sexual steroids (flare up effect), in the long term these agents cause the desensitization of GnRH receptors to GnRH and a temporary and reversible downregulation of the hormonal cascade of the hypothalamic-pituitary-gonadal (HPG) axis (Padula Citation2005; Trigg et al. Citation2006; Fagerstone et al. Citation2010; Palm et al. Citation2012; Lucas Citation2014; Massei and Cowan, Citation2014). However, there is conflicting evidence about the impact of these agents on testosterone levels, which is the main gonadal hormone driving male reproductive activity (Lincoln Citation1987; Beker and Katz Citation1997; Baker et al. Citation2004; Barrell et al. Citation2009). Testosterone usually interacts with cortisol, another steroid hormone released by the hypothalamic-pituitary-adrenal (HPA) axis in response to stressful events and energetic needs (Mormède et al. Citation2007; Bonier et al. Citation2009). The HPG and the HPA axes are strictly related and mutually modulate; hence, any alteration of the HPG affects the HPA (Viau Citation2002).
Features such as identity, rank, and age impact on the reproductive quality of mammals and may be reflected in their scent (Rasmussen et al. Citation2002; LaDue et al. Citation2021). Chemical signals are linked directly to physiological conditions and are expected to be more honest than other sexual signals (Hasson Citation1997); specifically, odours are affected by gonadal hormones during the breeding season (Petrulis Citation2013). Social odours influence both reproductive behaviour and the activation of the HPG axis in mammals (Petrulis Citation2013). For instance, scents of opposite-sex individuals can lead an animal to increase proceptive behaviours, including vocalizations, marking, exploratory, and inspection behaviours, to favour the encounter with mating partners in several mammal species (Vaglio et al. Citation2020).
Red deer (Cervus elaphus) and fallow deer (Dama dama) are traditionally managed by humans in farms or wildlife parks (Asa & Porton Citation2005). In such captive facilities, contraception is highly desirable because of space limitation, inbreeding risk, and needed control of aggressive behaviours associated with the rutting season (Asa & Porton Citation2005). In addition, testicular activity and testosterone concentration regulate the cycle of antlers, and surgical castration of adults can cause the persistence of antlers covered with velvet that can grow disorganized creating a “perruque”, which negatively impacts their welfare (Fletcher et al. Citation2016). Both species are seasonal breeders, with the rutting season occurring over autumn in the Northern Hemisphere (Lincoln Citation1985; Asher et al. Citation1993; Asher Citation2011). During this period of the year, the high testosterone levels cause physiological, morphological, and behavioural changes, including the growth of testes, production of spermatozoa, increase of the mass of neck muscles (i.e., hypertrophy), as well as display of agonistic behaviours and acoustic calls (Asher et al. Citation1993; Kierdorf et al. Citation2004; Malo et al. Citation2009; Landete-Castillejos et al. Citation2019). The rutting season has high energetic costs and may cause stress, which ultimately determines an increase in cortisol levels. However, other authors have found both low and high cortisol levels during the breeding season, and its interaction with testosterone remain uncertain (Huber et al. Citation2003; Konjević et al. Citation2011; Pavitt et al. Citation2015; de la Peña et al. Citation2021).
Cervids use multimodal communication to advertise their reproductive status (Massei & Bowyer Citation1999). Specifically, red deer use low pitched roars, whose frequency of emission changes during the breeding season (Bocci et al. Citation2013). Similarly, fallow deer employ groans during breeding seasons both in male-male competition and female choice; these vocalizations are useful for individual recognition (Vannoni & McElligott Citation2008). In addition, deer use visual cues in meaningful locations, such as forest edges or elevated sites (Massei & Bowyer Citation1999). For instance, during the breeding season fallow deer perform territorial behaviours (Massei & Bowyer Citation1999). In cervids visual and olfactory cues are often associated, and deer scent-gland odour secretions convey information about age, sex, and population identity to conspecifics. Their role in reproductive strategies is however currently understudied (Lawson et al. Citation2001; de la Peña et al. Citation2019). Previous studies do not rule out a possible important role of chemical communication during the breeding season. For example, in the urine of male red deer, short-chain carboxylic acids and aromatic compounds increase during the breeding season (Bakke & Figenschou Citation1990). Another study suggests that, during the rutting season, Iberian red deer males (Cervus elaphus hispanicus) involved in high male-male competition may communicate their dominance and conditions using volatile compounds (de la Peña et al. Citation2019).
This preliminary study examined the effects of pharmacological castration on physiological patterns and volatile chemical profiles, by combining faecal endocrinology with chemical investigation of scent-gland odour secretions, in captive pharmacologically castrated (via 4.7 mg deslorelin acetate implants), red and fallow deer. Particularly, we tested the following hypotheses and predictions:
Castration affects testosterone levels in deer. We predicted that castration would result in decreased testosterone concentration during the autumn, and no difference in its concentration throughout the rest of the year.
There is a direct relationship between testosterone and cortisol levels. We predicted that cortisol and testosterone concentrations would show a positive correlation during both breeding and non-breeding seasons.
There is a direct relationship between sex hormone levels and chemical profiles of metatarsal scent-gland odour secretions in castrated male subjects. We predicted that volatile chemical profiles would change according to testosterone levels during both breeding and non-breeding seasons.
2 Materials and methods
2.1 Ethical assessment
The study was evaluated and authorized by the Ethical Committee of the Veterinary Department at the local health authority in Tuscany (USL - Unità Sanitaria Locale of Toscana Centro, Italy).
2.2 Study subjects & anaesthesiologic protocol
The study subjects were four male red deer and two male fallow deer (N = 6) housed together with seven females (5 red deer and 2 fallow deer) at the CRAS Semia onlus rescue centre in a semi-free ranging naturalistic 1.4 ha mixed-sex and mixed-species enclosure, subdivided into two areas of 0.9 and 0.5 hectares, and including grass and olive trees. Subjects were provided with hay during the year and commercial pellet once a day, three times a week, during summer and spring time, and they had access to fresh tree branches and two drinking points. All the animals had free access to the same quality of food items and water, meaning that we can exclude any dietary impact on the chemical compounds excreted by their metatarsal scent-glands. All study subjects were identified via ear-tags for their recognition ().
Table I. Identification, age and species of the study subjects.
We carried out the study from September 2020 to August 2021. The 8th of September 2020 implants of deslorelin acetate (Suprelorin® F, Virbac, 4.7 mg) were deposited subcutaneously on the left side of the animals’ neck and the first batch of faecal and plasma samples were collected for measurement of hormonal levels. Particularly, the implant was placed at the same time for red and fallow deer, but the reproductive period (rutting season) begins at different times for the two species (i.e., from middle of September in red deer, and from the end of September in fallow deer (Varuzza Citation2019)). The second sampling day was 34 days after the implantation. We carried out a further two samplings (at the end of January 2021 and at the beginning of March 2021) to monitor the reproductive status. We suspended the sampling protocol over the summer due to the weather conditions (i.e., very high temperature). Thus, we completed the last sampling event at the end of August 2021 before the following reproductive season. We collected samples in the morning, from 9.00 am to 12.00 pm, and immediately transferred them to the University of Bologna where we stored them in a − 20°C freezer.
We darted the animals using a CO2-powered rifle (DAN-Inject, model JM Special, Børkop, Denmark) and 3 ml Telinject syringes with 2.0 × 38 mm needles. We used a solution of dry tiletamine/zolazepam (Zoletil®100, Virbac) reconstituted with 5 ml of xylazine HCL (Nerfasin® 100, le vet Beheer b.v.) (1:1:2 = tiletamine: zolazepam: xylazine) as anaesthetic combination. We utilized a dosage of 1.4–1.6 ml per dart according to the estimated weight of the single individual. The first time that we anaesthetized them, we referred to the range and mean weight reported for the same species in the bibliography. Once the animals were asleep, we weighed them with an appropriate scale. The mean body weight ±SD (standard deviation) of red and fallow deer was respectively 126.30 ± 28.97 and 80.30 ± 18.81 kg but varied during the year. Once sedated, we placed the deer in right lateral recumbency, we tied together their two hind legs and the right foreleg, and put on their face a mask to prevent the sight. We monitored anaesthesia measuring SO2, temperature, heart, and respiratory rate, and by checking mucous membrane colour and capillary refill time, palpebral, perineal, and corneal reflexes. We administered an antagonist dose of 0.13 ± 0.03 mg/kg atipamezole (Antisedan®, Orion Pharma, Espoo, Finland) intramuscularly after at least 45 minutes from the anaesthesia.
2.3 Endocrinological sampling & analysis
We used plasma and faeces to measure the level of cortisol and testosterone hormones throughout the study period. During the anaesthesia, we collected blood samples (n = 30) from the jugular vein (VACUTEST KIMA srl, 5 ml) and faeces (n = 26) from the rectum. We decided to duplicate the samples because anaesthesia and capture procedures can affect cortisol levels in blood samples (Bonier et al. Citation2009). After that, we transferred the samples at +4°C, using a freezer box with ice packs, and stored them in a −20°C freezer at the Physiology and Anatomy Service Laboratory of the Department of Veterinary Medical Sciences, University of Bologna (Italy) for laboratory analyses.
We determined cortisol and testosterone concentrations via radioimmunoassay (RIA) based on binding of 3 H-steroid by competitive adsorption (Fenske & Schonheiter Citation1991). All concentrations were expressed in pg/mg of faeces and ng/ml of plasma. With regards to faeces, we used the extraction methodology modified from Schatz and Palme (Citation2001) and Fontani et al. (Citation2014). We extracted cortisol and testosterone from 500 mg wet weight faeces with methanol-water solution (v/v 4:1) and ethyl ether. We vaporized the portion of ether under an airstream suction hood at 37°C. We dissolved the dry residue again into 0.5 ml PBS (0.05 M, pH 7.5). With regards to plasma, we performed the extraction of testosterone as described by Tamanini et al. (Citation1983).
We conducted cortisol and testosterone metabolites assay in faeces according to Tamanini et al. (Citation1983) and Gaiani et al. (Citation1984), respectively. We performed the cortisol RIA using an antiserum to cortisol-21-hemisuccinate-BSA (anti-rabbit), at a working dilution of 1:20 000 and 3 H-cortisol (30 pg/tube vial) as a tracer, and the testosterone RIA using an antiserum to testosterone-3-carboxymethyloxime-BSA (anti-rabbit), at a working dilution of 1:35000 and 3 H-testosterone (31 pg/tube vial) as a tracer. Validation parameters of analysis were sensitivity 0.19 pg/mg, intra-assay variability 5.9%, inter-assay variability 8.7%, for cortisol; sensitivity 1.1 pg/mg, intra-assay variability 6.2%, inter-assay variability 9.6%, for testosterone. We determined radioactivity using a liquid scintillation β counter and a linear standard curve, designed ad hoc by software Priamo (Motta & Degli Esposti Citation1981).
2.4 Odour sampling & analysis
We collected 24 odour samples (red deer = 16 samples; fallow deer = 8 samples) by rubbing sterile cotton swabs on the metatarsal gland, ten times using steady pressure (Setchell et al. Citation2010 Citation2011; Elwell et al. Citation2021). We placed all samples into sterile 10 ml screw-capped clear glass vials (Supelco thread: 18O.D. 22.5 mm × H 46 mm) closed by teflon-faced rubber septa and seals (1.3 mm thick) labelled with study subject identity and date. We transported the vials at +4°C and stored them at −20°C at the Physiology and Anatomy Service Laboratory of the Department of Veterinary Medical Sciences, University of Bologna (Italy), and then cold shipped them on dry ice to the Rosalind Franklin Science Centre, University of Wolverhampton (UK), for laboratory analyses.
We investigated the volatile component of metatarsal scent-gland odour secretions using established solid-phase microextraction (SPME) and gas chromatography-mass spectrometry (GC–MS). We applied the same methods used in our previous work on primate odour signals (reviewed in Walker & Vaglio Citation2021). Briefly, we introduced a 65 µm polydimethylsiloxane/divinylbenzene SPME syringe needle through the vial septum and exposed the fibre to the headspace above the sample in the vial for 15 min at 40°C. We analysed the adsorbed volatile analytes of all samples using a 5975C mass spectrometer (Agilent Technologies) EI, 70 eV, coupled directly to a 7890B gas chromatograph (Agilent Technologies) equipped with a fused silica HP5-MS UI capillary column (Agilent Technologies) 30 m × 0.25 mm cross bonded 5%-phenyl-95%-dimethylpolysiloxane, film thickness 0.25 µm. We maintained the injector and transfer line temperatures at 270°C and 280°C, respectively. We made injections in split-less mode (purge valve opened after 1 min) with a constant flow of helium carrier gas of 1 ml min−1. We started the oven temperature program at 45°C for 2 min, then we raised it by 4°C min−1 to 170°C, and finally by 20°C min−1 to 300 °C40.
We standardized peak retention times using retention time locking to alpha-pinene. We tentatively identified eluted compounds by comparing the experimental spectra with those of the mass-spectral library in ChemStation (Agilent Technologies) and NIST Database (National Institute of Standards and Technology), version MSD F.01.01.2317 (Agilent Technologies). We accepted a putative identification if the minimum matching factor was higher than 70%. To minimize the chance of misidentification and when more than one compound was a good match for the same GC peak, we considered the chromatographic retention time and compared it with those reported in the literature for the same chromatographic column type. We created a data matrix using the peak area relative to each identified compound using the integrated signal of the deconvoluted total ion current (TIC). We analysed all samples in a short period of time to minimize inter-assay variability. We overlaid chemical profiles from control filter paper on animal chemical profiles to identify compounds that did not derive from the animals and removed these from the results.
2.5 Statistical analysis
Due to the small size of both groups and the physiological individual variations of testosterone levels we assessed the variation in blood testosterone concentration following the deslorelin acetate implant focusing on individual study subjects. In order to avoid the potential influences of the acute stress caused by capture and handling procedures (Morgan & Tromborg Citation2007), we used faecal hormone concentrations for investigating testosterone and cortisol relationship.
To investigate the relationship between faecal cortisol and testosterone levels, we used the R package ”lme4” to run a Linear Mixed Model (LMM) (Bates et al. Citation2014). For this LMM analysis, cortisol was set as outcome variable, and testosterone, species, and their interaction as fixed variables. Subject ID was set as random effect. To test the effect of testosterone on the chemical profile, we calculated richness (total number of detected compounds in each sample), Shannon’s H (an index which takes into account the relative abundance of each compound within a sample) and total odour (total abundance of compounds per sample). We used the same R package to run a negative binomial Generalize Linear Mixed Model (GLMM) and two Linear Mixed Model (LMM) (Bates et al. Citation2014). Before running the analysis, we applied a logarithmic transformation to the total odour variable and Johnson transformation to Shannon’s H. In order to assess whether the relationship between odour profile and testosterone was present only for one of the two species examined, we set compound richness, Shannon’s H and total odour as outcome variables in separate models while testosterone, age, species, and the interaction of age and testosterone with species were set as fixed variables. We included subject ID as a random effect to control for multiple sampling of the same individual. We excluded one outlier for each model. Statistical significance was set at p < 0.05.
3 Results
3.1 Plasma and faecal hormone profiles
In red deer, plasma testosterone concentrations displayed a similar trend across the four study subjects, as shown in . After the implantation event (September 2021) plasma testosterone levels decreased initially (November 2021), then rose (January 2021), and finally decreased again (March 2022). The youngest individuals (CGD and CGS) showed another increase in plasma testosterone levels at the end of the study period (August 2022). In contrast, plasma testosterone levels decreased after the implantation event in fallow deer and then remained low over the entire study period in both study subjects ().
Figure 1. Plasma testosterone levels (ng/ml) of male red deer (Cervus elaphus) over the study period.
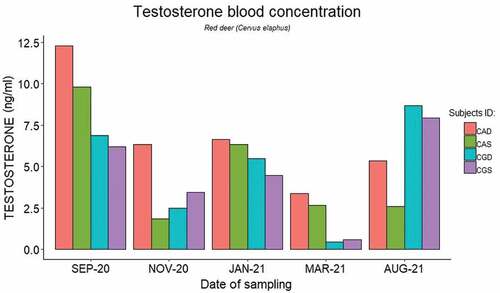
Our results showed no significant relationship between cortisol and testosterone levels in faeces in either of the study groups ().
Table II. Results of the LMM examining whether faecal cortisol concentration is significantly predicted by faecal testosterone level, species and the interaction between these two fixed factors. SE = standard error.
During the study period, one of the five female red deer gave birth (October 2021), while we did not record any pregnancy in fallow deer.
3.2 Odour profile
We detected a total of 138 (red deer) and 92 (fallow deer) volatile compounds in 16 (red deer) and 8 (fallow deer) scent-gland odour samples. These compounds included a range of naturally occurring odorous volatile compounds such as ketones, alcohols, aldehydes, terpenes, carboxylic acids, esters, volatile fatty acids and hydrocarbons. We could tentatively identify 82 (red deer) and 58 (fallow deer) compounds, with 31 compounds found in both red deer and fallow deer samples (). Further 42 (red deer) and 30 (fallow deer) compounds were classified as “unknowns”.
Table III. Volatile compounds present in swab samples from red deer (Cervus elpahus) metatarsal gland odour secretions identified tentatively using the ChemStation (Agilent Technologies) and NIST (version MSD F.01.01.2317) mass spectral databases, listed in order of retention time. Compounds in bold font were found in both red deer and fallow deer samples.
Table IV. Volatile compounds present in swab samples from fallow deer (Dama dama) metatarsal gland odour secretions identified tentatively using the ChemStation (Agilent Technologies) and NIST (version MSD F.01.01.2317) mass spectral databases, listed in order of retention time. Compounds in bold font were found in both red deer and fallow deer samples.
Our negative binomial GLMM showed that both testosterone and species significantly predicted compound richness, whereas there was no significant interaction effect between these two factors, and age did not result as a significant predictor (). More specifically, testosterone level positively predicted the richness of the volatile chemical profile (β ± SE = 0.05 ± 0.02, z = 3.28, p = .001), and fallow deer has a higher richness of the volatile chemical profile than red deer (β ± SE = 0.49 ± 0.13, z = 3.73, p < .001) (). In contrast, we did not find any impact of testosterone, species, and age or their interaction on the Shannon’s H and total odour of the chemical compounds ().
Table V. Results of the GLMMs examining whether richness of volatile chemical profile, during the pharmacological castration period (November 2020 to August 2021), is significantly predicted by plasma testosterone level, age and species and the interaction between species and the other two fixed factors. Significant results are in bold font. SE = standard error.
Table VI. Results of the LMM examining whether total odour of volatile chemical profiles, during the pharmacological castration period (November 2020 to August 2021), is significantly predicted by plasma testosterone level, species, age and the interaction between species and the other two fixed factors. SE = standard error.
Table VII. Results of the LMM examining whether Shannon’s H of volatile chemical profiles, during the pharmacological castration period (November 2020 to August 2021), is significantly predicted by plasma testosterone level, species, age and the interaction between species and the other two fixed factors. SE = standard error.
4 Discussion & conclusions
Seasonal testosterone fluctuations in red deer living in temperate regions and Mediterranean ecosystem are well known. Testosterone peaks at the end of the summer right before the rutting season in early autumn, then decreases during the winter and reaches its minimum level over the spring (Lincoln et al. Citation1972; Mulley Citation2007; Malo et al. Citation2009). In our study, plasma testosterone concentrations decreased in November during the breeding season, then rose in January, and bottomed out during the non-breeding season in March. One of the five female red deer gave birth in October, which is later than the usual physiological calving period (i.e., spring (Mulley Citation2007)). Given that in red deer the pregnancy usually lasts around 8 months (226–245 days) (Varuzza Citation2019), we can date the beginning of the pregnancy back to February. We cannot rule out that the effect of the implant on testosterone levels of red deer ended sooner than what we had expected, which could contribute to the new rise of testosterone levels in January and to the delayed beginning of the fertile period. Further research is, however, needed to explore the efficacy of GnRH agonists on the reproductive biology of female red deer.
Contrarily to red deer, after implantation fallow deer showed decreased plasma testosterone levels in November, with concentrations remaining low until the end of the study period. In fallow deer, the rutting season occurs over October and November (Mulley Citation2007), with maximum testicular activity between September and the second half of November. Our results, therefore, suggest both efficacy and duration of the implant in fallow deer.
The different influence of deslorelin acetate implants on the two study species could be due to the different timing of implantation compared to the start of the rutting season, which is slightly different in the two species (Varuzza Citation2019); the different body size and weight of the study subjects; and species-specific features. Other authors have reported differences in the efficacy and duration of GnRH agonists in different mammal species. For instance, deslorelin implants could not suppress spermatogenesis nor impact plasma testosterone in bulls (D’Occhio et al. Citation2000), but inhibited gonadotropin release in dogs (Junaidi et al. Citation2009), while in cheetahs the loss of fertility was reached using a higher dose than what is commercially available (4.7 mg) (Bertschinger et al. Citation2006).
We then examined the relationship between testosterone and cortisol profiles during the period after pharmacological castration. We did not find any significant relationship between hormone patterns, but this might be due to the small sample size of our study. The cortisol concentration can be affected by several factors (i.e., stressful events, circannual rhythm, physical activity) (Mormède et al. Citation2007; Bonier et al. Citation2009); hence regular faecal sampling throughout the year would be needed to fully understand the relationship between HPG and HPA, as well as the influence of chemical castration on cortisol levels. Prior studies have already highlighted the mutual influence between HPG and HPA (Viau Citation2002), but the mechanisms underpinning the interaction between these two endocrine systems are still unclear. Although there is a general agreement about the inhibitory effect of HPA products on HPG activity, some authors have hypothesised a positive interaction between testosterone and cortisol for some energy-expensive activities, such as mating efforts (Ketterson & Nolan Citation1999; Grebe et al. Citation2019), while the breeding period is certainly physiologically stressful due to environmental and social challenges (Mormède et al. Citation2007; Bonier et al. Citation2009).
We detected a total of 124 (red deer) and 88 (fallow deer) volatile chemical compounds in male metatarsal scent-gland odour secretions, including 31 compounds that were found in both species. Odour richness, but not total abundance or ratios of compounds, changed alongside testosterone levels. Specifically, we found two compounds in both species (i.e., propanoic acid, 2-methyl-, 3-hydroxy-2,2,4-trimethylpentyl ester); 5,9-undecadien-2-one, 6,10-dimethyl-, (E)-) and two compounds exclusive to red deer (i.e., cyclopropane, nonyl-; octane, 1,1'-oxybis-) only in samples collected over August, when testosterone levels rose again as male deer were approaching the new breeding season and the effect of deslorelin acetate implant was ended. Interestingly, these volatile compounds have already been identified in odour profiles of other animal species as playing a role in sexual communication. For instance, 5,9-undecadien-2-one, 6,10-dimethyl-, (E)- and cyclopropane, nonyl- have been suggested to act as sex pheromones in invertebrate species, such as longhorn beetles (Cerambycidae) (Meier et al. Citation2020), houseflies (Musca domestica) (Guo et al. Citation1991) and gypsy moths (Lymantria dispar) (Solari et al. Citation2007). Propanoic acid, 2-methyl-, 3-hydroxy-2,2,4-trimethylpentyl ester has been reported to have a function of urinary pheromone in mammals, including tigers (Panthera tigris) (Soso Citation2011) and the tree shrew (Tupaia belangeri) (Ye et al. Citation2018). Finally, 1–1′-oxybis octane acts as an indicator of different phases of pregnancy, and as a distinctive signal between the para-axillary and nipple-areola regions, in women (Vaglio et al. Citation2009).
Our results did not show a significant influence of age on the odour profile of the study subjects. On the contrary, other studies have highlighted the importance of age which would affect the volatile chemical profiles of the dark ventral patch of red deer which are used as sexual signals (Martín et al. Citation2014; de la Peña et al. Citation2019). In addition, in urine and interdigital gland odour secretions released by male white-tailed deer, differences have been found between chemical profiles of old/dominant versus young/subordinate individuals, likely because of their different hormonal status, especially considering testosterone levels (Gassett et al. Citation1996; Miller et al. Citation1998). The discrepancy with our results might be due to the small sample size of our study, which might not have allowed us to find significant differences in odour profiles of subjects with different ages.
Mammalian social systems rely on signals transferred between individuals conveying a variety of information, including individual and group identity (Thom & Hurst Citation2004). Particularly, an individual odour signature is made by multiple sources of chemosignals, including both non-volatile (i.e., proteins) and volatile compounds (Brennan & Kendrick Citation2006). In mammals, olfactory signals are vital in male-male competition and predator-prey interactions; these include male scent-gland odour providing reliable information to both male competitors and potential female mates (reviewed in Wyatt Citation2003), and “alert” alarm odour signals released by males when alarmed or pursued. For example, when concerned by potential predators, black-tailed deer (Odocoileus hemionus columbianus) expose the metatarsal gland on the hind leg, releasing a garlic-like odour, which causes other deer to lift their heads and scan the surroundings (Muller-Schwarze et al. Citation1984). We, therefore, argue that the deslorelin acetate implant may have suppressed the release of key compounds for sexual interactions in male red deer and thus disrupted deer socio-sexual communication.
Finally, we must acknowledge some major limitations of this preliminary study. First of all, we focused on limited data pools which included a relatively small sample size and unit of analysis. Moreover, pre-treatment sampling would be crucial, especially in light of the small sample size and the lack of control subjects. Particularly, a control set of females should be included to understand the volatile chemical compounds present and how these relate to steroid hormones. Additionally, plasma levels of deslorelin could be used to substantiate the conclusion that deslorelin wore off sooner in red deer.
In conclusion, on the basis of plasma testosterone patterns and volatile chemical profiles of metatarsal scent-gland odour secretions, our preliminary study supports the hypothesis that the deslorelin acetate implant could influence the reproductive status of male deer, but further studies are needed to understand its efficacy and duration, especially in red deer. Further work, using a larger sample size and both sexes, is needed to better understand the effects on animal health and the efficacy of GnRH agonists in cervids, focusing on the dosage and timing of the implants.
Acknowledgements
We acknowledge Enrico Loretti from USL-Toscana Centro for sampling authorization, and Tiziano De Lorentis, Andrea Zacchi and Martina Lamanna for their help with deer sampling. We thank Stefano Kaburu for his assistance with statistical analyses and Colin Dubreuil for editing. We are also grateful to the Animal Behaviour & Wildlife Conservation group (Wolverhampton) for useful comments on an earlier draft.
Disclosure statement
No potential conflict of interest was reported by the authors.
Data availability statement
The raw data supporting the conclusions of this article will be made available by the authors, without undue reservation.
Additional information
Funding
References
- Asa CS, Porton IJ. 2005. Wildlife Contraception: Issues, methods, and applications. Baltimore, Maryland: JHU Press. pp. 300.
- Asher GW. 2011. Reproductive cycles of deer. Animal Reproduction Science 124:170–175. DOI: 10.1016/j.anireprosci.2010.08.026.
- Asher GW, Fisher MW, Fennessy PF, Mackintosh CG, Jabbour HN, Morrow CJ. 1993. Oestrous synchronization, semen collection and artificial insemination of farmed red deer (Cervus elaphus) and fallow deer (Dama dama). Animal Reproduction Science 33:241–265. DOI: 10.1016/0378-4320(93)90118-B.
- Baker DL, Wild MA, Connor MM, Ravivarapu HB, Dunn RL, Nett TM. 2004. Gonadotropin-releasing hormone agonist: A new approach to reversible contraception in female deer. Journal of Wildlife Diseases 40(4):713–724. DOI: 10.7589/0090-3558-40.4.713.
- Bakke JM, Figenschou E. 1990. Volatile compounds from the red deer (Cervus elaphus). substances secreted via the urine. Comparative Biochemistry and Physiology Part A: Physiology 97:427–431. DOI: 10.1016/0300-9629(90)90635-6.
- Barrell GK, Schaafsma SCT, Ridgway MJ, Wellby M, Miller LA. 2009. Effects of a synthetic gonadotrophin-releasing hormone agonist, leuprolide, on rut-associated events in male red deer. Animal Production Science 49(12):1120. DOI: 10.1071/AN09080.
- Bates D, Maechler M, Bolker B, Walker S. 2014. lme4: Linear mixed-effects models using Eigen and S4. R Package Version 1(7):1–23.
- Beker SE, Katz LS. 1997. Contraception in wildlife management. Washington, D.C.: United States Department of Agriculture. pp. 11–21.
- Bertschinger HJ, Jago M, Nöthling JO, Human A. 2006. Repeated use of the GnRH analogue deslorelin to down-regulate reproduction in male cheetahs (Acinonyx jubatus). Theriogenology 66(6–7):1762–1767. DOI: 10.1016/j.theriogenology.2006.01.040.
- Bocci A, Telford M, Laiolo P. 2013. Determinants of the acoustic behaviour of red deer during breeding in a wild alpine population, and implications for species survey. Ethology Ecology & Evolution 25:52–69. DOI: 10.1080/03949370.2012.705331.
- Bonier F, Martin PR, Moore IT, Wingfield JC. 2009. Do baseline glucocorticoids predict fitness? Trends in Ecology & Evolution 24:634–642. DOI: 10.1016/j.tree.2009.04.013.
- Brennan PA, Kendrick KM. 2006. Mammalian social odours: Attraction and individual recognition. Philosophical Transactions of the Royal Society B: Biological Sciences 361(1476):2061–2078. DOI: 10.1098/rstb.2006.1931.
- D’Occhio MJ, Fordyce G, Whyte TR, Aspden WJ, Trigg TE. 2000. Reproductive responses of cattle to GnRH agonists. Animal Reproduction Science 60:433–442. DOI: 10.1016/S0378-4320(00)00078-6.
- de la Peña E, Barja I, Carranza J. 2021. Social environment with high intrasexual competition enhances the positive relationship between faecal testosterone and cortisol metabolite levels in red deer. Mammalian Biology 101:207–215. DOI: 10.1007/s42991-021-00100-x.
- de la Peña E, Martín J, Carranza J. 2019. The intensity of male-male competition may affect chemical scent constituents in the dark ventral patch of male Iberian red deer. Plos One 14:e0221980.
- Elwell E, Walker D, Vaglio S. 2021. Sexual dimorphism in crowned lemur scent-marking. Animals 11(7):2091. DOI: 10.3390/ani11072091.
- Fagerstone KA, Miller LA, Killian G, Yoder CA. 2010. Review of issues concerning the use of reproductive inhibitors, with particular emphasis on resolving human-wildlife conflicts in North America. Integrative Zoology 5:15–30. DOI: 10.1111/j.1749-4877.2010.00185.x.
- Fenske M, Schonheiter H. 1991. Thin-layer chromatography on silica-coated aluminium sheet as an adjunct to radioimmunoassay of steroids. Journal of Chromatography 563(1):178–183. DOI: 10.1016/0378-4347(91)80292-K.
- Fletcher J, Foster A, Goddard P, McSloy A. 2016. Managing antler problems in deer. Practice 38:513–519. DOI: 10.1136/inp.i5271.
- Fontani S, Vaglio S, Beghelli V, Mattioli M, Bacci S, Accorsi PA. 2014. Fecal concentrations of cortisol, testosterone, and progesterone in cotton-top tamarins hosted in different zoological parks: Relationship among physiological data, environmental conditions and behavioral patterns. Journal of Applied Animal Welfare Science 17:228–252. DOI: 10.1080/10888705.2014.916173.
- Gaiani R, Chiesa F, Mattioli M, Nannetti G, Galeati G. 1984. Androstenedione and testosterone concentrations in plasma and milk of the cow throughout pregnancy. Journal of Reproduction and Fertility 70:55–59. DOI: 10.1530/jrf.0.0700055.
- Gassett JW, Wiesler DP, Baker AG, Osborn DA, Miller KV, Marchinton RL, Novotny M. 1996. Volatile compounds from interdigital gland of male white-tailed deer (Odocoileus virginianus). Journal of Chemical Ecology 22(9):1689–1996. DOI: 10.1007/BF02272407.
- Grebe NM, Del Giudice M, Emery Thompson M, Nickels N, Ponzi D, Zilioli S, Maestripieri D, Gangestad SW. 2019. Testosterone, cortisol, and status-striving personality features: A review and empirical evaluation of the Dual hormone hypothesis. Hormones and Behavior 109:25–37. DOI: 10.1016/j.yhbeh.2019.01.006.
- Guo L, Latli B, Prestwich GD et al. 1991. Metabolically blocked analogs of housefly sex pheromone: II. Metabolism studies. Journal of Chemical Ecology 17(9):1769–1782. DOI: 10.1007/BF00993727.
- Hasson O. 1997. Towards a general theory of biological signalling. Journal of Theoretical Biology 185:139–156. DOI: 10.1006/jtbi.1996.0258.
- Huber S, Palme R, Arnold W. 2003. Effects of season, sex, and sample collection on concentrations of fecal cortisol metabolites in red deer (Cervus elaphus). General and Comparative Endocrinology 130:48–54. DOI: 10.1016/S0016-6480(02)00535-X.
- Junaidi A, Williamson P, Martin G, Blackberry M, Cummins J. 2009. Dose–response studies for pituitary and testicular function in male dogs treated with the GnRH superagonist, deslorelin. Reproduction in Domestic Animals 44:725–734. DOI: 10.1111/j.1439-0531.2008.01060.x.
- Ketterson ED, Nolan, Jr. 1999. Adaptation, exaptation, and constraint: A hormonal perspective. The American Naturalist 154(S1):S4–S25. DOI: 10.1086/303280.
- Kierdorf U, Kierdorf H, Schultz M, Rolf HJ. 2004. Histological structure of antlers in castrated male fallow deer (Dama dama). The Anatomical Record 281A:1352–1362. DOI: 10.1002/ar.a.20127.
- Konjević D, Janicki Z, Slavica A, Severin K, Krapinec K, Božić F, Palme R. 2011. Non-invasive monitoring of adrenocortical activity in free-ranging fallow deer (Dama dama L.). European Journal of Wildlife Research 57:77–81. DOI: 10.1007/s10344-010-0401-1.
- LaDue CA, Schulte BA, Kiso WK, Freeman EW. 2021. Musth and sexual selection in elephants: A review of signalling properties and potential fitness consequences. Behaviour 159(3–4):207–242. DOI: 10.1163/1568539X-bja10120.
- Landete-Castillejos T, Kierdorf H, Gomez S, Luna S, García AJ, Cappelli J, Pérez-Serrano M, Pérez-Barbería J, Gallego L, Kierdorf U. 2019. Antlers- Evolution, development, structure, composition, and biomechanics of an outstanding type of bone. Bone 128:115046. DOI: 10.1016/j.bone.2019.115046.
- Lawson RE, Putnam RJ, Fielding AH. 2001. Chemical communication in Eurasian deer (Cervidae): Do individual odours also code for attributes? Journal of Zoology 253:91–99. DOI: 10.1017/S0952836901000085.
- Lincoln GA. 1985. Seasonal breeding in deer. In: Fennessy PF, Drew KR, editors. Biology of deer production. Vol. 22. Wellington: Royal Society of New Zealand, Bulletin. pp. 165–179.
- Lincoln GA. 1987. Long-term stimulatory effects of a continuous infusion of LHRH agonist on testicular function in male red deer (Cervus elaphus). Reproduction 80:257–261. DOI: 10.1530/jrf.0.0800257.
- Lincoln GA, Guinness F, Short RV. 1972. The way in which testosterone controls the social and sexual behavior of the red deer stag (Cervus elaphus). Hormones and Behavior 3(4):375–396. DOI: 10.1016/0018-506X(72)90027-X.
- Lucas X. 2014. Clinical use of deslorelin (GnRH agonist) in companion animals: A review. Reproduction in Domestic Animals 49:64–71. DOI: 10.1111/rda.12388.
- Malo AF, Roldan ERS, Garde JJ, Soler AJ, Vicente J, Gortazar C, Gomendio M. 2009. What does testosterone do for red deer males? Proceedings of the Royal Society B: Biological Sciences 276:971–980.
- Martín J, Carranza J, López P, Alarcos S, Pérez-González J. 2014. A new sexual signal in rutting male red deer: Age related chemical scent constituents in the belly black spot. Mammalian Biology 79(6):362–368. DOI: 10.1016/j.mambio.2014.07.005.
- Massei G, Bowyer RT. 1999. Scent marking in fallow deer: Effects of lekking behavior on rubbing and wallowing. Journal of Mammalogy 80:633–638. DOI: 10.2307/1383307.
- Massei G, Cowan D. 2014. Fertility control to mitigate human–wildlife conflicts: A review. Wildlife Research 41:1. DOI: 10.1071/WR13141.
- Meier LR, Zou Y, Mongold-Diers JA et al. 2020. Pheromone composition and chemical ecology of six species of cerambycid beetles in the subfamily Lamiinae. Journal of Chemical Ecology 46(1):30–39. DOI: 10.1007/s10886-019-01128-7.
- Miller KV, Jemiolo B, Gassett JW, Jelinek I, Wiesler D, Novotny M. 1998. Putative chemical signals from white-tailed deer (Odocoileus virginianus): Social and seasonal effects on urinary volatile excretion in males. Journal of Chemical Ecology 24(4):673–683. DOI: 10.1023/A:1022342219469.
- Morgan KN, Tromborg CT. 2007. Sources of stress in captivity. Applied Animal Behaviour Science 102:262–302.
- Mormède P, Andanson S, Aupérin B, Beerda B, Guémené D, Malmkvist J, Manteca X, Manteuffel G, Prunet P, van Reenen CG et al. 2007. Exploration of the hypothalamic–pituitary–adrenal function as a tool to evaluate animal welfare. Physiology & Behavior 92:317–339. DOI: 10.1016/j.physbeh.2006.12.003.
- Motta M, Degli Esposti A. 1981. A computer program for mathematical treatment of data in radioimmunoassay. Computer Programs in Biomedicine 13:121–129. DOI: 10.1016/0010-468X(81)90090-8.
- Muller-Schwarze D, Altieri R, Porter N. 1984. Alert odor from skin gland in deer. Journal of Chemical Ecology 10(12):1707–1729. DOI: 10.1007/BF00987357.
- Mulley RC. 2007. Reproductive management of fallow deer. In: Current therapy in large animal theriogenology. 2nd ed. St. Louis: Sunders Elsevier. pp. 952–965.
- Padula AM. 2005. GnRH analogues—agonists and antagonists. Animal Reproduction Science 88:115–126. DOI: 10.1016/j.anireprosci.2005.05.005.
- Palm J, Balogh O, Reichler IM. 2012. The clinical use of Deslorelin acetate (Suprelorin®) in companion animal medicine. Schweizer Archiv für Tierheilkunde 154(1):7–12. DOI: 10.1024/0036-7281/a000286.
- Pavitt AT, Walling CA, Möstl E, Pemberton JM, Kruuk LEB. 2015. Cortisol but not testosterone is repeatable and varies with reproductive effort in wild red deer stags. General and Comparative Endocrinology 222:62–68. DOI: 10.1016/j.ygcen.2015.07.009.
- Petrulis A. 2013. Chemosignals, hormones and mammalian reproduction. Hormones and Behavior 63:723–741. DOI: 10.1016/j.yhbeh.2013.03.011.
- Rasmussen LEL, Riddle HS, Krishnamurthy V. 2002. Mellifluous matures to malodorous in musth. Nature 415:975–976. DOI: 10.1038/415975a.
- Schatz S, Palme R. 2001. Measurement of faecal cortisol metabolites in cats and dogs: A non-invasive method for evaluating adrenocortical function. Veterinary Research Communications 25(4):271–287. DOI: 10.1023/A:1010626608498.
- Setchell JM, Vaglio S, Abbott KM, Moggi-Cecchi J, Boscaro F, Pieraccini G, Knapp L. 2011. Odour signals MHC genotype in an Old World monkey. Proceedings Biological Sciences 278(1703):274–280. DOI: 10.1098/rspb.2010.0571.
- Setchell JM, Vaglio S, Moggi-Cecchi J, Boscaro F, Calamai L, Knapp L. 2010. Chemical composition of scent-gland secretions in an Old World monkey (Mandrillus sphinx): Influence of sex, male status, and individual identity. Chemical Senses 35(3):205–220. DOI: 10.1093/chemse/bjp105.
- Solari P, Crnjar R, Frongia A, Sollai G, Secci F, Spiga M, Masala C, Liscia A. 2007. Oxaspiropentane derivatives as effective sex pheromone analogues in the gypsy moth: Electrophysiological and behavioral evidence. Chemical Senses 32(8):755–763. DOI: 10.1093/chemse/bjm043.
- Soso SB. 2011. Ethnochemistry related to Siberian and Bengal tiger pheromones. Agricultural and Biosystems Engineering Conference Proceedings and Presentations, Iowa State University, Iowa, US, pp.1–36.
- Tamanini C, Giordano N, Chiesa F, Seren E. 1983. Plasma cortisol variations induced in the stallion by mating. Acta Endocrinol 102:447–450.
- Thom MD, Hurst JL. 2004. Individual recognition by scent. Annales Zoologici Fennici 41:765–787.
- Trigg TE, Doyle AG, Walsh JD, Swangchan-Uthai T. 2006. A review of advances in the use of the GnRH agonist deslorelin in control of reproduction. Theriogenology 66(6–7):1507–1512. DOI: 10.1016/j.theriogenology.2006.02.037.
- Vaglio S, Minicozzi P, Bonometti E, Mello G, Chiarelli B. 2009. Volatile signals during pregnancy: A possible chemical basis for mother-infant recognition. Journal of Chemical Ecology 35(1):131–139. DOI: 10.1007/s10886-008-9573-5.
- Vaglio S, Rodriguez Villanueva M, Ducroix L, Consiglio R, Kim JA, Neilands P, Stucky K, Lameira A. 2020. Female copulation calls vary with male ejaculation in captive olive baboons. Behaviour 157(8–9):807–822. DOI: 10.1163/1568539X-bja10024.
- Vannoni E, McElligott AG. 2008. Low frequency groans indicate larger and more dominant fallow deer (Dama dama) Males. Plos One 3:e3113. DOI: 10.1371/journal.pone.0003113.
- Varuzza P. 2019. Ungulati: Capriolo, cervo, daino, muflone e cinghiale. Teggiano, SA: Geographica Srl. pp. 174, 228.
- Viau V. 2002. Functional cross-talk between the hypothalamic-pituitary-gonadal and -adrenal axes. Journal of Neuroendocrinology 14:506–513. DOI: 10.1046/j.1365-2826.2002.00798.x.
- Walker D, Vaglio S. 2021. Sampling and analysis of animal scent signals. Journal of Visualized Experiments: JoVE 168:e60902. DOI:10.3791/60902.
- Wyatt T. 2003. Pheromones and Animal Behaviour. Cambridge, UK: Cambridge University Press.
- Ye F, Zuo M, Zhu W, Gao W, Zhang H, Hou D, Wang Z. 2018. Analysis of the chemical components in the urine of the tree shrew (Tupaia belangeri) by HS-SPME-GC/MS. ACTA Theriologica Sinica 38:01.