Abstract
Very few studies have investigated the influence of fish on the degradation rate of microplastic particles (MPs). It could be expected that their effect might be not only direct, through digestion and passage through the gut, but also indirect, through the alteration of the density and composition of the bacterial community on the surface of MPs. This study aims to test the short-term effects of fish presence altering the water chemical parameters and the abundance and genetic diversity of the aquatic bacteria colonising MPs, which may contribute to their faster degradation. This was tested in a triplicate experiment, with each replicate lasting 10 days in the presence or absence of spherical MPs, polystyrene (Ps) or polyethylene (Pe) and the presence or absence of a perch. The animal was separated from the MPs with a plankton net. We determined the chemical parameters of the water, the number of bacteria in the water and on the MPs’ surface, the taxonomic diversity of bacteria in the water and those present on the MPs using 16S rRNA gene (16S rDNA) sequencing, and the alterations of the MPs’ surface using a scanning electron microscope. The exposure to fish increased the concentration of ammonium, nitrates, and orthophosphates and the number of bacteria in the water, which in turn resulted in an increase in the abundance of bacteria and the number of taxa at the family level on the MPs’ surface. The positive effect of fish exudates on the abundance of bacteria on MPs’ surface was greater in the case of Pe than in Ps. The observed effects did not affect the MPs’ surface in any of the types of plastic during the experiment, but they may play a significant role in MPs decomposition over a longer than applied time period.
1. Introduction
Since the invention of organic polymers in the early 1900s, annual production of those light, multipurpose, insulation, and cheap materials has consistently increased, reaching 368 million tons in 2019 (Plastics Europe, and EPRO Citation2021). It has long been known that plastic accumulates in the environment due to improper waste disposal practices (Kenyon & Kridler Citation1969). However, until 1972, the main focus was on larger plastic items. It was only E. J. Carpenter who noticed the microplastics contamination (Carpenter et al. Citation1972), although the term itself was coined in an article investigating traces of plastic fragments and fibres in marine habitats, entitled Lost at Sea: Where Is All the Plastic? (Citation2004), which ignited the microplastics research boom.
Microplastics are any synthetic solid particles or polymeric matrices with a regular or irregular shape and a size ranging from 1 µm to 5 mm (Cole et al. Citation2011); other operationally defined size fractions are macroplastics (≥5 mm; Moore Citation2008; Lechthaler et al. Citation2020) and nanoplastics (<1 µm; Klaine et al. Citation2011; Gigault et al. Citation2018; Maszczyk et al. Citation2022a Citation2022b), of either primary or secondary manufacturing origin (purposely produced in microscopic sizes or degraded from larger plastic fragments) (Cole et al. Citation2011). Polypropylene, polyethylene, polystyrene, polyvinylchloride, and polyethylene terephthalate are the most commonly detected microplastic contaminants in environments around the globe (e.g. Ng & Obbard Citation2006; Hwang et al. Citation2020; Stanton et al. Citation2020). Microplastic particles (MPs) are commonly found in environments ranging from marine (Cole et al. Citation2011) and freshwater (Eerkes-Medrano et al. Citation2015), through soil ecosystems (Chae & An Citation2018), even in hard-to-reach places such as the Mariana Trench (Peng et al. Citation2018), Arctic and Antarctic (Bessa et al. Citation2019) or in the sediments of remote mountain lakes (Allen et al. Citation2019). MPs are also present in food items such as table salt (Danopoulos et al. Citation2020) and beer (Shruti et al. Citation2020) and were also found in animals (Browne et al. Citation2008), including human (Ragusa et al. Citation2021) tissues. The earliest traces of microplastics in living organisms were found in the digestive tissues of freshwater fish in a museum collection dating back to the 1950s (Hou et al. Citation2021). Due to the increasing worldwide production of plastic materials, the concentrations of MPs in the environment are increasing, and predictive models show that this trend will only accelerate in the coming years if appropriate mitigation measures are not taken (van Wijnen et al. Citation2019).
Currently, plastic pollution is receiving worldwide attention due to emerging discoveries regarding its impact on the environment and human health. The influence of microplastic pollution was confirmed at all integrative levels of biological organisation, including genetic (Sun et al. Citation2021), cellular (Prinz & Špela Citation2020), physiological (Franzellitti et al. Citation2019), organismal (Schrank et al. Citation2019), population (Amariei et al. Citation2021) and community (Lozano & Rillig Citation2020; Zebrowski et al. Citation2022). The effect may differ depending on particle degradability (biodegradable or non-biodegradable; Tang et al. Citation2021; Zebrowski et al. Citation2022), size and shape (Covernton et al. Citation2019), chemical properties (Xu et al. Citation2018), colour (Chen et al. Citation2020) and concentration (Sun et al. Citation2022). However, the reported effects in microplastic studies range from no observed toxicity to observations of a variety of toxicological endpoints (Booth & Sřrensen Citation2020). Furthermore, the concentrations used in the studies that found the effect usually far exceed those found in the natural environment (Lenz et al. Citation2016; Ockenden et al. Citation2021).
It has been demonstrated that various living organisms can affect the qualitative and quantitative (crushing MPs into a larger number of smaller fractions) characteristics of MPs. The presence of organisms can affect the circulation of MPs in the environment, including their removal from the water column by deposition in sediments (Pukos et al. Citation2023) and transfer within food webs (Elizalde-Velázque et al. Citation2020). Biotic degradation of plastics can occur directly: physically (by biting or chewing (So et al. Citation2022)) or chemically (enzymes during digestive degradation (Dawson et al. Citation2018; Cornwall Citation2021)).
Among the life forms examined in this context, microorganisms have received the most attention (Bahl et al. Citation2020; Matjašič et al. Citation2021; Venkatesh et al. Citation2021). Despite plastic’s hydrophobicity, its possession of stable covalent bonds and its high molecular weight, it has been suggested that, due to evolutionary selective pressure, the more the environment is exposed to plastic pollution, the higher its plastic-degrading potential (Yoshida et al. Citation2016). Plastic degrading bacteria have been discovered in habitats ranging from the open ocean to insect (Danso et al. Citation2019) and clitellate (Huerta Lwanga et al. Citation2018) digestive tracts.
Microbial plastic degradation can be divided into two categories: direct (a microbe using plastic as a source of nutrients) or indirect, causing deterioration (alteration of physical and chemical traits) or degradation (fragmentation) of a polymer through exposure to a microorganism’s metabolic products (Singh & Sharma Citation2008). Indirect biotic degradation might as well occur by organism-associated microbial activity (Widyananto et al. Citation2021). However, the indirect type of biotic degradation is the least studied. Studies on free-living and animal-associated bacterial plastic degradation are scarce; only one study confirmed the effect of coral-associated bacteria (Widyananto et al. Citation2021).
According to a systematic literature review on biodegradation studies on synthetic plastics, most of the bacteria claimed to possess those properties belong to the phyla Proteobacteria (48.0%), Firmicutes (37.4%), and Actinobacteriota (9.8%). Other phyla, although with rare mention of plastic-degrading strains, are as follows: Acidobacteriota, Actinobacteriota, Gemmatimonadota, Bacteroidota, Cyanobacteria, Fusobacteriota, Deinococcota and Nitrospirota. The most common genera mentioned were Pseudomonas, Bacillus and Brevibacillus (Matjašič et al., Citation2021).
MPs are good substrates for microbial colonisation due to their low biodegradability, high surface-area-to-volume ratio, and hydrophobicity (Murphy et al. Citation2020), because of this they provide new microbial niches in aquatic environments (Yang et al. Citation2020). The colonisation of microplastics is well documented and occurs within a few days (Harrison et al. Citation2014). Apart from outside factors, like temperature or water flow speed, microplastic characteristics, such as surface roughness (Kerr & Cowling Citation2003) and polymer type (Frère et al. Citation2018) influence the process of biofouling and the composition and structure of that bacterial community. It is well established that bacteria growing in biofilms are usually physiologically and taxonomically distinct from free-living bacteria. Biofilms are typically characterised by dense clusters of bacterial cells. These cells secrete extracellular polymeric substances that hold the cell aggregates together in a biofilm matrix (Hall-Stoodley et al. Citation2004).
In the natural environment, MPs come into contact with a variety of bacterial strains, including bacteria associated with freshwater fish, which have not been studied in-depth in the context of MPs’ colonisation and degradation potential and their influence on the genetic diversity of the MPs’ bacterial community. Fishes possess a diverse associated bacterial community (Wilson et al. Citation2007), which increases the possibility that some of these bacterial strains could potentially have a biochemical or physical impact on MPs.
Feeding habits and genotype clearly influence the gastrointestinal microbiota of fish, but Proteobacteria (including Enterobacter, Aeromonas, Acinetobacter, Vibrio, Pseudomonas, and Achromobacter) and Firmicutes are the dominant phyla in all fish species (e.g. Cahill Citation1990). Bacteroidota (e.g. Flavobacterium) and Actinobacteriota (e.g. Corynebacterium and Micrococcus) are other dominant phyla, except in carnivorous fish (Cahill Citation1990; Li et al. Citation2014). Other dominant microbiota in the intestine of freshwater fish are represented by Fusobacteriota and Tenericutes (Kashinskaya et al. Citation2019). This general pattern was confirmed for the microbiota of the perch (Perca fluviatilis), which is dominated by the phyla Proteobacteria, Firmicutes and Fusobacteriota, as well as Tenericutes (Kashinskaya et al. Citation2019). As the composition of the microbial communities associated with the fish is dominated by the phyla in which bacterial taxa having the ability to break down MPs were identified most often (e.g. Proteobacteria, Firmicutes, Actinobacteriota, Bacteroidota, and Fusobacteriota), it is very likely that the fish presence may result in an increase in the contribution of those taxa on the surface of MPs, which in turn may result in an increase in the degradation rate of MPs. The composition of the microbiota of perch at the genus level varied between different studies, being dominated either by Myroides, Prochlorococcus, Anabaenopsis, Cetobacterium and Fusobacteriaceae (Zha et al., Citation2018) or Aeromonas spp. and Pseudomonas spp. (Goldschmidt-Clermont et al., Citation2008) or Escherichia spp., Bacillus spp., Aerobacter spp., and Proteus spp. (Horsley Citation1977).
So far, the bacteria used in biodegradation studies have primarily come from plastic-polluted environments or animal intestines (Matjašič et al. Citation2021), without taking into account the degradation potential of animal-associated free-living bacteria. Due to the fact that the presence of aquatic organisms, including fish, changes both the water chemistry (e.g. the content of nutrients and pH) and the taxonomic composition of microorganisms, it might affect the rate of MPs’ degradation. These assumptions have yet to be experimentally validated. Therefore, the aim of our research was to test several hypotheses concerning the effect of fish presence on the bacterial community colonising MPs and in turn, their potential to increase the decomposition rate of MPs. The first: the presence of a fish affects the quantitative (density) and qualitative (taxonomy) characteristics of free-living bacteria and bacterial communities colonising the MPs’ surface by changing water chemistry parameters and introducing new bacterial strains. The second: there is a notable difference in fish influence on bacterial communities attached to the surface of different types of MPs. The third: the presence of the fish indirectly (through alterations of the water chemistry and bacterial composition on MPs particles) influences the quality features of the MPs’ surface, measured as the diameter and circumference of the particles.
2. Materials and methods
2.1. Experimental animals
Juvenile European perch (Perca fluviatilis) were collected by seining in Lake Roś, located in the Masurian Lake District in Poland, a region where microplastic freshwater contamination has been confirmed (Kaliszewicz et al. Citation2020). Planktivorous fish are abundant in this postglacial, dimictic, eutrophic lake (Jachner Citation1991) that is also exposed to strong anthropogenic pressure. During the day, juvenile perch, which are planktivorous, migrate there to the littoral, where they prey mainly on copepods (Jachner Citation1991). All captured fish measured less than 7 cm in length. Before the experiment, the animals were kept for 6 days in an aquarium with preconditioned tap water (homogenised and filtered through a 2 µm mesh filter), an air pump, and an aquarium heater, in order to acclimate them to the laboratory environment, and then randomly selected for appropriate variants. The experimental procedure was approved by the First Warsaw Local Ethics Committee for Animal Experimentation (Permit protocol No. 1350P1/2022).
2.2. Microplastics
In the experiments, we used two different types of synthetic, non-biodegradable polymers, with a density similar to that of natural water (Zhou et al. Citation2021), in the form of microspheres. The first one was polystyrene (Ps), slightly heavier than water, which causes its faster sedimentation (d = 1.05 g × cm−3), with a mean diameter of 258.3 µm, catalogue number PS250K (Lab261®, USA). The second type of plastic was a high-density polyethylene (Pe), slightly lighter than water (d = 0.96 g × cm−3), which causes floating close to the surface, with a mean diameter of 275 µm, catalogue number 110616–4 (Cospheric®, UK). Those two polymers are among the materials most commonly identified as microplastic pollutants (Wu et al. Citation2016).
2.3. Experimental setup
The experimental system for fish was comprised of eighteen 5 L glass containers placed randomly in a water bath (L = 220, W = 40, H = 40 cm, V = 352 L) holding internal submersible water-heaters (Aquael® Neoheater 150 W) and water pumps (Aquael® Circulator 500), inside a room, which allowed to maintain stable temperature (21 ± 0.2°C) and light conditions. The light intensity reflected the summer photoperiod (16 L:8°D, 10.0 ± 0.5 µmol°×°m−2 × s−1, just under the surface of each of the containers) measured with Li-Cor 189 quantum sensor measuring radiance (Li-Cor Biosciences®). The potential influence of external light sources was eliminated by wrapping the walls of the water bath with a black, opaque film. The water bath was filled with aerated tap water, which was conditioned 6 days before the experiments (filtered through 2 µm mesh filters). The experimental containers were connected to the 8-channel aeration pumps (Hailea® ACO-9630) via pipes ending with glass tubes in order to minimise the contact of experimental plastic with laboratory plastic. In each of the 18 containers, a 150 µm net attached to a U-shaped frame was placed, which separated MPs from the animal in order to avoid the possibility of potential MP degradation as a result of passage through the digestive system. The intentional placement of the net in control tanks was aimed at ensuring uniform experimental conditions. A concentration of 3000 MPs × L−1 was utilized to promote adequate surface area for bacterial proliferation. During the experiment, we minimised the contact of the experimental organisms with plastic objects other than those used as a factor in the experiment. For example, all containers, tubes, and frames that came into contact with water in each tank were made of glass.
2.4. Experimental procedure
The experiment was conducted in July 2021 at the Hydrobiological Station in Pilchy, University of Warsaw, thanks to the authorities of the station. Eighteen containers were the combination of six variants replicated simultaneously three times, during a 10-day experiment. Each day at 10 a.m., the fish were fed with frozen bloodworms, and temperature and oxygenation were measured using an oxygen probe (YSI ProODO). Any leftover food debris was immediately removed. After 10 days, we collected samples of water and MPs. We examined the variants with the presence or absence of a fish and the presence of two types of microplastics. The experiment included the following variants: a fish and Ps particles (F-Ps), a fish and Pe particles (F-Pe), Pe particles only (Pe), Ps particles only (Ps), fish only (F) and water only (C) as a control.
A randomly selected perch was placed in each container with a fish variant (F, F-Pe and F-Ps). The experimental conditions, i.e. the size and structure, the amount of water, light exposure, temperature, and oxygen supply, were the same for each glass container, representing a single replicate of one of the six variants.
At the end of the experiment, in order to examine changes in water chemistry caused by the presence of a fish or possibly by the presence of MPs, concentrations of ammonium nitrogen, nitrates, and orthophosphates were measured in each of the 18 glass containers. For the analysis of ammonium ions, we used a method proposed by Holmes et al. Citation1999. For the analysis of total nitrogen, we used commercially certified Merck-Millipore cell tests (Spectroquant® nitrogen (Total) Cell Test 1.14537) according to the manufacturer’s instructions, using the Merck Spectroquant Pharo 300 spectrophotometer. The orthophosphate concentrations were determined using the molybdenum method (Nagul et al. Citation2015) using a Shimadzu UV-VIS 1201 spectrophotometer.
For the calculation of bacteria abundance, samples of MPs and water were conserved with 2% formalin and processed according to Porter and Feig (Citation1980). Free-living bacteria and bacteria attached to MPs were stained with DAPI (4’,6-diamidino-2-phenylindole, 10 min, temp. 24°C, final DAPI concentration – 1 µg L−1), suspended on the surface of 0.2-µm, black polycarbonate membrane filters (Millipore) by filtration and then examined under Nikon epifluorescence E450 microscope equipped with Nikon Digital Camera DXM 1200 F and NIS elements software (Nikon). In water samples, free-living bacteria were counted in 10 randomly selected 10 fields of view. In MPs’ samples, biofilm bacteria were counted on the surface of 10 randomly selected particles and expressed as the number of bacteria per µm2.
For taxonomic identification of bacteria on MPs nearly 2000 particles were collected through a sterilised strainer and immediately frozen for further DNA isolation. For taxonomic identification of free-living bacteria 200 ml of water from the experimental tank was filtered through the 0.2-µm polycarbonate filter (Merck Isopore™ Membrane Filters), and the filter was also placed in the Eppendorf probe and frozen. The filters were cut into small pieces prior to DNA isolation. DNA extraction was performed using the Gene MATRIX Soil DNA Purification Kit (EURx, Poland) according to the manufacturer’s instructions.
In order to examine the surface and determine any potentially visible surface alterations caused by the bacteria and biofilm presence, we used scanning electron microscopy (SEM). The images of the particles were obtained in the Laboratory of Electron and Confocal Microscopy at the Faculty of Biology, University of Warsaw. The dried microplastic particles were mounted on a SEM mounting stub, sputter-coated with gold using the POLARON SC7620 metal sputtering machine by Microtech, and examined with the use of a LEO 1430VP ZEISS scanning electron microscope with 400 × magnification.
For the ratio of the changed surface to unchanged, for the number of visible bacteria on the surface and for the presence or absence of biofilm on SEM photographs, we used ImageJ 1.53 g software (Schindelin et al. Citation2012).
2.5. Data analyses
The statistical analysis was performed using the R platform (v.4.2.0). In all statistical tests, the significance level was set to α = 0.05. Before selecting an appropriate test, we checked the assumption for ANOVA (analysis of variance) by performing the Shapiro–Wilk normality test and Levene’s test for homogeneity of variance.
To test the differences in the abiotic parameters (temperature and oxygen concentration) between all the experimental treatments, we performed a three-way mixed ANOVA using the “ezANOVA” function (the “ez” package v.4.4–0). The Greenhouse–Geisser correction was applied if the sphericity checked by Mauchly’s test was violated. The presence of the fish and MPs (Ps or Pe) were set as between-subject factors, and time as a within-subject factor. To test the effects of the presence of a fish and MPs (Ps or Pe) on the number of bacteria in water, the chemical parameters of water quality (the concentration of ammonium nitrogen, nitrates and orthophosphates) and the effect of the presence of a fish on the number of bacteria on different types of MPs and the microplastics’ physical properties (diameter and circumference), we conducted a two-way ANOVA using the “aov_car” function (“afex” package v.1.1–1).
To determine the taxonomic composition of the bacterial community, Bray–Curtis-based NMDS (nonparametric multidimensional scaling) was performed on relative abundance of bacteria domain representatives on the family level.
Planned contrasts for estimated marginal means (EMMs; the “emmeans” package v.1.7.5) were used to compare the effect of fish with control separately for each of the types of microplastics on the chemical parameters of water quality, on the number of free-living bacteria, on the number of bacteria on MPs and on MPs’ physical properties. The “holm” p-value adjustment was applied to control Type I error inflation as a result of multiple testing.
3. Results
3.1. Microplastics physical properties – diameter and circumference
The presence of fish did not affect the diameter and circumference of MPs at the end of experiments (, )); however, the circumference depended on the type of MPs (, )). The diameter and circumference of Pe (in relation to Ps) were greater in both the presence and absence of fish (Table S1, Supplementary materials, )).
Figure 1. (a) Selected parameters characterising the surface of the MP: diameter and circumference at the end of the experiments (mean ± 1SD) in all of the treatments. Statistically significant differences between treatments are denoted with different letters, (b) SEM images of MPs at the end of the experiments in all of the treatments. Magnification ×400.
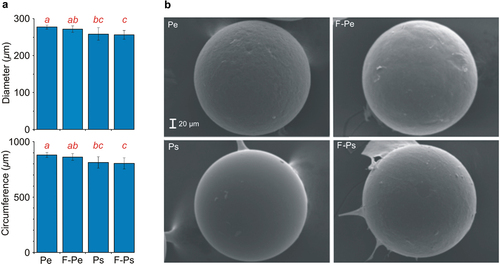
Table I. The results of two-way ANOVA to test the effect of the presence of the fish (F) and MPs type on MPs’ physical properties (diameter and circumference) at the end of experiments. Statistically significant (α = 0.05) differences are marked with bold (df – degrees of freedom. SS – Sum of Squares. F – F-statistics, P – p-value).
3.2. Experimental conditions
Oxygen concentration was affected by the presence of the fish (). The effect was apparent in both the absence of MPs and in the presence of Pe (). The temperature value and oxygen concentration were not affected by the presence of MPs (, ).
Figure 2. Experimental conditions: temperature and oxygen concentration as the mean (± 1SD) for all of the experiments in each of the experimental days, and orthophosphates, ammonium ions and total nitrogen concentrations at the end of the experiments (mean ± 1SD) in all of the treatments: the control (c), and the presence of the fish (f), polyethylene (Pe), fish and polyethylene (F-Pe), polystyrene (Ps) and fish and polystyrene (F-Ps). Statistically significant differences between treatments are denoted with different letters.
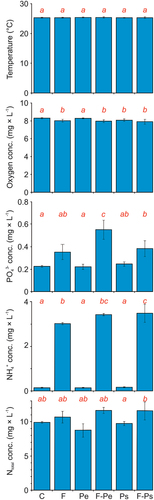
Table II. Results of the three-way mixed ANOVA to test the effect of all experimental treatments (F – presence of the fish, type of MPs and Ti – time) on the abiotic parameters (temperature and oxygen concentrations) and the results of the two-way ANOVA to test the effect of the presence of the fish and type of MPs on the chemical parameters of water quality (the concentrations of orthophosphates, ammonium ions, and total nitrogen). Statistically significant (α = 0.05) differences are marked with bold (df – degrees of freedom, F – F-statistics, P – p-value).
3.3. Chemical parameters of water quality
Generally, the presence of a fish had a significant effect on the concentrations of orthophosphates and ammonium ions, however there was no significant effect of fish on the total nitrogen concentration (). The presence of MPs did not affect any of the chemical parameters of water (). The concentration of orthophosphates was greater in the presence of fish than in its absence in the treatments with MPs (Table S2, ). The concentration of orthophosphates was also significantly higher in the presence of the fish and Pe, compared to the presence of the fish without Pe (Table S2, ). In the presence of the fish, the concentration of orthophosphates was significantly higher in the treatment with Pe than in the treatment with Ps (Table S2, ).
The ammonia concentration was higher in the presence of the fish (both with and without MPs, independently of their type) compared to the control (Table S2, ), and was greater in the presence of fish than in its absence in both types of MPs (Table S2, ). Moreover, the concentration of ammonia was significantly higher in the presence of the fish and Ps, compared to the presence of the fish without Ps (Table S2, ). The total nitrogen concentration was significantly higher in the treatment with fish and Ps compared to the treatment with Ps without fish (Table S2, ).
3.4. Number of bacteria
The number of free-living bacteria was affected by the fish presence and by the type of MPs (, )). In all variants with the presence of the fish (with and without each of the types of MPs) the number of bacteria was greater compared to the control (Table S3, )). In the Pe treatments, the number of bacteria was greater in the presence than in the absence of fish (Table S3, )).
Figure 3. The number of bacteria in water (A), and on the MPs’ surface (B) at the end of the experiments (mean ± 1SD) in all of the treatments. Statistically significant differences between treatments are denoted with different letters.
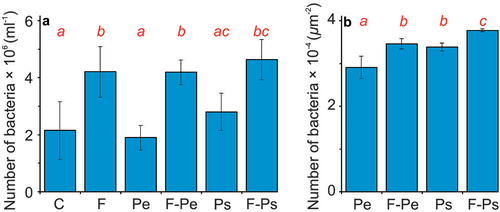
Table III. The results of a two-way ANOVA to test the effect of the presence of the fish (F) and microplastics particles (MPs) on the number of free-living bacteria in the experimental media and on the number of bacteria on MPs at the end of the experiment. Statistically significant differences (α = 0.05) are marked with bold (df – degrees of freedom, SS – Sum of Squares, F – F-statistics, P – p-value).
In the case of the number of bacteria on the MPs’ surface, we observed both the effect of the fish presence and the type of MPs (, )). The number of bacteria on the MPs’ surface was greater in the presence than in the absence of fish and at the surface of Ps than Pe particles (Table. S3, )). The analysis of the planned contrasts revealed that the effect of the fish was significant in each of the plastic types, and the difference between MP types was significant in both the presence and absence of fish (Table S3, )).
3.5. Taxonomic composition of bacterial community
The most abundant bacterial taxa at the phylum level in all samples were Proteobacteria, followed by Bacteroidota and Verrucomicrobiota. In free-living bacterial communities, greater contribution of Verrucomicrobiota and Actinobacteriota was apparent in the fish than in no-fish media. The most apparent difference between free-living bacteria and bacteria attached to MPs particles was the lower contribution of Bacteroidota and Proteobacteria and the greater contribution of Bdellovibrionota in the latter than in the former. Moreover, Actinobacteriota were present in water samples in the fish treatments but not on the surface of particles, neither in fish nor in no-fish treatments. Also, Cyanobacteria was present only in free-living bacteria fraction in several treatments (Pe, F-Pe and Ps), but not on the surface of MPs ()).
Figure 4. The relative abundances (top 13 most abundant ASVs) of the dominant bacteria taxa at the phylum level in water (a) and on the microplastic particle surface (b) at the end of the experiments (mean ± 1SD) in all of the treatments. If the frequency was lower than 0.2%, the taxa were assigned to the “Other” category.
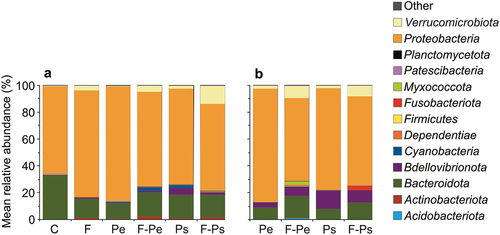
On the MPs’ surface, greater contributions of Verrucomicrobiota and Bacteroidota and a lower contribution of Proteobacteria were in the fish medium in relation to the no fish medium. On the surface of Ps particles in the fish medium, the significant phylum was Fusobacteriota, and on the surface of Pe particles in the fish medium, the significant phyla were Myxococcota and Acidobacteriota ().
The number of taxa at the family level of the free-living bacteria was lower in the fish medium, and greater in the presence of MPs (in both fish and no fish medium) in relation to the control ()). The number of taxa at the family level of bacteria on MPs’ surfaces was greater in the presence than in the absence of fish in both types of MPs ()). In the absence of fish, the number of taxa on MPs was lower compared to the number of taxa of free-living bacteria. In the presence of fish, this relation was reversed.
Figure 5. The mean number of taxa at the family level (a, b) and Simpson 1-D index (± 1SD, c, d) in water (a, c) and on the microplastic particles surface (b, d) at the end of the experiments in all of the treatments.
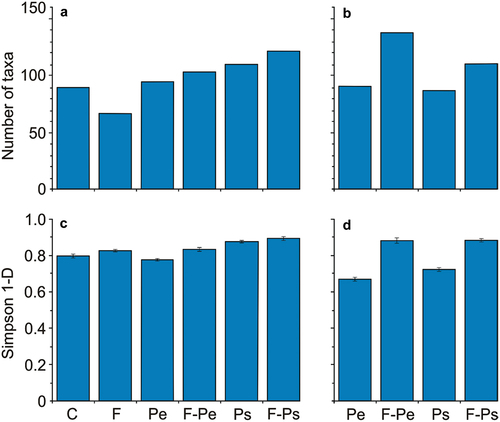
The Simpson 1-D index for the free-living bacteria was relatively higher in the presence of fish. The effect of MPs was only apparent in the case of Ps ()). The Simpson 1-D index for samples collected from MPs’ surface was significantly greater in the presence of fish compared to its absence for both types of MPs ()). In the absence of fish, the Simpson 1-D index for the taxa on MPs was lower compared to its value for free-living bacteria. In the presence of fish, this relationship was reversed.
The NMDS of the values of the Bray–Curtis index calculated on the basis of the relative abundances of taxa on family level in water and on the MP surface revealed five distinct groups of samples: (1) control water samples, (2) water samples from variants in the presence of fish and Ps or Pe and in the absence of fish and presence of Pe, (3) PS water samples in the absence of fish, (4) samples on the surface of MPs of both types in the absence of fish, (5) samples on the surface of MPs of both types in the presence of fish (). In general, at the family level the taxonomic composition of the biofilm bacteria community is different from that of the free-living bacteria community in the context of different proportions of the same bacterial families on the particles and in water ().
Figure 6. NMDS of the values of the Bray–Curtis index calculated on the basis of relative abundances of taxa on family level in water and on the microplastic particle surface at the end of the experiments (mean ± 1SD) in all of the treatments, separately in each of the two experiments for which the analysis was performed (a and b in the subscript).
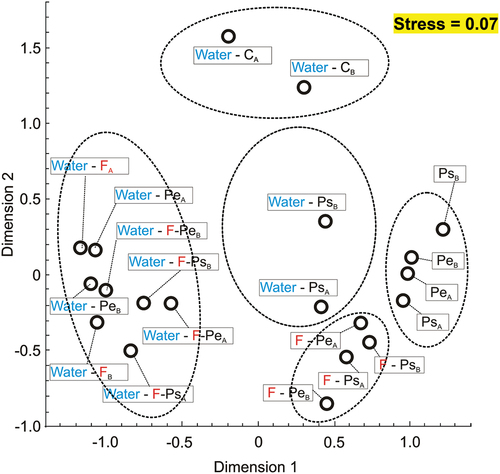
4. Discussion
The results of our study confirmed the first hypothesis, as the presence of a fish affects the quantitative (density) and qualitative (taxonomy) characteristics of free-living bacteria and bacterial communities colonising the MPs’ surface. The effects were accompanied by a large increase in the concentrations of orthophosphates and ammonium ions and the nitrogen concentration was higher in the treatment with fish and Ps. The effect of the fish on the increase in concentrations of dissolved mineral phosphorus and nitrogen is consistent with the numerous theoretical (e.g. Zimmer et al. Citation2006; Verant et al. Citation2007) and experimental (Kitchell et al. Citation1975; Threlkeld Citation1987; Vanni & Findlay Citation1990) studies on the effect of fish egestion and excretion on water chemistry, which revealed that it can be an important source of nutrients in aquatic ecosystems (e.g. Atkinson et al. Citation2017). The potential for significant contribution of a fish to phosphorus recycling in limnetic systems via excretion was first raised by Kitchell et al. (Citation1975), who concluded that phosphorus excretion by bluegill (Lepomis macrochirus) in a small lake may support algal populations during periods of low phosphorus availability. In other studies, it has been revealed that nutrient release by fish might play an important role in phosphorus cycling and algal production (Threlkeld Citation1987) and that excretion of phosphorus by fish can be equal to or even greater than that of zooplankton (e.g. Vanni & Findlay Citation1990; Carpenter et al., Citation1992).
It has been revealed that fish activity may increase the abundance of free-living bacteria directly through released nutrients and indirectly through trophic interactions (e.g. Jeppesen et al. Citation1998; Villéger et al. Citation2019), although most of the previous studies did not disentangle these effects. For instance, in the long-term study of shallow and hypertrophic Lake Sřbygĺrd (Denmark), it has been revealed that temporal changes in fish stock affected through cascading effects the abundance of bacterial communities, although this effect was less apparent than the cascading effect on zooplankton and phytoplankton (Jeppesen et al. Citation1998). In other study, it has been revealed that the Mediterranean fish – Sparus aurata had a positive effect of nutrient excretion on the abundance of the microbial plankton, including bacterioplankton in the absence of large zooplankton when their growth was limited by nutrient availability (Villéger et al. Citation2019). As the dominant organisms in our experimental media were bacteria, it could be assumed that the increase in their abundance was only due to direct effect i.e. due to nutrients released by the fish. We are aware of only a single previous study in which a similar observation was made (Maszczyk & Bartosiewicz Citation2012). In this study, chemicals originating from fish activity fertilised the environment enough for a significant increase in bacterial abundance, which positively affected the growth and other life history parameters of their consumer – the planktonic cladoceran Daphnia. More importantly, in our study the greater abundance of the free-living bacteria was parallel to a greater abundance of bacteria on the MPs surface. To our knowledge, this is the first evidence in the literature concerning this issue. As only some bacterial strains possess the ability to decompose plastics, an increased abundance of bacteria in the presence of fish exudates increases the chance of more efficient MPs decomposition.
The results of our study also revealed that the nutrient excretion by fish altered the taxonomic composition of the free-living bacteria, which is consistent with numerous previous studies (e.g. Schaus & Vanni Citation2000; Villéger et al. Citation2019; Molina & Fernández Citation2020). For instance, it has been revealed that the nutrient excretion by the Mediterranean fish – Sparus aurata altered the community structure of the microbial plankton, including heterotrophic bacteria in the absence of large zooplankton (Villéger et al. Citation2019). A similar effect was observed by Schaus and Vanni (Citation2000), who found that the gizzard shad (Dorosoma cepedianum) excretion resulted in changes in the relative abundance of different taxa within the phototrophic unicellular organisms, including Cyanobacteria. Although the aforementioned studies indicated that the presence of fish exudates differentiates bacterial composition, they did not disentangle the direct effect of nutrients released by the fish or the indirect effect of the metabolites excreted by other organisms present in the experiments. Another example of the study concerned the effect of dissolved organic matter and inorganic nitrogen release through fish (Salmo salar) epithelium mucus on the natural bacterial assemblages from Northern Patagonia, Chile (Molina & Fernández Citation2020). The study revealed that fish mucus can cause rapid modifications in microbial assemblages, which was apparent in the relative increase in the abundance of Proteobacteria and Bacteroidota. The results of our study are not consistent with this observation, as we did not find any difference in the relative contribution of Proteobacteria and Bacteroidota between the fish and no fish media. Instead, Verrucomicrobiota and Actinobacteriota were more numerous in the fish media. Moreover, Actinobacteriota was specific only to the fish media. Overall, the number of taxa at the family level of the free-living bacteria was lower, but their diversity was greater in the fish medium. It could be assumed that the change in bacterial composition in the fish media was due to the net effect of altered physicochemical water parameters, including nutrients and oxygen concentrations, and enrichment of the media by introducing fish-associated bacterial strains. As different bacterial strains have different environmental requirements, it could be expected that altered environmental parameters due to the presence of fish stimulate changes in interactions within and between different bacterial taxa, having a profound impact on the outcome of their competition (e.g. Hibbing et al. Citation2009). For the same reasons, decreased oxygen concentrations in fish treatments, which were caused by the fish’s respiration, might have influenced the composition of free-living bacteria as well. Overall, the changes in microbial composition obtained in our study in the fish medium are consistent with the broad literature concerning the composition of fish-associated bacteria, in which Proteobacteria is the dominant phylum in all fish species, and Actinobacteriota are also well represented (Cahill Citation1990; Li et al. Citation2014).
In our study, the change in the taxonomic composition of free-living bacteria was accompanied by a change in the taxonomic composition of bacterial assemblages on the surface of the MPs. Overall, in the bacterial assemblages on MPs particles, there was a lower contribution of Bacteroidota, Proteobacteria, Actinobacteriota, and Cyanobacteria and a greater contribution of Bdellovibrionota, Fusobacteriota, Myxococcota, Acidobacteriota, and Verrucomicrobiota than in free-living bacteria fraction. The results are consistent with the numerous studies revealing that biofilm communities on MPs differ from the communities in the surrounding environment (e.g. Rummel et al. Citation2017; Ogonowski et al. Citation2018; Miao et al. Citation2019; Rosato et al. Citation2020). Therefore, MPs can be considered a distinct ecological habitat for diverse microbial communities, the “plastisphere” (Zettler et al. Citation2013), potentially characterised by distinct microbial and ecological functions (Miao et al. Citation2019). Moreover, our study seems to be the first one concerning the alteration of the composition of bacterial assemblages on the MPs surface in the presence of fish. It is clear from the study that the number of taxa and their variability in the bacterial assemblages on the MPs surface increased in the presence of fish. This was evident in the increase in the relative contributions of Fusobacteriota, Myxococcota, Acidobacteriota, Bacteroidota and Verrucomicrobiota, which was accompanied by a decrease in the relative contribution of Proteobacteria on the MPs surface in the fish media in relation to no fish media, which suggests that the presence of the fish introduces new bacterial strains with a greater potential to create biofilms. Dominant bacterial strains in the absence of fish are potentially being displaced competitively by fish-associated bacteria due to the competition for surface. Due to the fact that only some strains possess the ability to decompose MPs, it is not possible to state whether the fish exudates modify the rate of MP decomposition based on how it affects the relative contribution of different taxa at the phylum level. However, as the composition of the microbial communities on MPs surface associated with fish presence is more diverse, it can be assumed that the chance that such a community contains bacteria with the ability to decompose MPs increases.
The results also confirmed predictions of the second hypothesis as we observed apparent differences in the fish effect on bacterial communities associated with the surface of different types of MPs. The positive effect of fish was greater on the abundance of the bacterial community on the surface of Pe than on Ps. Although our study seems to be the first one concerning the effect of fish exudates on the bacterial communities associated with the surface of different types of MPs, the results of our study are consistent with previous observations that the microbial community on MPs’ surface depends on the polymer type (e.g. Hori et al., Citation2010; Fotopoulou et al., Citation2012; Hossain et al. Citation2019; Parrish & Fahrenfeld Citation2019; Rosato et al. Citation2020; Tu et al. Citation2021). For instance, it has been revealed that within 2 weeks of incubation, the bacterial abundance was greatest on PVC, lower on Pe, and lowest on PET, Pp, and Ps pellets (Rosato et al. Citation2020). Also, Hossain et al. (Citation2019) showed that bacterial colonisation of MPs is affected by the physicochemical properties of MPs, including their surface roughness and the type of plastic, although they did not find a difference in the bacterial density at the surface of the types of plastics used in our study (HDPE and Ps). However, it should be pointed out that in our study, the effect of fish was similar in terms of the number of taxa and diversity of taxonomic composition on the surface of both types of MPs. The only differences were apparently greater effects of fish on the contribution of Fusobacteriota on the surface of Ps and a greater effect of fish on the contribution of Myxococcota and Acidobacteriota on the surface of Pe. This is visible also on the SEM photographs ()), which indicate that Ps has a relatively smooth surface in comparison with Pe with a more granular structure on the surface and with that some Fusobacteriota have strong abilities to attach to smoother surfaces (Moses et al. Citation2020).
Additionally, the presence of different MPs also had a slight but significant effect on water chemistry, and this effect was different in the fish and no-fish media. That is, the presence of Pe results in an increase in the concentrations of orthophosphates and the presence of Ps results in an increase in the concentrations of ammonia only in fish treatments. This is in accordance with studies indicating that the presence of polystyrene increases ammonia excretion of black rockfish (Sebastes schlegelii; Yin et al. Citation2019) or disturbs it in medaka (Oryzias melastigma) gills (Zheng & Wang Citation2022). Another explanation may consist of the differential effect of MPs on the qualitative and quantitative compositions of free-living and associated with MPs bacterial assemblages (e.g. Rosato et al. Citation2020), which may utilise nutrients produced by fish to a different extent.
The last hypothesis, that the presence of the fish indirectly (i.e., through alterations of the water chemistry and bacterial composition on MPs particles) influences the quality features of the MPs’ surface, was not confirmed, as after 10 days, there was no significant change in the circumference and diameter of the particles of any type. However, the changes in the physicochemical parameters of the water as well as the quantitative and qualitative changes in bacteria in the presence of the fish exudates observed in our study suggest that a longer exposure to the fish exudates could accelerate the deterioration or decomposition of the MPs.
5. Conclusions
Overall, the results revealed that the presence of a fish affected the chemical parameters of the water and the quantitative (density) and qualitative (taxonomy) characteristics of free-living bacteria and bacterial communities colonising the MPs’ surface. Although those changes did not affect the apparent modification of the surface of MPs, they suggest that longer than applied in our study, exposure to fish may have an effect on the decomposition of MPs. To the best of our knowledge, these results provide the first evidence of the direct effect of aquatic animals on the plastisphere and, in turn, their significance on the self-purification of water from plastics.
Supplemental Material
Download MS Word (23.1 KB)Acknowledgements
All applicable institutional and national guidelines for the care and use of animals were followed.
Disclosure statement
No potential conflict of interest was reported by the author(s).
Supplementary material
Supplemental data for this article can be accessed online at https://doi.org/10.1080/24750263.2023.2217200.
Additional information
Funding
References
- Allen S, Allen D, Phoenix VR, Le Roux G, Durántez Jiménez P, Simonneau A, Binet S, Galop D. 2019. Atmospheric transport and deposition of microplastics in a remote mountain catchment. Nature Geoscience 12(5):339–344. DOI: 10.1038/s41561-019-0335-5.
- Amariei G, Rosal R, Fernández-Pińas F, Koelmans AA. 2021. Negative food dilution and positive biofilm carrier effects of microplastic ingestion by D. magna cause tipping points at the population level. Environmental Pollution 294:118622. DOI: 10.1016/j.envpol.2021.118622.
- Atkinson CL, Capps KA, Rugenski AT, Vanni MJ. 2017. Consumer‐driven nutrient dynamics in freshwater ecosystems: From individuals to ecosystems. Biological Reviews 92(4):2003–2023. DOI: 10.1111/brv.12318.
- Bahl S, Dolma J, Jyot Singh J, Sehgal S. 2020. Biodegradation of plastics: A state of the art review. Materials Today: Proceedings 39:31–34. DOI: 10.1016/j.matpr.2020.06.096.
- Bessa F, Ratcliffe N, Otero V, Sobral P, Marques JC, Waluda CM, Trathan PN, Xavier JC. 2019. Microplastics in gentoo penguins from the Antarctic region. Scientific Reports 9(1):14191. DOI: 10.1038/s41598-019-50621-2.
- Booth AM, Sřrensen L. 2020. Microplastic fate and impacts in the environment. In: Rocha-Santos T, Costa M, Mouneyrac C, editors. Handbook of microplastics in the environment. Cham: Springer. pp. 1–24. DOI: 10.1007/978-3-030-10618-8.
- Browne MA, Dissanayake A, Galloway TS, Lowe DM, Thompson RC. 2008. Ingested microscopic plastic translocates to the circulatory system of the Mussel, Mytilus edulis (L.). Environmental Science & Technology 42(13):5026–5031. DOI: 10.1021/es800249a.
- Cahill MM. 1990. Bacterial flora of fishes: A review. Microbial Ecology 19(1):21–41. DOI: 10.1007/BF02015051.
- Carpenter SR. 1992. Destabilization of planktonic ecosystems and blooms of blue-green algae. In: Kitchell JF, editor. Food web management: A case study of Lake Mendota. New York, NY: Springer-Verlag. pp. 461–482. DOI: 10.1007/978-1-4612-4410-3_23.
- Carpenter EJ, Anderson SJ, Harvey GR, Miklas HP, Peck BB. 1972. Polystyrene spherules in coastal waters. Science 178(4062):749–750. DOI: 10.1126/science.178.4062.749.
- Chae Y, An YJ. 2018. Current research trends on plastic pollution and ecological impacts on the soil ecosystem: A review. Environmental Pollution 240:387–395. DOI: 10.1016/j.envpol.2018.05.008.
- Chen Q, Li Y, Li B. 2020. Is color a matter of concern during microplastic exposure to Scenedesmus obliquus and Daphnia magna? Journal of Hazardous Materials 383:121224. DOI: 10.1016/j.jhazmat.2019.121224.
- Cole M, Lindeque P, Halsband C, Galloway TS. 2011. Microplastics as contaminants in the marine environment: A review. Marine Pollution Bulletin 62(12):2588–2597. DOI: 10.1016/j.marpolbul.2011.09.025.
- Cornwall W. 2021. The plastic eaters. Science 373(6550):36–39. DOI: 10.1126/science.373.6550.36.
- Covernton GA, Pearce CM, Gurney-Smith HJ, Chastain SG, Ross PS, Dower JF, Dudas SE. 2019. Size and shape matter: A preliminary analysis of microplastic sampling technique in seawater studies with implications for ecological risk assessment. Science of the Total Environment 667:124–132. DOI: 10.1016/j.scitotenv.2019.02.346.
- Danopoulos E, Jenner L, Twiddy M, Rotchell JM. 2020. Microplastic contamination of salt intended for human consumption: A systematic review and meta-analysis. SN Applied Sciences 2(12):1950. DOI: 10.1007/s42452-020-03749-0.
- Danso D, Chow J, Streit WR. 2019. Plastics: Environmental and biotechnological perspectives on microbial degradation. Applied and Environmental Microbiology 85(19):e01095–19. DOI: 10.1128/aem.01095-19.
- Dawson AL, Kawaguchi S, King CK, Townsend KA, King R, Huston WM, Bengtson Nash SM. 2018. Turning microplastics into nanoplastics through digestive fragmentation by Antarctic krill. Nature Communications 9(1):1001. DOI: 10.1038/s41467-018-03465-9.
- Eerkes-Medrano D, Thompson RC, Aldridge DC. 2015. Microplastics in freshwater systems: A review of the emerging threats, identification of knowledge gaps and prioritisation of research needs. Water Research 75:63–82. DOI: 10.1016/j.watres.2015.02.012.
- Elizalde-Velázquez A, Carcano AM, Crago J, Green MJ, Shah SA, and Cañas-Carrell JE. 2020. Translocation, trophic transfer, accumulation and depuration of polystyrene microplastics in Daphnia magna and Pimephales promelas. Environmental Pollution 259:113937. DOI: 10.1016/j.envpol.2020.113937.
- Fotopoulou KN, Karapanagioti HK. 2012. Surface properties of beached plastic pellets. Marine Environmental Research 81:70–77. DOI: 10.1016/j.marenvres.2012.08.010.
- Franzellitti S, Canesi L, Auguste M, Wathsala RHGR, Fabbri E. 2019. Microplastic exposure and effects in aquatic organisms: A physiological perspective. Environmental Toxicology and Pharmacology 68:37–51. DOI: 10.1016/j.etap.2019.03.009.
- Frère L, Maignien L, Chalopin M, Huvet A, Rinnert E, Morrison H, Kerninon S, Cassone A-L, Lambert C, Reveillaud J, Paul-Pont I. 2018. Microplastic bacterial communities in the Bay of Brest: Influence of polymer type and size. Environmental Pollution 242:614–625. DOI: 10.1016/j.envpol.2018.07.023.
- Gigault J, ter Halle A, Baudrimont M, Pascal PY, Gauffre F, Phi TL, El Hadri H, Grassl B, Reynaud S. 2018. Current opinion: What is a nanoplastic? Environmental Pollution 235:1030–1034. DOI: 10.1016/j.envpol.2018.01.024.
- Goldschmidt-Clermont E, Wahli T, Frey J, Burr SE. 2008. Identification of bacteria from the normal flora of perch, Perca fluviatilis L., and evaluation of their inhibitory potential towards Aeromonas species. Journal of Fish Diseases 31(5):353–359. DOI: 10.1111/j.1365-2761.2008.00912.x.
- Hall-Stoodley L, Costerton JW, Stoodley P. 2004. Bacterial biofilms: From the natural environment to infectious diseases. Nature Reviews. Microbiology 2(2):95–108. DOI: 10.1038/nrmicro821.
- Harrison JP, Schratzberger M, Sapp M, Osborn AM. 2014. Rapid bacterial colonization of low-density polyethylene microplastics in coastal sediment microcosms. BMC Microbiology 14(1):232. DOI: 10.1186/s12866-014-0232-4.
- Hibbing ME, Fuqua C, Parsek MR, Peterson SB. 2009. Bacterial competition: Surviving and thriving in the microbial jungle. Nature Reviews. Microbiology [online] 8(1):15–25. DOI: 10.1038/nrmicro2259.
- Holmes RM, Aminot A, Kérouel R, Hooker BA, and Peterson BJ 1999. A simple and precise method for measuring ammonium in marine and freshwater ecosystems. Canadian Journal of Fisheries and Aquatic Sciences 56(10):1801–1808. DOI:10.1139/f99-128.
- Hori K, Matsumoto S. 2010. Bacterial adhesion: From mechanism to control. Biochemical Engineering Journal 48(3):424–434. DOI: 10.1016/j.bej.2009.11.014.
- Horsley RW. 1977. A review of the bacterial flora of teleosts and elasmobranchs, including methods for its analysis. Journal of Fish Biology 10(6):529–553. DOI: 10.1111/j.1095-8649.1977.tb04086.x.
- Hossain MR, Jiang M, Wei Q, Leff LG. 2019. Microplastic surface properties affect bacterial colonization in freshwater. Journal of Basic Microbiology 59(1):54–61. DOI: 10.1002/jobm.201800174.
- Hou L, McMahan CD, McNeish RE, Munno K, Rochman CM, Hoellein TJ. 2021. A fish tale: A century of museum specimens reveal increasing microplastic concentrations in freshwater fish. Ecological Applications 31(5):e02320. DOI: 10.1002/eap.2320.
- Huerta Lwanga E, Thapa B, Yang X, Gertsen H, Salánki T, Geissen V, Garbeva P. 2018. Decay of low-density polyethylene by bacteria extracted from earthworm’s guts: A potential for soil restoration. Science of the Total Environment 624:753–757. DOI: 10.1016/j.scitotenv.2017.12.144.
- Hwang J, Choi D, Han S, Jung SY, Choi J, Hong J. 2020. Potential toxicity of polystyrene microplastic particles. Scientific Reports 10(1):7391. DOI: 10.1038/s41598-020-64464-9.
- Jachner A. 1991. Food and habitat partitioning among juveniles of three fish species in the pelagial of a mesotrophic lake. Hydrobiologia 226(2):81–89. DOI: 10.1007/bf00006809.
- Jeppesen E, Sřndergaard M, Jensen JP, Mortensen E, Hansen AM, Jřrgensen T. 1998. Cascading trophic interactions from fish to bacteria and nutrients after reduced sewage loading: An 18-year study of a shallow hypertrophic lake. Ecosystems 1(3):250–267. DOI: 10.1007/s100219900020.
- Kaliszewicz A, Winczek M, Karaban K, Kurzydłowski D, Górska M, Koselak W, Romanowski J. 2020. The contamination of inland waters by microplastic fibres under different anthropogenic pressure: Preliminary study in Central Europe (Poland). Waste Management and Research: The Journal for a Sustainable Circular Economy 38(11):1231–1238. DOI: 10.1177/0734242X20938448.
- Kashinskaya EN, Simonov EP, Izvekova GI, Parshukov AN, Andree KB, Solovyev MM. 2019. Composition of the microbial communities in the gastrointestinal tract of perch (Perca fluviatilis L. 1758) and cestodes parasitizing the perch digestive tract. Journal of Fish Diseases 43(1):23–38. DOI: 10.1111/jfd.13096.
- Kenyon KW, Kridler E. 1969. Laysan albatrosses swallow indigestible matter. The Auk 86(2):339–343. DOI: 10.2307/4083505.
- Kerr A, Cowling MJ. 2003. The effects of surface topography on the accumulation of biofouling. Philosophical Magazine 83(24):2779–2795. DOI: 10.1080/1478643031000148451.
- Kitchell JF, Koonce JF, Tennis PS. 1975. Phosphorus flux through fishes. SIL Proc 19:2478–2484. DOI: 10.1080/03680770.1974.11896332.
- Klaine SJ, Koelmans AA, Horne N, Carley S, Handy RD, Kapustka L, Nowack B, von der Kammer F. 2011. Paradigms to assess the environmental impact of manufactured nanomaterials. Environmental Toxicology and Chemistry 31(1):3–14. DOI: 10.1002/etc.733.
- Lechthaler S, Waldschläger K, Stauch G, Schüttrumpf H. 2020. The way of macroplastic through the environment. Environments 7(10):73. DOI: 10.3390/environments7100073.
- Lenz R, Enders K, Nielsen TG. 2016. Microplastic exposure studies should be environmentally realistic. Proceedings of the National Academy of Sciences of United States of America 113(29):E4121–E4122. DOI: 10.1073/pnas.1606615113.
- Li J, Ni J, Li J, Wang C, Li X, Wu S, Zhang T, Yu Y, Yan Q. 2014. Comparative study on gastrointestinal microbiota of eight fish species with different feeding habits. Journal of Applied Microbiology 117(6):1750–1760. DOI: 10.1111/jam.12663.
- Lozano YM, Rillig MC. 2020. Effects of microplastic fibers and drought on plant communities. Environmental Science & Technology 54(10):6166–6173. DOI: 10.1021/acs.est.0c01051.
- Maszczyk P, Bartosiewicz M. 2012. Threat or treat: The role of fish exudates in the growth and life history of Daphnia. Ecosphere 3(10):1–19. DOI: 10.1890/ES12-00146.1.
- Maszczyk P, Kiersztyn B, Gozzo S, Kowalczyk G, Jimenez-Lamana J, Szpunar J, Pijanowska J, Jines-Muñoz C, Zebrowski ML, Babkiewicz E. 2022b. Combined effects of polystyrene nanoplastics and enrofloxacin on the life histories and gut microbiota of Daphnia magna. Water 14(21):3403. DOI: 10.3390/w14213403.
- Maszczyk P, Pijanowska J, Mrówka P, Babkiewicz E. 2022a. Effects of nanoplastics on aquatic organisms. In: Szpunar J, Jiménez-Lamana J, editors. Environmental nanopollutants: Sources, occurrence, analysis and fate. Royal Society of Chemistry Press. pp. 156–210. DOI: 10.1039/9781839166570-00156.
- Matjašič T, Simčič T, Medvešček N, Bajt O, Dreo T, Mori N. 2021. Critical evaluation of biodegradation studies on synthetic plastics through a systematic literature review. Science of the Total Environment 752:141959. DOI: 10.1016/j.scitotenv.2020.141959.
- Miao L, Wang P, Hou J, Yao Y, Liu Z, Liu S, Li T. 2019. Distinct community structure and microbial functions of biofilms colonizing microplastics. Science of the Total Environment 650:2395–2402. DOI: 10.1016/j.scitotenv.2018.09.378.
- Molina V, Fernández C. 2020. Bacterioplankton response to nitrogen and dissolved organic matter produced from salmon mucus. Microbiology Open 9(12):e1132. DOI: 10.1002/mbo3.1132.
- Moore CJ. 2008. Synthetic polymers in the marine environment: A rapidly increasing, long-term threat. Environmental Research 108(2):131–139. DOI: 10.1016/j.envres.2008.07.025.
- Moses O, Nemcovsky CE, Lewinstein I, Zoabi H, Weinreb M, Levartovsky S, Matalon S. 2020. In vitro preliminary evaluation of bacterial attachment on grooved and smooth healing abutments. Applied Sciences 10(13):4426. DOI: 10.3390/app10134426.
- Murphy L, Germaine K, Dowling DN, Kakouli-Duarte T, Cleary J. 2020. Association of potential human pathogens with microplastics in freshwater systems. In: Cocca M, ed., Proceedings of the 2nd International Conference on Microplastic Pollution in the Mediterranean Sea, ICMPMS 2019. Springer Water. Springer, Cham. DOI: 10.1007/978-3-030-45909-3_19.
- Nagul EA, McKelvie ID, Worsfold P, Kolev SD. 2015. The molybdenum blue reaction for the determination of orthophosphate revisited: Opening the black box. Analytica Chimica Acta [online] 890:60–82. DOI: 10.1016/j.aca.2015.07.030.
- Ng KL, Obbard JP. 2006. Prevalence of microplastics in Singapore’s coastal marine environment. Marine Pollution Bulletin 52(7):761–767. DOI: 10.1016/j.marpolbul.2005.11.017.
- Ockenden A, Tremblay LA, Dikareva N, Simon KS. 2021. Towards more ecologically relevant investigations of the impacts of microplastic pollution in freshwater ecosystems. Science of the Total Environment 792:148507. DOI: 10.1016/j.scitotenv.2021.148507.
- Ogonowski M, Motiei A, Ininbergs K, Hell E, Gerdes Z, Udekwu KI, Bacsik Z, Gorokhova E. 2018. Evidence for selective bacterial community structuring on microplastics. Environmental Microbiology 20(8):2796–2808. DOI: 10.1111/1462-2920.14120.
- Parrish K, Fahrenfeld NL. 2019. Microplastic biofilm in fresh- and wastewater as a function of microparticle type and size class. Environmental Science: Water Research and Technology 5:495–505. DOI: 10.1039/C8EW00712H.
- Peng X, Chen M, Chen S, Dasgupta S, Xu H, Ta K, Du M, Li J, Guo Z, Bai S. 2018. Microplastics contaminate the deepest part of the world’s ocean. Geochemical Perspectives Letters 9:1–5. DOI: 10.7185/geochemlet.1829.
- Plastics Europe, and EPRO. 2021. Plastics the Facts 2021 • Plastics Europe. [online] Plastics Europe. Available: https://plasticseurope.org/knowledge-hub/plastics-the-facts-2021/. Accessed Dec 2020.
- Porter KG, Feig YS. 1980. The use of DAPI for identifying and counting aquatic microflora. Limnology and Oceanography 25(5):943–948. DOI: 10.4319/lo.1980.25.5.0943.
- Prinz N, Korez Š. 2020. Understanding how microplastics affect marine biota on the cellular level is important for assessing ecosystem function: a review. In YOUMARES 9-The Oceans: Our Research, Our Future: Proceedings of the 2018 conference for YOUng MArine RESearcher in Oldenburg, Germany. Springer International Publishing. pp. 101–120. DOI: 10.1007/978-3-030-20389-4_6.
- Pukos S, Maszczyk P, Dąbrowski K, Zebrowski ML, Babkiewicz E. 2023. The effect of planktivorous fish on the vertical flux of polystyrene microplastics. European Zoological Journal.
- Ragusa A, Svelato A, Santacroce C, Catalano P, Notarstefano V, Carnevali O, Papa F, Rongioletti MCA, Baiocco F, Draghi S, D’amore E, Rinaldo D, Matta M, Giorgini E. 2021. Plasticenta: First evidence of microplastics in human placenta. Environment International [online] 146:106274. DOI: 10.1016/j.envint.2020.106274.
- Rosato A, Barone M, Negroni A, Brigidi P, Fava F, Xu P, Candela M, Zanaroli G. 2020. Microbial colonization of different microplastic types and biotransformation of sorbed PCBs by a marine anaerobic bacterial community. Science of the Total Environment 705:135790. DOI: 10.1016/j.scitotenv.2019.135790.
- Rummel CD, Jahnke A, Gorokhova E, Kühnel D, Schmitt-Jansen M. 2017. Impacts of biofilm formation on the fate and potential effects of microplastic in the aquatic environment. Environmental Science and Technology Letters 4(7):258–267. DOI: 10.1021/acs.estlett.7b00164.
- Schaus MH, Vanni MJ. 2000. Effects of gizzard shad on phytoplankton and nutrient dynamics: Role of sediment feeding and fish size. Ecology 81(6):1701–1719. DOI: 10.2307/177318.
- Schindelin J, Arganda-Carreras I, Frise E, Kaynig V, Longair M, Pietzsch T, Preibisch S, Reuden C, Saalfed SB, Tineves J-Y, White JD, Hartenstein V, Eliceiri K, Tomancak PS, Cardona A. 2012. Fiji: An open-source platform for biological-image analysis. Nature Methods 9(7):676–682. DOI: 10.1038/nmeth.2019.
- Schrank I, Trotter B, Dummert J, Scholz-Böttcher BM, Löder MGJ, Laforsch C. 2019. Effects of microplastic particles and leaching additive on the life history and morphology of Daphnia magna. Environmental Pollution [online] 255:113233. DOI: 10.1016/j.envpol.2019.113233.
- Shruti VC, Pérez-Guevara F, Elizalde-Martínez I, Kutralam-Muniasamy G. 2020. First study of its kind on the microplastic contamination of soft drinks, cold tea and energy drinks – Future research and environmental considerations. Science of the Total Environment 726:138580. DOI: 10.1016/j.scitotenv.2020.138580.
- Singh B, Sharma N. 2008. Mechanistic implications of plastic degradation. Polymer Degradation and Stability 93(3):561–584. DOI: 10.1016/j.polymdegradstab.2007.11.008.
- So MWK, Vorsatz LD, Cannicci S, Not C. 2022. Fate of plastic in the environment: From macro to nano by macrofauna. Environmental Pollution 300:118920. DOI: 10.1016/j.envpol.2022.118920.
- Stanton T, Johnson M, Nathanail P, MacNaughtan W, Gomes RL. 2020. Freshwater microplastic concentrations vary through both space and time. Environmental Pollution 263(Pt B):114481. DOI: 10.1016/j.envpol.2020.
- Sun Y, Duan C, Cao N, Li X, Li X, Chen Y, Huang Y, Wang J. 2022. Effects of microplastics on soil microbiome: The impacts of polymer type, shape, and concentration. Science of the Total Environment 806:150516. DOI: 10.1016/j.scitotenv.2021.150516.
- Sun R, Xu K, Yu L, Pu Y, Xiong F, He Y, Huang Q, Tang M, Chen M, Yin L, Zhang J, Pu Y. 2021. Preliminary study on impacts of polystyrene microplastics on the hematological system and gene expression in bone marrow cells of mice. Ecotoxicology and Environmental Safety 218:112296. DOI: 10.1016/j.ecoenv.2021.112296.
- Tang L, Su C, Chen Y, Xian Y, Hui X, Ye Z, Chen M, Zhu F, Zhong H. 2021. Influence of biodegradable polybutylene succinate and non-biodegradable polyvinyl chloride microplastics on anammox sludge: Performance evaluation, suppression effect and metagenomic analysis. Journal of Hazardous Materials 401:123337. DOI: 10.1016/j.jhazmat.2020.123337.
- Thompson RC. 2004. Lost at sea: Where is all the plastic? Science 304(5672):838. DOI: 10.1126/science.1094559.
- Threlkeld ST. 1987. Experimental evaluation of trophic-cascade and nutrient-mediated effects of planktivorous fish on plankton community structure. In: Kerfoot WC, Sih A, editors. Predation: Direct and indirect impacts on aquatic communities. Hanover, NH: New England Univ. Press. pp. 161–173. DOI: 10.1126/science.238.4823.93.
- Tu C, Liu Y, Li L, Li Y, Vogts A, Luo Y, Waniek JJ. 2021. Structural and functional characteristics of microplastic associated biofilms in response to temporal dynamics and polymer types. Bulletin of Environmental Contamination and Toxicology 107(4):633–639. DOI: 10.1007/s00128-021-03333-1.
- Vanni MJ, Findlay DL. 1990. Trophic cascades and phytoplankton community structure. Ecology 71(3):921–937. DOI: 10.2307/1937363.
- Van Wijnen J, Ragas AMJ, Kroeze C. 2019. Modelling global river export of microplastics to the marine environment: Sources and future trends. Science of the Total Environment 673:392–401. DOI: 10.1016/j.scitotenv.2019.04.078.
- Venkatesh S, Mahboob S, Govindarajan M, Al-Ghanim KA, Ahmed Z, Al-Mulhm N, Gayathri R, Vijayalakshmi S. 2021. Microbial degradation of plastics: Sustainable approach to tackling environmental threats facing big cities of the future. Journal of King Saud University – Science 33(3):101362. DOI: 10.1016/j.jksus.2021.101362.
- Verant ML, Konsti ML, Zimmer KD, Deans CA. 2007. Factors influencing nitrogen and phosphorus excretion rates of fish in a shallow lake. Freshwater Biology 52(10):1968–1981. DOI: 10.1111/j.1365-2427.2007.01820.x.
- Villéger S, Fouilland E, Argenty J, Bouvier C, Carré C, Bouvier T. 2019. Interspecific differences in the effect of fish on marine microbial plankton. Aquatic Microbial Ecology 82(3):289–298. DOI: 10.3354/ame01897.
- Widyananto PA, Muchlissin SI, Sabdono A, Yulianto B, Hamid FS, Radjasa OK. 2021. Biodegradation of polyethylene microplastic using culturable coral-associated bacteria isolated from corals of Karimunjawa National Park. ILMU KELAUTAN: Indonesian Journal of Marine Sciences 26(4):237–246. DOI: 10.14710/ik.ijms.26.4.237-246.
- Wilson B, Danilowicz BS, Meijer WG. 2007. The diversity of bacterial communities associated with Atlantic Cod Gadus morhua. Microbial Ecology 55(3):425–434. DOI: 10.1007/s00248-007-9288-0.
- Wu WM, Yang J, Criddle CS. 2016. Microplastics pollution and reduction strategies. Frontiers of Environmental Science and Engineering [online] 11(1). DOI: 10.1007/s11783-017-0897-7.
- Xu P, Peng G, Su L, Gao Y, Gao L, Li D. 2018. Microplastic risk assessment in surface waters: A case study in the Changjiang Estuary, China. Marine Pollution Bulletin 133:647–654. DOI: 10.1016/j.marpolbul.2018.06.020.
- Yang Y, Liu W, Zhang Z, Grossart HP, Gadd GM. 2020. Microplastics provide new microbial niches in aquatic environments. Applied Microbiology and Biotechnology 104(15):6501–6511. DOI: 10.1007/s00253-020-10704-x.
- Yin L, Liu H, Cui H, Chen B, Li L, Wu F. 2019. Impacts of polystyrene microplastics on the behavior and metabolism in a marine demersal teleost, black rockfish (Sebastes schlegelii). Journal of Hazardous Materials 380:120861. DOI: 10.1016/j.jhazmat.2019.120861.
- Yoshida S, Hiraga K, Takehana T, Taniguchi I, Yamaji H, Maeda Y, Toyohara K, Miyamoto K, Kimura Y, Oda K. 2016. A bacterium that degrades and assimilates poly(ethylene terephthalate). Science 351(6278):1196–1199. DOI: 10.1126/science.aad6359.
- Zebrowski ML, Babkiewicz E, Błażejewska A, Pukos S, Wawrzeńczak J, Wilczynski W, Zebrowski J, Ślusarczyk M, Maszczyk P. 2022. The effect of microplastics on the interspecific competition of Daphnia. Environmental Pollution 313:120121. DOI: 10.1016/j.envpol.2022.120121.
- Zettler ER, Mincer TJ, Amaral-Zettler LA. 2013. Life in the “plastisphere”: Microbial communities on plastic marine debris. Environmental Science & Technology 47(13):7137–7146. DOI: 10.1021/es401288x.
- Zha Y, Eiler A, Johansson F, Svanbäck R. 2018. Effects of predation stress and food ration on perch gut microbiota. Microbiome 6(1). DOI: 10.1186/s40168-018-0400-0.
- Zheng S, Wang WX. 2022. Disturbing ion regulation and excretion in medaka (Oryzias melastigma) gills by microplastics: Insights from the gut-gill axis. Science of the Total Environment 159353. DOI: 10.1016/j.scitotenv.2022.159353.
- Zhou G, Wang Q, Li J, Li Q, Xu H, Ye Q, Wang Y, Shu S, Zhang J. 2021. Removal of polystyrene and polyethylene microplastics using PAC and FeCl3 coagulation: Performance and mechanism. Science of the Total Environment 752:141837. DOI: 10.1016/j.scitotenv.2020.141837.
- Zimmer KD, Herwig BR, Laurich LM. 2006. Nutrient excretion by fish in wetland ecosystems and its potential to support algal production. Limnology and Oceanography 51(1):197–207. DOI: 10.4319/lo.2006.51.1.0197.