Abstract
The purpose of this study was to evaluate the sensitivity of hemocytes of adult Steatoda grossa females to cadmium and copper administered orally for 4 weeks or 12 months, and to check the metabolic condition of these cells after immunostimulation with phorbol 12-myristate 13-acetate (PMA). The viability of hemocytes (flow cytometry and luminescence techniques), their ultrastructure (transmission electron microscopy), and the antioxidant activity of hemolymph (superoxide dismutase, catalase, total antioxidant capacity; TAC) were analyzed. The results showed that, compared to copper, cadmium caused severe necrotic changes in hemocytes and impaired oxygen burst reactions and antioxidant responses, regardless of the exposure time. Copper induced degenerative changes only during short-term exposure, but its long-term intoxication did not impair the metabolic processes of hemocytes. Administration of PMA to spiders that were chronically exposed to either of the metals caused an increase in TAC levels in the hemolymph. Although the concentration of ATP in hemocytes was reduced relative to the control, the ADP/ATP ratio did not change, precluding a strong depletion of cellular energy resources. The metabolic condition of hemocytes stabilized with prolonged metal exposure, indicating the activation of defense mechanisms under operating stressors.
Highlights
Cadmium impaired oxygen burst reactions and antioxidant responses in hemocytes of spiders.
Copper induced degenerative changes in hemocytes only during short-term exposure.
Chronic intoxication with metals not impair energetic processes in hemocytes of spiders.
1. Introduction
Although several ecotoxicological studies have focused on the cellular effects of metal contamination in insects, the mechanisms underlying tolerance to high metal concentrations in spiders remain poorly understood (Chen et al. Citation2011). There is a lack of information on the type and effectiveness of immune responses in these predators under the condition of simultaneous exposure to metal pollution and infection. In an environment contaminated with heavy metals, the occurrence and survival of spiders as obligatory predators and macroconcentrators of metals depend on their ability to tolerate an excess of xenobiotics entering the organisms, mainly through the diet. In such conditions, the organisms’ survival and efficient functioning are both dependent on the immune system status. Similar to other animals, in spiders the immune response resulting from pathogen invasion is based on the mechanisms of cellular and humoral immunity, with hemocytes playing a critical role (Fukuzawa et al. Citation2008). In particular, granulocytes and plasmatocytes act as key players in immune responses in spiders and other arthropods (Ribeiro & Brehélin Citation2006). This is related to, inter alia, the involvement of these cells in processes such as phagocytosis, encapsulation, nodulation, and production of antibacterial proteins (Fukuzawa et al. Citation2008; Foelix Citation2011; Riciluca et al. Citation2012; Kuhn–Nentwig & Nentwig Citation2013; Kuhn–Nentwig et al. Citation2014). The interaction of hemocytes with bacteria or the changes in the internal environment of the organism leads to almost immediate release of antibacterial agents, such as reactive oxygen and nitrogen species, and antibacterial proteins to the hemolymph (Baumann et al. Citation2010). Reactive oxygen species are produced during the reaction associated with respiratory burst, which both in vertebrates and invertebrates is one of the basic mechanisms to combat infection. Similarly, nitric oxide which stimulates the immune response and exhibits antibacterial properties is produced in an organism in response to microbial invasion (Homa et al. Citation2013). Previous studies on female Steatoda grossa spiders confirmed the sensitivity of hemocytes following the administration of a synthetic immune stimulator (phorbol myristate acetate) and bacterial suspensions, which resulted in changes in the proportion of different types of hemocytes and enhancement of degenerative processes in the cells (Wiśniewska et al. Citation2022). Additionally, changes in the proteome of hemolymph were recorded in these conditions (Wiśniewska et al. Citation2023). Physiological and biochemical defense reactions triggered in an organism usually lead to changes in the cellular concentrations of adenine nucleotides (ATP, ADP, AMP). These metabolites are the most universal enzymatic effectors, with ATP being a positive effector of the enzymes regulating anabolic pathways and AMP activating the regulatory enzymes of catabolic pathways. The ratio of intracellular ADP and ATP concentrations can serve as a biomarker of the cellular energetic status, allowing predictions of future events in cells, such as proliferation or cell death. Changes in ATP concentrations following metal exposition were confirmed in spiders as well as in laboratory in a field study (Wilczek Citation1996; Wilczek et al. Citation2018). Similarly, changes in enzymatic and nonenzymatic antioxidant parameters were observed in individuals exposed to natural and anthropogenic stress factors (Stalmach et al. Citation2015a).
This study aimed to compare the sensitivity of hemocytes in S. grossa spiders, serving as the test species, to sublethal doses of the xenobiotic cadmium and biogenic copper present in their feed. The comparison was based on the duration of intoxication, distinguishing between short-term and long-term exposure. Additionally, the study analyzed the hemocytes’ ability to react through a respiratory burst when exposed to heavy metal-contaminated food after receiving an administration of a synthetic immunostimulant known as phorbol myristate acetate (PMA). This administration aimed to simulate infection conditions. PMA is known to activate protein kinase C and participate in signal transduction through cellular membrane receptors. It can also stimulate immune cells to undergo oxygen burst reactions upon contact with microbes (Roy et al. Citation1995; Nomura et al. Citation2007). Given the high prooxidative activity of the applied factors, this study analyzed the levels of selected enzymatic and nonenzymatic antioxidants in the spiders’ hemolymph. Furthermore, an assessment of the ultrastructure of the hemocytes was conducted, primarily focusing on identifying any apoptotic, necrotic, or autophagic processes. These processes serve as potential indicators of the effects resulting from individual or mutual interactions between the metals and the immunostimulant used.
2. Materials and methods
2.1. Characteristics of experimental groups
The study was performed on the hemolymph of adult female S. grossa spiders, as model species for obligatory carnivorous arthropods. As a cosmopolitan and synanthropic species, S. grossa is commonly found in the basements and attics of buildings, and different sheltered places. The individuals used in the study were from a multigeneration experimental colony maintained in laboratory conditions as described in previous studies (Stalmach et al. Citation2015a, Citation2015b; Wilczek et al. Citation2017, Citation2018, Citation2019). These were divided into three main groups: a control group (CT) was fed with Drosophila hydei flies, bred on unpolluted standard medium (Wilczek et al. Citation2017), and two experimental groups, Cu_int and Cd_int, receiving food contaminated with metals. Metal-exposed individuals were fed D. hydei flies, which were grown on a standard medium supplemented with CdCl2 (0.25 mM; Cd_int) or CuSO4 (0.234 mM; Cu_int) in sublethal concentrations for spiders and their preys. All the examined individuals were exposed to metals for 4 weeks (short-term intoxication) or 12 months (long-term intoxication). During this period, the individuals were fed two or three flies on average, reared on a given medium (control or contaminated with metals), every 2 days. The method of metal administration to S. grossa spiders simulated to the natural exposure of these obligatory predators to metals because they are absorbed mainly via the digestive tract. Regardless of the experimental groups, the mortality of specimens did not exceed 1%.
Within each of the main groups, a part of the individuals were immunostimulated with phorbol 12-myristate 13-acetate (PMA; Sigma-Aldrich Co., St. Louis, MO, USA). Finally, 12 experimental groups were distinguished: a control nonimmunostimulated group receiving uncontaminated food (CT); control immunostimulated group receiving uncontaminated food (CT_PMA); Cu- or Cd-intoxicated (both short- and long term) and nonimmunostimulated group (Cd_int/Cu_int); and Cd- or Cu-intoxicated (both short- and long term) and immunostimulated group (Cd_PMA/Cu_PMA).
At the appropriate time of the experiment, individuals were anesthetized on ice, and 0.5 µl solution containing 0.1 µg·ml−1 of PMA was injected by a puncture in the back part of the opisthosoma. Previous studies have proven that at the mentioned dose PMA can cause quantitative and qualitative changes in the hemocyte subpopulations of adult S. grossa females (Wiśniewska et al. Citation2022, Citation2023). After 24 h of the injection of PMA, the hemolymph was collected from the spiders (one individual per sample; n = 5–6 per each group). For this, the spiders were anesthetized again on ice, and a sample of hemolymph (average 5 µl per individual) was obtained by a puncture to the edge of the leg attached to the prosoma. Immediately after collection, the hemolymph samples were suspended in phosphate-buffered saline and analyzed according to cytometric, spectrophotometric, and luminescence procedures. For microscopic analyses, prosomas and opisthosomas were isolated. A total of 100 female spiders were used for all analyses.
2.2. Light and transmission electron microscopy
The prosomas and opisthosomas of spiders from all experimental groups (sample; n = 3 per each group) were fixed with 2.5% glutaraldehyde in a 0.1 M sodium phosphate buffer (made of Na2HPO4·7H2O and NaH2PO4∙H2O) (pH 7.4, 4°C, 2 h) and postfixed in 2% osmium tetroxide in a 0.1 M phosphate buffer (4°C, 1.5 h). Then, tissues were dehydrated, embedded, and cut as described by Rost–Roszkowska et al. (Citation2020). Semi–thin sections were stained with 1% methylene blue in 0.5% borax and observed using an Olympus BX60 light microscope. Ultrathin sections were stained with uranyl acetate and lead citrate and analyzed using a Hitachi H500 transmission electron microscope at 75 kV.
2.3. LysoTracker Red staining: labeling autophagosomes and autolysosomes
LysoTracker Red staining is commonly used to investigate strongly acidic organelles such as lysosomes and autolysosomes, where the dye selectively accumulates. Briefly, the body fragments of females from all experimental groups without fixation were incubated in 2.5 mmol/L LysoTracker Red DND-99 (Molecular Probes, L 7528; Thermo Fisher Scientific, Waltham, MA, USA) and 1 mg/ml DAPI (4′,6-diamidine-2′-phenylindole dihydrochloride) (Sigma-Aldrich) as described by Rost–Roszkowska et al. (Citation2020) The slides were analyzed using an Olympus FluoView FV1000 confocal microscope with a 559 nm laser for LysoTracker Red dye and 405 nm laser for the DAPI dye.
2.4. Flow cytometric assessment of viability of hemocytes
The quantitative measure of cell viability in the hemolymph of spiders was determined by the flow cytometric technique using the Annexin V-FITC (fluorescein isothiocyanate) apoptosis detection kit (Abcam®, № ab14085). Simultaneously, using Annexin V-FITC (green fluorescence) and PI (propidium iodide; red fluorescence), apoptotic and necrotic cells were distinguished (viable cells: Annexin V-FITC–/PI–; early apoptotic cells: Annexin V-FITC+/PI–; late apoptotic cells: Annexin V-FITC+/PI+; necrotic cells: Annexin V-FITC–/PI+). According to the manufacturer’s recommendations and our modifications, 5 µl of Annexin V-FITC and 2.5 µl of PI were added to 100 μl of a previously prepared hemocyte suspension. The solution was incubated for 15 min in darkness at room temperature, and then each sample was added with 100 μl of PBS. The cell suspensions were analyzed in the flow cytometer Beckman Coulter CytoFLEX using CytExpert Acquisition and Analysis Software (Beckman Coulter, United States). Light emission was induced in 5,000 cells from each experimental sample.
2.5. ATP level and ADP/ATP ratio
The ATP level and ADP/ATP ratio were determined using the ApoSENSOR™ ATP Cell Viability Bioluminescence Assay Kit (BioVision, № K254) and ApoSENSOR ADP/ATP Ratio Bioluminescence Assay Kit (BioVision, № K255), according to the manufacturer’s protocols. The absolute level of ATP in the samples was calculated by generating an ATP standard curve (using the ATP standard provided in the kit). The results were expressed as nmol ATP ∙ mg–1 protein.
2.6. Antioxidative enzymes
2.6.1. Superoxidase dismutase (SOD; EC 15.1.1)
The SOD activity was measured in the hemolymph of spiders by performing Creative Biolabs’ SOD assay, according to the manufacturer’s protocol, using the colorimetric technique. SOD is an enzyme that catalyzes the dismutation of superoxide into O2 and H2O2. In the assay, superoxide (O2●) is provided by a xanthine oxidase-catalyzed reaction. This superoxide reacts with the WST-1 dye to form a colored product. SOD scavenges O2●, and as a result less superoxide is available for the chromogenic reaction. The SOD activity in the sample is determined based on the color intensity (OD440nm). The activity of SOD in the samples was calculated by generating a standard curve t.t.OD vs [SOD] (U/ml). The results were expressed as U SOD mg–1 protein.
2.6.2. Catalase (CAT; EC 1.11.1.6)
CAT was determined, as described by to Orr (Citation1970), as a decrease in absorbance at 25°C (thermal coefficient Q10 = 1.1) for 30s at 230 nm (ε = 40.0 ODU ∙ M–1 × cm–1) in a 50 µl solution of 0.05 mM Na2HPO4–KH2PO4 buffer (pH 7.4) containing 10 mM H2O2 (approximately 30%, Sigma). The reaction was initiated by adding 100 µl of the sample. Each sample was prepared by diluting 5 µl hemolymph in 496 µl of 0.05 mM Na2HPO4–KH2PO4 buffer (pH 7.4). The decomposition of H2O2 was monitored spectrophotometrically, and the results were expressed as mmol CAT ∙ mg–1 protein.
2.6.3. Total antioxidant capacity (TAC)
TAC was measured, as described by Re et al. (Citation1999), based on the reduction of ABTS+● (2,2′-azino-bis (3-ethylbenzothiazoline-6-sulfonic acid) diammonium salt) radical cation produced from ABTS. The radical-scavenging reaction was initiated by adding 10 µl of the homogenate to 90 µl of the ABTS+● solution. The absorbance of the mixture was recorded at 734 nm. The change in absorbance was related to the absorbance changes of a standard solution of Trolox (97% pure, Sigma-Aldrich; ±6-hydroxy-2,5,7,8-tetramethylchromane-2-carboxylic acid, a water-soluble analog of vitamin E). The antioxidant activity of the samples was investigated using the Trolox-equivalent antioxidant capacity test. The results were expressed as µM Trolox equivalent mg–1 protein.
Protein content was measured using Bradford’s method (Citation1976), with bovine serum albumin (protein content > 95%, Fluka) as the standard. Samples were determined spectrophotometrically at 495 nm.
2.7. Statistical analyses
Statistical analyses were performed with STATISTICA 13.1 (StatSoft Inc., Poland). Normality was checked using the Shapiro–Wilk test. The results were expressed as median and quartile deviation (25th and 75th percentiles). The Mann–Whitney U test (P < 0.05) was used to evaluate the significance of differences between the experimental groups in terms of the use of immunostimulant (NS/PMA), type of metals (CT vs Cd/Cu), and period of exposure to metals (short- or long term). The significance of differences between the experimental groups in the levels of the examined parameters considering metal exposure, time exposition on metals, and immunostimulation (PMA) was assessed using the analysis of variance (ANOVA/MANOVA; p < 0.05).
3. Results
3.1. Qualitative assessment of degenerative changes in hemocytes
Light microscopy allowed the observation of prohemocytes, plasmatocytes, and granulocytes in the hemolymph and between organs, such as muscles and the digestive tract, including midgut glands, as shown in our previous studies (Wilczek et al. Citation2018). Because most of the hemocytes were granulocytes, our study focused on this group of cells. This type of hemocytes were more abundant in the PMA-injected groups (C_PMA, Cd_PMA, and Cu_PMA) ()).
Figure 1. Localization of granulocytes in the hemocoel of Steatoda grossa during the short-term experiment. (a) Control animals (CT). Scale bar = 12 µm. (b) CT_PMA experimental group. Scale bar = 10 µm. (c) Cd experimental group. Scale bar = 12 µm. (d) Cd_PMA experimental group. Scale bar = 10 µm. (e) Cu experimental group. Scale bar = 13 µm. (f) Cu_PMA experimental group. Scale bar = 10 µm. Hemolymph (h), prohemocytes (1), plasmatocytes (2), granulocytes (3). Light microscope.
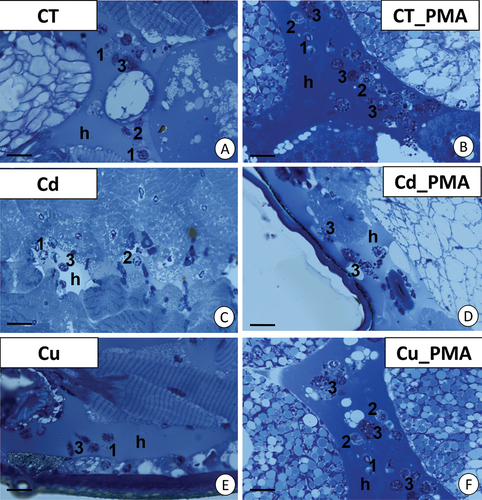
3.1.1. Control animals—the fine structure of granulocytes
The ultrastructure of hemocytes in the individuals from the control group has been described in detail in our previous papers (Wilczek et al. Citation2018; Wiśniewska et al. Citation2022). In these individuals, oval-shaped granulocytes possessed a lobular nucleus with electron-dense patches of heterochromatin. The entire cytoplasm was filled with homogenous and heterogenous granules, possessing material of different electron densities, along with the majority of mitochondria and glycogen granules. There were also small vesicles with electron-lucent content and cisterns of the endoplasmic reticulum ()).
Figure 2. TEM images of granulocytes of adult specimens of Steatoda grossa from the control experimental groups: CT (a, b) and CT_PMA (c–e). Nuclei (n), granules of different electron densities (g), mitochondria (black arrows), glycogen granules (black circles), vacuoles (v), cisterns of the endoplasmic reticulum (ER). TEM. (a) Scale bar = 1.3 µm. (b) Scale bar = 1.2 µm. (c) Scale bar = 1.4 µm. (d) Scale bar = 1.2 µm. (e) Scale bar = 1.2 µm.
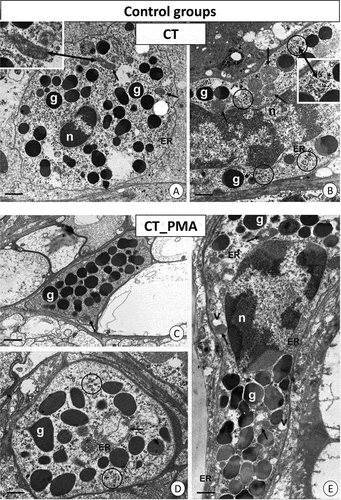
The cytoplasm of granulocytes in the individuals from the C_PMA group showed a distinct increase in the number of electron-dense homogenous granules and cisterns of the endoplasmic reticulum as well as small vacuoles with electron-lucent content (). However, there was a decrease in the electron density of granules. Glycogen granules were still found. More necrotic granulocytes with electron-lucent cytoplasm containing fewer distended cell organelles were observed (not shown).
3.1.2. Short-term experiment—the fine structure of granulocytes
The cytoplasm of most granulocytes in the individuals from the Cd_–int group showed a decrease in the number of organelles, including mitochondria or cisterns of the endoplasmic reticulum and granules with electron-dense content. No reduction in the number of mitochondrial cristae or changes in matrix electron density was observed. Glycogen granules were not detected, while vacuoles with electron-lucent content were noted (). Majority of granulocytes showed necrotic changes. Their cytoplasm was electron-lucent with a few degenerated organelles, such as cisterns of the endoplasmic reticulum and mitochondria, but there were no homogenous or heterogenous granules ().
Figure 3. Short-term experiment. TEM images of granulocytes of adult specimens of Steatoda grossa from the Cd (a–d) and Cd_PMA (e–g) experimental groups. Nuclei (n), granules of different electron densities (g), mitochondria (black arrows), vacuoles (v), cisterns of the endoplasmic reticulum (ER). TEM. (a) Scale bar = 1.3 µm. (b) Scale bar = 1.4 µm. (c) Scale bar = 1.6 µm. (d) Scale bar = 1.3 µm. (e) Scale bar = 1.2 µm. (f) Scale bar = 1.4 µm. (g) Scale bar = 1.6 µm.

After the injection of PMA, electron-dense and homogenous granules were not observed throughout the cytoplasm of granulocytes in the Cd_PMA group but these were concentrated in the vicinity of the cell membrane; in contrast, the perinuclear cytoplasm was devoid of these granules. The lobular nucleus possessed large electron-dense patches of heterochromatin. Mitochondria were correctly structured in the Cd_PMA group compared to the Cd experimental group. Single vacuoles with an electron-lucent interior and cisterns of the endoplasmic reticulum were observed in the cytoplasm. Glycogen granules were absent (). There were also necrotic granulocytes with electron-lucent cytoplasm possessing fewer organelles. These contained only single granules characteristic of granulocytes and degenerated mitochondria ().
Unlike the cadmium-intoxicated groups, the granulocytes in the individuals from the Cu_int group did not show any differences compared to the control group (C) (). After the injection of PMA, lobular-shaped nuclei of granulocytes showed more highly condensed heterochromatin than the granulocytes observed in the individuals from groups C and Cu. No changes in the ultrastructure of the remaining organelles were observed ().
Figure 4. Short-term experiment. TEM images of granulocytes of adult specimens of Steatoda grossa from the Cu (a, b) and Cu_PMA (c, d) experimental groups. Nuclei (n), granules of different electron densities (g), mitochondria (black arrows), glycogen granules (black circles), vacuoles (v), cisterns of the endoplasmic reticulum (ER). TEM. (a) Scale bar = 1.4 µm. (b) Scale bar = 1.2 µm. (c) Scale bar = 1.2 µm. (d) Scale bar = 1.4 µm.
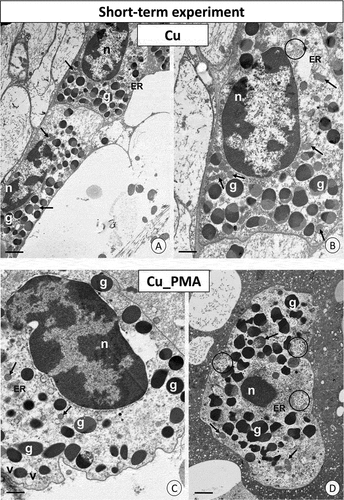
3.1.3. Long–term experiment—the fine structure of granulocytes
Following long-term cadmium intoxication, the hemocytes’ cytoplasm displayed degenerated cellular organelles. Notably, single vacuoles with an electron-lucent interior were observed. In contrast to individuals exposed to cadmium for a short duration, those from the long-term intoxicated group exhibited isolated instances and small clusters of glycogen granules (). Additionally, necrotic cells with cytoplasm lacking organelles were present. In the Cd_PMA group, after chronic intoxication, organelles such as mitochondria and cisterns of the endoplasmic reticulum maintained their proper structure, resembling those observed in the short-term Cd_PMA group. All cell organelles appeared normal when compared to the control group C. However, the cytoplasm of hemocytes still exhibited a relatively lower presence of organelles compared to the short-term Cd_PMA group. Single and small clusters of glycogen granules were visible (). Additionally, some necrotic cells were noted. Unlike the cadmium groups, the granulocytes of individuals exposed to long-term consumption of copper-contaminated food exhibited no alterations in cell organelles. However, the cytoplasm remained deficient in organelles. Although most organelles displayed the correct structure, the majority of mitochondria still lacked cristae and featured an electron-lucent matrix (). Regarding individuals from the long-term Cu_PMA experimental group, granulocytes exhibited unchanged ultrastructure (). However, sporadic necrotic hemocytes with electron-lucent cytoplasm poor in organelles were also observed ().
Figure 5. Long-term experiment. TEM images of granulocytes of adult specimens of Steatoda grossa from the Cd (a, b) and Cd_PMA (c, d) experimental groups. Nuclei (n), granules of different electron densities (g), mitochondria (black arrows), vacuoles (v), cisterns of the endoplasmic reticulum (ER), and glycogen granules (black circles). TEM. (a) Scale bar = 1.5 µm. (b) Scale bar = 1.2 µm. (c) Scale bar = 1.2 µm. (d) Scale bar = 0.8 µm.
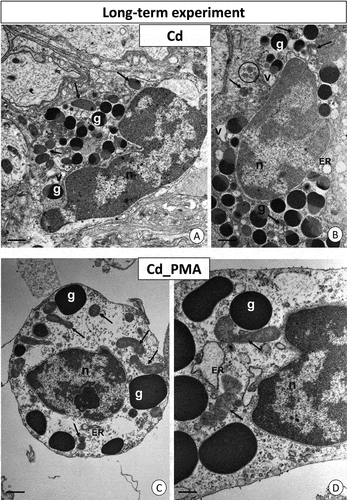
Figure 6. Long-term experiment. TEM images of granulocytes of adult specimens of Steatoda grossa from the Cu (a, b) and Cu_PMA (c, d) experimental groups. Nuclei (n), granules of different electron densities (g), mitochondria (black arrows), cisterns of the endoplasmic reticulum (ER). TEM. (a) Scale bar = 1.2 µm. (b) Scale bar = 0.9 µm. (c) Scale bar = 1.2 µm. (d) Scale bar = 0.8 µm.
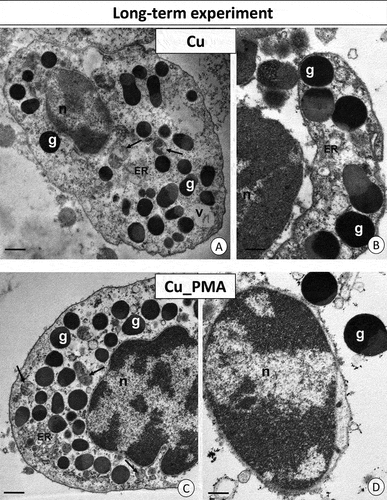
3.1.4. Autophagy
LysoTracker Red allowed the qualitative analysis of strongly acidic organelles and structures (e.g., autolysosomes, lysosomes) in the hemocytes present in the hemocoel. The signals emitted by acidic organelles were stronger in the hemocytes of individuals from the short-term experimental groups compared to the control groups. Furthermore, they were evident and stronger in the hemocytes of Cd and Cd_PMA experimental groups compared to the hemocytes of Cu and Cu_PMA experimental groups. No differences in signal strength were detected in the hemocytes of spiders of all experimental groups after the long-term experiment ().
3.2. Quantitative assessment of degenerative changes in hemocytes
Among the grouping variables examined—metal, duration of metal exposure, and immunostimulation with PMA—it was observed that the metal variable had the greatest influence on the differences between similarly treated spider groups. The combined effects of metal and PMA, as well as time and PMA, were found to be influential in terms of the percentage of necrotic cells. However, these factors did not show any significant differentiation in the percentage of early apoptotic cells in the hemolymph of this particular species ().
Table I. Analysis of variance (ANOVA/MANOVA) for examined parameters in the hemolymph of S. grossa spiders, considering metal exposure, time exposition on metals, and immunostimulation (PMA) as categorical factors (p < 0.05).
3.2.1. Flow cytometric analyses
A significant, nearly twofold, increase in the percentage of early apoptotic hemocytes was recorded only in the short-term Cu-exposed and nonstimulated group with respect to the control (P = 0.03) (). There were no statistically significant differences in the number of apoptotic cells between the other groups. In turn, some changes were noted in the percentage of late apoptotic hemocytes in the hemolymph of spiders after both short- and long-term copper and cadmium administration (). In the case of short-term Cu_int and Cd_int groups which were not immunostimulated, the percentage of late apoptotic hemocytes in the hemolymph (NS) was nearly fourfold (P = 0.005) and ninefold (P = 0.005) higher compared to the control. Among long-term-intoxicated groups, a nearly sixfold increase in the percentage of late apoptotic hemocytes was observed in PMA-stimulated Cu_int group (P = 0.013) and nonstimulated Cd_int group (P = 0.023), with respect to the control.
Figure 8. Percentage of early apoptotic cells (a), late apoptotic cells (b), and necrotic cells (c) in the hemolymph of Steatoda grossa in control group (CT) exposed to metals (Cu_int, Cd_int) and immunostimulant (PMA). Different letters (a, b) indicate significant differences between immunostimulated (PMA) and non-immunostimulated (NS) experimental groups within main groups (CT, Cu-int, and Cd-int); * indicates significant differences between CT group and Cu_int/Cd_int within NS or PMA groups; a, b indicate significant differences between short-term and long-term exposition within each metal groups and subgroups: NS or PMA; The Mann–Whitney U test (Median and quartile deviation: 25th and 75th percentiles; Q25 and Q75) of the analyzed parameters; p < 0.05); n = 3–7.
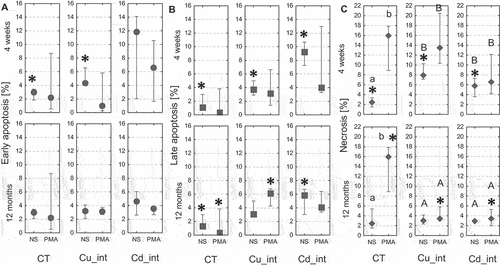
More than an eightfold increase in the number of necrotic cells (P = 0.002) was observed in the hemolymph of control individuals after PMA injection with respect to the nonstimulated control (). However, the degree of necrosis severity in the hemocytes of spiders treated with metal-contaminated food for short- or long term was almost similar respectively in PMA and nonstimulated groups.
Nevertheless, the percentage of necrotic hemocytes in the short-term Cd-exposed group was over twofold higher than the long-term Cd-exposed group that was either nonstimulated (P = 0.022) or stimulated with PMA (P = 0.045). Short-term Cu-exposed individuals from the nonstimulated group had 2.5-fold higher percentage of necrotic cells (P = 0.005) and threefold higher necrosis severity after PMA injection (P = 0.002), compared with long-term Cu-intoxicated spiders (). Spiders exposed long term to metals and additionally stimulated with PMA had nearly fivefold lower necrotic cells compared to the complementary control group. On the other hand, in individuals that were exposed short term to any metal and not stimulated, the number of necrotic cells was significantly higher than in control.
3.2.2. Concentration of ATP and ADP/ATP ratio
Regardless of the duration of exposition to cadmium-contaminated food, the ATP level in the hemocytes of nonimmunostimulated and PMA-stimulated individuals was nearly tenfold lower compared to the control (short-term exposition: nonstimulated, P = 0.005 and PMA, P = 0.005; long-term exposition: NS, P = 0.010 and PMA, P = 0.022) (). The ATP level in the hemocytes of short–term Cu-exposed spiders was close to that of the control. However, the ATP concentrations in the hemocytes of long-term Cu-exposed spiders were significantly (average 10-fold) lower than the complementary control individuals (nonstimulated, P = 0.02 and PMA, P = 0.03).
Figure 9. Concentrations of ATP (a) and rate of ADP/ATP (b) in the hemolymph of Steatoda grossa in control group (CT) exposed to metals (Cu_int, Cd_int) and immunostimulant (PMA). a, b indicate significant differences between short-term and long-term exposition within each metal groups and subgroups: NS or PMA; The Mann–Whitney U test (Median and quartile deviation: 25th and 75th percentiles; Q25 and Q75) of the analyzed parameters; p < 0.05); n = 3–7.
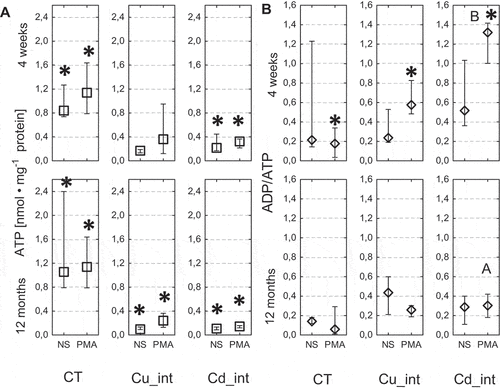
The ADP/ATP ratio in the hemocytes of control nonstimulated (CT) and control immunostimulated (CT_PMA) individuals was low and close to 0.2. In turn, in the short-term Cd- or Cu-exposed and immunostimulated spiders, the ADP/ATP ratio was, respectively, twofold (P = 0.011) and sixfold (P = 0.019) higher compared with the complementary control group (CT_PMA) (). There were no statistically significant differences in the ADP/ATP ratio between the nonstimulated Cu- or Cd-fed groups and the control group. In the case of individuals exposed long term to metals, the ADP/ATP ratio was almost similar. Additionally, the ADP/ATP ratio in the hemocytes of individuals exposed to PMA and intoxicated short term with cadmium were nearly fourfold higher, compared to the complementary group intoxicated long term with this metal (P = 0.024).
3.2.3. Antioxidative parameters
In control individuals that were stimulated with PMA, SOD and CAT activities were significantly higher compared with the nonstimulated group (respectively P = 0.02 and P = 0.01). Furthermore, the TAC level in the stimulated group was significantly lower compared to the control nonstimulated group (P = 0.04). Only in individuals that were long-term intoxicated with Cu and stimulated with PMA, the SOD activity was higher compared with the nonimmunostimulated control group (P = 0.03). Additionally, the SOD activity in the hemolymph of spiders long-term intoxicated with Cu was nearly eightfold higher compared to that in short-term exposure groups (P = 0.03) (). No significant differences in the level of this parameter were recorded after long-term Cd intoxication. Similarly, the SOD activity in the hemolymph of spiders exposed short term to food contaminated with either of the metals was close to that of the control. In contrast, the CAT activity in the hemolymph of spiders exposed short term to copper or cadmium and additionally immunostimulated with PMA was nearly sixfold lower, compared to the complementary control groups (Cu, P = 0.01; Cd, P = 0.02) (). The CAT activity in the hemolymph of spiders long-term exposed to metal-contaminated food was close to that of the control. Moreover, the CAT activity in the hemolymph of control individuals after PMA injection was significantly higher compared to the nonstimulated group (NS). Additionally, the CAT activity in the spiders of stimulated groups during short-term experiment was nearly fivefold higher than in the complementary groups of long-term experiment (P = 0.02) ().
Figure 10. Activity of superoxidae dismutase (SOD; a), catalase (CAT; b) and Total antioxidant capacity (TAC; c) in the hemolymph of Steatoda grossa in control group (CT) exposed to metals (Cu_int, Cd_int) and immunostimulant (PMA). Different letters (a, b) indicate significant differences between immunostimulated (PMA) and non-immunostimulated (NS) experimental groups within main groups (CT, Cu-int, and Cd-int); * indicates significant differences between CT group and Cu_int/Cd_int within NS or PMA groups; a, b indicate significant differences between short-term and long-term exposition within each metal groups and subgroups: NS or PMA; The Mann–Whitney U test (Median and quartile deviation: 25th and 75th percentiles; Q25 and Q75) of the analyzed parameters; p < 0.05); n = 3–7.
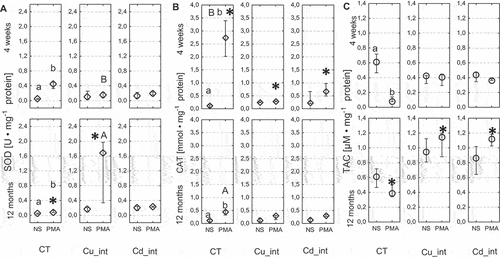
The TAC level in the hemocytes of spiders short-term exposed to food contaminated with metals, and stimulated or not stimulated with PMA, was close to that of the control. Only in the hemolymph of spiders long-term exposed to metals and immunostimulated, the TAC level was threefold higher as compared to the complementary control groups (P = 0.01). No significant differences in the level of this parameter were recorded in the long-term-intoxicated and nonstimulated individuals ().
4. Discussion
Hemocytes, which are the main effectors of the spiders’ immune system, showed quantitative changes in viability, as confirmed by qualitative changes at the ultrastructural level under the influence of the applied metals and PMA. TEM micrographs revealed changes in the ultrastructure of mainly granulocytes, as these cells were the most abundant in the analyzed preparations. PMA was used in our study because previous experiments indicated it as an effective factor in changing the quantitative proportion of individual hemocyte subpopulations (Wiśniewska et al. Citation2022). Moreover, other studies on the hemocytes of various invertebrate species, including earthworms, crabs, or bivalves, have shown the differential effects of a specific type of immunostimulation on the number of individual hemocyte subpopulations and the quality of immune responses (Homa et al. Citation2013; Rebelo et al. Citation2013, Citation2016; Zhou et al. Citation2018). PMA potentiates oxidative burst in hemocytes, resulting in the release of ROS such as superoxide anion (O2●–), which is one of the key components of the innate immune system of organisms (Damascena et al. Citation2022). The oxidative burst of hemocytes results in the effective elimination of infectious organisms; however, it can also be harmful to hemocytes, causing their degeneration. Our study showed an increase in SOD and CAT activities in control individuals after PMA treatment, which is presumably a manifestation of defense responses to increasing concentrations of ROS. However, the ATP concentration and the ADP/ATP ratio did not change significantly in response to the applied stimulant. Low values of the ADP/ATP ratio, resulting from an increase in the number of ATP molecules in cells, are an indicator of metabolic disturbances. Nevertheless, a statistically significant increase in the percentage of necrotic cells was noted in the hemolymph of control individuals treated with PMA. TEM images also showed necrotic changes in the ultrastructure of hemocytes after PMA treatment. Our study indicates that the oxygen burst reaction in hemocytes triggers a number of changes in the immune system of spiders and may represent an important defense mechanism activated during infection (Wiśniewska et al. Citation2022, Citation2023).
Autophagy serves as an additional mechanism for cellular protection. It involves the encapsulation of various organelles within autophagosomes, which are subsequently targeted by lysosomes for cytoplasmic component digestion. In some cases, this process can lead to the demise of the entire cell. Autophagy also facilitates the degradation of degenerated or disrupted organelles, as well as reserved material (e.g., lipids, proteins, and saccharides), and even xenobiotics (e.g., heavy metals) (Klionsky & Emr Citation2000; Klionsky et al. Citation2016; Lipovšek et al. Citation2018; Rost-Roszkowska et al. Citation2018). The breakdown of reserved material can be utilized as an energy source for different protective mechanisms. Notably, autophagy can act as a prosurvival factor, protecting the cells against death in response to certain stressors (Rost–Roszkowska et al. Citation2020, Citation2022). During our studies, we observed stronger signals emitted by acidic organelles (e.g., lysosomes and autolysosomes) in the hemocytes of individuals from the short-term experimental groups compared to the control groups. These signals were particularly pronounced in the hemocytes of the Cd and Cd_PMA experimental groups when compared to the hemocytes of the Cu and Cu_PMA experimental groups. Additionally, a reduction in glycogen content was observed in our investigations of hemocytes. This observation suggests that autophagy may have been activated as a protective response against cell death, primarily during the short-term experiments, while it did not prove to be a sufficient process for cellular protection during prolonged exposure to the experimental conditions. However, it is important to note that autophagy can be accompanied by necrosis and/or apoptosis. In the case of necrosis, cell organelles swell, and their membranes experience disruption, resulting in an electron-lucent cytoplasm devoid of organelles (Rost–Roszkowska et al. Citation2020; Ostróżka et al. Citation2022, Citation2022). The ultrastructural alterations observed in our studies resemble necrosis, suggesting that this process was activated under experimental conditions.
For both metals, the 4-week exposure resulted in an increase in the percentage of apoptotic and necrotic cells relative to the control. However, the qualitative analysis by TEM highlighted the presence of granulocytes with damaged cell membranes and cytoplasm deficient in cell organelles, mainly in Cd-exposed individuals. Mitochondria had a disrupted membrane in this experimental group, and an increased frequency of autophagic changes was also recorded. The differences in the structure of cell organelles in the hemocytes of Cd-intoxicated spiders may have resulted from the increased production of ROS in the cells, causing damage to cellular components. The mechanism behind the induction of ROS generation by cadmium is poorly understood. It has been suggested that cadmium indirectly enhances ROS formation by inhibiting enzymatic and nonenzymatic antioxidant systems, thereby increasing cellular ROS concentrations (Liu et al. Citation2009). It has also been proposed that cadmium displaces Fe (II) and Cu (I) from cytoplasmic and membrane proteins, increasing the amounts of free copper and iron ions, which enter into Fenton reactions contributing to increased ROS concentrations in the cells (Waisberg et al. Citation2003; Valko et al. Citation2006). In the case of hemocytes from copper-intoxicated spiders, changes in cell ultrastructure were minimal; however, an increased percentage of cells with apoptotic and necrotic features was observed. Copper undergoes redox cycling and readily enters into reactions that generate ROS, so the observed changes could have been the result of oxidative stress directly induced by Cu (Valko et al. Citation2006). Regardless of the metal administered, spider hemocytes showed low concentrations of ATP. Previous studies have also shown a decrease in ATP concentration and an increase in the ADP/ATP ratio in the hemocytes of female Steatoda grossa spiders after short-term exposure to cadmium (Wilczek et al. Citation2018). Moreover, laboratory tests confirmed that cadmium administered through the diet to S. grossa spiders had genotoxic effects on hemocytes and decreased their viability (Stalmach et al. Citation2015b). However, PMA administration did not potentiate the severity of degenerative changes in hemocytes from both Cd- and Cu-exposed groups, as the percentage of necrotic hemocytes was similar to that in complementary controls. The hemocytes of PMA-treated individuals showed changes predictive of degenerative processes: a decrease in the ATP concentration and an increase in the ADP/ATP ratio, as well as a decrease in the CAT activity. This suggests that the metabolic condition of hemocytes was impaired by metals administered for the short term but the process of oxygen burst in these cells was inhibited after PMA administration.
Chronic exposure of spiders to Cd-contaminated food attenuated the activity of SOD and CAT in hemocytes after PMA administration. However, the percentage of hemocytes showing necrotic features in the Cd-intoxicated group was lower than in the control stimulated individuals. Long-term exposure to copper did not increase degenerative changes in cells either during immunostimulation or in its absence. Increased SOD activity was recorded in copper-exposed and immunostimulated individuals. In both Cd- and Cu-exposed groups, the administration of PMA resulted in an increase in the TAC levels in the hemolymph. Although ATP concentration in hemocytes decreased relative to the control, the ADP/ATP ratio did not change, indicating the stability of cellular energy resources. Other studies have also proven that the effects of metals on immune cells are ambiguous and complex. For example, in human neutrophils that were stimulated or unstimulated with PMA, exposure to cadmium at certain concentrations caused a reduction in ROS production (Dahlgren & Karlsson Citation1999; Poniedziałek et al. Citation2012). Some studies showed that cadmium had no effect on ROS production, for example, in human polymorphonuclear leukocytes and human bronchial epithelial cells (Zhong et al. Citation1990; Schmid et al. Citation2007). In contrast, a study by Freitas et al. (Citation2010) indicated that the exposure of human neutrophils to Cd (II) in the presence of PMA resulted in synergistically increased stimulation of oxidative burst, while exposure to other metals, including copper, had no effect on the activation of these cells by PMA. In addition, among the tested metals, only Cd was capable of activating the oxidative burst reaction at nontoxic concentrations. The authors of the cited study further reported that Cd (II) was capable of inducing neutrophil oxidative burst through the activation of protein kinase C, excluding the involvement of other cellular pathways in the ROS generation mediated by this metal (Freitas et al. Citation2010). In contrast, copper stimulated the function of Carassius auratus L. macrophages at low concentrations (up to 100 ppb) but had an inhibitory effect at higher concentrations (175 ppb) (Muhvich et al. Citation1995). Inhibition of the respiratory burst response was also recorded with an increase in the concentration of ceruloplasmin (Cp) in the blood of pregnant women (Varfolomeeva et al. Citation2016). This highlights that, depending on their nature, concentration, and time of interaction, metals can modulate the innate immune response and thus change the effectiveness of protection against pathogens.
Thus, it may be concluded that under conditions of chronic metal exposure and likely increased concentrations of ROS in the body, spiders can maintain redox balance, as evidenced by high TAC levels after PMA administration. The maintenance of antioxidant defense capacity under increased oxidative stress indicates the activation of defense mechanisms to prevent severe damage to cellular macromolecules. As shown in previous studies, spiders can either produce a high amount of metallothioneins or reduce their uptake, in response to increasing concentrations of metals in the body by storing them in the form of mineral granules in the cells of the midgut glands (Migula et al. Citation2013; Wilczek Citation2017).
Ultrastructural analysis of the hemocytes of metal-intoxicated individuals showed that the degree of damage caused by a metal depended on its type. Intoxication with cadmium and administration of PMA resulted in an increase in the number of cells with necrotic features and the appearance of granulocytes with a reduced number of organelles. In contrast, copper-treated and immunostimulated individuals showed mostly normal granulocytes, and only a few necrotic cells. Our previous study evaluating the changes in the proteome of S. grossa hemolymph following long-term metal exposure showed increased production of Cp in individuals inoculated with cadmium alone, while simultaneous immunostimulation with this xenobiotic decreased the production of the mentioned protein (Wiśniewska et al. Citation2023). However, although Cp protein binds to excess copper and participates in iron metabolism in the body, there are no reports showing its altered expression in the hemolymph of individuals injected with this nutrient, both alone and after immunostimulation. In a study by Sugawara and Sugawara (Citation1984), cadmium injection into rats resulted in an increase in Cu concentration in microsomes and the stimulation of serum Cp activity, which was explained as an effect of increased Cp synthesis in the liver. In addition, Cp has also been shown to participate in defense mechanisms during the onset of oxidative stress. On the other hand, our previous work on S. grossa revealed that copper was less accumulated than cadmium. This suggests that the increased excretion of copper may have reduced its toxic effects on the organism (Wilczek et al. Citation2018). Furthermore, copper undergoes binding in invertebrates, including spiders, with hemocyanin, acting as a respiratory dye. In our study, all seven hemocyanin chains (A, B, C, D, E, F, G) were identified in the hemolymph of S. grossa spiders (Wiśniewska et al. Citation2023), similar to a study on another spider species, Brachypelma albopilosa of the Theraphosidae family (Trabalon et al. Citation2010). In cadmium-exposed individuals, immunostimulation resulted in a decreased expression of E- and A-chain-containing hemocyanin and an increased expression of G-chain-containing protein, while in copper-intoxicated spiders it increased the expression of E-, F-, and G-chain-containing proteins (Wiśniewska et al. Citation2023). As a copper-containing respiratory pigment, hemocyanin not only performs respiratory function but may also play an immune role in spiders (Trabalon et al. Citation2010), as it does in other invertebrates (Destoumieux–Garzón et al. Citation2001; Zhang et al. Citation2004, Citation2009). Moreover, this multifunctional protein participates in antioxidant defense due to its iron-chelating ability and thus reduces the risk of biomolecule destruction resulting from the initiation of harmful chain reactions mediated by oxygen free radicals (Mileva et al. Citation2017). In addition, it was shown to significantly stimulate SOD activity and reduce malondialdehyde levels in the serum and liver of Sarcoma-180 tumor-bearing mice (S180) (Liu et al. Citation2017).
The results suggest that prolonged exposure to copper may have led to increased production of copper-binding proteins, promoting processes that inhibit degenerative changes in cells.
5. Conclusions
Our study showed that cadmium, compared to copper, caused severe necrotic changes in hemocytes and impaired oxygen burst reactions and antioxidant responses in spiders, regardless of the exposure time. Copper induced degenerative changes only during short-term exposure, but chronic intoxication with this nutrient did not impair metabolic processes in hemocytes, indicating the activation of defense mechanisms. Qualitative analysis of the status of hemocytes as well as quantitative assessment of cell viability and the antioxidant activity of the hemolymph of S. grossa spiders, as a test species, can be helpful to predict the efficiency of antioxidative defense and the ability to maintain immune status after metal exposure in this group of carnivorous invertebrates. The results obtained from this study offer comparative data on the spider’s reactions to metals, which can aid in interpreting findings from field studies.
Disclosure statement
No potential conflict of interest was reported by the author(s).
Additional information
Funding
References
- Baumann T, Kämpfer U, Schürch S, Schaller J, Largiadèr C, Nentwig W, Kuhn–Nentwig L. 2010. Ctenidins: Antimicrobial glycine–rich peptides from the hemocytes of the spider Cupiennius salei. Cellular and Molecular Life Science 67(16):2787–2798. DOI: 10.1007/s00018-010-0364-0.
- Bradford MM. 1976. A rapid and sensitive method for the quantitation of microgram quantities of protein utilizing the principle of protein dye–binding. Analytical Biochemistry 72:248–254. DOI: 10.1016/0003-2697(76)90527-3.
- Chen X, Zhang Z, Liu R, Zhang X, Chen J, Peng Y. 2011. Effects of the metals lead and zinc on the growth, development, and reproduction of Pardosa astrigera (Araneae: Lycosidae). Bulletin of Environmental Contamination and Toxicology 86:203–207. DOI: 10.1007/s00128-011-0194-2.
- Dahlgren C, Karlsson A. 1999. Respiratory burst in human neutrophils. Journal of Immunological Methods 232:3–14. DOI: 10.1016/s0022-1759(99)00146-5.
- Damascena HL, Silveira WAA, Castro MS, Fontes W. 2022. Neutrophil activated by the famous and potent PMA (Phorbol myristate acetate). Cells 11:2889. DOI: 10.3390/cells1118288.
- Destoumieux–Garzón D, Saulnier D, Garnier J, Jouffrey C, Bulet P, Bachere E. 2001. Crustacean immunity: Antifungal peptides are generated from the c terminus of shrimp hemocyanin in response to microbial challenge. Journal of Biological Chemistry 276(50):47070–47077. DOI: 10.1074/jbc.M103817200.
- Foelix RF. 2011. Biology of spiders. 3rd ed. USA: Oxford University Press. pp. 66–74.
- Freitas M, Jlfc L, Porto G, Fernandes E. 2010. Metal–induced oxidative burst in isolated human neutrophils. Microchemical Journal 96:167–171. DOI: 10.1016/j.microc.2010.03.002.
- Fukuzawa HA, Vellutini CB, Lorenzini MD, Silva J, Mortara IP, Silva MCJ AR, Daffre S. 2008. The role of hemocytes in the immunity of the spider Acanthoscurria gomesiana. Developmental and Comparative Immunology 32:716–725. DOI: 10.1016/j.dci.2007.11.002.
- Homa J, Stalmach M, Wilczek G, Kolaczkowska E. 2016. Effective activation of antioxidant system by immune–relevant factors reversely correlates with apoptosis of Eisenia andrei coelomocytes. Journal of Comparative Physiology B: Biochemical Systems and Environmental Physiology 186(4):417–430. DOI: 10.1007/s00360-016-0973-5.
- Homa J, Zorska A, Wesołowski D, Chadznska M. 2013. Dermal exposure to immunostimulants induces changes in activity and proliferation of coelomocytes of Eisenia andrei. Journal of Comparative Physiology B: Biochemical Systems and Environmental Physiology 183(3):313–322. DOI: 10.1007/s00360-012-0710-7.
- Klionsky DJ . 2016. Guidelines for the use and interpretation of assays for monitoring autophagy (3rd edition). Autophagy 12:1–222. DOI: 10.1080/15548627.2015.1100356.
- Klionsky DJ, Emr SD. 2000. Autophagy as a regulated pathway of cellular degradation. Science 290:1717–1721. DOI: 10.1126/science.290.5497.1717.
- Kuhn–Nentwig L, Kopp LS, Nentwig W, Haenni B, Streitberger K, Schürch S, Schaller J. 2014. Functional differentiation of spider hemocytes by light and transmission electron microscopy, and MALDI–MS–imaging. Developmental and Comparative Immunology 43(1):59–67. DOI: 10.1016/j.dci.2013.10.008.
- Kuhn–Nentwig L, Nentwig W. 2013. The immune system of spiders. In: Nentwig W, editor. Spider ecophysiology. New York Dordrecht London: Springer Heilderberg. pp. 81–91.
- Lipovšek S, Leitinger G, Novak T, Janžekovič F, Gorgoń S, Kamińska K, Rost-Roszkowska M. 2018. Changes in the midgut cells in the European cave spider, Meta menardi, during starvation in spring and autumn. Histochemistry and Cell Biology 149:245–260. DOI: 10.1007/s00418-017-1623-z.
- Liu J, Qu W, Kadiiska MB. 2009. Role of oxidative stress in cadmium toxicity and carcinogenesis. Toxicology and Applied Pharmacology 238:209–214. DOI: 10.1016/j.taap.2009.01.029.
- Liu S, Zheng L, Aweya JJ, Zheng Z, Zhong M, Chen J, Wang F, Yueling Zhang Y. 2017. Litopenaeus vannamei hemocyanin exhibits antitumor activity in S180 mouse model in vivo. PLoS ONE 12(8):e0183783. DOI: 10.1371/journal.pone.0183783.
- Migula P, Wilczek G, Babczyńska A. 2013.Effects of Heavy Metal Contamination. In: Nentwig W, editor. Spider Ecophysiology, Springer–Verlag, Berlin, Heidelberg. pp. 403–414. DOI: 10.1007/978-3-642-33989-9_30
- Mileva M, Raynova Y, Kindekov I, Krastev D, Idakieva K. 2017. In vitro investigation of the antioxidant properties of Cancer pagurus. Hemocyanin Bulgarian Chemical Communications 49(1):11–16.
- Muhvich AG, Jones RT, Kane AS, Anderson RS, Reimscheussel R. 1995. Effects of chronic copper exposure on the macrophage chemiluminescent response and gill histology in goldfish (Carassius auratus L.). Fish and Shellfish Immunology 5:251–264. DOI: 10.1006/fsim.1995.0025.
- Nomura N, Nomura M, Sugiyama K, Hamada JI. 2007. Phorbol 12–myristate 13–acetate (PMA) – Induced migration of glioblastoma cells is mediated via p38MAPK/Hsp27 pathway. Biochemcal Pharmacology 74(5):690–701. DOI:10.1016/j.bcp.2007.06.018.
- Orr CWM. 1970. The inhibition of catalase (Hydrogen peroxide: Hydrogen peroxide oxidoreductase EC. 1.11.1.6) by ascorbate. In: Colowick S, Kaplan N, Mc Cormack D, and Wright L, editors. Methods in Enzymology. NY: Academic Press. pp. 59–62.
- Ostróżka A, Tiffert Z, Wilczek G, Rost-Roszkowska MM. 2022. Can insecticide-free clean water regenerate the midgut epithelium of the freshwater shrimp after dimethoate treatment? Micron 155:103162. DOI: 10.1016/j.micron.2021.103162.
- Poniedziałek B, Rzymski P, Wiktorowicz K. 2012. In vitro effect of cadmium on the function of human lymphocytes and neutrophils. Central European Journal of Immunology 37(2):110–113.
- Rebelo MF, Figueiredo ES, Mariante RM, Nóbrega A, Barros CM, Allodi S. 2013. New insights from the Oyster Crassostrea rhizophorae on bivalve circulating hemocytes. PLoS One 8(2):1–6. DOI: 10.1371/journal.pone.0057384.
- Re R, Pellegrini N, Proteggente A, Pannala A, Yang M, and Rice-Evans C. 1999. Antioxidant activity applying an improved ABTS radical cation decolorization assay. Free Radical Biology & Medicine 26(9–10):1231–1237.
- Ribeiro C, Brehélin M. 2006. Insect haemocytes: What type of cell is that? Journal of Insect Physiology 52(5):417–429. DOI: 10.1016/j.jinsphys.2006.01.005.
- Riciluca KCT, Sayegh RSR, Melo RL, Silva PI. 2012. Rondonin an antifungal peptide from spider (Acanthoscurria rondoniae) haemolymph. Research in Immunology 2:66–71. DOI: 10.1016/j.rinim.2012.03.001.
- Rost–Roszkowska M, Poprawa I, Chajec Ł, Chachulska–Żymełka A, Wilczek G, Wilczek P, Student S, Skowronek M, Nadgórska–Socha A, Leśniewska M. 2020. Influence of soil contaminated with cadmium on cell death in the digestive epithelium of soil centipede Lithobius forficatus (Myriapoda, Chilopoda. European Zoological Journal 87:242–262. DOI: 10.1080/24750263.2020.1757168.
- Rost-Roszkowska M, Poprawa I, Ł C, Chachulska-Żymełka A, Wilczek G, Skowronek M, Student S, Leśniewska M. 2022. Hazards related to the presence of cadmium in food – Studies on the European soil centipede, Lithobius forficatus. Science of the Total Environment 845:157298. DOI: 10.1016/j.scitotenv.2022.157298.
- Rost-Roszkowska MM, Janelt K, Poprawa I. 2018. The role of autophagy in the midgut epithelium of Parachela (Tardigrada). Zoomorphology 137:501–509. DOI: 10.1007/s00435-018-0407-x.
- Roy S, Noda Y, Eckert V, Eckert V, Trabe MG, Mori A, Liburdy R, Packer L. 1995. The phorbol 12–myristate 13– Acetate (PMA)– Induced oxidative burst in rat peritoneal neutrophils is increased by a 0.1 mT (60 Hz) magnetic field. FEBS Letters 376(3):164–166. DOI: 10.1016/0014-5793(95)01266-X.
- Schmid M, Zimmermann M, Krug HF, Sures B. 2007. Influence of platinum, palladium and rhodium as compared with cadmium, nickel and chromium on cell viability and oxidative stress in human bronchial epithelial cells. Environment International 33:385–390. DOI: 10.1016/j.envint.2006.12.003.
- Stalmach M, Wilczek G, Homa J, Szulińska E. 2015a. Antioxidative and immunological responses in the haemolymph of wolf spider Xerolycosa nemoralis (Lycosidae) exposed to starvation and dimethoate. Environmental Pollution 206:551–559. DOI: 10.1016/j.envpol.2015.08.007.
- Stalmach M, Wilczek G, Wilczek P, Skowronek M, Mędrzak M. 2015b. DNA damage in haemocytes and midgut gland cells of Steatoda grossa (Theridiidae) spiders exposed to food contaminated with cadmium. Ecotoxicology and Environmental Safety 113:353–361. DOI: 10.1016/j.ecoenv.2014.12.023.
- Sugawara N, Sugawara C. 1984. Comparative study of effect of acute administration of cadmium and silver on ceruloplasmin and metallothionein: Involvement of disposition of copper, iron, and zinc. Environmental Research 35(2):507–515. DOI:10.1016/0013-9351(84)90157-9.
- Trabalon M, Carapito C, Voinot F, Martrette J–M, Dorsselaer A, Glibert C, Bertile F. 2010. Differences in Brachypelma albopilosa (Theraphosidae) hemolymph proteome between subadult and adult feamales. Journal of Experimental Zoology 313A(10):651–659. DOI: 10.1002/jez.636.
- Valko M, Rhodes CJ, Moncol J, Izakovic M, Mazur M. 2006. Free radicals, metals and antioxidants in oxidative stress–induced cancer. Chemico-Biological Interactions 160:1–40. DOI: 10.1016/j.cbi.2005.12.009.
- Varfolomeeva EY, Semenova EV, Sokolov AV, Aplin KD, Timofeeva KE, Vasilyev VB, Filatov MV. 2016. Ceruloplasmin decreases respiratory burst reaction during pregnancy. Free Radical Research 50(8):909–919. DOI: 10.1080/10715762.2016.1197395.
- Waisberg M, Joseph P, Hale B, Beyersmann D. 2003. Molecular and cellular mechanisms of cadmium carcinogenesis. Toxicology 192:95–117. DOI: 10.1016/s0300-483x(03)00305-6.
- Wilczek G. 1996. Adenine nucleotides and adenylate energy charge as measures of physiological condition of spiders exposed to environmental stress. Studia Societatis Scientiarum Torunensis. Sectio G (Physiologia), Uniwersytet Mikołaja Kopernika, Toruń 4(3):137–142.
- Wilczek G. 2017. The use of spiders in the assessment of cellular effects of environmental stressors. In: Larramendy M, editor. Ecotoxicology and genotoxicology: Non–traditional terrestrial models. Issues in toxicology. UK: Royal Society of Chemistry. pp. 96–124.
- Wilczek G, Karcz J, Putko A, Kędziorski A, Wilczek P, Stalmach M, Szulinska E. 2017. The effect of ingested cadmium on the calorific value and structural properties of hunting webs produced by Steatoda grossa (Theridiidae) spiders. Science of the Total Environment 586:1298–1307. DOI: 10.1016/j.scitotenv.2017.02.143.
- Wilczek G, Karcz J, Rost–Roszkowska M, Kędziorski A, Wilczek P, Skowronek M, Wiśniewska K, Kaszuba F, Surmiak K. 2019. Evaluation of selected biological properties of the hunting web spider (Steatoda grossa, Theridiidae) in the aspekt of short– And long–term exposure to cadmium. Science of the Total Environment 656:297–306. DOI: 10.1016/j.scitotenv.2018.11.374.
- Wilczek G, Wiśniewska K, Kozina B, Wilczek P, Rost–Roszkowska M, Stalmach M, Skowronek M, Kaszuba F. 2018. Effects of food contamined with cadmium and copper on hemocytes of Steatoda grossa (Araneae: Theridiidae). Ecotoxicology and Environmental Safety 149:267–274. DOI: 10.1016/j.ecoenv.2017.12.007.
- Wiśniewska K, Rost–Roszkowska M, Homa J, Kasperkiewicz K, Surmiak– Stalmach K, Szulińska E, Wilczek G. 2022. The effect of selected immunostimulants on hemocytes of the false black widow Steatoda grossa (Theridiidae) spiders under chronic exposition to cadmium. Comparative Biochemistry and Physiology Part - C. Toxicology and Pharmacology 252:109221. DOI: 10.1016/j.cbpc.2021.109221.
- Wiśniewska K, Siatkowska M, Komorowski P, Napieralska, Kasperkiewicz K, Surmiak–Stalmach K, Wilczek G. 2023. Effects of chronic exposure to cadmium and copper on the proteome profile of hemolymph in false widow spider Steatoda grossa (Theridiidae). Ecotoxicology and Environmental Safety 249:114448. DOI: 10.1016/j.ecoenv.2022.114448.
- Zhang X, Huang C, Qin Q. 2004. Antiviral properties of hemocyanin isolated from shrimp Penaeus monodon. Antiviral Research 61(2):93–99. DOI: 10.1016/j.antiviral.2003.08.019.
- Zhang Y, Shen G, Yu Y, Zhu H. 2009. The hormetic effect of cadmium on the activity of antioxidant enzymes in the earthworm Eisenia fetida. Environmental Pollution 157(11):3064–3068. DOI: 10.1016/j.envpol.2009.05.039.
- Zhong ZJ, Troll W, Koenig KL, Frenkel K. 1990. Carcinogenic sulfide salts of nickel and cadmium induce H2O2 formation by human polymorphonuclear leukocytes. Cancer Res 50:7564–7570.
- Zhou Y, Gu WB, Tu DD, Zhu QH, Zhou ZK, Chen YY, Shu MA. 2018. Hemocytes of the mud crab Scylla paramamosain: Cytometric, morphological characterization and involvement in immune responses. Fish and Shellfish Immunology 72:459–469. DOI: 10.1016/j.fsi.2017.10.055.