Abstract
Aphids (Hemiptera: Aphididae) are herbivorous insects which deprive plants of nutrients by feeding on the phloem sap and transmission of plant viruses. In the present study, the susceptibility of selected white-berried grapevine (Vitis spp.) cultivars “Aurora”, “Bianca”, “Muskat Letni” and “Solaris” to the black bean aphid (Aphis fabae Scop.) and the green peach aphid (Myzus persicae (Sulz.) was evaluated using the Electrical Penetration Graph (EPG) technique. The content of major flavonoids and stilbenoids in grapevine leaves and leaf anatomy parameters that might have affected aphid probing were also analyzed. Based on the success rate in reaching the phloem vessels and feeding on phloem sap, the studied grapevine cultivars can be assigned to various groups of susceptibility. Cultivars “Bianca” and “Muskalt Letni” are susceptible to A. fabae and M. persicae, respectively (aphids reach phloem phase and show short bouts of sap ingestion); “Muskat Letni” shows low susceptibility to A. fabae, while “Aurora” and “Bianca” to M. persicae (only 50% aphids reach phloem phase and no sap ingestion occurs); “Solaris” is resistant to A. fabae and M. persicae (no phloem phase present). Anatomical structure of leaves was similar in all cultivars and no mechanical barriers for aphid probing were observed. However, the content of individual flavonoids and silbenoids differed among cultivars. We hypothesize that the high content of piceid in the leaves of “Solaris” may be responsible for the rejection of this cultivar by both aphid species. The study demonstrated that the risk of the direct damage due to A. fabae and M. persicae feeding on grapevines is low. However, considering the intense aphid probing activity within leaf epidermis and mesophyll, A. fabae and M. persicae may pose a serious threat as vectors of non-persistent and semi-persistent viruses to white-berried grapevines.
Introduction
Grapevines (Vitis spp.) are cultivated on nearly 7 million hectares worldwide and give approximately 80 million tonnes of grapes (OIV Citation2019; FAOSTAT Citation2022). The production of grapes is threatened by a multitude of different abiotic and biotic factors, the latter embracing bacteria, fungi, parasitic plants, and animals, of which parasitic fungi and arthropods are of the most serious concern (Bentley et al. Citation2005; Hocking et al. Citation2007; Vincent et al. Citation2012; Kassemeyer Citation2017; Ponti et al. Citation2018; Vitulo et al. Citation2019).
Aphids (Hemiptera: Aphididae) may affect grapevines directly by nutrient deprivation and indirectly, as virus vectors (Katis et al. Citation2007). To reach their basic food source – phloem sap in sieve elements – aphids use their mouthparts’ stylets to penetrate plant tissues both inter- and intracellularly: the stylets move towards phloem basically within apoplast but they habitually puncture cells adjacent to their route to examine plant tissue structure and chemical composition (Klingauf Citation1987; Tjallingii & Esch Citation1993; Powell et al. Citation2006; Pettersson et al. Citation2017). This particular probing makes aphids very efficient vectors of plant non-circulative and circulative viruses, which they transmit in a non-persisent, semi-persistent, and persistent ways (Ng & Perry Citation2004; Katis et al. Citation2007; Brault et al. Citation2010; Stevens & Lacomme Citation2017). The guild of grapevine-associated viruses include mainly viruses specific to grapevines but also those that attack a large variety of other plant species worldwide, such as Alfalfa mosaic virus (AMV), Bean common mosaic virus (BCMV), Broad bean wilt virus (BBMV), and Cucumber mosaic virus (CMV) (Sastry et al. Citation2019; Fuchs Citation2020). These viruses are transmitted very effectively by the black bean aphid Aphis fabae Scop. and the green peach aphid Myzus persicae (Sulz.) (Chan et al. Citation1991; Wamonje et al. Citation2020), which are extremely polyphagous, thus able to infest several hundred of plant species of different families, including grapevines (Holman Citation2009; Blackman & Eastop Citation2017).
During probing, aphids are confronted with the morphology, anatomy, and chemical composition of plant tissues, from cuticle and epidermis down to vascular tissues xylem and phloem (Powell et al. Citation2006; Smith & Chuang Citation2014). These plant traits determine aphid-host plant specialization on one hand, and on the other hand, plant susceptibility to aphid infestation (Züst & Agrawal Citation2016; Escudero-Martinez et al. Citation2021). Aphid resistance mechanisms in plants are considered valuable resources for sustainable pest management strategies. In particular, antixenosis, i.e., the detrimental effect of plant traits on herbivores upon their arrival or first attempts to feed is of great value (Dogimont et al. Citation2010; Stec et al. Citation2021; Alhmedi et al. Citation2022; Luo et al. Citation2022).
In our previous study (Paprocka et al. Citation2023), we evaluated the susceptibility of three grapevine species Vitis amurensis Rupr., V. riparia Michaux, and V. vinifera L. to A. fabae and M. persicae. We determined that these grapevines are relatively – although not equally – resistant to aphid infestation and the differences in acceptability may be attributed to the content of individual flavonoids and stilbenoids. The majority of grapevines cultivated today are interspecies “hybrids” which differ in the proportions of the basic species (Keller Citation2015). As the process of hybridization might have affected the grapevine resistance to aphids, the present research was carried out to study the susceptibility of selected white-berried grapevine hybrid cultivars to A. fabae and M. persicae infestation. For this purpose, aphid probing behavior was monitored with the use of Electrical Penetration Graph (EPG) technique known also as electropenetrography, which allows the real-time recording of aphid stylet penetration in plant tissues (Tjallingii Citation2006). We also analyzed the leaf phenolic compounds and leaf anatomy of the studied white-berried grapevine cultivars in search for antixenosis factors that might have affected aphid probing in leaf tissues.
Material and methods
Cultures of plants and aphids
Grapevines
The grapevines used in experiments were obtained from various sources: cultivars “Aurora” (syn. “Seibel 5279”) (France) VIVC number 784 (Maul & Töpfer Citation2022a) and “Solaris” (Germany) VIVC number 20,340 (Maul & Töpfer Citation2022b) were provided by Gartenland Polska Sp. z o.o., Łódź, Poland; cultivar “Bianca” (Hungary) VIVC number 1321 (Maul & Töpfer Citation2022c) was purchased from Global Flowers Ltd, Rotherham, UK through Sklep Ogrodniczy Plumeria (plumeria.sklep.pl), and “Muskat Letni” (Moldova) VIVC number 25,015 (Maul & Töpfer Citation2022d) – from Winnica Julia, Stary Kisielin-Zielona Góra, Poland. All plants were grown in commercial soil in plastic pots (vol. 4 dm3), in the laboratory at 20°C, 65% r.h., and L16:8D photoperiod. For all experiments, 1–2-year old plants were used.
Aphids
Laboratory rearings of Aphis fabae Scop. and Myzus persicae (Sulz.) were carried out as multiclonal colonies on Vicia faba L. cv. White Windsor and Brassica pekinensis (Lour.) Rupr. cv. Hilton, respectively, in the laboratory at 20°C, 65% r.h., and L16:8D photoperiod in a growing chamber Sanyo MLR-351 H (Sanyo Electronics Co. Ltd.).
Electronic monitoring of aphid probing behavior
Probing behavior of Aphis fabae and Myzus persicae on white-berried grapevine cultivars was monitored using the technique of electronic registration known as Electrical Penetration Graph (EPG) technique or electropenetrography that is frequently employed in insect–plant relationship studies (Will et al. Citation2007; Philippi et al. Citation2015). By using EPG technique it is possible to monitor aphid probing and feeding behavior within plant tissues and localize natural plant resistance factors (Dancewicz et al. Citation2016; Zhang et al. Citation2017; Stec et al. Citation2021). This technique allows a separate analysis of aphid behavior at pre-ingestive (within non-phloem tissues before the first phloem or xylem phase) and ingestive (within the phloem or xylem) phases of probing (Pettersson et al. Citation2007). In the experimental set-up, aphid and plant are made parts of an electric circuit, which is completed when the aphid inserts its stylets into the plant. Weak voltage is supplied in the circuit, and all changing electric properties are recorded as EPG waveforms that can be correlated with aphid activities and stylet position in plant tissues (Tjallingii Citation1994, Citation2006). The following EPG waveform patterns were distinguished: “np” (no probing, when aphid stylets did not have contact with plant tissues), “C” (pathway phase – penetration of epidermis and parenchyma/mesophyll), “E1e” (salivation into apoplast), “E1” (salivation into sieve elements), “E2” including “E1/E2” transitions (ingestion of phloem sap), “F” (derailed stylet activities representing undetermined difficulties in probing) and “G” (ingestion of xylem sap) (Tjallingii Citation2006) ().
Figure 1. The visualization of characteristic aphid probing activities recorded on grapevine leaves using Electrical penetration graph (EPG) technique. “probe” – aphid stylets in plant tissues, “np” – no probing (= aphid stylets outside plant tissues), “C” – pathway activity in apoplast with intracellular punctures “pd”, “E1e” – watery salivation into apoplast, “E1” – watery salivation into sieve elements, “E2” – phloem sap ingestion, “F” – derailed stylet activities in apoplast, “G” – sap ingestion from xylem vessels.
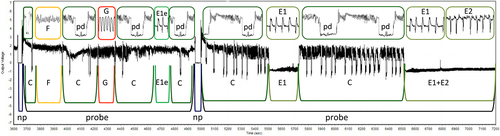
The parameters describing aphid behavior during probing and feeding, such as total time of probing, proportion of phloem patterns E1 and E2, number of probes, etc., are good indicators of plant suitability or interference of probing by chemical or physical factors in plant tissues (Mayoral et al. Citation1996; EPG-Systems Citation2022). In the present study, the waveform patterns that were not terminated before the end of the experimental period (8 h) were included in the calculations. In sequential parameters, when time to waveforms related to phloem phase (E1 or E2) or xylem phase (G) was calculated, only individuals that showed the particular phase were included in calculations and statistical analysis. In non-sequential parameters, when a given waveform had not been recorded for an individual, the duration of that waveform was given the value of 0.
In the present study, aphids for EPG experiments were 2–3 days old (2–3 days after the final moult) viviparous apterous A. fabae and M. persicae, which were attached to a golden wire electrode with silver paint and starved for 1 h prior to the experiments. Aphids were positioned in the centre of the adaxial side of a grapevine leaf and had a freedom to move limited by the length of the golden wire electrode (ca. 3 cm). The experiments were repeated 24 times for each species of grapevine and aphid. Each replicate consisted of a freshly prepared aphid and a plant. Incomplete recordings (i.e., recordings that terminated due to aphid falling from the plant or where EPG signal was unclear) were rejected from analysis, so the final number of replications used for analyses was as follows: A. fabae/’Aurora’, n = 20; A. fabae/’Bianca’, n = 18; A. fabae/’Muskat Letni’, n = 18; A.fabae/’Solaris’, n = 17, and M. persicae/’Aurora’, n = 19; M. persicae/’Bianca’, n = 22; M. persicae/’Muskat Letni’, n = 20, M. persicae/’Solaris’, n = 22. Probing behavior of aphids was monitored for 8 hs continuously and all bioassays started at 10:00–11:00 h MEST (Middle European Summer Time). Giga-8 DC EPG system with a 1 GΩ of input resistance (EPG Systems, Wageningen, The Netherlands) was used to record EPGs. EPGs were recorded and analyzed using Stylet+ Software (EPG Systems, Wageningen, The Netherlands). Signals were saved on the computer and the various behavioral phases were labelled manually using the Stylet+ software.
High-performance liquid chromatography of flavonoids and stilbenoids
The dried leaves of white-berried grapevine cultivars “Aurora”, “Bianca”, “Muskat Letni”, and “Solaris” (1.2 g of each) were homogenized in an aqueous ethanol solution (80%) using a Diax 900 homogenizer. The resulting suspension was centrifuged (12000 rpm, 10 min) and the supernatant solution was collected in a graduated flask and the pellet was reconditioned. This operation was repeated three times, and the obtained extracts were combined. The homogenization procedure in combination with the extraction was carried out in such a way that the final volume of the extract was 100 ml. From the prepared ethanol extracts, 10 ml was taken and evaporated to dryness in a rotary evaporator under reduced pressure at 40°C. The dry extracts were dissolved in 100% methanol to a volume of 1 ml. Resulting methanolic extracts containing flavonoids compounds were analyzed by HPLC–ESI-MS/MS.
The content of flavonoids apigenin, catechin, epicatechin, hesperetin, isorhamnetin, kaempferol, luteolin, naryngin, quercetin, rutin, taxifolin and stilbenoids ampelopsin, piceid, resveratrol, viniferin was determined. The selection of the phenolic compounds for analysis was based on literature data reporting the chemical composition of vegetative parts of grapevine (Castillo-Munoz et al. Citation2010; Pawlus et al. Citation2012; Portu et al. Citation2015; Biais et al. Citation2017; Aliaño-Gonzalez et al. Citation2020; Goufo et al. Citation2020; Baroi et al. Citation2022).
Individual pure flavonoids and stilbenoids were purchased from Sigma–Aldrich (Poland). Ethanol, HPLC gradient grade methanol and acetonitrile were supplied by Merck (Germany). Formic acid was purchased from Sigma-Aldrich (Poland). Stock standard solutions of individual phenolic compounds (50 mg/l) were prepared by dissolving appropriate amounts of solid reagents in methanol. Mixed working standard solutions of flavonoid compounds at 20, 10, 5, 2.5 and 1 mg/l concentrations were prepared by appropriate dilutions of stock standard solutions.
The chromatographic analysis was carried out with a Shimadzu LC system, comprising a LC20-AD binary pump, a DGU-20A5 degasser, a CTO-20AC column oven and a SIL-20AC autosampler, connected to a 3200 QTRAP hybrid triple quadrupole (Applied Biosystem, MDS SCIEX, USA) with electrospray ionization source (ESI) operated in negative-ion mode. Phenolic compounds were separated on a Phenomenex Luna C-18 column (100 × 2.0 mm × 3.0 µm) with a pre-column, both maintained at 30°C. A 7.4 mmol/l solution of formic acid (pH 2.8, eluent A) and acetonitrile (eluent B) were used. The mobile phase was delivered at 0.2 ml/min in a linear gradient mode as follows: 0–2 min 10% B, 30 min 60% B, 40 min 100% B, 55 min 10% B. Flavonoids and stilbenoids were identified by comparing their retention times and m/z values of precursor and resulting fragmentation product ions in their MS and MS/MS spectra, respectively, to those obtained for respective standard solutions analyzed under the same conditions. The quantification of flavonoids was done using calibration curves obtained in the SRM (single reaction mode) (Biesaga & Pyrzynska Citation2013; Sergiel et al. Citation2014).
Analysis of leaf anatomy
For anatomical measurements, 0.5 mm thick pieces from distal and proximal parts of the leaves were cut and fixed for 24 hours in Karmovsky’s fixative containing 10% paraformaldehyde and 25% glutaraldehyde in 0.2 M phosphate buffer (pH 7.2). The fixed material was rinsed three times (15 min. each time) in 0.1 M phosphate buffer (pH 7.4), dehydrated in a graded ethanol series (for 10 min. in 30%, 50%, 70%, and 96% ethanol and 2 × 10 min. in 99.8% ethanol), and placed in 100% polypropylene oxide (2 × 10 min). The slices were then placed overnight in a 1:1 mixture of epon resin (5.25 ml Poly/Bed 812, 3.25 ml DDSA, 0.75 ml MNA, 0.175 DMP) and propyl oxide. Next day, the plant samples were embedded in 100% epon resin. Transverse slices (0.5 μm) from distal and proximal sections of the leaves were obtained using a rotary automatic microtome Leica HistoCore Nanocut R. Slices were stained with toluidine blue, mounted on slides and observed under light microscope Carl Zeiss Axio Imager 2 coupled with Zeiss AxioCam ERc 5s and ZEN Lite computer programme at 400× magnification.
Anatomical evaluation of grapevine leaves included measurements of the abaxial epidermal cell thickness, the distance from abaxial leaf surface to phloem and the simulation of the shortest aphid stylet penetration pathway from abaxial leaf surface to phloem (). The stylet pathway was expressed as intrercellular area within epidermis and mesophyll (μm2). Three measurements at three different sites of the leaf midrib at proximal and distal sections of the leaf were taken. The average of the three measurements was considered one replication. The number of replications (= analyzed slices) was n = 9.
Statistical analysis
The values of EPG variables describing the aphid probing behavior were calculated manually and individually for every individual and the mean and standard errors were subsequently calculated using the EPG analysis Excel worksheet created by the authors especially for this study. Data thus obtained were analyzed by Kruskal–Wallis test and post-hoc multiple comparisons of mean ranks for all groups (Dunn’s test). The analysis was performed using Statistica 13.3 package. The results were also analyzed using multivariate methods. A canonical variate analysis (CVA) was applied in order to present a multi-trait assessment of the similarity of the tested cultivars in a lower number of dimensions with the least possible loss of information (Rencher Citation1992). It makes it possible to show variation in cultivars in terms of all the observed traits in the graphic form. In the present study, the selected traits for CVA were the total duration of no probing, total duration of probing in non-phloem tissues, and total duration of probing in phloem vessels. The Mahalanobis distance was suggested as a measure of “polytrait” cultivars diversity (Seidler-Łożykowska & Bocianowski Citation2012), the significance of which was verified by means of critical value Dα called “the least significant distance” (Mahalanobis Citation1936). Mahalanobis distances were calculated for the all pairs of cultivars. All CVA analyses were conducted using the GenStat 18 statistical software package.
For anatomical measurements, dual comparisons were applied. First, differences between values obtained from distal and proximal parts of the leaves were evaluated within each grapevine species by Mann-Whitney U-test. Second, differences among grapevine species using values obtained from distal and proximal parts of the leaves were evaluated by Kruskal–Wallis test and post-hoc multiple comparisons of mean ranks for all groups (Dunn’s test). The analysis was performed using Statistica 13.3 package.
Results
Aphid probing behavior
The 8-hour EPG monitoring of Aphis fabae and Myzus persicae probing behavior on white-berried grapevine cultivars revealed activities defined as no-probing (= aphid stylets outside plant tissues) and probing (= aphid stylets within plant tissues). Probing activities embraced the non-vascular phase (= aphid stylets in epidermis and mesophyll), xylem phase “G” (= aphid stylets in xylem vessels and ingestion of xylem sap), and phloem phase “E” (= aphid stylets in sieve elements). The non-vascular phase included progressive stylet movements in apoplast with intracellular punctures (= pathway phase) “C”, derailed stylet movements “F”, and watery salivation into apoplast – “E1e”. Phloem phase occurred very rarely and if present, it consisted of “E1” (= salivation) or E1 and E2 (= salivation and ingestion) (, ).
Figure 3. Temporal changes in probing behavior of Aphis fabae and Myzus persicae on white-berried grapevine cultivars “Aurora”, “Bianca”, “Muskat Letni”, and “Solaris”. np - no-probing, C – pathway, E1 – watery salivation into sieve elements, E2 – phloem sap ingestion, F – unidentified difficulties in penetration, E1e – watery salivation into apoplast, G – xylem sap ingestion, “total” – cumulative proportion of aphid activities during the 8-hour EPG monitoring.
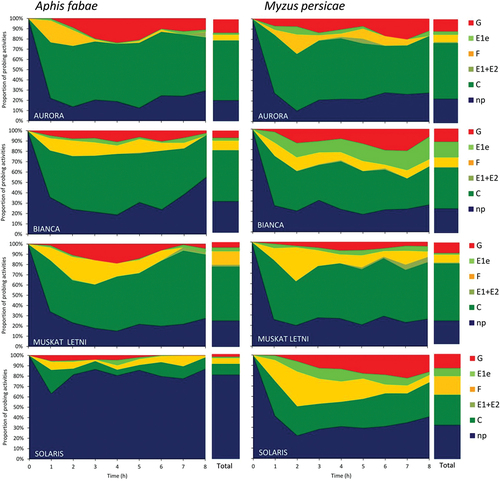
Table I. Probing behavior of Aphis fabae on white-berried grapevine (Vitis sp.) cultivars “Aurora”, “Bianca”, “Muskat Letni”, “Solaris”.
Table II. Probing behavior of Myzus persicae on white-berried grapevine (Vitis sp.) cultivars “Aurora”, “Bianca”, “Muskat Letni”, “Solaris”.
In A. fabae, stylet probing activities on “Aurora”, “Bianca” and “Muskat Letni” occupied similar proportions of the 8-hour experimental time, which were 79.0%, 69.8%, and 76.5%, respectively. In contrast, aphids on “Solaris” spent 81% of time on no-probing, which means that their stylets were outside plant tissues most of the time (, ). After having access to the leaves, aphids started to probe within 3–7 minutes on “Aurora”, “Bianca” and “Muskat Letni”, while on “Solaris”, the onset of probing was significantly delayed and reached 17.4 minutes on average. The number of individual probes, i.e., the periods when aphid stylets were within plant tissues, was highest on “Muskat Letni” and lowest on “Solaris”. At the same time, the mean duration of an individual probe was longest on “Aurora” and “Bianca” and shortest on “Solaris”. On “Solaris”, the short 2-minute probes predominated, while on “Aurora” – the most common were probes longer than 10 minutes. In all A. fabae on all grapevine cultivars, pathway phase was the main probing activity, and within pathway – the activity “C”. The occurrence and mean durations of other activities in non-vascular tissues – “E1e” and “F” – were similar in all A. fabae on all grapevine cultivars (, ). Xylem phase “G” occurred in 70%, 44.4%, 33.3% and 29.4% of aphids on “Aurora”, “Bianca”, “Muskat Letni” and “Solaris”, respectively (, ). The first xylem phase appeared within 2.5–3 hours from the onset of the experiment on average, on “Aurora”, “Bianca” and “Muskat Letni”, while on “Solaris” – within 0.8 hour (, ). There were no significant differences in the duration of xylem phase among aphids on different grapevine cultivars. However, the proportion of this activity among all aphid activities varied and was highest on “Aurora” (13.3%) and lowest on “Solaris” (2.5%) (). The phloem phase occurred only in A. fabae on “Aurora”, “Bianca” and “Muskat Letni” in 35%, 39%, and 56% EPG-recorded aphids, respectively (, ). In aphids on “Aurora” and “Muskat Letni”, the phloem phase consisted only of “E1” activity while in aphids on “Bianca”, activity “E2” occurred in two out of 18 aphids but the duration of this activity was negligible (, ). The first phloem phase occurred within 4.6–6.4 hours from the onset of probing ().
Figure 4. Proportion of Aphis fabae and Myzus persicae that reached vascular tissues – xylem and phloem – in the leaves of white-berried grapevine cultivars “Aurora”, “Bianca”, “Muskat Letni”, and “Solaris”.
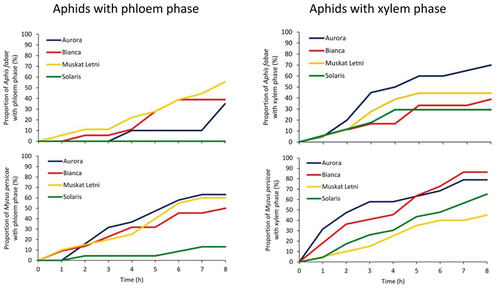
In M. persicae, stylet probng activities occupied similar proportions of the experimental time on all grapevine cultivars, which were from 67.4% on “Solaris” to 76.5% on “Aurora” (, ). After having access to the leaves, aphids started to probe within 1.5–5.8 minutes, the shortest time to 1st probe was on “Aurora” and the longest – on “Solaris”. The number of individual probes was lowest on “Bianca” and highest on “Muskat Letni” but the mean duration of individual probes did not differ significantly among aphids on different grapevine cultivars. On all cultivars, short 2-minute probes predominated, from 42% of all probes on “Aurora” to 50% of all probes on “Solaris”. The frequency of probes longer than 10 minutes was highest on “Aurora” and “Bianca” (30%) and lowest on “Solaris” (15%). Phloem phase occurred in 4.1% probes in aphids on “Bianca” and in 0.6% on “Solaris” (). In all M. persicae on all grapevine cultivars, pathway phase “C” was the main probing activity. Pathway “C” was shortest in aphids on “Solaris”. The occurrence and mean durations of other activities in non-vascular tissues – “E1e” and “F” – were similar in all M. persicae on all white-berried grapevine cultivars (, ). Xylem phase “G” occurred in 78.9%, 86.4%, 45.0% and 65.2% “Aurora”, “Bianca”, “Muskat Letni” and “Solaris”, respectively (, ).
The first xylem phase appeared within 2–4 hours from the onset of the experiment on average. The total duration of xylem phase in aphids on different grapevine cultivars was similar but the proportion of this activity among all activities varied and ranged from 5.0% in aphids on “Muskat Letni” to 12.3 on “Bianca” and “Solaris” (). The phloem phase occurred in 63%, 50%, 60%, and 13% M. persicae on “Aurora”, “Bianca”, “Muskat Letni”, and “Solaris”, respectively (, ). In aphids on “Aurora”, “Bianca” and “Solaris”, the phloem phase consisted only of “E1” activity while in aphids on “Muskat Letni”, activity “E2” occurred in one out of 20 aphids but the duration of this activity was negligible (, ). The first phloem phase occurred within 3.3–3.8 hours from the onset of probing ().
The variability of the quantitative traits (total durations of no probing, probing in non-phloem tissues, and probing in phloem vessels) of the cultivars in terms of the first two canonical variables (V1 and V2) is shown in . In the graph, the coordinates of the point for particular cultivar are the values for the first and second canonical variables, respectively. In A. fabae, the first canonical variable (V1) accounted for 97.97% of the total multivariate variability between the individual cultivars. The greatest variation in terms of all the traits, based on the measured Mahalanobis distances, was found for cultivars “Aurora” and “Solaris” (the Mahalanobis distance between them amounted to 2.9338). The greatest similarity was found for cultivars “Aurora” and “Muskat Letni” (0.2691) (). In M. persicae, the first canonical variable (V1) accounted for 81.06% of the total multivariate variability between the individual cultivars. The greatest variation in terms of all the traits, based on the measured Mahalanobis distances, was found for cultivars “Muskat Letni” and “Solaris” (the Mahalanobis distance between them amounted to 0.6686). The greatest similarity was found for cultivars “Aurora” and “Bianca” (0.1647) ().
Figure 5. The variability of the quantitative traits – total durations of no probing, probing in non-phloem tissues, and probing in phloem vessels by Aphis fabae (upper graph) and Myzus persicae (lower graph) – of the white-berried grapevine cultivars in terms of the first two canonical variables. In the graph, the coordinates of the point for particular cultivar are the values for the first and second canonical variables, respectively.
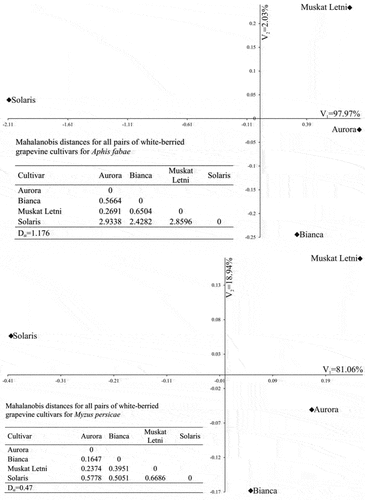
Flavonoids and stilbenoids in grapevine leaves
In the leaves of the white-berried grapevine cultivars studied, both flavonoids and stilbenoids were identified (, ). However, the total amount, the spectrum and the proportion of individual phenolic compounds differed among cultivars. The total amount of analyzed phenolic compounds ranged from 13.5 μg/g fresh weight in “Bianca” to 70.1 μg/g in “Solaris” (). Flavonoids constituted approximately 60% of total phenolics in “Aurora” and “Solaris” and 99.5% and 95.5% in “Bianca” and “Muskat Letni”, respectively. In “Aurora” and “Solaris”, six flavonoids (two flavanols and four flavonols) and three stilbenoids, while in “Bianca” and “Muskat Letni” – four flavonoids (two flavanols and two flavonols) and one stilbenoid were detected. In “Aurora” and “Bianca”, the most abundant flavonoid was quercetin (33% and 82% of total phenolic compounds, respectively), in “Muskat Letni” – rutin (47%), and in “Solaris” – qurcetin and catechin (19%). Of the stilbenoids, piceid occurred in all cultivars, while resveratrol and viniferin – only in “Aurora” and “Solaris”. In “Aurora”, the most abundant was resveratrol (25% of total phenolics), while in “Solaris” – piceid (38.3% of total phenolics) (, ).
Figure 6. Proportion of phenolic compounds analyzed in white-berried grapevine cultivars “Aurora”, “Bianca”, “Muskat Letni”, and “Solaris”.
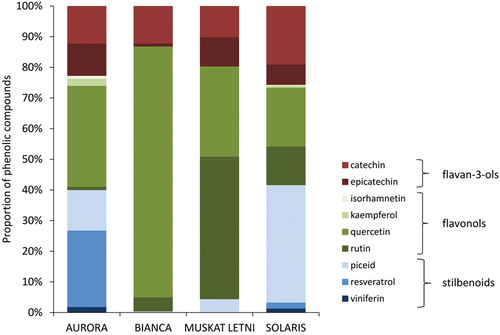
Table III. Phenolic compounds analyzed in the leaves of the white-berried grapevine cultivars “Aurora”, “Bianca”, “Muskat Letni”, “Solaris” (μg/g dry weight).
Anatomy of grapevine leaves
The anatomical structure of grapevine leaves was observed using transverse sections of proximal and distal parts of the leaves, at the region of the midrib. The visual examination revealed adaxial epidermis, two-three layers of collenchyma, a stratum of parenchyma, and the vascular bundle surrounded by a sheath (). No distinct differences in the anatomical organization of leaf tissues occurred among the studied grapevine cultivars (). The thickness of abaxial epidermis cells in proximal part of grapevine leaves ranged from 5.0 µm in “Aurora” and “Muskat Letni” to 6.2 μm in “Bianca” and was approximately 5.4–5.6 µm in distal parts of all cultivars. The distance from leaf surface to phloem ranged from 59.8 µm in “Solaris” to 93.7 µm in “Muskat Letni” (proximal part) and from 74.4 µm in “Bianca” to 94.5 µm in “Aurora” (distal part). Significant differences between values in proximal and distal parts of the leaves occurred in “Aurora” and “Solaris”. The value of the simulated shortest aphid stylet penetration pathway was lowest in “Aurora” (131.1 μm2) and highest in “Bianca” and “Muskat Letni” (265.9 μm2 and 250.4 μm2, respectively) in proximal parts and lowest in “Bianca” and highest in “Solaris” (191.2 μm2 and 248.6 μm2, respectively) in distal parts. Significant differences between values in proximal and distal parts of the leaves occurred in “Aurora” and “Solaris” ().
Figure 7. Representative samples of transverse leaf sections at the main veins of the leaves of the white-berried grapevine cultivars “Aurora”, “Bianca”, “Muskat Letni”, “Solaris” observed under light microscope Carl Zeiss Axio Imager 2 coupled with Zeiss AxioCam ERc 5s and ZEN lite computer programme (400x magnification). 1 – adaxial epidermis; 2 – collenchyma; 3 – parenchyma; 4 – phloem; 5 – xylem.
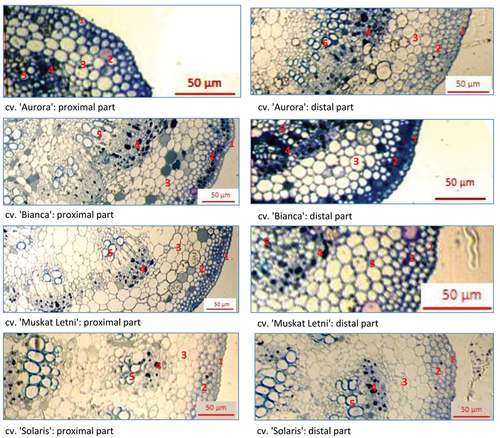
Table IV. Anatomical measurements of leaves of white-berried grapevine cultivars “Aurora”, “Bianca”, “Muskat Letni”, “Solaris”.
Discussion
In the present study, we investigated the behavioral response of two species of aphids – A. fabae and M. persicae to selected white-berried grapevine cultivars “Aurora”, “Bianca”, “Muskat Letni”, and “Solaris”. Our aim was to reveal the potential of these grapevines to resist aphid infestation and uncover the constitutive physical and/or chemical barriers which may prevent the aphids from colonization of these grapevines. Plant traits including the naturally existing variation in plant chemistry and anatomical structure are usually the starting points, called “phenotyping”, for further breeding of cultivars resistant to pest infestation (War et al. Citation2012; Reynolds et al. Citation2020).
The real-time EPG monitoring of aphid activities during probing in grapevine leaf tissues exposed a variation in behavior in both aphid species studied, depending on grapevine cultivar and aphid species. The observed differences occurred before aphids started probing and both when aphid stylets were in peripheral leaf tissues – epidermis and mesophyll – and in vascular tissues – xylem and phloem.
The probing activity of A. fabae started right after the aphids had access to the leaves on all cultivars except on “Solaris”, where the first probe was significantly delayed. In contrast, M. persicae did not show such delay: there were no clear-cut differences in aphid behavior before the first probe on grapevine cultivars. Aphids usually tend to start probing with no delay when no repellent stimuli are present at plant surface (Powell et al. Citation1997). It is likely that the leaves of “Solaris” grapevine emit volatiles or plant cuticle contains chemicals that discourage aphids to probe. However, this aspect would require further studies on grapevine headspace volatiles and/or cuticle allelochemical components. We also showed that aphid response to “Solaris” before the first probe depended on aphid species: A. fabae was more sensitive than M. persicae during the first contact with the plant and responded negatively to cultivar “Solaris”. The value of the parameter describing the onset of probing on plants recorded by electropenetrography is affected by the conditions of the EPG experimental setup. Aphids have limited freedom to move on the plant substrate as they are attached to a golden wire, which is usually 2–3 cm long. It cannot be excluded that aphids would have walked away from the plant if they had been free to move. However, even under the experimental circumstances, the tethered aphids are reluctant to probe in the presence of repellents and no probing prevails in their overall activities (Dancewicz et al. Citation2020). Both A. fabae and M. persicae were rather reluctant to penetrate the deeper leaf tissues of white-berried grapevines. In A. fabae, no probing constituted 20–30% of all activities on “Aurora”, “Bianca” and “Muskat Letni” and 80% on “Solaris” and in M. persicae, no probing amounted to 24% of all activities on “Aurora” and “Bianca” and to 33% on “Solaris”. The individual probes consisted mainly of pathway activity and their duration typically did not exceed 10 minutes in all aphids on all grapevine cultivars, which means that the probes were terminated within non-vascular tissues. It is estimated that when a probe lasts more than 10 minutes, the stylets reach beyond several layers of cells in the leaf and they may reach the vascular bundle within that time (Pettersson et al. Citation2017). In many of the recorded aphids on the leaves of white-berried grapevines, the probes were terminated when stylets were within epidermis: probes shorter than 2 minutes do not reach beyond epidermis (Van Hoof Citation1958). Some of the probes on grapevines were long enough to reach the vascular bundle, at least the xylem vessels, but the success rate was low in all aphids and much lower in A. fabae than in M. persicae. In compatible plant-aphid associations, the prevalence of no probing does not exceed 10–15% of all activities and usually almost all aphids reach phloem vessels and start ingesting phloem sap (Prado & Tjallingii Citation1997; Gabryś & Pawluk Citation1999; Gabryś & Tjallingii Citation2002; Stec et al. Citation2021).
The insertion of stylets into the plant is the beginning of aphid activity within non-vascular tissues, epidermis and parenchyma/leaf mesophyll, called pathway. Pathway consists of progressive movements of stylets in plant apoplast, i.e. in the secondary cell walls, and occasional puncturing of cells adjacent to stylet track for gustatory purposes (Tjallingii & Esch Citation1993; Pettersson et al. Citation2007). During pathway and especially following the evaluation of contents from the probed cells, aphids make decisions whether to continue or terminate probes (Pettersson et al. Citation2017). Therefore, the duration of the pathway probing, the number and duration of individual probes and the success in reaching phloem sieve elements are good indicators of plant suitability for aphids and reflect the presence of factors that either stimulate or deter aphid probing during the pre-ingestive phase (Van Helden & Tjallingii Citation1993; Mayoral et al. Citation1996; Gabryś & Pawluk Citation1999; Gabryś & Tjallingii Citation2002; Philippi et al. Citation2015; Van Emden Citation2017; Wróblewska-Kurdyk et al. Citation2020). On the grapevine cultivars studied, the total duration of pathway activity in A. fabae was similar on all cultivars except on “Solaris”, where it was fivefold shorter than on “Aurora”, “Bianca” and “Muskat Letni”. In M. persicae, the duration of pathway was similar on “Aurora” and “Muskat Letni” and 1.4 and 1.8 times shorter on “Bianca” and “Solaris”, respectively. It is likely that deterrent factors present in grapevine mesophyll, probably of chemical nature, were responsible for premature termination of most probes, that is, before reaching phloem sieve elements. This is often observed in aphids on non-host plants or plants treated with deterrent allelochemicals (Prado & Tjallingii Citation1997; Boratyński et al. Citation2016; Souza et al. Citation2020).
Sometimes, aphid stylets get “derailed” during their route to the vascular bundle and remain “trapped” within plant tissues for various periods of time, which is visualized as EPG waveform “F” (Pettersson et al. Citation2017). The causes of such incidents are unknown but are often attributed to difficulties in penetration (Alvarez et al. Citation2006; Pettersson et al. Citation2017). In the present study, the incidence of “F” was similar in all grapevine cultivars both in A. fabae and M. persicae and the duration of this activity did not differ from the EPG-recorded aphid-plant combinations reported by other authors (Marchetti et al. Citation2009; Stec et al. Citation2021). Another activity in non-vascular tissues, the meaning of which is also unclear and which is reported quite rarely, is the secretion of watery saliva into apoplast, visualized as the EPG waveform “E1e” (Huang et al. Citation2012; Wu et al. Citation2013). Both A. fabae and M. persicae on all grapevines though, showed this activity quite often. The proportion of “E1e” in A. fabae activities was approximately 2.5% of all activities on all grapevine cultivars and in M. persicae, it was 3% on “Aurora” and “Muskat Letni” and 7.4% and 15% on “Bianca”. The total duration of this activity did not differ significantly among cultivars in either aphid species. The frequent occurrence of extracellular watery salivation by aphids on grapevines is difficult to interpret. Aphid watery saliva contains various enzymes such as polyphenoloxidases, peroxidases and oxidoreductases that are associated with detoxication of plant allelochemicals including phenols (Miles Citation1999; Van Bel & Will Citation2016). We hypothesize that aphids probably needed to inactivate allelochemicals which occurred in the apoplast of the grapevine leaf tissues.
When aphid stylets reach the vascular bundle, they can enter either the xylem or the phloem vessels. The xylem serves as the transporting tissue for water and mineral salts (Kim et al. Citation2014). Aphids occasionally ingest the xylem sap for osmoregulation and especially under dehydration stress (Spiller et al. Citation1990; Powell & Hardie Citation2002; Pompon et al. Citation2010; Pettersson et al. Citation2017). Xylem sap ingestion occurred relatively frequently in A. fabae and M. persicae and the proportion of this activity ranged from 2.5% on “Solaris” to 13.3% in “Aurora” in A. fabae and from 5% on “Muskat Letni” to 13.4% on “Solaris” in M. persicae. However, there were no evident differences in the total duration of xylem sap ingestion activity in either A. fabae or M. persicae on the studied white-berried grapevines.
In contrast to the xylem sap, the phloem sap is the major source of nutrients for aphids; therefore the success in reaching phloem vessels is crucial for aphid survival (Douglas Citation2003; Douglas & Van Emden Citation2017). In A. fabae and M. persicae on grapevines, this success was very low. Only approximately 50% of A. fabae and M. persicae on “Aurora”, “Bianca”, and “Muskat Letni” reached phloem vessels but on “Solaris” − 1% of M. persicae and none of A. fabae. The phloem phase starts always with salivation into sieve elements to counter plant defense mechanisms and is followed by passive sap ingestion (Pettersson et al. Citation2017). The sustained, longer than 10 minutes, uninterrupted ingestion of phloem sap by aphids is considered as the acceptance of the plant as a host (Pettersson et al. Citation2017). In almost all aphids that reached sieve elements on all white-berried cultivars, the phloem phase was terminated after short periods of salivation. No ingestion was observed on any grapevine cultivar except in two individuals of A. fabae on “Bianca” and one individual of M. persicae on “Muskat Letni”. At the same time, the duration of phloem sap ingestion by these few aphids was very short.
The identified differences in the probing behavior of aphids prior to the contact with the phloem sap on the white-berried grapevines may relate to the levels of defense mechanisms in the studied cultivars. The EPG experiment and the CVA that followed showed that A. fabae and M. persicae definitely rejected the grapevine “Solaris”, which means that this cultivar is highly resistant to both aphid species. On “Solaris”, probing activity in peripheral tissues was significantly limited in A. fabae and considerably reduced in M. persicae. Grapevine cultivars “Aurora” and “Bianca” are also resistant but the resistance mechanisms seem weaker than in “Solaris”: aphids on these cultivars showed higher success in reaching phloem vessels than on “Solaris” but the duration of phloem phase was very low. In contrast, the cultivar “Muskat Letni” appears only relatively resistant: aphids on this cultivar showed similar success in reaching phloem as on “Aurora” and “Bianca” but the duration of the phloem phase was slightly longer than on those cultivars. Three kinds of plant constitutive defense mechanisms are usually considered when discussing plant resistance to herbivores: antibiosis, antixenosis, and tolerance (Smith Citation2005). Antibiosis occurs when the negative effects of a resistant plant affect the biology of an arthropod attempting to use that plant as a host, without an effect on the insect’s behavior while antixenosis is based on the modification of herbivore behavior by plant factors, which results in the inability of a plant to serve as a host, and tolerance is the ability of a plant to survive the infestation by herbivores and compensate the losses (Smith Citation2005; Le Roux et al. Citation2008; Smith & Chuang Citation2014; Van Emden Citation2017). Antibiosis and antixenosis rely on physical barriers and/or chemical “weapons” – toxins – in plant tissues that have adverse effects on insect biology and/or behavior (Smith Citation2005; Van Emden Citation2017). In our study on the white-berried grapevine cultivars, we concentrated on the effect of different cultivars on aphids. We determined that antibiosis was not the likely cause of grapevine resistance to A. fabae and M. persicae because no aphid consumed plant sap and its effects on aphid biology could not be determined. Nevertheless, the detrimental effect of grapevine sap cannot be excluded. We concentrated on probable antixenosis factors in grapevines and we investigated the internal anatomical structure of the leaves and the content of selected phenolic compounds that are the major line of defense in many plant species, including grapevines (Treutter Citation2006; War et al. Citation2012; Teixeira et al. Citation2013; Singh et al. Citation2021). The leaves of the white-berried grapevine cultivars did not differ in their anatomical structure and no apparent mechanical barriers were observed in their tissues. Therefore, we may assume that physical barriers were not the cause of aphid reluctance to probe beyond the few peripheral layers of cells in the leaves. In contrast, grapevine leaf tissues differed in the qualitative and quantitative content of the analyzed phenolics – flavonoids and stilbenoids. The highest content of analyzed phenolics occurred in “Aurora” and “Solaris”. “Aurora” and “Solaris” contained the highest amount of stilbenoids in relation to “Bianca” and “Muskat Letni”. The main stilbenoids were resveratrol and piceid, the latter being the glycoside of the former and both having high biological activities (Fabris et al. Citation2008; Su et al. Citation2013; Pardal et al. Citation2014; Storniolo et al. Citation2014). Considering that “Aurora” was relatively less resistant to aphids than “Solaris”, we presume that the specificity of ‘Solaris’s allelochemicals may be responsible for the strong antixenosis resistance of this cultivar to A. fabae and M. persicae. We hypothesize that the absolute high content of piceid and its relatively high abundance in the leaves of “Solaris” in comparison to other white-berried grapevine cultivars may discourage the aphids from probing. The data on the effect of resveratrol or piceid against insects are scarce. Resveratrol appeared toxic to fall armyworm Spodoptera frugiperda (Lepidoptera: Noctuidae), and piceid – a feeding deterrent to Japanese termite Reticulitermes speratus (Blattodea: Rhinotermitidae) (Singh et al. Citation2021). Further studies involving the application of artificial diets would be required to clarify the role of piceid and resveratrol as aphid deterrents.
The specificities of aphid behavior on white-berried grapevines described in the present study may have consequences in the transmission of viruses by A. fabae and M. persicae to the individual cultivars. Specifically, virions of non-persistent viruses are acquired and inoculated during brief cell punctures in epidermis and parenchyma, while semi-persistent and persistent viruses require longer duration of aphid stylet penetration to be transferred (Prado & Tjallingii Citation1994; Fereres & Moreno Citation2009; Moreno et al. Citation2012; Fereres & Perry Citation2019). The non-persistent and semi-persistent (= non-circulative) viruses are transmitted on aphid stylets and foregut while the persistent (= circulative) viruses must enter the aphid body through its digestive system to be subsequently inoculated into the plant with the saliva (Katis et al. Citation2007; Brault et al. Citation2010; Fereres & Raccah Citation2015). The transmission on aphid stylets requires just that the cells of plant epidermis and mesophyll are punctured during stylet pathway activities (Katis et al. Citation2007; Fereres & Raccah Citation2015). In this respect, A. fabae and M. persicae may be considered as potential threats to white-berried grapevines because they demonstrated vigorous probing activity in the grapevine leaf peripheral tissues. In contrast, the transmission of circulative viruses requires the ingestion of sap from sieve elements (Katis et al. Citation2007; Fereres & Raccah Citation2015). As both A. fabae and M. persicae reached phloem vessels very rarely and none showed long bouts of sap ingestion, it may be presumed that these aphid species will not be effective in transmitting persistent viruses to grapevines.
In summary, the results of our study demonstrate that white-berried grapevines are not suitable hosts for A. fabae and M. persicae: none of the aphids ingested the phloem sap in a sustained manner, which means that all aphids rejected the grapevines as host plants. There was a clear effect of antixenosis factors in non-phloem tissues of grapevines on aphid behavior. The strongest antixenosis mechanisms occurred in the grapevine cultivar “Solaris” and probably the stilbenoid piceid was responsible for the anti-probing effect. Generally, A. fabae and M. persicae may be considered as potential vectors of some viruses transmitted to grapevines exclusively in a non-persistent and semi-persistent manner.
Author contributions
Conceptualization, M.P., K.D., B.G.; methodology, B.G., K.D., M.D., I.S., M.B., J.B.; formal analysis, M.P., K.D., B.K., M.D., I.S., M.B., J.B.; investigation, M.P., B.K., M.D., I.S., M.B., J.M.; resources, K.D., B.G.; data curation, K.D.; writing − original draft preparation, K.D., B.G.; writing − review and editing, K.D., B.G.; visualization, M.P., M.D., J.B; supervision, K.D., B.G. All authors have read and agreed to the published version of the manuscript.
Disclosure statement
No potential conflict of interest was reported by the author(s).
Data availability statement
The datasets used and/or analyzed during the current study are available from the corresponding author on reasonable request.
Additional information
Funding
References
- Alhmedi A, Bylemans D, Bangels E, Belien T. 2022. Cultivar-mediated effects on apple–Dysaphis plantaginea interaction. Journal of Pest Science 95:1303–1315. DOI: 10.1007/s10340-021-01460-6.
- Aliaño-Gonzalez MJ, Richard T, Cantos-Villar E. 2020. Grapevine cane extracts: Raw plant material, extraction methods, quantification, and applications. Biomolecules 10:1195. DOI: 10.3390/biom10081195.
- Alvarez AE, Tjallingii WF, Garzo E, Vleeshouwers V, Dicke M, Vosman B. 2006. Location of resistance factors in the leaves of potato and wild tuber-bearing Solanum species to the aphid Myzus persicae. Entomologia Experimentalis Et Applicata 121:145–157. DOI: 10.1111/j.1570-8703.2006.00464.x.
- Baroi AM, Popitiu M, Fierascu I, Sărdărescu I-D, Fierascu RC. 2022. Grapevine wastes: A rich source of antioxidants and other biologically active compounds. Antioxidants 11:393. DOI: 10.3390/antiox11020393.
- Bentley WJ, Varela L, Daane KM. 2005. Grapes, insects ecology and control. In: Pimentel D, editor. Encyclopedia of pest management. New York: Taylor & Francis. pp. 1–8.
- Biais B, Krisa S, Cluzet S, Da Costa G, Waffo-Teguo P, Merillon J-M, Richard T. 2017. Antioxidant and cytoprotective activities of grapevine stilbenes. Journal of Agricultural and Food Chemistry 65(24):4952–4960. DOI: 10.1021/acs.jafc.7b01254.
- Biesaga M, Pyrzynska K. 2013. Stability of bioactive polyphenols from honey during different extraction methods. Food Chemistry 136(1):46–54. DOI: 10.1016/j.foodchem.2012.07.095.
- Blackman RL, Eastop VF. 2017. Taxonomic issues. In: van Emden H, Harrington R, editors. Aphids as crop pests. Wallingford, (Oxfordshire, UK); Boston (MA): CAB International. pp. 1–36.
- Boratyński F, Dancewicz K, Paprocka M, Gabryś B, Wawrzeńczyk C, Falabella P. 2016. Chemo-enzymatic synthesis of optically active γ- and δ-decalactones and their effect on aphid probing, feeding and settling behavior. PLoS ONE 11(1):e0146160. DOI: 10.1371/journal.pone.0146160.
- Brault V, Uzest M, Monsion B, Jacquot E, Blanc S. 2010. Aphids as transport devices for plant viruses. Comptes rendus biologies 333(6–7):524–538. DOI: 10.1016/j.crvi.2010.04.001.
- Castillo-Munoz N, Gomez-Alonso S, Garcıa-Romero E, Hermosın-Gutierrez I. 2010. Flavonol profiles of Vitis vinifera white grape cultivars. Journal of Food Composition & Analysis 23:699–705. DOI: 10.1016/j.jfca.2010.03.017.
- Chan CK, Forbes AR, Raworth DA. 1991. Aphid-transmitted viruses and their vectors of the world. Technical Bulletin 1991-3E. Vancouver: Research Branch Agriculture Canada.
- Dancewicz K, Sznajder K, Załuski D, Kordan B, Gabryś B. 2016. Behavioral sensitivity of Myzus persicae to volatile isoprenoids in plant tissues. Entomologia Experimentalis et Applicata 160(3):229–240. DOI: 10.1111/eea.12480.
- Dancewicz K, Szumny A, Wawrzeńczyk C, Gabryś B. 2020. Repellent and antifeedant activities of citral-derived lactones against the peach potato aphid. International Journal of Molecular Sciences 21:8029. DOI: 10.3390/ijms21218029.
- Dogimont C, Bendahmane A, Chovelon V, Boissot N. 2010. Host plant resistance to aphids in cultivated crops: Genetic and molecular bases, and interactions with aphid populations. Comptes rendus biologies 333(6–7):566–573. DOI: 10.1016/j.crvi.2010.04.003.
- Douglas AE. 2003. The nutritional physiology of aphids. Advances in Insect Physiology 31:73–140. DOI: 10.1016/S0065-2806(03)31002-1.
- Douglas AE, Van Emden H. 2017. Nutrition and symbiosis. In: van Emden H, Harrington R, editors. Aphids as crop pests. Wallingford: CABI. pp. 114–131.
- EPG Systems, Wageningen, The Netherlands. 2022. Available: https://www.epgsystems.eu/. Accessed May 2022 13.
- Escudero-Martinez C, Leybourne D, Bos J. 2021. Plant resistance in different cell layers affects aphid probing and feeding behavior during non-host and poor-host interactions. Bulletin of Entomological Research 111(1):31–38. DOI: 10.1017/S0007485320000231.
- Fabris S, Momo F, Ravagnan G, Stevanato R. 2008. Antioxidant properties of resveratrol and piceid on lipid peroxidation in micelles and monolamellar liposomes. Biophysical Chemistry 135(1–3):76–83. DOI: 10.1016/j.bpc.2008.03.005.
- FAOSTAT. 2022. Food and Agriculture Organisation Statistical Database. Available: https://www.fao.org/faostat/en/#data/QCL. Accessed Jul 2022 3.
- Fereres A, Moreno A. 2009. Behavioral aspects influencing plant virus transmission by homopteran insects. Virus Research 141:158–168. DOI: 10.1016/j.virusres.2008.10.020.
- Fereres A, Perry KL. 2019. Movement Between Plants: Horizontal Transmission. In: Palukaitis P, García-Arenal F, editors. Cucumber mosaic virus. St Paul (MN): American Phytopathological Society. pp. 173–184.
- Fereres A, Raccah B. 2015. Plant virus transmission by insects. In: eLS. John Wiley & Sons, Ltd (Ed.). DOI: 10.1002/9780470015902.a0000760.pub3.
- Fuchs M. 2020. Grapevine viruses: A multitude of diverse species with simple but overall poorly adopted management solutions in the vineyard. Journal of Plant Pathology 102:643–653. DOI: 10.1007/s42161-020-00579-2.
- Gabryś B, Pawluk M. 1999. Acceptability of different species of Brassicaceae as hosts for the cabbage aphid. Entomologia Experimentalis et Applicata 91:105–109. DOI: 10.1046/j.1570-7458.1999.00471.x.
- Gabryś B, Tjallingii WF. 2002. The role of singrin in host plant recognition by aphdis during initial plant penetration. Entomologia Experimentalis et Applicata 104:89–93. DOI: 10.1046/j.1570-7458.2002.00994.x.
- Goufo P, Singh RK, Cortez IA. 2020. Reference list of phenolic compounds (including stilbenes) in grapevine (Vitis vinifera L.) roots, woods, canes, stems, and leaves. Antioxidants 9:398. DOI: 10.3390/antiox9050398.
- Hocking AD, Leong SL, Kazi BA, Emmett RW, Scott ES. 2007. Fungi and mycotoxins in vineyards and grape products. International Journal of Food Microbiology 119(1–2):84–88. DOI: 10.1016/j.ijfoodmicro.2007.07.031.
- Holman J. 2009. The aphids and their host plants. In: Host plant catalog of aphids. Online. DOI: 10.1007/978-1-4020-8286-3.
- Huang F, Tjallingii WF, Zhang P, Zhang J, Lu Y, Lin J. 2012. EPG waveform characteristics of solenopsis mealybug stylet penetration on cotton. Entomologia Experimentalis et Applicata 143:47–54. DOI: 10.1111/j.1570-7458.2012.01233.x.
- Kassemeyer HH. 2017. Fungi of grapes. In: König H, Unden G, Fröhlich J, editors. Biology of microorganisms on grapes, in must and in wine. Cham: Springer. pp. 103–132. DOI:10.1007/978-3-319-60021-5_4.
- Katis NI, Tsitsipis JA, Stevens M, Powell G. 2007. Transmission of plant viruses. In: Van Emden H, Harrington R, editors. Aphids as crop pests. Wallingford (Oxfordshire, UK): CAB International. pp. 353–390.
- Keller M. 2015. Botany and anatomy: 1-57. In: The science of grapevines. 2nd ed. Academic Press.
- Kim HJ, Park J, Hwang I. 2014. Investigating water transport through the xylem network in vascular plants. Journal of Experimental Botany 65(7):1895–1904. DOI: 10.1093/jxb/eru075.
- Klingauf FA. 1987. Host plant finding and acceptance. In: Minks A, Harrewijn P, editors. Aphids, their biology, natural enemies and control. Vol. 2B. Amsterdam: Elsevier Sc. Publ. pp. 209–223.
- Le Roux V, Dugravot S, Campan E, Vincent FC, Giordanengo P. 2008. Wild Solanum resistance to aphids: Antobiosis or antixenosis? Journal of Economic Entomology 101:584–591. DOI: 10.1603/0022-0493(2008)101[584:WSRTAA]2.0.CO;2.
- Luo K, Zhao H, Wang X, Kang Z. 2022. Prevalent pest management strategies for grainaphids: opportunities and challenges. Frontiers in Plant Science 12:790919. DOI: 10.3389/fpls.2021.790919.
- Mahalanobis PC. 1936. On the generalized distance in statistics. Proceedings of the National Academy of Sciences, India 12:49–55.
- Marchetti E, Civolani S, Leis M, Chicca M, Tjallingii WF, Pasqualini E, Baronio P. 2009. Tissue location of resistance in apple to the rosy apple aphid established by electrical penetration graphs. Bulletin of Insectology 62(2):203–208.
- Maul E, Töpfer R. 2022a. Vitis International variety catalogue. (www.Vivc.de). Available: https://www.vivc.de/index.php?r=passport%2Fview&id=784. Accessed Jul 2022 3.
- Maul E, Töpfer R. 2022b. Vitis International variety catalogue. (www.Vivc.de). Available: https://www.vivc.de/index.php?r=passport%2Fview&id=20340. Accessed Jul 2022 3.
- Maul E, Töpfer R. 2022c. Vitis International variety catalogue. (www.Vivc.de). Available: https://www.vivc.de/index.php?r=passport%2Fview&id=1321. (www.Vivc.de). Accessed Jul 2022 3.
- Maul E, Töpfer R. 2022d. Vitis International variety catalogue. (www.Vivc.de). Available: https://vinograd.info/sorta/stolovye/myskat-Letnii.html. Accessed Jul 2022 3.
- Mayoral AM, Tjallingii WF, Castanera E. 1996. Probing behavior of Diuraphis noxia on five cereal species with different hydroxyamic acid levels. Entomologia Experimentalis et Applicata 78:341–348. DOI: 10.1111/j.1570-7458.1996.tb00799.x.
- Miles P. 1999. Aphid saliva. Biological Reviews 74:41–85. DOI: 10.1017/S0006323198005271.
- Moreno A, Tjallingii WF, Fernandez-Mata G, Fereres A. 2012. Differences in the mechanism of inoculation between a semi-persistent and non-persistent aphid-transmitted plant virus. The Journal of General Virology 93:662–667. DOI: 10.1099/vir.0.037887-0.
- Ng JCK, Perry KL. 2004. Transmission of plant viruses by aphid vectors. Molecular Plant Pathology 5(5):505–511. DOI: 10.1111/J.1364-3703.2004.00240.X.
- OIV. 2019. International organisation of vine and wine statistical report on world vitiviniculture. Available: https://www.oiv.int/en/statistiques/recherche. Accessed Jul 2022 3.
- Paprocka M, Dancewicz K, Kordan B, Damszel M, Sergiel I, Biesaga M, Mroczek J, Arroyo Garcia RA, Gabryś B. 2023. Probing behavior of Aphis fabae and Myzus persicae on three species of grapevines with analysis of grapevine leaf anatomy and allelochemicals. European Zoological Journal 90(1):83–100. DOI: 10.1080/24750263.2022.2162615.
- Pardal D, Caro M, Tueros I, Barranco A, Navarro V. 2014. Resveratrol and piceid metabolites and their fat-reduction effects in zebrafish larvae. Zebrafish 11(1):32–40. DOI: 10.1089/zeb.2013.0893.
- Pawlus AD, Waffo-Téguo P, Shaver J, Mérillon JM. 2012. Stilbenoid Chemistry from Wine and the Genus Vitis, a review. Journal international des sciences de la vigne et du vin 46(2):57–111. DOI: 10.20870/oeno-one.2012.46.2.1512.
- Pettersson J, Tjallingii WF, Hardie J. 2007. Host-plant selection and feeding. In: Van Emden H, Harrington R, editors. Aphids as crop pests. Wallingford (Oxfordshire, UK): CAB International. pp. 87–113.
- Pettersson J, Tjallingii WF, Hardie J. 2017. Host-plant selection and feeding. In: Van Emden H, Harrington R, editors. Aphids as crop pests. Wallingford (Oxfordshire, UK): CAB International. pp. 173–195.
- Philippi J, Schliephake E, Jürgens H-U, Jansen G, Ordon F. 2015. Feeding behavior of aphids on narrow-leafed lupin (Lupinus angustifolius) genotypes varying in the content of quinolizidine alkaloids. Entomologia Experimentalis et Applicata 156:37–51. DOI: 10.1111/eea.12313.
- Pompon J, Quiring D, Giordanengo P, Pelletier Y. 2010. Role of xylem consumption on osmoregulation in Macrosiphum euphorbiae (Thomas). Journal of Insect Physiology 56(6):610–615. DOI: 10.1016/j.jinsphys.2009.12.009.
- Ponti L, Gutierrez AP, Boggia A, Neteler M. 2018. Analysis of grape production in the face of climate change. Climate 6:20. DOI: 10.3390/cli6020020.
- Portu J, López-Alfaro I, Gómez-Alonso S, López R, Garde-Cerdán T. 2015. Changes on grape phenolic composition induced by grapevine foliar applications of phenylalanine and urea. Food Chemistry 180:171–180. DOI: 10.1016/j.foodchem.2015.02.042.
- Powell G, Hardie J. 2002. Xylem ingestion by winged aphids. Entomologia Experimentalis et Applicata 104:103–108. DOI: 10.1046/j.1570-7458.2002.00996.x.
- Powell G, Hardie J, Pickett JA. 1997. Laboratory evaluation of antifeedant compounds for inhibiting settling by cereal aphids. Entomologia Experimentalis et Applicata 84:189–193. DOI: 10.1046/j.1570-7458.1997.00214.x.
- Powell G, Tosh CR, Hardie J. 2006. Host plant selection by aphids: Behavioral, evolutionary, and applied perspectives. Annual Review of Entomology 51:309–330. DOI: 10.1146/annurev.ento.51.110104.151107.
- Prado E, Tjallingii WF. 1994. Aphid activities during sieveelement punctures. Entomologia Experimentalis et Applicata 72:157–165. DOI: 10.1111/j.1570-7458.1994.tb01813.x.
- Prado E, Tjallingii WF. 1997. Effects of previous plant infestation on sieve element acceptance by two aphids. Entomologia Experimentalis et Applicata 82:189–200. DOI: 10.1046/j.1570-7458.1997.00130.x.
- Rencher AC. 1992. Interpretation of canonical discriminant functions, canonical variates, and principal components. American Statistician 46(3):217–225. DOI: 10.1080/00031305.1992.10475889.
- Reynolds M, Chapman S, Crespo-Herrera L, Molero G, Mondal S, Pequeno DNL, Pinto F, Pinera-Chavez FJ, Poland J, Rivera-Amado C, Saint Pierre C, Sukumaran S. 2020. Breeder friendly phenotyping. Plant Science: An International Journal of Experimental Plant Biology 295:110396. DOI: 10.1016/j.plantsci.2019.110396.
- Sastry KS, Mandal B, Hammond J, Scott SW, Briddon RW. 2019. Encyclopedia of plant viruses and viroids. 1st ed. New Delhi: Springer.
- Seidler-Łożykowska K, Bocianowski J. 2012. Evaluation of variability of morphological traits of selected caraway (Carum carvi L.) genotypes. Industrial Crops & Products 35:140–145. DOI: 10.1016/j.indcrop.2011.06.026.
- Sergiel I, Pohl P, Biesaga M. 2014. Characterisation of honeys according to their content of phenolic compounds using high performance liquid chromatography/tandem mass spectrometry. Food Chemistry 145:404–408. DOI: 10.1016/j.foodchem.2013.08.068.
- Singh S, Kaur I, Kariyat R. 2021. The multifunctional roles of polyphenols in plant-Herbivore interactions. International Journal of Molecular Sciences 22:1442. DOI: 10.3390/ijms22031442.
- Smith CM. 2005. Plant resistance to arthropods. Molecular and conventional approaches. The Netherlands: Springer.
- Smith CM, Chuang WP. 2014. Plant resistance to aphid feeding: Behavioral, physiological, genetic and molecular cues regulate aphid host selection and feeding. Pest Management Science 70:528–540. DOI: 10.1002/ps.3689.
- Souza MF, Davis JA, Ranger C. 2020. Detailed characterization of Melanaphis sacchari (Hemiptera: Aphididae) feeding behavior on different host plants. Environmental Entomology 49(3):683–691. DOI: 10.1093/ee/nvaa036.
- Spiller N, Koenders L, Tjallingii W. 1990. Xylem ingestion by aphids – a strategy for maintaining water balance. Entomologia Experimentalis Et Applicata 55:101–104. DOI: 10.1111/j.1570-7458.1990.tb01352.x.
- Stec K, Kordan B, Gabryś B. 2021. Effect of soy leaf flavonoids on pea aphid probing behavior. Insects 12:756. DOI: 10.3390/insects12080756.
- Stevens M, Lacomme C. 2017. Trensmission of plant viruses. In: Van Emden H, Harrington R, editors. Aphids as crop pests. Wallingford (Oxfordshire, UK): CAB International. pp. 323–361.
- Storniolo CE, Quifer-Rada P, Lamuela-Raventos RM, Moreno JJ. 2014. Piceid presents antiproliferative effects in intestinal epithelial Caco-2 cells, effects unrelated to resveratrol release. Food & Function 5:2137–2144. DOI: 10.1039/C4FO00305E.
- Su D, Cheng Y, Liu M, Liu D, Cui H, Zhang B, Zhou S, Yang T, Mei Q, Mukhopadhyay P. 2013. Comparision of piceid and resveratrol in antioxidation and antiproliferation activities in vitro. PLoS ONE 8(1):e54505. DOI: 10.1371/journal.pone.0054505.
- Teixeira A, Eiras-Dias J, Castellarin SD, Gerós H. 11 2013. Berry phenolics of grapevine under challenging environments. International Journal of Molecular Sciences 14(9):18711–18739. DOI: 10.3390/ijms140918711.
- Tjallingii WF. 1994. Sieve element acceptance by aphids. European Journal of Entomology 91:47–52.
- Tjallingii WF. 2006. Salivary secretions by aphids interacting with proteins of phloem wound responses. Journal of Experimental Botany 57(4):739–745. DOI: 10.1093/jxb/erj088.
- Tjallingii WF, Esch THH. 1993. Fine structure of ahid stylet routes in plant tissues in correlation with EPG signals. Physiological Entomology 18:317–328. DOI: 10.1111/j.1365-3032.1993.tb00604.x.
- Treutter D. 2006. Significance of flavonoids in plant resistance: A review. Environmental Chemistry Letters 4:147. DOI: 10.1007/s10311-006-0068-8.
- Van Bel AJE, Will T. 2016. Functional evaluation of proteins in watery and gel saliva of aphids. Frontiers in Plant Science 7:1840. DOI: 10.3389/fpls.2016.01840.
- Van Emden HF. 2017. Host-plant resistance. In: Van Emden H, Harrington R, editors. Aphids as crop pests. Wallingford (Oxfordshire, UK): CABI. pp. 515–532.
- Van Helden M, Tjallingii WF. 1993. Tissue localisation of lettuce resistance to the aphid Nasonovia ribisnigri using electrical penetration graphs. Entomologia Experimentalis et Applicata 68(3):269–278. DOI: 10.1111/j.1570-7458.1993.tb01713.x.
- Van Hoof HA. 1958. An investigation of the biological transmission of a non-persistent virus. Wageningen: The Agricultural University.
- Vincent C, Isaacs R, Bostanian NJ, Lasnier J. 2012. Principles of arthropod pest management in vineyards. In: Noubar J, Bostanian C, Isaacs R, editors. Arthropod management in vineyards: Pests, approaches, and future directions. Dordrecht-Heidelberg-New York-London: Springer. pp. 1–16.
- Vitulo N, Lemos Jr WJF, Calgaro M, Confalone M, Felis GE, Zapparoli G, Nardi T. 2019. Bark and grape microbiome of Vitis vinifera: Influence of geographic patterns and agronomic management on bacterial diversity. Frontiers in Microbiology 9:3203. DOI: 10.3389/fmicb.2018.03203.
- Wamonje FO, Donnelly R, Tungadi TD, Murphy AM, Pate AE, Woodcock C, Caulfield J, Mutuku JM, Bruce TJA, Gilligan CA, Pickett JA, Carr JP. 2020. Different plant viruses induce changes in feeding behavior of specialist and generalist aphids on common bean that are likely to enhance virus transmission. Frontiers in Plant Science 10:1811. DOI: 10.3389/fpls.2019.01811.
- War AR, Paulraj MG, Ahmad T, Buhroo AA, Hussain B, Ignacimuthu S, Sharma HC. 1 2012. Mechanisms of plant defense against insect herbivores. Plant Signaling & Behavior 7(10):1306–1320. DOI: 10.4161/psb.21663.
- Will T, Tjallingii WF, Thönnessen A, van Bel AJ. 2007. Molecular sabotage of plant defense by aphid saliva. Proceedings of the National Academy of Sciences USA 104(25):10536–10541. DOI: 10.1073/pnas.0703535104.
- Wróblewska-Kurdyk A, Dancewicz K, Gliszczyńska A, Gabryś B. 2020. New insight into the behavior modifying activity of two natural sesquiterpenoids farnesol and nerolidol towards Myzus persicae (Sulzer) (Homoptera: Aphididae). Bulletin of Entomological Research 110(2):249–258. DOI: 10.1017/S0007485319000609.
- Wu D, Zeng L, Zhou A, Xu Y. 2013. Effects of Solenopsis invicta (Hymenoptera: Formicidae) tending on the probing behavior of Phenacoccuss olenopsis (Hemiptera: Pseudococcidae). The Florida Entomologist 96(4):1343–1349. DOI: 10.1653/024.096.0413.
- Zhang Y, Fan J, Francis F, Chen J. 2017. Watery saliva secreted by the grain aphid Sitobion avenae stimulates aphid resistance in wheat. Journal of Agricultural and Food Chemistry 65(40):8798–8805. DOI: 10.1021/acs.jafc.7b03141.
- Züst T, Agrawal A. 2016. Mechanisms and evolution of plant resistance to aphids. Nature Plants 2:15206. DOI: 10.1038/nplants.2015.206.