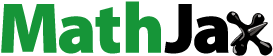
Abstract
The round goby (Neogobius melanostomus) is an invasive species that can be used as a raw material in the food industry and also as a bioindicator of heavy metal pollution in the environment. In this study, Cd, Co, Cr, Mo, Ni, and Pb were determined in water, bottom sediment, muscle, liver, gills, and gonads of round goby from Dąbie Lake, Szczecin Lagoon, and Puck Bay (all in southern Baltic Sea) using atomic absorption spectrometry. Fulton’s condition factor, gonadal and hepatosomatic indices (GSI, HSI) were calculated. Based on estimated daily intake (EDI), target hazard quotient (THQ), and carcinogenic risk (CR), we assessed the human health risk related to the consumption of heavy metals in the round goby. The fish had length and weight ranges of 15.0–16.7 cm and 55.3–80.9 g, respectively. Fulton’s condition factor ranged from 1.26 to 2.48 and was highest (p < 0.05) for fish from Dąbie Lake. The range of metal concentrations (in μg kg−1) ranged from 2.92 (Cd in muscles of fish from Szczecin Lagoon) to 106.4 (Ni in muscles of fish from Dąbie Lake). The highest concentrations of Cd, Co, Mo, and Pb were found in livers, Ni in muscles and gonads, and Cr in gills. The calculated EDIs were below the reference oral dose (RfD) and maximum for Cd (1.6% RfD) and Pb (15.1% RfD). The hazard quotient was less than 1. In conclusion, the round goby has a higher potential to accumulate metals from water than from bottom sediments. Consumption of its muscle should not pose a health risk (cancer risk can be considered acceptable). Due to Pb accumulation, moderate consumption should be recommended for pregnant women and children. Finally, our results indicate that the round goby (especially its liver) can be used as a bioindicator to monitor metal contamination of aquatic ecosystems.
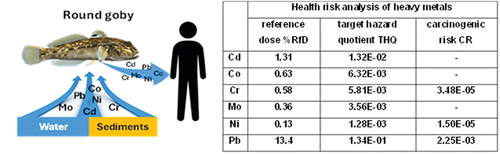
1. Introduction
Trace heavy metals include both superfluous metals with toxic properties (Cd and Pb) and those essential for the normal functioning of the body but harmful in excess (e.g. Co, Cr, Mo, Ni). The growing concern is the increasing availability of both types of heavy metals to organisms due to the progressive environmental pollution recorded in all regions of the world. In addition, toxic heavy metals can bioaccumulate in the trophic network, ultimately threatening human health. Therefore, to assess the state of the environment and the directions of the flow of heavy metals through the trophic network, we should constantly monitor the pollution of each element of the ecosystem (Briffa et al. Citation2020).
Fish are the main consumers in the trophic pyramid of the aquatic ecosystem and are particularly vulnerable to the accumulation of heavy metals ingested with food, dissolved in water, and deposited in bottom sediments. On one hand, fish containing metals in concentrations above the allowable limit are considered unsafe for consumption (Garai et al. Citation2021) but research on heavy metal levels in fish can be used also to monitor the pollution of the aquatic environment (Canpolat et al. Citation2016; Ai et al. Citation2022).
Heavy metal levels are usually measured in fish muscle (the main food product) and only sometimes in whole fish and individual metabolizing organs such as gonads, gills, and liver. However, muscle generally accumulates lower concentrations of metals, and results based on whole-body analysis may inaccurately assess trophic transfer in different tissues, especially metabolically active organs, such as the liver (Gao et al. Citation2021; Cordeli et al. Citation2023).
Toxicological studies mainly focus on commercially fished species. However, to meet the needs of environmental monitoring, it is useful to select mandatory indicator species. It is assumed that a bioindicator should be a sedentary species that is common in the ecosystem, easily adaptable to a new environment, and resistant to pollution (Linde-Arias et al. Citation2008). Particularly useful are invasive species, whose numbers can be limited via intensive fishing and use in the food industry (Czerniejewski et al. Citation2023). One such species is the round goby (Neogobius melanostomus), a representative from the family Gobiidae which often have numerous populations and live in easily accessible coastal waters (Czerniejewski & Brysiewicz Citation2018; Omidi et al. Citation2020). This highly invasive fish first appeared in Europe in 1990 (Hel Peninsula, southern Baltic Sea) (Skóra & Stolarski Citation1993). It has rapidly expanded to reach almost all of the Baltic Sea and many other water bodies in Europe. It is also currently establishing large populations in Asia Minor and North America (Sapota Citation2004; Oesterwind et al. Citation2017; Rakauskas et al. Citation2020).
In this study we (i) analyzed the accumulation of trace heavy metals (Cd, Co, Cr, Mo, Ni, and Pb) in muscles, gills, livers, and gonads of an invasive fish round goby (Neogobius melanostomus) from three water bodies in the southern Baltic Sea; (ii) compared the potential of fish muscles and organs to accumulate heavy metals from water and bottom sediments (bioconcentration factor BCF); and (iii) assessed human health risks associated with the consumption of round goby muscles.
2. Methods
2.1. Characteristics of the studied water bodies
The study was conducted in three water bodies in the southern part of the Baltic Sea catchment area, namely Dąbie Lake, Szczecin Lagoon, and Puck Bay (). The common characteristics of these water bodies are relatively shallow average depth and high anthropopressure, but they differ in the impact of seawater on their chemical conditions.
Figure 1. Location of water, bottom sediment, and fish sampling sites from Dąbie Lake, Szczecin Lagoon and Puck Bay.
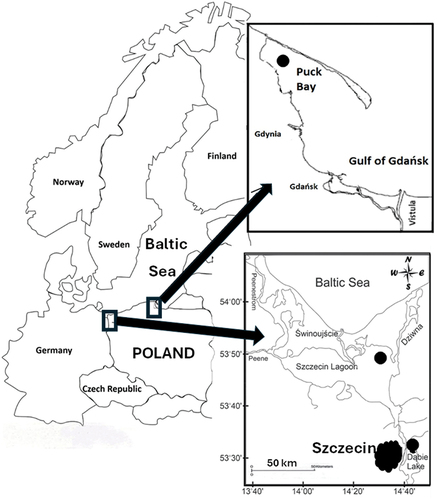
Dąbie Lake (tertiary estuary of the Oder River, area 56 km2, average depth 2.61 m, maximum 7 m). The lake flows directly into the East Oder and is connected to the West Oder by a network of channels. In the northern area, the lake is connected by a wide channel to the southern end of the Szczecin Lagoon. The entire lake is located within the administrative boundaries of the Szczecin agglomeration, which has a strong impact on its ecological status, contributing to the high trophic state index of the reservoir and also polluting the waters with heavy metals (Nędzarek et al. Citation2007, Citation2007; Daniszewski Citation2013).
The Szczecin Lagoon (secondary estuary of the Oder River, area 687 km2, mean depth 3.8 m, maximum 8.5 m) is mainly fed by the waters of the Oder River (about 17 km3 per year, accounting for 94% of the lagoon’s water budget). It is connected to the Baltic Sea by the Peenestrom Strait, and Świna and Dziwna Rivers, and contains an artificially dredged shipping channel (10.5 m deep) connecting the ports of Świnoujscie and Szczecin. The bottom of its shallow areas is covered with sand and a thin layer of organic silt, and at depths of over 4 m the sediments are muddy. The salinity of the basin varies from 0.5 to 2 PSU, during the inflow of Baltic waters it exceeds 6 PSU. The fish fauna consists of freshwater and brackish water species (e.g. roach, perch, zander, bream, ruffe), migratory species (e.g. eel, sea trout), and marine species (e.g. herring) (Glasby et al. Citation2004; Bangel et al. Citation2004; Radziejewska & Schrenewski Citation2008; Schrenewski et al. Citation2019).
Puck Bay forms the northwestern part of the Gulf of Gdańsk (about 1.4% of its area) and is divided into a shallow inner part (average depth of 3.1 m, maximum 9.4 m) and an outer part (average depth of 20.5 m, maximum 54 m). Puck Bay is separated from the Baltic Sea by the Hel Peninsula in the north and is open to the Gulf of Gdańsk in the east, resulting in water salinity ranging from 7.31 to 7.65 PSU. Hydrological and hydrochemical conditions are mainly determined by saline water inflows from the Gulf of Gdańsk, into which the Vistula River flows (the largest river in the region, with an average flow of more than 1,000 m3 s−1). The waters and bottom sediments of Puck Bay are classified as moderately contaminated with heavy metals (Szefer et al. Citation1998; Glasby et al. Citation2004; Dybowski et al. Citation2020).
2.2. Characteristics of the round goby
The round goby (N. melanostomus) is a Ponto-Caspian benthophilous species that originally inhabited mainly fresh and brackish waters (rivers, estuaries) and coastal areas of the Black, Azov, and Caspian Seas (Pinchuk et al. Citation2003). Today, it is one of the most common invasive fishes in the world (Rakauskas et al. Citation2020). The success of the species as an invader is mainly attributed to its ecological plasticity. The aggressive behavior of the round goby helps it compete with native fish species for habitat and food, e.g. by feeding on their eggs and offspring (Matern et al. Citation2021).
Reductions in filter feeders also affect algal biomass, leading to changes in community composition and alterations in trophic relationships (Rakauskas et al. Citation2020).
2.3. Fish, water, and bottom sediments sample collection
Fish for research were obtained from professional fishermen in June and July 2022. Sixteen fish (8 females and 8 males each) were taken from each water body. Immediately after capture, the fish were weighed on an Axis 2000 electronic balance (±0.1 g), and the total length (TL) and standard length (SL) were measured using an electronic caliper (±0.1 mm). The fish were then stored in clean labelled polythene bags and transported in ice bags to the laboratory for further analyses of the metal content in their tissues. Fish aged from 3+ to 5+ years were used in the study. These were mainly fish aged 4+, accounting for 68.75% of fish from Puck Bay and over 75.00% of fish from Dąbie Lake and Szczecin Lagoon. The proportion of fish aged 3+ was 18.75% for fish from Dąbie Lake and 25.00% for fish from Szczecin Lagoon and Puck Bay. Fish aged 5+ were only caught in Dąbie Lake and Puck Bay, accounting for 6.25% of the examined fish. The age of the fish was determined from the annual rings on the scales (Grula et al. Citation2012).
At the same time, 3 water samples were collected from 0.5 m depth in the fishing area using a Ruttner Water Sampler with integrated thermometer (KC-Denmark A/S), and 4 bottom sediment samples were collected using a 2500 cm2 Van Veen Grab Sampler (KC-Denmark A/S).
2.4. Dissection of sampled fish
At the laboratory, gills, gonads, liver, and muscle were removed from each fish, weighed and frozen at −20°C until heavy metal content was determined. The dissection (according to the method of Habib et al. (Citation2024)) was performed on a plastic tray with the help of a sterilized porcelain knife.
2.5. Condition indexes
The Fulton’s condition factor (K) was calculated for each fish according to the following formula (Ritterbusch-Nauwerck Citation1995):
where: W – weight of fish (in g), TL – total length of fish (in cm). In addition, the gonadosomatic index (GSI) and hepatosomatic index (HSI) were determined, according to the following formulas (Nunes et al. Citation2011):
where: Wg – the weight of gonads (in g), WH – the weight of liver (in g), W – the weight of fish (in g).
2.6. Elemental analysis
Water samples were digested with HNO3 at a volume ratio of 10:1 at 100°C. Bottom sediments, after drying to a constant weight at 90°C, were sieved through a 2 mm sieve. The bottom sediment fraction thus obtained was digested with concentrated HNO3 at a ratio of 5 g dry weight of sediment to 10 mL of HNO3. Digestion time 30 min, temperature 200°C. The solution obtained was diluted to 25 mL.
Muscle, gill, and liver samples (1.00 ± 0.01 g wet weight) and gonad samples (0.1 ± 0.01 g wet weight) were digested in 6.0 mL of a mixture of HNO3 and HClO4 (volume ratio 5:1). After digestion, the samples were diluted with water to a total volume of 25 mL. Samples were digested in a high-pressure microwave digester (Speedwave Xpert, Berghof, Eningen, Germany). Ultrapure-concentrated HNO3 and HClO4 (Merck, Germany) and Milli-Q (18.2 MΩ) water were used.
Heavy metals (Cd, Co, Cr, Mo, Ni, Pb) were determined by graphite furnace atomic absorption (GFFA) using a Hitachi ZA3000 series polarized Zeeman atomic absorption spectrometer (Hitachi High Technologies Corporation, Tokyo, Japan). Calibration curves were established using certified standard solutions (1000 mg L−1) from Merck (Germany).
2.7. Quality control and quality assurance
Reagent contamination was checked using reagent blanks. A spike-and-recovery test was performed on random water, bottom sediment, and fish samples to verify the accuracy and precision of the analytical method and digestion. The accuracy of the analytical method was tested using the dogfish liver NCR-DOLT-5 reference material (National Research Council Canada). Elemental recoveries were 95% (for Cd), 102% (for Co and Cr), 96% (for Mo and Pb) and 110% (for Ni).
2.8. Bioconcentration factor
The accumulation potential of heavy metals in the fish tissue was referenced as bioconcentration factor (BCF). According to Jitar et al. (Citation2015) and Vrhovnik et al. (Citation2013), BCF is defined as follows:
where: Cfish tissue – concentration of heavy metal in the fish gills, muscles, liver, or gonads (in mg kg−1); C – concentration of heavy metal in water (in mg L−1) or bottom sediments (in mg kg−1).
2.9. Estimated daily intake
The estimated daily intake (EDI; in mg kg−1 day−1) of heavy metals through the consumption of fish muscle was calculated using the following equation (Nędzarek & Czerniejewski Citation2022; Zhou et al. Citation2021):
where: Ci is the element concentration in the fish muscle (mg kg−1 wet weight); IR is the fish muscle ingestion rate (0.03 kg day−1); and BW is the average body weight (70 kg for adults).
The resulting EDI values were compared with the reference (safe) oral dose of the element (RfD) (Li et al. Citation2014; US EPA Citation2023). According to the NYS DOH (Citation2007), if the ratio of the EDI value of an element to the RfD value is equal to or less than RfD, the risk will be at a minimum level; if it is 1–5 times more than the RfD, the risk is low; if it is 5–10 times more than the RfD, the risk is low; if it is 10 times more than the RfD, the risk will be high.
2.10. Human health risk assessment
The assessment of risk associated with consumption of heavy metals contained in fish muscle followed the procedure of Tan et al. (Citation2021) and US EPA (Citation2000, Citation2023). The non-carcinogenic target hazard quotient (THQ) was calculated by the equation:
where: EF is the exposure frequency (365 days year−1); ED is the exposure duration equivalent to the average human lifetime (70 years); IR is the fish muscle ingestion rate (30 g day−1); Ci is the element concentration in the fish muscle (mg kg−1); RfD is the oral reference dose for contaminant (mg kg−1 day−1); BW is the average body weight (70 kg for adults); and AT is the exposure time for non-carcinogens (365 days year−1 ED). THQ represents the ratio of exposure level to a substance over a specified period to the reference dose (RfD) of that particular substance. Thus, THQ ≥ 1 indicates potential health hazards associated with the consumption of certain foods.
To evaluate the potential risk of adverse health effects from a mixture of elements the hazard index (HI) was calculated as the sum of THQ for each element:
The carcinogenic risk (CR) was calculated for Cr, Ni, and Pb, using the formula:
where the parameter has been defined to formula 6, except for CSF, which is the cancer slope factor set by the US EPA (Citation2023). According to the US EPA (Citation2000), a lifetime cancer risk under 1E–06 is negligible, a risk over 1E–04 is unacceptable, and a range of 1E-06–1E-04 is acceptable.
For the EDI and THQ calculations, we used the average element concentration in each of the compared fish muscle samples.
2.11. Statistical analysis
The statistical difference among the detected heavy metals of water, bottom sediments, and different fish organs (gills, muscles, liver, and gonads) was assessed through one-way ANOVA using the Statistica v13.3 software from TIBCO Software Inc. Biological traits between females and males were compared using the Mann-Whitney U test. The significance of differences (p < 0.05) was verified using Tukey’s post hoc test. Pearson correlation coefficient (r) for the interdependence of the determined heavy metals in the body parts of fish was also used. Moreover, to identify the underlying components in the data and to assess the association between the metals Principal Component Analysis (PCA) was applied.
3. Results
3.1. Water and bottom sediments
Metal concentrations in water (in μg L−1) ranged from 0.026 (for Cd in Dąbie Lake) to 2.177 (for Ni in Szczecin Lagoon).In bottom sediments, metal concentrations (in mg kg−1) ranged from 0.011 (for Cd in Puck Bay sediments) to 5.368 (for Pb in Dąbie Lake) ().
Table I. Comparative analysis of heavy metals (mean ± standard deviation) detected in water and bottom sediments of Dąbie Lake, Szczecin Lagoon, and Puck Bay.
Significant (p < 0.05) differences in concentrations of selected metals between water bodies were also found. Mo was highest in Szczecin Lagoon water, Cd, Cr, Ni, and Pb in Dąbie Lake and Szczecin Lagoon, and Co in Szczecin Lagoon and Puck Bay ().
In contrast, significantly (p < 0.05) highest concentrations of heavy metals were found in sediments from Dąbie Lake and significantly (p < 0.05) lowest concentrations in sediments from Puck Bay. Sediments from the Szczecin Lagoon had significantly different concentrations of Cd, Co, Mo, and Pb from the other basins (they can be considered intermediate with respect to sediments from Dąbie Lake and Puck Bay). In contrast, Cr and Ni concentrations from this basin were not significantly higher (p > 0.05) than those recorded in sediments from Puck Bay.
3.2. Biological characteristics of the studied fish
Morphometric data, Fulton’s condition factor (K), gonadosomatic index (GSI), and hepatosomatic index (HSI) for the round goby are summarized in . The range of total length was 14.00–18.90 cm, and only for fish from Puck Bay was there a significantly (p < 0.05) higher body length of males than females.
Table II. Comparative analysis of round goby (average ± standard deviation) between Dąbie Lake, Szczecin Lagoon, and Puck Bay (F – female; M – male; GSI – gonadosomatic index; HIS – hepatosomatic index).
The round goby body length did not differ significantly (p > 0.05) by water body. The unit weight of fish ranged from 38.75–100.14 g, with fish from Dąbie Lake and Szczecin Lagoon being significantly heavier (p < 0.05). The K-factor ranged from 1.26 to 2.48, and significantly (p < 0.05) fish from Dąbie Lake had the highest value. Fish had different values of GSI (0.26 to 2.12) and HSI (1.86 to 2.74) indices. The highest values (p < 0.05) of the GSI index were recorded in fish from Dąbie Lake and Puck Bay. In contrast, fish from the Szczecin Lagoon had significantly (p < 0.05) higher HSI values. These two parameters differentiated between female and male fish only in Szczecin Lagoon (GSI significantly higher in males – Mann-Whitney U test Z value = 2.025234, p = 0.040093) and Puck Bay (HSI significantly higher in females – Mann-Whitney U test Z value = −1.94289, p = 0.049883).
3.3. Heavy metals in fish
Detailed results of heavy metal concentrations in round goby by sex are presented in Supplementary Material (Table S1).
It was shown that there were no significant differences (p > 0.05) in the concentrations of heavy metals in different parts of the body of the fish by sex, and such division was not taken into account in further consideration (the results thus compiled are presented in ).
Table III. Comparison of heavy metals (average ± standard deviation; concentration in g kg−1) between the studied organs of fish from Dąbie Lake, Szczecin Lagoon, and Puck Bay.
In general, the averaged concentrations (in μg kg−1) of heavy metals in the round goby were lowest for Co (13.27) and Cd (14.17) and about three times higher for the other elements, i.e. 41.11 for Cr, 43.25 for Mo, 44.21 for Ni, and 42.09 for Pb.
The range of metal concentrations (in μg kg−1) was wider, ranging from 2.92 to 106.4 (Cd and Ni in the muscles of Szczecin Lagoon and Dąbie Lake fish, respectively) (). Based on the average values in different parts of the fish body, the metals can be grouped into the following concentration ranges ():
- < 10 μg kg−1: Co (in muscle, gonads, and gills), Cd (in muscle and gonads);
- 11 – 30 μg kg−1: Cd, Mo, Cr (gonads), Pb (gills), Cd, Ni, Cr, Co (liver);
- 31 – 50 μg kg−1: Cr, Mo, Pb (muscle), Mo, Ni (gills), Pb, Ni (gonads);
- > 51 μg kg−1: Ni (muscle), Pb, Mo (liver), Cr. (gills)
No consistent trend of metal concentrations in the fish body was observed. However, the following individual series can be indicated ():
Cd: muscle ≤ gills < gonads < liver;
Co: muscle < gonads ≤ gills < liver;
Cr: gonads < liver < muscle < gills;
Mo: gonads < gills ≤ muscle < liver;
Ni: liver ≤ gills < gonads ≤ muscle;
Pb: gills < gonads ≤ muscle ≤ liver.
As can be seen, the liver was the organ with significantly (p < 0.05) the highest concentrations of Cd, Co, Mo, and Pb. Muscle was another “concentrator” of metals, with significantly (p < 0.05) the highest accumulation of Ni, and similar to gonads accumulated Pb and similar to gills accumulated Mo. Gills accumulated significantly (p < 0.05) the most Cr. In contrast, gonads accumulated the least heavy metals, especially Cr and Mo, but also Co and Pb. Gonads had relatively high concentrations of Ni (not significantly less than muscle) and Cd (concentration significantly lower than liver Cd concentration) ().
In the PCA analysis, the cumulative variances of the first two components (PC1 and PC2) were 60.0% and 71.3% (). For Dąbie Lake, PC1 was positively loaded with Cd (0.92) and Co (0.93) and PC2 was negatively loaded with Mo (−0.72), Ni (−0.66), and Cr (−0.49).
For Szczecin Lagoon, PC1 was positively loaded with Cd (0.69), Co (0.86), Mo (0.78), and Pb (0.79) and PC2 was negatively loaded with Cr (−0.69).
For Puck Bay, PC1 was positively loaded with Cd (0.85), Co (0.93), and Mo (0.89) and PC2 was negatively loaded with Cr (−0.84). Based on these two principal components, the differentiation of different parts of the body of the fish according to the content of the elements determined was shown. In each basin, the liver stood out compared to the other parts of the fish’s body. At the same time, in the livers of fish from Dąbie Lake and Szczecin Lagoon, most of the elements were positively correlated with each other (0.53 < r < 0.85). However, in the livers of fish from Puck Bay, significant correlations were found only for Co-Ni (r = 0.9), Co-Pb (r = 0.61), and Ni-Pb (r = 0.77) (Table S2). In the muscles of fish from Dąbie Lake, all elements were correlated with each other (0.61 < r < 0.88). In contrast, in the muscles of fish from Szczecin Lagoon and Puck Bay, such mutual correlations were much lower. Gonads and gills showed the lowest mutual correlations between elements (Table S2).
When comparing the same body parts of the fish, there was mostly no significant (p > 0.05) variation in heavy metal concentrations due to the water body in which the fish were caught. However, Dąbie Lake stood out – fish from this water body had significantly (p < 0.05) highest concentrations of Mo and Pb in gills, Cd, Co, Cr, and Ni in muscles, Cd, Ni, and Pb in livers, and Cd, Co, Cr, and Pb in gonads. Only the concentrations of Cd in muscles and gonads and Pb in livers of Szczecin Lagoon fish were not significantly different from their concentrations in these body parts of Dąbie Lake fish ().
shows Pearson’s linear correlation coefficients for the dependence of metal concentrations on the weight of fish body parts. Most metals in fish muscles were significantly correlated with fish weight (0.56 < r < 0.89) (except Mo – Puck Bay and Co – Szczecin Lagoon). Almost all metals contained in gonads were not correlated with gonad weight (except Co – Dąbie Lake). However, metals contained in the liver were correlated with the weight of this organ in fish from Szczecin Lagoon (0.55 < r < 0.78). For fish from Puck Bay, such correlations were shown for Co, Cr, and Pb, and for fish from Dąbie Lake only for Cr.
Table IV. Pearson’s correlation coefficients (r) for the dependence of metal concentrations in muscle, liver, and gonads on body weight, liver weight, and gonad weight of fish from Dąbie Lake (DL), Szczecin Lagoon (SL) and Puck Bay (PB), respectively.
3.4. Bioconcentration factor
The values of bioconcentration factors of metals determined against bottom sediments (BCFBS) were in the range of 0.003–2.259 and those determined against water (BCFW) were in the range of 8.5–985 ().
Table V. Bioconcentration of heavy metals in different fish tissues (BFCW – Bioconcentration factor for water; BCFBS – Bioconcentration factor for bottom sediments).
For most elements, BCFBS was less than 0.06. Higher values of this factor were found for Cd (often BCFBS >0.1) and Mo (often BCFBS >0.2). The highest BFCBS values were also found for these elements (1.223 and 2.259 for Cd in gonads and livers of Puck Bay fish, respectively; 0.656 and 1.306 for Mo in muscles and livers of Puck Bay fish, respectively). When comparing the reservoirs, BCFBS was the highest (p < 0.05) for fish from Puck Bay and lowest for fish from Dąbie Lake ().
BCFW values were mostly below 50. BCFW >100 was found for Cd (gills, liver, gonads) and also for Co and Pb (liver). Extremely high BCFW were found for Cd in the livers and gonads of fish from Dąbie Lake (985 and 455.1), Szczecin Lagoon (473.1 and 446.9), and Puck Bay (955.7 and 517.2). When comparing water bodies, it should be noted that the statistically significantly lowest BCFW values (p < 0.05) were always found for fish from Szczecin Lagoon and more often for fish from Dąbie Lake than for fish from Puck Bay ().
3.5. Human health risk assessments
The estimated daily intake (EDI, in mg kg−1 day−1) of heavy metals from the consumption of 30 g of fish muscle ranged from 1.10E–06 (for Cd, fish from Puck Bay) to 4.56E–05 (for Ni, fish from Dąbie Lake). At the same time, higher EDIs for all metals were recorded for fish muscle from Dąbie Lake ().
Table VI. Health risk analysis of heavy metals: estimated daily intake (EDI) and comparison with reference dose (%RfD), target hazard quotient (THQ), hazard index (HI), carcinogenic risk (CR) and total carcinogenic risk (ΣCR) for mean concentration of elements in the fish muscle from Dąbie Lake, Szczecin Lagoon and Puck Bay.
The EDI values were mostly less than 1% of the RfD. They were minimal for Ni (from 0.07 to 0.12%RfD) and much higher for Cd (from 1.10 to 1.60%RfD) and Pb (from 11.7 to 15.1%RfD). The highest EDI values were found for fish from Dąbie Lake ().
The target hazard quotient (THQ) ranged from 7.48E–04 (for Ni, Szczecin Lagoon) to 1.51E–01 (for Pb, Dąbie Lake). Comparing this parameter with the RfD, only the intake of Mo and Ni with fish muscle did not exceed the reference dose. In contrast, the hazard index (calculated as ) did not exceed the value of 2E–01 and was slightly lower for fish from Puck Bay and higher for fish from Dąbie Lake ().
The carcinogenic risk (CR) was determined taking into account the intake of Cr, Ni, and Pb contained in fish muscle (). The lowest CR values were found for Ni (6.16E–04 and 6.63E–04 for fish from Szczecin Lagoon and Puck Bay, respectively) and the highest for Pb (from 1.38E–01 to 1.78E–01, Puck Bay and Dabbie Lake, respectively). Considering the summed carcinogenic risk, the lowest value was found for fish from Puck Bay (1.40E–01) and the highest for fish from Dąbie Lake (1.83E–01) ().
4. Discussion
4.1. Water and bottom sediments
The water bodies studied have a shallow average depth and a large surface area, and under favorable conditions (e.g. strong winds) mixing of water masses can reach the bottom. These waters are strongly influenced by the waters of the Vistula (Puck Bay) and the Oder (Dąbie Lake, Szczecin Lagoon) (Radziejewska & Schrenewski Citation2008; Dybowski et al. Citation2020). The water quality and heavy metal contamination of the bottom sediments of these rivers has improved in recent decades (Helios-Rybicka et al. Citation2005; Jaskuła & Sojka Citation2022), which has had a positive impact on the environmental status of these basins. Nevertheless, the waters and bottom sediments of Dąbie Lake and Szczecin Lagoon (which are adjacent elements of the Oder estuary) have higher levels of heavy metals than Puck Bay, which is open to the direct influence of marine waters. An important factor in this difference may be, as suggested by Kucharski et al. (Citation2022), the shipbuilding industry, which releases metals into the environment during ship maintenance. On the other hand, the waters of the investigated water bodies meet the applicable environmental quality standards contained in the Regulation of the Minister of Infrastructure of 2021 on the classification of the ecological status, ecological potential, and chemical status of surface waters (Regulation Citation2021). The following concentrations of Cd < 0.2, Ni < 8.6 and Pb < 1.3 (in μg dm−3) are allowed for transitional and coastal waters.
4.2. Fish size
The studied round gobies were characterized by body sizes typical for this species outside its natural habitat. For example, in the Gulf of Gdańsk the round goby grows to about 18 cm (Sapota Citation2004), in the Danube basin to 15.3 cm (Slovakia), 15 cm (Austria), and 16 cm (Hungary) (Polačik et al. Citation2008; Grula et al. Citation2012). In contrast, round goby grows up to 25.0 cm in waters within their natural range (Berg Citation1949). This was also true for their weight, which varied between populations but was within the range most commonly reported in the literature. For example, round goby from the Danube River (Slovakia) were characterized by weights up to 69 g (Grula et al. Citation2012), and fish from the Azov Sea had weights in the range of 48–91 g at a length of 12–15 cm (Mazygula et al. Citation2019) and 29–32 g at a length of 12–14 cm (Sigacheva & Skuratovskaya Citation2022), and fish from the Black Sea were 60 g at a length of 16 cm (Stancheva et al. Citation2013). Also, previous studies on the population structure of round goby in Szczecin Lagoon (Czerniejewski & Brysiewicz Citation2018) and Pomeranian Bay (Rybczyk et al. Citation2020) found a similar range of unit weights of 6.6–146 g and 20.0–220 g, respectively. The demonstrated significantly higher condition of the Dąbie Lake round goby may be due to the influence of better environmental conditions (including food resources, substrate type, and population density). However, the values of Fulton’s coefficients in this study were similar to the results for round goby populations from the Pomeranian Bay (Rybczyk et al. Citation2020), Rhine and Danube (Polačik et al. Citation2009; Brandner et al. Citation2013).
The round goby also varied in their GSI and HSI values. The GSI index can be considered generally low for the species, but typical for fish immediately after spawning (Dashinov & Uzunova Citation2021). On the other hand, the HSI index, which describes the state of energy stored in the fish, is considered a good indicator of the recent foraging activity of the fish. The HSI values found were lower than those recorded in the Danube round goby population (3.2–6.5%) (Brandner et al. Citation2013). This may be because fish were captured for the study immediately after spawning. Before spawning, mobilization of hepatic reserves for gonad maturation occurs and liver weight decreases (Pawar et al. Citation2020). Lower HIS in Dąbie Lake round goby may also have been caused by the negative effects of heavy metals (especially Pb) on the fish. A similar effect of high metal concentrations on HSI values was found by Mazygula et al. (Citation2019) in round goby from the Sea of Azov.
4.3. Heavy metal levels in the round goby
In general, the demonstrated different magnitudes of heavy metal concentrations and the differentiation of fish body parts based on the degree of metal accumulation were consistent with the results of other similar studies (e.g. Subotić et al. Citation2013; Díaz-de-Alba et al. Citation2021; Nędzarek et al. Citation2021; Gao et al. Citation2021; Ai et al. Citation2022; Cordeli et al. Citation2023; Habib et al. Citation2024; Jarf et al. Citation2024).
The reported lower concentrations of Cd and Co and higher concentrations of other heavy metals in the round goby were consistent with studies on other fish species (e.g., Canpolat et al. Citation2016; Jarf et al. Citation2024), where variation was associated with different concentrations of these elements in the environment, analogous to our study. For example, in our study, Cd and Co had the lowest concentrations in the water, and the round goby accumulated heavy metals from the water (issues discussed in Section 4.4.). The role of essential trace elements (including Co, Cr, Mo, and Ni) in many biochemical processes in the body is important. For example, they act as cofactors for enzymes, are involved in lipid metabolism, activate hormones, or stabilize DNA. It is significant that toxic metals (Cd, Pb) accumulate in the body during the detoxification process, which can lead to elevated concentrations of these trace elements in fish even at their low levels in the environment (Zoroddu et al. Citation2019; Kumar et al. Citation2024).
The distribution of metals in individual fish tissues and organs depends on their affinity for metals, the degree of metal uptake and accumulation, and the body’s ability to excrete metals. In general, lower concentrations of heavy metals are observed in muscle and higher concentrations in the gills, liver, and kidneys (metabolically active organs). Gills are important entry points for heavy metals because they are negatively charged and can attract metal cations present in the water. The liver, on the other hand, is the organ responsible for their detoxification – it produces metal-binding proteins and accumulates them. This results in higher concentrations of heavy metals in these organs compared to muscle (they have fewer binding proteins) (Garai et al. Citation2021; Nędzarek et al. Citation2021; Cordeli et al. Citation2023; Habib et al. Citation2024). On the other hand, the mostly lower concentrations of heavy metals in gonads can be explained by the body’s defense mechanism to protect reproductive cells from toxic elements (Khristoforova et al. Citation2015).
The level of heavy metal accumulation in fish depends on the habitat, especially its pollution (Subotić et al. Citation2013). This is also true for round goby, as shown by a few reports. For example, round goby from the Black Sea (Bulgarian coast) had Pb and Cd concentrations similar to the fish we studied (30 and 6 μg kg−1 w.w. respectively) (Stancheva et al. Citation2013). In contrast, Pb concentrations in the liver of round goby from the Gulf of Gorgan (Caspian Sea, Iran) increased with environmental pollution from 33 to 60 μg kg−1 dry weight (Omidi et al. Citation2020).
Proportional (e.g., Canpolat et al. Citation2016), negative, or no (e.g., Conard et al. Citation2021) relationships have been reported between metal concentration and fish size and/or weight. For the round goby studied, these relationships can be considered proportional, as indicated by significant positive correlations of metal concentrations with fish weight from all water bodies (). Such homogeneity was not observed for the liver and gonads. Significant correlations of metals with liver weight were shown mainly for fish from Szczecin Lagoon and no correlation for gonads of fish from any basin. The differences in the correlations shown may be due to the quality of the environmental conditions and the diet of the fish, as suggested, for example, by Conard et al. (Citation2021). Thus, the physical significance of the principal components in the PCA analysis could be attributed to different habitat conditions for the round goby. The effect of this influence could be illustrated in the PCA plots by the different positions of muscles and gills for round goby from Dąbie Lake (freshwater) compared to Szczecin Lagoon (brackish water) and Puck Bay (sea water). Only gonads and liver were always located in the same regions on the PCA maps, which could be due to the characteristic metabolic activity of these organs. However, due to the high seasonality of gonadal processes (related to the reproductive cycle), it should be concluded that it is the liver of the round goby that should be used as an organ for routine environmental monitoring, as it can provide comparable data on environmental quality. An important argument for this may be the demonstrated strong positive correlation of heavy metals in the liver (and also in muscle). As reported e.g. by Kumar et al. (Citation2024), such correlated metals have similar dispersion properties and bioaccumulation homology in the body.
4.4. Bioconcentration factor
At BCF < 1000, the chemical is not considered to be significantly bioaccumulative (Costanza et al. Citation2012). Based on this, it can be concluded that the heavy metals tested did not show significant bioaccumulation in the round goby. Increased bioaccumulation was mainly shown by Cd, followed by Ni and Pb, especially in fish liver, to a lesser extent in gonads and gills, and lowest in muscle. This phenomenon can be explained by the physiological functions that these organs perform in the functioning of the body and the associated tendency to selectively accumulate individual elements.
At the same time, the round goby had a higher potential to accumulate heavy metals from water than from bottom sediments (BCFW > BCFBS). Similar relationships were found by Tao et al. (Citation2012), Canpolat et al. (Citation2016), and Nędzarek et al. (Citation2021). In general, elements dissolving in water form more mobile ionic forms, while combining with organic (sedimentary) matter they form chelates that are difficult to dissolve, making them less accessible to the trophic network (Jaskuła & Sojka Citation2022; Liu et al. Citation2023). The hydrodynamic regime specific to each water body is also likely to be important in the studied waters, similar to what Sigacheva et al. (Citation2022) showed in their study of Taganrog Bay (Azov Sea). They suggest that at shallow depths and with high hydrodynamic activity, sediments are subject to displacement, taking contaminants with them and eventually accumulating in the deep water zone. Our water bodies are also characterized by high water dynamics, are shallow (although include areas with some deep waters – the deepest in Puck Bay) and are subject to intense mixing of the entire water mass in the vertical section. They also have high water exchange rates. Thus, these may be factors that significantly influence the accumulation of metals by the fish under study. This may be supported by seasonal migrations – the round goby spends summer and fall in the benthic coastal zone, but migrates to deep zones in winter and early spring, leading to changes in its diet (Rakauskas et al. Citation2020; Conard et al. Citation2021).
The variation in both BCFs between water bodies indicates the different forms of heavy metals available to fish in these water bodies. The often significantly (p < 0.05) higher values of both BCFs for Puck Bay suggest that both sediments and water of this basin contained more mobile forms of heavy metals and thus were more readily available to fish compared to Dąbie Lake and especially Szczecin Lagoon (for which the values of both BCFs were mostly significantly lowest). However, the explanation of these differences requires further studies that take into account the speciation of metals and their flow through the entire trophic network. The additional consideration (in addition to water and bottom sediments) of a variety of aquatic consumers and primary producers will allow us to understand the trophic mechanism of metal flow in these highly dynamic waters, as demonstrated in the studies by Gao et al. (Citation2021) and Zheng et al. (Citation2023).
Following Canpolat et al. (Citation2016), it can be concluded that the concentration level of heavy metals in the water of the studied water bodies did not indicate a direct toxic risk to fish. This risk may have been reduced by the typically high content of Ca and Mg in these water bodies (Glasby et al. Citation2004; Nędzarek et al. Citation2007). This is because the toxicity of heavy metals decreases with increasing water hardness. This is a combined effect of chemical processes (decreased bioavailability of metals due to their speciation) and physiological mechanisms (e.g. decreased permeability of toxic metals across cell membranes with increased Ca2+ concentration, for which Cd is an antagonist) (Ghosh & Adhikari Citation2006; Pratap Citation2008).
4.5. Human health risk assessment
The heavy metals studied are trace elements, with Cd and Pb generally considered toxic and Co, Cr, Mo, and Ni being necessary for normal bodily function in small amounts, although showing adverse effects at elevated concentrations (Zoroddu et al. Citation2019). The estimated daily intake (EDI) of all heavy metals reported in our study was well below the reference (safe) oral dose (RfD) established by the US EPA (Citation2023). However, it should be noted that with an intake of 30 g of fish muscle per day (a portion calculated based on the annual fish consumption in Poland, estimated at about 12 kg per capita), the consumer will ingest more than 1% of the recommended safe dose for Cd and more than 10% for Pb.
For food safety reasons, provisional tolerable daily intake (PTDI) values have been established for Cd and Pb, which are 0.83 and 1.5 µg kg−1 d−1, respectively (EFSA Citation2010; FAO/WHO Citation2011). In our study, the EDI values were lower than the PTDI for these metals, which also suggests that the flesh of the fish tested should not pose a health risk. Furthermore, the concentrations of Cd and Pb in the round goby did not exceed the European Community standard (No. 1881/2006), which sets the maximum permitted levels in marine fish for both at 0.05 mg kg−1 w.w (EC Citation2011).
These target hazard quotients (e.g., according to Tan et al. Citation2021) can be considered low, as the THQ values were below the permissible level (THQ <1). The cancer risk (CR) can be considered acceptable due to Ni consumption (CR at 10–5) or elevated due to Cd (CR at 10–5) and especially Pb (CR > 10−3) consumption. When considering the risk, it is also necessary to take into account the sum effect of the mixture of heavy metals because the potential carcinogenic risk increases as a result of cumulative exposure (Djedjibegovic et al. Citation2020).
In our study, the hazard index was much lower than 1. The main factor of exposure to the mixture of metals was Pb. It should also be considered that the risk of health exposure is proportionally higher when consuming a larger portion of fish than the one used in the calculations (30 g portion). Moderation in fish consumption should therefore be recommended, especially for pregnant women and also for children, for whom the PTDI for lead (0.5 µg kg−1 d−1) is lower than that recommended for adults.
5. Conclusions
The studied water bodies differed in heavy metal abundance (higher concentrations were mostly recorded in Dąbie Lake and Szczecin Lagoon than in Puck Bay). Bottom sediments had higher concentrations of heavy metals than water.
The size and weight of the studied round goby were similar to those of adults of this species from other non-native European populations.
The level of heavy metal accumulation differed in different parts of the round goby’s body and varied from one water body to another. In most cases, the highest concentrations of metals were found in fish from Dąbie Lake. The liver accumulated the highest amount of metals (maximum concentrations of Cd, Co, Mo, Pb), followed by the muscles and the least by the gonads and gills. Concentrations of heavy metals in round goby were correlated with their concentration in the environment, and the fish had a higher potential to accumulate metals from water than from bottom sediments (BCFW > BCFBS).
Consumption of round goby muscle from the southern Baltic Sea should not pose a health risk because the estimated daily intake (EDI) of heavy metals was well below the reference (safe) oral dose (RfD) and the cancer risk (CR) can be considered acceptable. However, due to the concentration of Pb in round goby muscles, moderate fish consumption should be recommended for pregnant women and children.
The results obtained can be used in the selection of indicator species when developing methods for biological assessment of aquatic environmental quality based on heavy metal bioconcentration processes. At the same time, we suggest that the round goby liver should be used as an organ for routine environmental monitoring. The inclusion of round goby as an indicator species can also be part of measures to reduce populations of these invasive fish in ecosystems where they are an alien species.
Supplemental Material
Download MS Word (12.7 KB)Supplemental Material
Download MS Word (25.8 KB)Supplemental Material
Download MS Word (29 KB)Disclosure statement
No potential conflict of interest was reported by the author(s).
Supplemental material
Supplemental data for this article can be accessed online at https://doi.org/10.1080/24750263.2024.2354696
Additional information
Funding
References
- Ai L, Ma B, Shao S, Zhang L, Zhang L. 2022. Heavy metals in Chinese freshwater fish: Levels, regional distribution, sources and health risk assessment. Science of the Total Environment 853:158455. DOI: 10.1016/j.scitotenv.2022.158455
- Bangel H, Schernewski G, Bachor A, Landsberg-Uczciwek M. 2004. Spatial pattern and long-term development of water quality in the Oder estuary. In: Schernewski G, Dolch T, editors. The Oder Lagoon – against the background of the European water framework directive. Vol. 57. Warnemünde, Germany: Marine Science Reports. pp. 17–65.
- Berg LS. 1949. Freshwater fishes of the USSR and adjacent countries. Leningrad, USSR: Academy of Sciences USSR Zoological Institute. p. 850.
- Brandner J, Auerswald K, Cerwenka AF, Schliewen UK, Geist J. 2013. Comparative feeding ecology of invasive Ponto-Caspian gobies. Hydrobiologia 703(1):113–131. DOI: 10.1007/s10750-012-1349-9
- Briffa J, Sinagra E, Blundell R. 2020. Heavy metal pollution in the environment and their toxicological effects on humans. Heliyon 6(9):e04691. DOI: 10.1016/j.heliyon.2020.e04691
- Canpolat O, Eroglu M, Dusukcan M. 2016. Transfer factor of some heavy metals in muscle of Cyprinus carpio. Fresenius Environmental Bulletin 25(11):4988–4994.
- Conard WM, Gerig BS, Lovin LM, Bunnell B, Lamberti GA. 2021. Metal accumulation in Lake Michigan prey fish: Influence of ontogeny, trophic position, and habitat. Journal of Great Lakes Research 47(6):1746–1755. DOI: 10.1016/j.jglr.2021.08.019
- Cordeli AN, Oprea L, Creţu M, Dediu L, Coadă MT, Mînzală DN. 2023. Bioaccumulation of metals in some fish species from the Romanian Danube River: A review. Fishes 8(8):387. DOI: 10.3390/fishes8080387
- Costanza J, Lynch DG, Boethling RS, Arnot JA. 2012. Use of the bioaccumulation factor to screen chemicals for bioaccumulation potential. Environmental Toxicology and Chemistry 31(10):2261–2268. DOI: 10.1002/etc.1944
- Czerniejewski P, Bienkiewicz G, Tokarczyk G. 2023. Nutritional quality and fatty acid composition of invasive Chinese Mitten Crab from Odra Estuary (Baltic Basin). Foods 12(16):3088. DOI: 10.3390/foods12163088
- Czerniejewski P, Brysiewicz A. 2018. Condition and population structure of the round goby (Neogobius melanostomus Pallas, 1811) in Szczecin Lagoon in 2010–2014. Journal of Water and Land Development 37(1):49–55. DOI: 10.2478/jwld-2018-0024
- Daniszewski P. 2013. Heavy metals in the waters of Dąbie Lake (West-Pomeranian Voievodship, North-West Poland). International Letters of Chemistry, Physics and Astronomy 12:72–81. DOI: 10.56431/p-7ejl06
- Dashinov D, Uzunova E. 2021. Reproductive biology of pioneer round gobies (Neogobius melanostomus Pallas, 1814) at the edge of their invasion front in three small rivers (Lower Danube Basin, Bulgaria). Journal of Vertebrate Biology 70(4):1–12. DOI: 10.25225/jvb.21026
- Díaz-de-Alba M, Granado-Castro MD, Galindo-Riaño MD, Casanueva-Marenco MJ. 2021. Comprehensive assessment and potential ecological risk of trace element pollution (As, Ni, Co and Cr) in aquatic environmental samples from an industrialized area. International Journal of Environmental Research Public Health 18(14):7348. DOI: 10.3390/ijerph18147348
- Djedjibegovic J, Marjanovic A, Tahirovic D, Caklovica K, Turalic A, Lugusic A, Omeragic E, Sober M, Caklovica F. 2020. Heavy metals in commercial fish and seafood products and risk assessment in adult population in Bosnia and Herzegovina. Scientific Reports 10(1):13238. DOI: 10.1038/s41598-020-70205-9
- Dybowski D, Janecki M, Nowicki A, Dzierzbicka-Glowacka LA. 2020. Assessing the impact of chemical loads from agriculture holdings on the Puck Bay environment with the high-resolution ecosystem model of the Puck Bay, Southern Baltic Sea. Water 12(7):2068. DOI: 10.3390/w12072068
- E.C. European Commission. 2011. Commission Regulation (EU) No 420/2011 of 29 April 2011 amending Regulation (EC) No 1881/2006 setting maximum levels for certain contaminants in foodstuffs. https://www.fsai.ie/uploadedFiles/Reg420_2011.pdf
- E.F.S.A. European Food Safety Authority. 2010. EFSA panel on contaminants in the food chain (CONTAM); scientific opinion on lead in food. EFSA Journal 8(4):1570–1719. DOI: 10.2903/j.efsa.2010.1570
- F.A.O./W.H.O. Food and Agriculture Organization of the United Nations/World Health Organization. 2011. Evaluation of Certain Food Additives and Contaminants. Seventy-Third Report of the Joint FAO/WHO Expert Committee on Food Additives. WHO Technical Report Series Rome, Italy; Geneve, Switzerland: FAO/WHO. p. 960
- Gao Y, Wang R, Li Y, Ding X, Jian Y, Feng J, Zhu L. 2021. Trophic transfer of heavy metals in the marine food web based on tissue residuals. Science of the Total Environment 772:145064. DOI: 10.1016/j.scitotenv.2021.145064
- Garai P, Banerjee P, Mondal P, Saha NC. 2021. Effect of heavy metals on fishes: Toxicity and bioaccumulation. Journal of Clinical Toxicology S18:001.
- Ghosh L, Adhikari S. 2006. Accumulation of heavy metals in freshwater fish – an assessment of toxic interaction with calcium. American Journal of Food Technology 1(20):139–148. DOI: 10.3923/ajft.2006.139.148
- Glasby GP, Szefer P, Geldon J, Warzocha J. 2004. Heavy-metal pollution of sediments from Szczecin Lagoon and the Gdansk Basin, Poland. Science of the Total Environment 330(1–3):249–269. DOI: 10.1016/j.scitotenv.2004.04.004
- Grula D, Balážová M, Copp GH, Kováč W. 2012. Age and growth of invasive round goby Neogobius melanostomus from middle Danube. Central European Journal of Biology 7(30):448–459. DOI: 10.2478/s11535-012-0024-5
- Habib SS, Naz S, Fazio F, Cravana C, Ullah M, Rind KH, Attaullah S, Filiciotto F, Khayyam K. 2024. Assessment and bioaccumulation of heavy metals in water, fish (wild and farmed) and associated human health risk. Biological Trace Element Research 202(2):725–735. DOI: 10.1007/s12011-023-03703-2
- Helios-Rybicka E, Adamiec E, Aleksander-Kwaterczak U. 2005. Distribution of trace metals in the Odra River system: Water–suspended matter–sediments. Limnologica 35(3):185–198. DOI: 10.1016/j.limno.2005.04.002
- Jarf MP, Kamali A, Khara H, Pourang N, Shekarabi SPH. 2024. Microplastic pollution and heavy metal risk assessment in Perca fluviatilis from Anzali wetland: Implications for environmental health and human consumption. Science of the Total Environment 907:167978. DOI: 10.1016/j.scitotenv.2023.167978
- Jaskuła J, Sojka M. 2022. Assessment of spatial distribution of sediment contamination with heavy metals in the two biggest rivers in Poland. CATENA 211:105959. DOI: 10.1016/j.catena.2021.105959
- Jitar O, Teodosiu C, Oros A, Plavan G, Nicoara M. 2015. Bioaccumulation of heavy metals in marine organisms from the Romanian sector of the Black Sea. New Biotechnology 32(3):369–378. DOI: 10.1016/j.nbt.2014.11.004
- Khristoforova NK, Tsygankov VY, Boyarova MD, Lukyanova ON. 2015. Heavy metal contents in the pink salmon Oncorhynchus gorbuscha Walbaum, 1792 from Kuril Oceanic Waters during anadromous migration. Russian Journal of Marine Biology 41(6):479–484. DOI: 10.1134/S1063074015060085
- Kucharski D, Giebułtowicz J, Drobniewska A, Nałęcz-Jawecki G, Skowronek A, Strzelecka A, Mianowicz K, Drzewicz P. 2022. The study on contamination of bottom sediments from the Odra River estuary (SW Baltic Sea) by tributyltin using environmetric methods. Chemosphere 308:136133. DOI: 10.1016/j.chemosphere.2022.136133
- Kumar V, Swain HS, Upadhyay A, Ramteke MH, Sarkar DJ, Roy S, Das BK. 2024. Bioaccumulation of potentially toxic elements in commercially important food fish species from lower Gangetic stretch: Food security and human health risk assessment. Biological Trace Element Research 202(3):1235–1248. DOI: 10.1007/s12011-023-03743-8
- Li Z, Ma Z, van der Kuijp, TJ, Yuan Z, Huang L. 2014. A review of soil heavy metal pollution from mines in China: Pollution and health risk assessment. Science of the Total Environment 468–469:843–853. DOI: 10.1016/j.scitotenv.2013.08.090
- Linde-Arias AR, Inacio AF, Alburquerque C, Freire MM, Moreiro JC. 2008. Biomarkers in an invasive fish species, Oreochromis niloticus, to assess the effects of pollution in a highly degraded Brazilian River. Science of the Total Environment 399(1–3):186–192. DOI: 10.1016/j.scitotenv.2008.03.028
- Liu S, Yu F, Lang T, Ji Y, Fu Y, Zhang J, Ge C. 2023. Spatial distribution of heavy metal contaminants: The effects of water-sediment regulation in the Henan section of the Yellow River. Science of the Total Environment 892:164568. DOI: 10.1016/j.scitotenv.2023.164568
- Matern S, Herrmann J-P, Temming A. 2021. Differences in diet compositions and feeding strategies of invasive round goby Neogobius melanostomus and native black goby Gobius niger in the Western Baltic Sea. Aquatic Invasions 1(2):314–328. DOI: 10.3391/ai.2021.16.2.07
- Mazygula E, Kharlamova M, Skuratovskaya E. 2019. Assessment of the impact of oil and heavy metal pollution in the Azov Sea on the condition of the Neogobius Melanostomus (Pallas, 1814). IOP Conference Series: Earth and Environmental Science 272(3):032071. DOI: 10.1088/1755-1315/272/3/032071
- Nędzarek A, Czerniejewski P. 2022. Impact of polyaluminum chloride on the bioaccumulation of selected elements in the tissues of invasive spiny-cheek crayfish (Faxonius limosus) – potential risks to consumers. Science of the Total Environment 828:154435. DOI: 10.1016/j.scitotenv.2022.154435
- Nędzarek A, Kubiak J, Tórz A. 2007. Organic pollution of Lake Dąbie waters in 1997-2000. Electronic Journal of Polish Agricultural Universities, Fisheries 10(3):8. http://www.ejpau.media.pl/volume10/issue3/art-08.htlm
- Nędzarek A, Kubiak J, Tórz A, Machula S. 2007. Heavy metals in the waters of Dąbie lake in the years 1997-2000. Ecological Chemistry and Engineering 14(1):77–83.
- Nędzarek A, Rybczyk A, Bonisławska M, Tański A, Tórz A. 2021. Bioaccumulation of metals in tissues of Rutilus rutilus and Perca fluviatilis from lakes with poor ecological status – human health risk assessment. The European Zoological Journal 88(1):1084–1095. DOI: 10.1080/24750263.2021.1988160
- Nunes C, Silva A, Soares E, Ganias K. 2011. The use of hepatic and somatic indices and histological information to characterize the reproductive dynamics of Atlantic sardine Sardina pilchardus from the Portuguese Coast. Marine and Coastal Fisheries 3(1):127–144. DOI: 10.1080/19425120.2011.556911
- N.Y.S. D.O.H. Nev York State Department of Health, 2007. Hopewell precision area contamination: Appendix C – NYS DOH. In: Procedure for evaluating potential health risk for contaminants of concern. http://www.health.ny.gov/environmental/investigations/hopewell/appendc.html. Accessed Jan 2024 9.
- Oesterwind D, Bocka C, Försterb A, Gabela M, Henselera C, Kotterba P, Menge M, Myts D, Winkler HM. 2017. Predator and prey: The role of the round goby Neogobius melanostomus in the western Baltic. Marine Biology Research 13(2):188–197. DOI: 10.1080/17451000.2016.1241412
- Omidi F, Jafaryan H, Patimar R, Harsij M, Paknejad H. 2020. Biochemical biomarkers of skin mucus in Neogobius melanostomus for assessing lead pollution in the gulf of Gorgan (Iran). Toxicology Reports 7:109–117. DOI: 10.1016/j.toxrep.2019.12.003
- Pawar MB, Sa Q, Shankar TR. 2020. Studies on the fecundity (F), gonadosomatic index (GSI) of Cyprinus carpio (Common carp) due to administration of hormonal doses PGE, Ovaprim and Ovatide at FSPC, Aurangabad, Maharashtra, India. International Journal of Fisheries and Aquatic Studies 8(6):39–41. DOI: 10.22271/fish.2020.v8.i6a.2359
- Pinchuk V, Vasil‘eva ED, Vasil‘ev VP, Miller PJ. 2003. Neogobius melanostomus (Pallas, 1814). In: Miller P.J. ed. The Freshwater Fishes of Europe 8(1):293–345. AULA-Verlag GmbH Wiebelsheim.
- Polačik M, Janáč M, Jurajda P, Adámek Z, Ondračková M, Trichkova T, Vassilev M. 2009. Invasive gobies in the Danube: Invasion success facilitated by availability and selection of superior food resources. Ecology of Freshwater Fish 18(4):640–649. DOI: 10.1111/j.1600-0633.2009.00383.x
- Polačik M, Janáč M, Trichkova T, Vassilev M, Jurajda P, Jurajda P. 2008. The distribution and abundance of the Neogobius fishes in their native range (Bulgaria) with notes on the non-native range in the Danube River. Large Rivers 18(1–2):193–208. DOI: 10.1127/LR/18/2008/193
- Pratap HB. 2008. Effects of ambient and dietary cadmium on haematological parameters in Oreochromis mossambicus acclimatised to low- and high-calcium water. Comparative Clinical Pathology 17(2):133–136. DOI: 10.1007/s00580-007-0714-y
- Radziejewska T, Schrenewski G. 2008. The Szczecin (Oder-) Lagoon. In: Schiewer U, editor Ecology of Baltic Coastal Waters. Berlin; Heidelberg: Springer. pp. 115–129.
- Rakauskas V, Šidagytė-Copilas E, S S, Garbaras A. 2020. Invasive Neogobius melanostomus in the Lithuanian Baltic Sea coast: Trophic role and impact on the diet of piscivorous fish. Journal of Great Lakes Research 46(3):597–608. DOI: 10.1016/j.jglr.2020.03.005
- Regulation MI. 2021. Regulation of the ministry of infrastructure of 25 June 2021 on the classification of ecological status, ecological potential and chemical status, and the method of classification of the status of surface water bodies, as well as environmental quality standards for priority substances. Journals of Laws 2021:1475. https://leap.unep.org/en/countries/pl/national-legislation/regulation-classification-ecological-status-ecological-1. Accessed 10 January 2024.
- Ritterbusch-Nauwerck B. 1995. Condition or corpulence, fitness or fatness: A discussion of terms. Archiv für Hydrobiologie, Special Issues in Advanced Limnology 46:109–112.
- Rybczyk A, Czerniejewski P, Keszka S, Janowicz M, Brysiewicz A, Wawrzyniak W. 2020. First data of age, condition, growth rate and diet of invasive Neogobius melanostomus (Pallas, 1814) in the Pomeranian Bay, Poland. Journal of Water and Land Development 47(X–XII):142–149. DOI: 10.24425/jwld.2020.135041
- Sapota MR. 2004. The round goby (Neogobius melanostomus) in the Gulf of Gdańsk – a species introduction into the Baltic Sea. Hydrobiologia 514(1–3):219–224. DOI: 10.1023/B:hydr.0000018221.28439.ae
- Schrenewski G, Friedland R, Buer AL, Dahlke S, Drews B, Hoft S, Klumpe T, Schadach M, Schumacher J, Zaiko A. 2019. Ecological-social-economic assessment of zebra-mussel cultivation scenario for Oder (Szczecin) Lagoon. Journal of Coastal Conservation 23(5):913–929. DOI: 10.1007/s11852-018-0649-2
- Sigacheva T, Skuratovskaya E. 2022. Application of biochemical and morphophysiological parameters of round goby Neogobius melanostomus (Pallas, 1814) for assessment of marine ecological state. Environmental Science and Pollution Research 29(26):39323–39330. DOI: 10.1007/s11356-022-18962-0
- Sigacheva TB, Skuratovskaya EN, Kurshakov SV, Ryzhilov MS. 2022. A comparative analysis of biochemical parameters in the liver of round goby Neogobius melanostomus (Pallas, 1814) from two regions of the Taganrog Bay (Sea of Azov). Russian Journal of Marine Biology 48(1):19–25. DOI: 10.1134/S1063074022010114
- Skóra KE, Stolarski J. 1993. New fish species in the Gulf of Gdansk, Neogobius sp. [cf. Neogobius melanostomus (Pallas 1811)]. Bulletin of the Sea Fisheries Institute 1:128 83–84.
- Stancheva M, Merdzhanova A, Prtova E, Petrova D. 2013. Heavy metals and proximate composition of black sea sprat (Sprattus sprattus) and goby (Neogobius melanostomus). Bulgarian Journal of Agricultural Science 19:35–41.
- Subotić S, Višnjić Jeftić Ž, Spasić S, Hegediš A, Krpo-Ćetković J, Lenhardt M. 2013. Distribution and accumulation of elements (As, Cu, Fe, Hg, Mn, and Zn) in tissues of fish species from different trophic levels in the Danube River at the confluence with the Sava River (Serbia). Environmental Science and Pollution Research 20(8):5309–5317. DOI: 10.1007/s11356-013-1522-3
- Szefer P, Glasby GP, Kusak A, Szefer K, Jankowska H, Wolowicz M, Ali AA. 1998. Evaluation of the anthropogenic influx of metallic pollutants into Puck Bay, southern Baltic. Applied Geochemistry 13(3):293–304. DOI: 10.1016/S0883-2927(97)00098-X
- Tan Y, Peng B, Wu Y, Xiong L, Sun J, Peng G, Xufeng Bai X. 2021. Human health risk assessment of toxic heavy metal and metalloid intake via consumption of red swamp crayfish (Procambarus clarkii) from rice-crayfish co-culture fields in China. Food Control 128:108181. DOI: 10.1016/j.foodcont.2021.108181
- Tao Y, Yuan Z, Xiaona H, Wein M. 2012. Distribution and bioaccumulation of heavy metals in aquatic organisms of different trophic levels and potential health risk assessment from Taihu lake, China. Ecotoxicology and Environmental Safety 81:55–64. DOI: 10.1016/j.ecoenv.2012.04.014
- U.S. E.P.A. U.S. Environmental Protection Agency. 2000. Guidance for assessing chemical contaminant data for use in fish advisories, vol 2. Risk assessment and fish consumption limits, 3rd Edition. http://www.epa.gov/waterscience/fish/guidance.html. Accessed Jan 2024 9.
- U.S. E.P.A. U.S. Environmental Protection Agency. 2010. Risk based screening table. http://www.epa.gov/iriswebp/iris/index.html
- U.S. E.P.A. U.S. Environmental Protection Agency. 2023. Regional Screening Level (RSL) summary table (TR=1E-06, HQ=1) November 2023. https://semspub.epa.gov/work/HQ/400431.pdf. Accessed Jan 2024 10.
- Vrhovnik P, Arrebola JP, Serafimovski T, Dolenec T, Šmuc NR, Dolenec M, Mutch E. 2013. Potentially toxic contamination of sediments, water and two animal species in Lake Kalimanci, FYR Macedonia: Relevance to human health. Environmental Pollution 180:92–100. DOI: 10.1016/j.envpol.2013.05.004
- Zheng R, Liu Y, Zhang Z. 2023. Trophic transfer of heavy metals through aquatic food web in the largest mangrove reserve of China. Science of the Total Environment 899:165655. DOI: 10.1016/j.scitotenv.2023.165655
- Zhou M, Wu Q, Wu H, Liu J, Ning Y, Xie S, Huang W, Bi X. 2021. Enrichment of trace elements in red swamp crayfish: Influences of region and production method, and human health risk assessment. Aquaculture 535:736366. DOI: 10.1016/j.aquaculture.2021.736366
- Zoroddu MA, Aaseth J, Crisponi G, Medici S, Peana M, Nurchi VM. 2019. The essential metals for humans: A brief overview. Journal of Inorganic Biochemistry 195:120–129. DOI: 10.1016/j.jinorgbio.2019.03.013