ABSTRACT
OBJECTIVES: The impairment of cognitive functions in diabetes mellitus (DM) is well known. Nitric oxide (NO)-guanyly cyclase (GC)-cyclic guanosine monophosphate (cGMP) pathway is an important role in cognitive functions, and, consequently, phosphodiesterase-5 (PDE-5) inhibitors as well as NO-GC activators are of considerable interest in the treatment of cognitive deficits. Therefore, we aimed to investigate the effects of YC-1 (3-5-hydroxymethyl-2-furyl)-1-benzyl-indazole), an NO-GC activator, and zaprinast, a PDE-5 inhibitor, on cognitive dysfunction in streptozotocin (STZ)-induced diabetic rats.
METHODS: Male Wistar rats were divided into five groups (nine rats in each): Control, Vehicle (DMSO), Diabetic (50 mg/kg/i.p. streptozotocin), Diabetic-YC-1 (1 mg/kg/day/i.p., for 4 weeks), and Diabetic-Zaprinast (10 mg/kg/day/i.p., for 4 weeks) group. The Morris water maze, passive avoidance, locomotor activity, and foot shock sensitivity tests were applied to assess the effect of YC-1 and zaprinast on learning and memory. To explore the mechanisms of YC-1 and zaprinast on learning and memory performance, protein expressions of brain-derived neurotrophic factor (BDNF) in hippocampus were examined immunohistochemically.
RESULTS: Thirty days after the induction of DM, rats exhibited severe learning and memory dysfunctions associated with decreased BDNF expression. Both chronic YC-1 and zaprinast treatment improved cognitive performance and hippocampal BDNF expression in diabetic rats. In the locomotor activity and foot shock sensitivity test, no significant differences were observed between the groups. Furthermore, hyperglycaemia did not affect cognition enhancement effects of YC-1 or zaprinast in diabetic rats.
CONCLUSIONS: Our findings highlight the beneficial effects of nitric oxide-guanylyl cyclase activators and PDE-5 inhibitors to improve cognitive function in diabetes.
Introduction
Previously, it has been reported that soluble guanylate cyclase (sGC) and cyclic guanosine monophosphate (cGMP) have a major role in cerebral processes such as long-term potentiation (LTP) [Citation1,Citation2] and some forms of learning and memory, including object recognition [Citation3,Citation4], inhibitory avoidance learning [Citation5,Citation6], spatial learning in the Morris water maze (MWM) [Citation7], and conditional discrimination in a Y-maze test [Citation8,Citation9]. The glutamate-nitric oxide (NO)-cGMP pathway has been reported to be an important pathway, which modulates the levels of cGMP in brain in response to synaptic activity. This pathway leads to an increase in cGMP levels in response to activation of NMDA receptors. This nucleotide activates cGMP-dependent protein kinase (PKG) that phosphorylates and activates cGMP-degrading phosphodiesterase-5 (PDE-5). The activation of this glutamate-NO-cGMP-PKG-PDE-5 pathway has been shown to properly induce and maintain of LTP [Citation2,Citation10]. A novel synthetic compound YC-1 (3-5-hydroxymethyl-2-furyl)-1-benzyl-indazole) activates purified sGC and sensitizes the enzyme for NO in vitro, in human platelets, and in smooth muscle cells [Citation11,Citation12]. YC-1 has been shown to enhance LTP in hippocampal Schafer collateral CA1 synapse [Citation13] and amygdala [Citation14] via NO-cGMP-PKG-dependent pathway and to inhibit the scopolamine-induced memory deficits [Citation15]. Additionally, we previously found that YC-1 has beneficial effects on cognitive functions in aged rats [Citation16] as well. These findings suggest that YC-1 is a therapeutic potential as a drug candidate that improves learning and memory.
In addition, the phosphodiesterases regulate the activity of cGMP that play a crucial role in learning and memory [Citation10,Citation17]. Previous research has reported that cognitive impairment in diabetes may be due to the modulatory effects of PDE-5 enzyme on cGMP levels and PDE-5 inhibitors have protective effects in diabetic cognitive impairments [Citation18]. Zaprinast inhibits isoform hydrolysing cGMP nucleotide and it is described as the PDE-5 which is called cGMP-binding, cGMP-specific phosphodiesterase inhibitor. It inhibits mainly PDE-5 and PDE-6 and moderately inhibits PDE-9, PDE-10, and PDE-11 [Citation19,Citation20]. Moreover, zaprinast-induced PDE-5 inhibition causes vasorelaxation such as treatment with NO donors [Citation21]. GC and PKG inhibitors block the induction of LTP and also cGMP analogues or NO donors produce the potentiation of LTP [Citation22,Citation23]. Thus, it is demonstrated that cGMP/PKG pathway plays an important role in synaptic plasticity.
Diabetes mellitus (DM) is an endocrine disorder of carbohydrate metabolism resulting from insufficiency of insulin release, reduced insulin sensitivity or both [Citation24]. Diabetes is associated with functional and structural disorders in both the peripheral and central nervous system (CNS) [Citation24]. Several reports have indicated that diabetes may cause cognitive dysfunction. Learning and memory impairment in streptozotocin (STZ)-induced diabetic rats has been demonstrated in passive avoidance (PA) test [Citation18,Citation25,Citation26]. Diabetic animals also showed spatial learning and memory deficiency in MWM test [Citation25–28]. Cerebral cortex and hippocampus are critical brain regions for structural and functional cognitive deficits in diabetic rats. Especially, hippocampal synaptic plasticity alterations have been reported in diabetes [Citation25,Citation28]. The pathogenesis of the CNS changes associated with diabetes is multifactorial and may involve inflammation [Citation26], oxidative stress [Citation27], and microvascular dysfunction [Citation29]. Moreover, decreased neuronal nitric oxide synthase (nNOS) mRNA and protein levels have been observed in the cortex and hippocampus of diabetic rats, which may contribute to the cognitive deficits [Citation30]. Additionally, sildenafil significantly reversed the diabetes-induced cognitive deficits in STZ-diabetic rats, suggesting the crucial role of PDE-5 [Citation18].
Therefore, considering the importance of NO/cGMP/PKG pathway and DM in association with cognitive dysfunctions, the aim of this study was to investigate the effects of YC-1 and zaprinast on cognitive functions in STZ-induced diabetic rats.
Materials and methods
Animal housing and ethics statement
Male Wistar rats were divided into five groups (nine rats in each): Control, Vehicle (DMSO), Diabetic (50 mg/kg/i.p. streptozotocin), Diabetic-YC-1, and Diabetic-zaprinast group. Rats from Diabetic-YC-1 group received 1 mg/kg/day of YC-1 and Diabetic-Zaprinast group received zaprinast 10 mg/kg/day intraperitoneally after induction of diabetes for 4 weeks. The rats were obtained from Kocaeli University Experimental Medical Research and Application Center (DETAB, Kocaeli, Turkey). Rats were housed in gatherings of 4 per cage for 2 weeks preceding starting these examinations. Every behavioural trial was directed from 9:00 am to 12:00 pm under standard conditions (22 ± 2°C room temperature; 12-h light/dark cycle with lights on at 7:00 am). Animals were fed with tap water and food pellets ad libitum. In each experiment, rats were naive and different. All experiments documented in this study were conducted in accordance with the Regulation of Animal Research Ethics Committee in Turkey (July 6, 2006, Number 26220). Ethical approval was granted by the Kocaeli University Animal Research Ethics Committee (Project number: KOUHADYEK 5/9 2011/16).
Drugs and treatments
STZ, YC-1, and zaprinast were purchased from Sigma-Aldrich (Saint Louis, MO, USA). STZ was dissolved in citrate buffer (pH 4.4). YC-1 and zaprinast were dissolved in DMSO. Drugs were prepared fresh every day in distilled water and kept on ice during experiments.
Induction of DM and treatment schedule
DM was induced in rats by using our previously reported method with a minor modification [Citation26]. In brief, STZ was dissolved in 0.1 M sodium citrate buffer (pH 4.4) and administered at 50 mg/kg via the intraperitoneal route. STZ-treated rats received 5% glucose solution instead of water for the next 24 h in order to reduce the death risk due to hypoglycaemic shock. In order to measure glucose levels, blood samples were collected from the tail vein one week after STZ injection. Animals with fasting blood glucose levels >200 mg/dl were considered to have DM and used for further study. After confirmation of the induction of DM, separate groups of rats (n = 9) were administered YC-1 (1 mg/kg/day), zaprinast (10 mg/kg/day) or vehicle (1 ml/kg/day) intraperitoneally for 30 days. YC-1 and zaprinast doses were selected on the basis of previous reports [Citation16,Citation31].
At the end of the treatment schedule, rats were tested in the locomotor activity, footshock sensitivity, MWM, or PA tests. The learning and memory performance of rats were evaluated on days 32–39.
Locomotor activity test
Locomotor movements were evaluated with an animal activity monitoring system (May AMS 9701, Commat Ltd., Ankara, Turkey) including a plexiglass box (40 cm × 40 cm × 30 cm), a computer and open field activity software. Total locomotor activity was represented as the sum of the vertical, ambulatory, and stereotypic activities of the animals. The movement was checked for 5 min [Citation26].
PA test
PA task (model 7551, Ugo Basile, Italy) was utilized for the assessment of emotional memory based on contextual fear conditioning, as previously described [Citation32]. Briefly, rats learn to avoid a specific place associated with an aversive event. The diminishment of latency to avoid was used as learning. A guillotine door separated two-compartment containing (the light and dark chamber) apparatus was used. Rats placed in the light chamber after 20 s, the guillotine door separating was opened, and the initial latency to enter the dark chamber was recorded. When the rat entered the dark chamber, it was given a footshock of 0.5 mA for 3 s through the grid floor of the dark compartment. At that point, the rats were come back to their home cage. Twenty-four hours after the acquisition trial, a retention trial was performed. Recall of the shock stimulus was evaluated by returning the animals to the light compartment and recording their latency to enter the dark compartment (four paws in). No foot shock was applied in this trial. If the animal did not enter the dark compartment within 300 s, it was returned to its home cage and a latency of 300 s was recorded. This latency served as a measure of the retention performance of the PA response.
MWM test
A water tank (150 cm in diameter) was used to measure spatial memory as previously described [Citation32]. The platform was put in the centre of the southwest quadrant and submerged 1.5 cm below the surface of water, and a small black plastic ball was put on the water surface. The platform position remained stable over 4 days, and acquisition of finding the platform was assessed. Each of three starting positions (north, east, and west) was used once in a series of four randomly ordered trials. Each trial was terminated as soon as the rat had climbed onto the escape platform or when 60 s had elapsed. A rat was allowed to stay on the platform for 20 s. Then, it was taken from the platform, and the next trial was started. Rats that did not find the platform within 60 s were put on the platform by the experimenter and were allowed to stay there for 20 s. After completion of the third trial, rats were gently dried with a towel, kept warm for an hour, and returned to their home cage (Acquisition trial). A probe trial was utilized to evaluate the rat’s spatial retention of the location of the hidden platform on day 5. During this trial, the platform was removed from the maze and the rat was permitted to search the pool for 60 s in order to spent time in the quadrant that previously contained the hidden platform, so-called target quadrant. During this time, animals have learned the task and were expected to spend more time searching in target quadrant than in the other quadrants. All tests were conducted between 08:00 am and 12:00 pm.
Foot shock sensitivity
The procedure for the footshock sensitivity test was designed as described by Schmatz et al. [Citation33]. Reactivity to shock was evaluated in the same apparatus used for inhibitory avoidance, except that the platform was removed. The flinch and jump threshold was determined. All rats were placed on a grid and were habituated for a 3-min period before initiating a series of shocks (1 s) delivered at 10-s intervals. Shock intensities were ranged 0.1–0.5 mA in 0.1 mA increments. Adjustments in shock intensity were made based on the observed response of each animal. Specifically, one unit increased the shock intensity if there was no response and one unit lowered it if there was a response. A “flinch” response was defined as the withdrawal of one paw from the grid floor, and a “jump” response was defined as the withdrawal of three or four paws. Three measurements of each threshold were made, and the mean of each score was calculated for each animal.
Immunohistochemical analyses for brain-derived neurotrophic factor
After behavioural tests, rats were decapitated and the brain was removed and dissected into hippocampus for the immunohistochemical studies and they fixed with 10% neutral buffered formalin. Routine histological tissue procedure was performed and the tissues were embedded in paraffin. The primary rabbit polyclonal anti-BDNF antibody (Santa Cruz Biotechnology, Santa Cruz, CA USA) was applied. Negative control samples were prepared by replacing the primary antibody with the appropriate non-immune IgG at the same concentration. After several washes, the slides were incubated with a biotinylated secondary antibody (Histostain-Plus Kit, Broad Spectrum, Invitrogen, CA, USA) for 20 min and diaminobenzidine (DAB) (DAB Substrate Kit, Invitrogen #00-2014) was applied for visualization. Sections were briefly counterstained with Mayer’s haematoxylin (Invitrogen, CA, USA) and mounted with ClearMount (Invitrogen, CA, USA) on glass slides. The slides were examined under a light microscope (Olympus BX50, Tokyo, Japan). The photomicrographs were taken with a Lecia DM 100 system (Lecia DFC 290HD, Wetzlar, Hessen, Germany). One independent observer who was blind to the current study graded the staining intensity based on a semiquantitative scale ranging from no expression (0), to very weak (1+), moderate (2+), strong (3+), and very strong (4+) expression. The percentage of positive cells was defined as 0, <5%; 1, 6–15%; 2, 16–50%; 3, 51–80% and 4, >80% positive cell.
Statistical analysis
Data are the mean ± standard error of the mean (S.E.M.). Acquisition (1–4 day) latency scores in the MWM test were measured by two-way analyses of variance (ANOVA). The following were measured by one-way ANOVA: scores of the time spent in the escape-platform quadrant in the MWM; first-day and retention latencies in PA scores; total locomotor activity scores; and foot shock sensitivity scores. Further statistical analyses for individual groups were carried out using the Bonferroni test. The immunoreactivity scores were compared by the Kruskal–Wallis Test following Dunn’s multiple comparison test; p < .05 was considered significant.
Results
Effects of DM and systemic administration of YC-1 and zaprinast on animal weight
Plasma levels of glucose in DM (435.9 ± 14.6 mg/dl), DM-YC-1 (439.3 ± 15.2 mg/dl) and DM-zaprinast groups (379 ± 26.9 mg/dl) were significantly higher than those in non-DM control groups (121.1 ± 5.82 mg/dl) (p < .0001). At the beginning of the experiment, body weight (g) for the control group was 362 ± 7.7, for the DMSO group 354.9 ± 20.2, for the DM group was 327.9 ± 20.2, for the DM-YC-1 group was 345 ± 4.3, and for the DM-zaprinast group was 331.1 ± 4.1. The average body weight after 4 weeks of DM was significantly lower than that in control animals (F(4, 40)=142.33; p < 0.0001) but was not significantly different from that of YC-1-treated DM and also zaprinast-treated rats. Both YC-1 and zaprinast did not show any significant effect per se on the serum glucose level and body weight of rats.
Behavioural assessment
Effects of DM and systemic administration of YC-1 and zaprinast on locomotor activity and foot shock sensitivity test in rats
Control, DMSO, DM, and YC-1 or zaprinast treatment did not affect total locomotor activity (F(4.40)=0.065, p = .9919)(). Moreover, DM did not alter foot shock sensitivity, as demonstrated by the similar flinch (F(4ş,40)=0.3175; p = .8646) and jump (F(4.40)=0.6667; p = .6189) thresholds exhibited by the animals (). This finding suggested that neither DM nor YC-1 or zaprinast treatment caused gross motor disabilities upon testing.
Effects of systemic administration of YC-1 and zaprinast on DM-induced memory impairment in the PA test in rats
Retention times were evaluated in order to examine possible changes by both DM and YC-1 or zaprinast treatment by using PA test. There were no significant difference in between groups (F(4, 40)=0.8181, p = .5213) (A) on day 1 (training session). On the second day, however, there was a significant reduction in retention time in diabetic groups compared to controls. There was no significant change between control rat response with or without YC-1 or zaprinast treatment whereas YC-1 and zaprinast treatment significantly reversed the reduction in retention time diabetic groups (F(4,40)=13.83, p < .0001) ((B)).
Figure 3. (a) Acquisition and (b) Retention latencies in passive avoidance test separately Control, DMSO, Diabetes, Diabetes + YC-1 and Diabetes + Zaprinast groups (n = 9 in each group). Each value represents the mean ± S.E.M. There were no significant differences between the groups in the acquisition test. On the other hand, diabetic rats exhibited a significantly lower latency compared to control rats (***p < .001), and Diabetes+ YC-1 and Diabetes + Zaprinast groups showed higher latency compared to diabetic rats (###p < .001) in the retention test (one-way ANOVA, Bonferroni corrected). There were nine animals in each group.
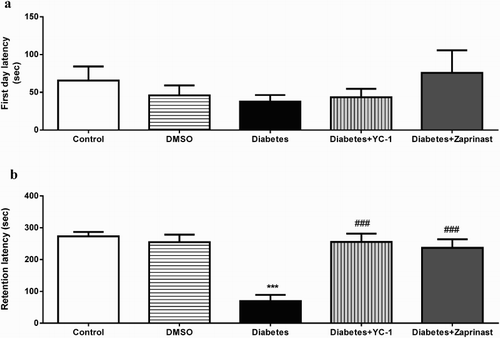
Effects of systemic administration of YC-1 and zaprinast on DM-induced memory impairment in the MWM test in rats
All rats exhibited lower latency time during first 4 days of the test for get to the platform (F(3, 160)=15.29, p < .0001). There was a further statistical difference in between groups by diabetic and YC-1 and zaprinast treatment (F(4,160)=32.94, p < .0001). There was no significant difference between days related with DM and treatment (F(12, 160)=0.24, p = .9963) ((A)).
Figure 4. Morris Water Maze (a) Acquisition (b) Probe test performance separately the Control, DMSO, Diabetes, Diabetes + YC-1 and Diabetes + Zaprinast groups (n = 9 in each group). Diabetes resulted in a significant disruption in learning as indicated by an increase in the escape latency compared with the control animals in the acquisition test (two-way ANOVA, Bonferroni test, effect of treatment, ***p < .001). In addition, both YC-1 (##p < .01, ##p < .01, ###p < .001, ##p < .01, respectively) and zaprinast (+++p < .001, ++p < .01, ++p < .01, +++p < .001, respectively) treatments had a significant effect on acquisition latency in the MWM test in the first 1–4 days compared to diabetic rats. There was a significant difference between diabetes and control groups in the time spent in the correct quadrant during the probe trial of the MWM test (one-way ANOVA, Bonferroni test, F(4,40)=7.330, p < .001). Both YC-1 and zaprinast prolonged the time spent in the correct quadrant.
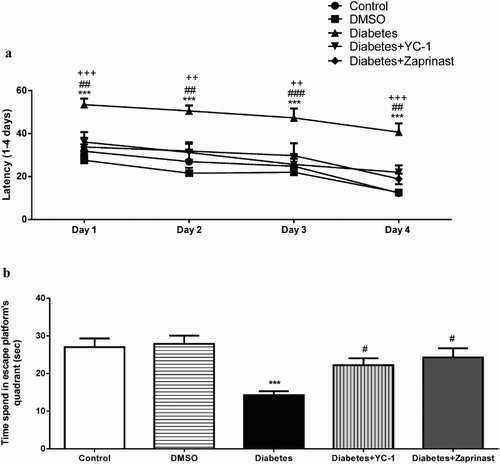
There was a significant difference between control and diabetic groups on the fifth day of the experiment in terms of time spent in the escape-platform quadrant during the probe trial of the MWM test. Also, YC-1 and zaprinast drawn out the time spent in the escape-platform quadrant, suggesting that YC-1 and zaprinast treatment reversed the reduction in the time spent in escape-platform’s quadrant back to control values (p < .001, B) (F(4,40)=7.330, p < .001) ((B)).
Effect of DM and systemic administration of YC-1 and zaprinast on protein expression of brain-derived neurotrophic factor
There was a significant decrease in brain-derived neurotrophic factor (BDNF) protein expression in the hippocampal CA1 region in the diabetic rats, (p < .05, ), whereas treatment with YC-1 and zaprinast significantly increased levels of BDNF protein in the hippocampal CA1 regions in diabetic rats (p < .05, ). In diabetic rats receiving YC-1 and zaprinast, BDNF-protein expressions were similar to those in the control group (p < .05, ).
Figure 5. Representative image illustrating BDNF expression (arrows) in hippocampal formation. Control (a), Diabetes (b), Diabetes + YC-1 (c) and Diabetes + Zaprinast group in CA1 region. BDNF expression was decreased in diabetes in CA1 region of the hippocampus, whereas in diabetes + YC-1 and diabetes + zaprinast rats BDNF was similar to those in the control group in the CA1 regions. Scale bars: 50 μm.
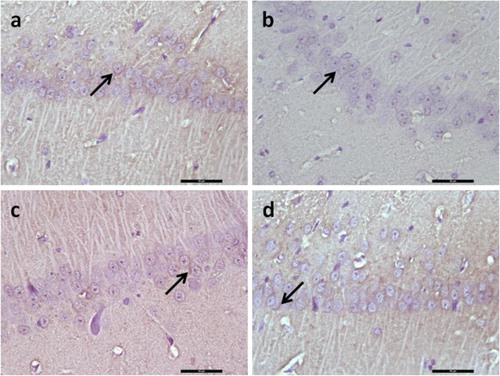
Discussion
Overall results of the current study showed that chronic administration of YC-1 and zaprinast caused changes in BDNF expression in diabetes. It indicates that most probably increase in BDNF expression might point out the amygdala-dependent emotional and hippocampus-dependent spatial learning-memorial performance of the diabetic rats and also significantly prevented the diabetes-related reduction in the BDNF immunoreactivity in the hippocampus CA1 zones which may to some extent contribute to correct the behavioural functions in the diabetic rats.
Cognitive decline in DM is well known, however, the exact cause is unclear. Increasing evidence suggests that neuroinflammation [Citation26], alterations in cellular calcium homeostasis [Citation34], cholinergic neurotransmission, and NO/cGMP pathway [Citation18,Citation33] may play an important role in diabetes-mediated cognitive impairments. DM is also known to lead functional and structural deficits in the peripheral and CNS as well as cognitive dysfunctions [Citation24,Citation35,Citation36]. Besides, it has been reported in various studies that NO may play a major role in DM. Modulation of sGC by NO has a major role in the modulation of cerebral processes including some forms of learning and memory [Citation37,Citation38]. NO does not only function as a neuronal messenger, it is a strong oxidizing agent and may cause inflammation, nitro-oxidative stress, mitochondrial dysfunction, and apoptosis [Citation39–41]. It has been demonstrated that changes in the activity and expression of both of eNOS and nNOS isoforms play important roles in the cognitive decline in the aged brain via reducing cGMP synthesis [Citation32,Citation42,Citation43]. Furthermore, decreased nNOS expression in the hippocampus might result in a number of cognitive disorders in the diabetic rats [Citation18,Citation30]. It was recently reported that reduced nNOS expression in rat hippocampus might contribute to deficits in hippocampal-dependent learning and LTP in diabetic rats [Citation30]. The recent experimental studies suggest that the exogenously administered YC-1 and zaprinast improve the learning and memory functions, and have a therapeutic potential [Citation15]. Both behavioural and neurochemical beneficial effects of YC-1 in the rats were first reported by Chien et al. [Citation15]. Furthermore, YC-1 improved the spatial working memory and PA memory in the aged male rats [Citation16]. In the study, chronically administrated YC-1 has been shown to markedly shorten the escape latency in the task of water maze and increase the retention scores in PA task in biological aged rats [Citation16]. These results are consistent with the findings of previous data shown by other researchers such as an increase in various learning and memory performances in the naive animals by YC-1 administration and ensure protection against the learning and memorial deficits caused by scopolamine [Citation15]. In addition to the behavioural studies, it was reported that YC-1 significantly increased LTP in hippocampal and amygdala slices via NO-cGMP-PKG-dependent pathway [Citation13]. In the present study, the chronic YC-1 administration had no effect on the locomotor activity of diabetic rats then the animals were trained to find the hidden platform to determine its spatial learning-memory. For the four days when the five separate groups were trained, the platform finding duration was adversely affected in the course of the memoric direction finding, and the exploratory time was reduced and the spatial memory functions were distorted in the platform area, depending on the diabetes compared to the control group even in the probe test. No significant difference was found among the groups during the locomotor activity test and foot shock sensitivity test, hence such a disorder in performance is understood not be a result of the motor or sensational deficit. At the last day of the water maze trial, a probe test was carried out to evaluate the performance of animals in learning the place of platform. The animals in diabetic group performed the explorative behaviour in the quadrant of hidden platform in a shorter time, compared to the control group, while the YC-1 administered diabetic animals demonstrated a performance similar to that of the control group of animals. In the PA test, the first-day performance was identical in all the groups, while the performances evaluated 24 h later were lower depending on the diabetes, and the YC-1 administered diabetic animals had a “second-day latency” identical to the controls. Considering all the behaviour data, the findings obtained in the present study are observed to be consistent with the other available findings. In consequence, 0.1 mg/kg chronically administered YC-1 selectively corrects the behaviour performance in the diabetic rats.
Today there is accumulating evidence that PDEs have noticeable effects on cognition. Especially endogenous PDE-5 has been documented to have a major role in the cognitive functions [Citation31,Citation44,Citation45]. PDE-5 is expressed in certain brain areas such as the cerebellar Purkinje cells [Citation46] hippocampus, caudate, substantia nigra, and cerebellum [Citation47]. It is reported that PDEs can affect neuronal cell survival and also play a part in neurodegenerative diseases, such as Alzheimer’s disease when functioning incorrectly [Citation48]. Many clinical and experimental findings point to the fact that NO-cGMP-PKG is the central mechanism of a network of signalling pathways that interconnects neuroinflammation, neurodegeneration, and cognitive disorders, leading to increased pharmaceutical interest in PDE-5 inhibitors as promising therapeutic agents for neurodiseases. Inhibition of PDE-5 and accumulation of cGMP may inhibit neuroinflammation and improve synaptic plasticity and memory [Citation49,Citation50]. Latest findings suggest that PDE-5 has a crucial role in the processes of cognition under normal and/or pathological situations [Citation50,Citation51]. It also regulates neurogenesis and apoptosis [Citation52]. Although the underlying mechanism has yet to be fully elucidated, it has been suggested that PDE-5 inhibitors enhance memory via a central mechanism [Citation53]. Recently authors have reported diabetes-related cognitive decline and PDE-5 inhibitors might be beneficial to diabetes-induced cognitive deficits in rats [Citation18]. Despite the significant pharmacological effects of zaprinast, less is known about its role in cognition.
In addition, hyperglycaemia recognized as an important cause of diabetes associated cognitive dysfunction using antihyperglycaemic drugs and insulin sensitizers [Citation54,Citation55]. It has been reported that better glycaemic control has been found beneficial in diabetes-induced cognitive deficits [Citation56,Citation57]. However, neither YC-1 nor zaprinast treatment was found to affect blood glucose levels in this study, while improving memory dysfunction in STZ-induced diabetic rats. To our knowledge, there is only one systematic review, which explores glycaemic control in people with diabetes after chronic use of PDE-5 inhibitor [Citation58]. Chronic use of PDE-5 inhibitors, particularly sildenafil, increases NO formation via the endothelial nitric oxide synthase (eNOS) pathway, as shown in an in vitro study [Citation59]. Restoration of cGMP levels by inhibiting PDE-5 could be a prophylactic measure against the development of insulin resistance via improving endothelial dysfunction. Prolongation of NO/cGMP/PKG signalling by PDE-5 inhibitors could favour stimulation of blood flow and improve insulin action on muscle glucose uptake, thereby decreasing the plasma glucose level. It has been also reported that NO/cGMP can stimulate glucose transport to skeletal muscle [Citation60]. However, the available evidence suggests inconclusive benefit of PDE-5 inhibitors on glycaemic control as measured by Haemoglobin A1c in type 2 diabetes. Authors have reported that PDE-5 inhibitors do not affect on glycaemic outcome in hyperinsulinaemic insulin resistance conditions especially in type 2 diabetics due to the insulin-induced upregulation of PDE-5 expression [Citation58]. Therefore, insulin-induced PDE-5 upregulation appears to be one of the reasons of our negative findings. The long-term benefits of PDE-5 inhibitors on glycaemic control remain to be determined. Therefore, useful effects of both YC-1 and zaprinast on cognition in diabetic rats may be independent of the glucose-lowering potential. A similar hypothesis has recently been proposed by our study of diabetic vascular dementia [Citation26].
BDNF is a member of the neurotrophin family of growth factors and plays an important role in cognitive function [Citation61]. Furthermore, BDNF has been shown to play a critical role in the pathophysiology of diabetes known to be decreased and therefore might one of the causes of cognitive deficits in diabetic rats [Citation62,Citation63]. In the current study, BDNF immunoreactivity found significantly decreased in the hippocampus CA1 zones of the diabetic rats in comparison with the nondiabetic control group which could indicate the importance/relation of BDNF in memory deficit in diabetes. Furthermore, YC-1 and zaprinast treatment reversed this effect and caused a significant increase in BDNF in the hippocampus, which might be a part of neuroprotective effects of these drugs. Similar results were reported previously with chronic tadalafil treatment in aged mice where tadalafil enhanced memory by increasing BDNF level and dendritic spine density in apical dendrites on CA1 hippocampal pyramidal neurons, which may contribute to enhancing learning and memory process [Citation64]. Compatible with the previous findings of BDNF levels has shown to be increased in the hippocampus after chronic sildenafil treatment in a mouse model of Alzheimer’s disease [Citation65]. These results are also consistent with previous studies demonstrating that NO contributes to long-term memory via the activation of sGC-cGMP-dependent protein kinase, and CRE-binding protein (CREB) phosphorylation [Citation66]. It is well known that the activation of CREB promotes the transcription of BDNF, which has been proposed to contribute to the memory enhancing effects of sildenafil [Citation67]. In addition, it has recently been demonstrated that CREB is rapidly phosphorylated after treatment of YC-1 and also learning and memory is improved in several tasks involving different brain regions [Citation13].
Our study had certain limitations. First of all, we do not know the detailed underlying mechanism caused by YC-1 and zaprinast although it has been postulated that most probably through NO/cGMP/PKG signalling pathway. Secondly, changes in BDNF expression levels might be related to amygdala-dependent emotional and hippocampus-dependent spatial learning-memorial performance changes but in BDNF expression levels at the related sites, which requires further research in order to be fully understood.
Taken together, the findings of this study show that the long-term administration of YC-1 and zaprinast increases the BDNF levels in hippocampus, and hence might have beneficial effects on cognitive deficits in diabetes. The full mechanism of action and pathologies related with cognitive dysfunctions at a molecular level could be very important for better understanding and might provide novel approaches in the treatment.
Acknowledgement
This study was presented at 25th European College of Neuropsychopharmacology Congress, October 13–17, 2012, Vienna, Austria.
Disclosure statement
No potential conflict of interest was reported by the authors.
References
- Boulton CL, Southam E, Garthwaite J. Nitric oxide-dependent long-term potentiation is blocked by a specific inhibitor of soluble guanylyl cyclase. Neuroscience. 1995;69:699–703. doi: 10.1016/0306-4522(95)00349-N
- Monfort P, Munoz MD, Kosenko E, et al. Long-term potentiation in hippocampus involves sequential activation of soluble guanylate cyclase, cGMP-dependent protein kinase and cGMP-degrading phosphodiesterase. J Neurosci. 2002;22:10116–10122. doi: 10.1523/JNEUROSCI.22-23-10116.2002
- Prickaerts J, Sik A, van Staveren WC, et al. Phosphodiesterase type 5 inhibition improves early memory consolidation of object information. Neurochem Int. 2004;45:915–928. doi: 10.1016/j.neuint.2004.03.022
- Rutten K, Vente JD, Sik A, et al. The selective PDE5 inhibitor, sildenafil, improves object memory in Swiss mice and increases cGMP levels in hippocampal slices. Behav Brain Res. 2005;164:11–16. doi: 10.1016/j.bbr.2005.04.021
- Bernabeu R, Schmitz P, Faillace MP, et al. Hippocampal cGMP and cAMP are differentially involved in memory processing of inhibitory avoidance learning. NeuroReport. 1996;7:585–588. doi: 10.1097/00001756-199601310-00050
- Bernabeu R, Schroder N, Quevedo J, et al. Further evidence for the involvement of a hippocampal cGMP/cGMP-dependent protein kinase cascade in memory consolidation. NeuroReport. 1997;8:2221–2224. doi: 10.1097/00001756-199707070-00026
- Smith S, Dringenberg HC, Bennett BM, et al. A novel nitrate ester reverses the cognitive impairment caused by scopolamine in the Morris water maze. NeuroReport. 2000;11:3883–3886. doi: 10.1097/00001756-200011270-00055
- Piedrafita B, Cauli O, Montoliu C, et al. The function of the glutamate-nitric oxide-cGMP pathway in brain in vivo, learning ability decrease in parallel in mature compared to young rats. Learn Mem. 2007;14:254–258. doi: 10.1101/lm.541307
- Llansola M, Hernandez-Viadel M, Erceg S, et al. Increasing the function of the glutamate-nitric oxide cGMP pathway increases the ability to learn a Y maze task. J Neurosci Res. 2009;87:2351–2355. doi: 10.1002/jnr.22064
- Xu Y, Zhang HT, O’Donnell JM. Phosphodiesterases in the central nervous system: implications in mood and cognitive disorders. Handb Exp Pharmacol. 2011;204:447–485. doi:10.1007/978-3-642-17969-3-19 doi: 10.1007/978-3-642-17969-3_19
- Ko FN, Wu CC, Kuo SC, et al. YC-1, a novel activator of platelet guanylate cyclase. Blood. 1994;84:4226–4233.
- Mulsch A, Bauersachs J, Schafer A, et al. Effect of YC-1, an NO-independent, superoxide-sensitive stimulator of soluble guanylyl cyclase, on smooth muscle responsiveness to nitrovasodilators. Br J Pharmacol. 1997;120:681–689. doi: 10.1038/sj.bjp.0700982
- Chien WL, Liang KC, Teng CM, et al. Enhancement of long-term potentiation by a potent nitric oxide-guanylyl cyclase activator, 3-(5-Hydroxymethyl-2-furyl)-1-benzyl-indazole. Mol Pharmacol. 2003;63(6):1322–1328. doi: 10.1124/mol.63.6.1322
- Chien WL, Liang KC, Fu WM. Enhancement of active shuttle avoidance response by the NO-cGMP-PKG activator YC-1. Eur J Pharmacol. 2008;590(1-3):233–240. doi: 10.1016/j.ejphar.2008.06.040
- Chien WL, Liang KC, Teng CM, et al. Enhancement of learning behaviour by a potent nitric oxide-guanylate cyclase activator YC-1. Eur J Neurosci. 2005;21(6):1679–1688. doi: 10.1111/j.1460-9568.2005.03993.x
- Komsuoglu Celikyurt I, Utkan T, Ozer C, et al. Effects of YC-1 on learning and memory functions of aged rats. Med Sci Monit Basic Res. 2014;20:130–137. doi: 10.12659/MSMBR.891064
- Francis SH, Blount MA, Corbin JD. Mammalian cyclic nucleotide phosphodiesterases: molecular mechanisms and physiological functions. Physiol Rev. 2011;91:651–690. doi: 10.1152/physrev.00030.2010
- Patil CS, Singh VP, Kulkarni SK. Modulatory effect of sildenafil in diabetes and electroconvulsive shock-induced cognitive dysfunction in rats. Pharmacol Rep. 2006;58(3):373–380.
- Murray KJ. Phosphodiesterase VA inhibitors. Drug News Perspect. 1993;6:150–156.
- Zhang X, Feng Q, Cote RH. Efficacy and selectivity of phosphodiesterase-targeted drugs to inhibit photoreceptor phosphodiesterase (PDE6) in retinal photoreceptors. Invest Ophthalmol Vis Sci. 2005;46(9):3060–3066. doi: 10.1167/iovs.05-0257
- Medina P, Segarra G, Martinez-Leon JB, et al. Relaxation induced by cGMP phosphodiesterase inhibitors sildenafil and zaprinast in human vessels. Ann Thorac Surg. 2000;70:1327–1331. doi: 10.1016/S0003-4975(00)01914-7
- Zhuo M, Hu Y, Schultz C, et al. Role of guanylyl cyclase and cGMP-dependent protein kinase in long-term potentiation. Nature. 1994;368:635–639. doi: 10.1038/368635a0
- Feil R, Kleppisch T. NO/cGMP-dependent modulation of synaptic transmission. Handb Exp Pharmacol. 2008;184:529–560. doi: 10.1007/978-3-540-74805-2_16
- American Diabetes Association. Diagnosis and classification of diabetes mellitus. Diabetes care. 2009; 32(Suppl 1): S62–S67.
- Baydas G, Nedzvetskii VS, Nerush PA, et al. Altered expression of NCAM in hippocampus and cortex may underlie memory and learning deficits in rats with streptozotocin-induced diabetes mellitus. Life Sci. 2003;73(15):1907–1916. doi: 10.1016/S0024-3205(03)00561-7
- Utkan T, Yazir Y, Karson A, et al. Etanercept improves cognitive performance and increases eNOS and BDNF expression during experimental vascular dementia in streptozotocin-induced diabetes. Curr Neurovasc Res. 2015;12(2):135–146. doi: 10.2174/1567202612666150311111340
- Tuzcu M, Baydas G. Effect of melatonin and vitamin E on diabetes-induced learning and memory impairment in rats. Eur J Pharmacol. 2006;537(1-3):106–110. doi: 10.1016/j.ejphar.2006.03.024
- Jaiswal S, Torgal SS, Mishra S. Neuroprotective effect of epalrestat on memory impairment in streptozotocin-induced type-2 diabetic rats using different behavioral models. Asian J Pharm Clin Res. 2018;11:411–415. doi: 10.22159/ajpcr.2018.v11i1.23313
- Manschot SM, Biessels GJ, Cameron NE, et al. Angiotensin converting enzyme inhibition partially prevents deficits in water maze performance, hippocampal synaptic plasticity and cerebral blood flow in streptozotocin-diabetic rats. Brain Res. 2003;966(2):274–282. doi: 10.1016/S0006-8993(02)04211-7
- Reagan LP, McEwen BS. Diabetes, but not stress, reduces neuronal nitric oxide synthase expression in rat hippocampus: implications for hippocampal synaptic plasticity. NeuroReport. 2002;13(14):1801–1804. doi: 10.1097/00001756-200210070-00022
- Domek-Łopacinska K, Strosznajder JB. The effect of selective inhibition of cyclic GMP hydrolyzing phosphodiesterases 2 and 5 on learning and memory processes and nitric oxide synthase activity in brain during aging. Brain Res. 2008;1216:68–77. doi: 10.1016/j.brainres.2008.02.108
- Utkan T, Gocmez SS, Ozer C, et al. Selective and nonselective neuronal NOS inhibitors impair cognitive function in the three panel runway and passive avoidance tasks in rats. Pharmacol Biochem Behav. 2012;101(4):515–519. doi: 10.1016/j.pbb.2012.02.020
- Schmatz R, Mazzanti CM, Spanevello R, et al. Resveratrol prevents memory deficits and the increase in acetylcholinesterase activity in streptozotocin-induced diabetic rats. Eur J Pharmacol. 2009;610(1-3):42–48. doi: 10.1016/j.ejphar.2009.03.032
- Cameron NE, Eaton SE, Cotter MA, et al. Vascular factors and metabolic interactions in the pathogenesis of diabetic neuropathy. Diabetologia. 2001;44(11):1973–1988. doi: 10.1007/s001250100001
- Moheet A, Mangia S, Seaquist ER. Impact of diabetes on cognitive function and brain structure. Ann NY Acad Sci. 2015;1353(1):60–71. doi: 10.1111/nyas.12807
- Sima AAF, Kamiya H, Lia ZG. Insulin, C-peptide, hyperglicemia, and central nervous system complications in diabetes. Eur J Pharmacol. 2004;490:187–197. doi: 10.1016/j.ejphar.2004.02.056
- Hawkins RD, Son H, Arancio O. Nitric oxide as a retrograde messenger during long-term potentiation in hippocampus. Prog Brain Res. 1998;118:155–172. doi: 10.1016/S0079-6123(08)63206-9
- Neitz A, Mergia E, Imbrosci B, et al. Postsynaptic NO/cGMP increases NMDA receptor currents via hyperpolarization-activated cyclic nucleotide-gated channels in the hippocampus. Cereb Cortex. 2014;24(7):1923–1936. doi: 10.1093/cercor/bht048
- Law A, Gauthier S, Quirion R. Say NO to Alzheimer’s disease: the putative links between nitric oxide and dementia of Alzheimer’s type. Brain Res Brain Res Rev. 2001;35:73–96. doi: 10.1016/S0165-0173(00)00051-5
- Malinski T. Nitric oxide and nitroxidative stress in Alzheimer’s disease. J Alzheimers Dis. 2007;11(2):207–218. doi: 10.3233/JAD-2007-11208
- Chalimoniuk M, Strosznajder JB. Aging modulates nitric oxide synthesis and cGMP levels in hippocampus and cerebellum: effects of amyloid β peptide. Mol Chem Neuropathol. 1999;35(1–3):77–95. doi: 10.1007/BF02815117
- Kelly MP. Cyclic nucleotide signaling changes associated with normal aging and age-related diseases of the brain. Cell Sign. 2018;42:281–291. doi: 10.1016/j.cellsig.2017.11.004
- Domek-Łopacińska KU, Strosznajder JB. Cyclic GMP and nitric oxide synthase in aging and Alzheimer’s disease. Mol Neurobiol. 2010;41(2-3):129–137. doi: 10.1007/s12035-010-8104-x
- Yin C, Deng Y, Gao J, et al. Icariside II, a novel phosphodiesterase-5 inhibitor, attenuates streptozotocin-induced cognitive deficits in rats. Neurosci. 2016;328:69–79. doi: 10.1016/j.neuroscience.2016.04.022
- Prickaerts J, Steinbusch HW, Smits JF, et al. Possible role of nitric oxide-cyclic GMP pathway in object recognition memory: effects of 7-nitroindazole and zaprinast. Eur J Pharmacol. 1997;337(2–3):125–136. doi: 10.1016/S0014-2999(97)01301-0
- Shimizu-Albergine M, Rybalkin SD, Rybalkina IG, Feil R, et al. Individual cerebellar Purkinje cells express different cGMP phosphodiesterases (PDEs): in vivo phosphorylation of cGMP-specific PDE (PDE5) as an indicator of cGMP-dependent protein kinase (PKG) activation. J Neurosci. 2003;23(16):6452–6459. doi: 10.1523/JNEUROSCI.23-16-06452.2003
- Van Staveren WC, Steinbusch HW, Markerink-Van Ittersum M, et al. mRNA expression patterns of the cGMP-hydrolyzing phosphodiesterases types 2, 5, and 9 during development of the rat brain. J Comp Neurol. 2003;467(4):566–580. doi: 10.1002/cne.10955
- Bollen E, Prickaerts J. Phosphodiesterases in neurodegenerative disorders. IUBMB Life. 2012;64(12):965–970. doi: 10.1002/iub.1104
- Zhang J, Guoab J, Zhao Z, et al. Phosphodiesterase-5 inhibitor sildenafil prevents neuroinflammation, lowers beta-amyloid levels and improves cognitive performance in APP/PS1 transgenic mice. Behav Brain Res. 2013;250:230–237. doi: 10.1016/j.bbr.2013.05.017
- Peixoto CA, Nunes AK, Garcia-Osta A. Phosphodiesterase-5 inhibitors: action on the signaling pathways of neuroinflammation, neurodegeneration, and cognition. Mediators Inflamm. 2015;2015:1–17. doi:10.1155/2015/940207.
- Schmidt CJ. Phosphodiesterase inhibitors as potential cognition enhancing agents. Curr Top Med Chem. 2010;10(2):222–230. doi: 10.2174/156802610790411009
- Sabayan B, Zamiri N, Farshchizarabi S, et al. Phosphodiesterase-5 inhibitors: novel weapons against Alzheimer’s disease? Int J Neurosci. 2010;120(12):746–751. doi:10.3109/00207454.2010.520381.
- Akkerman S, Blokland A, van Goethem N, et al. PDE5 inhibition improves acquisition processes after learning via a central mechanism. Neuropharmacology. 2015;97:233–239. doi: 10.1016/j.neuropharm.2015.04.019
- Messier C. Impact of impaired glucose tolerance and type 2 diabetes on cognitive aging. Neurobiol Aging. 2005;26(Suppl 1):26–30. doi: 10.1016/j.neurobiolaging.2005.09.014
- Ryan CM, Freed MI, Rood JA, et al. Improving metabolic control leads to better working memory in adults with type 2 diabetes. Diabetes Care. 2006;29(2):345–351. doi: 10.2337/diacare.29.02.06.dc05-1626
- Suji G, Sivakami S. Approaches to the treatment of diabetes mellitus: an overview. Cell Mol Biol (Noisy-le-grand). 2003;49(4):635–639.
- Biessels GJ, Kerssen A, de Haan EH, et al. Cognitive dysfunction and diabetes: implications for primary care. Prim Care Diabetes. 2007;1(4):187–193. doi: 10.1016/j.pcd.2007.10.002
- Poolsup N, Suksomboon N, Aung N. Effect of phosphodiesterase-5 inhibitors on glycemic control in person with type 2 diabetes mellitus: A systematic review and meta-analysis. J Clin Trans Endocrinol. 2016;6:50–55.
- Mammi C, Pastore D, Lombardo MF, et al. Sildenafil reduces insulin-resistance in human endothelial cells. PLoS One. 2011;6:e14542. doi: 10.1371/journal.pone.0014542
- Etgen GJ, Fryburg DA, Gibbs EM. Nitric oxide stimulates skeletal muscle glucose transport through a calcium/contraction-and phosphatidylinositol-3-kinase-independent pathway. Diabetes. 1997;46:1915–1919. doi: 10.2337/diab.46.11.1915
- Yamada K, Nabeshima T. Brain-derived neurotrophic factor/TrkB signaling in memory processes. J Pharmacol Sci. 2003;91(4):267–270. doi: 10.1254/jphs.91.267
- Caletti G, Almeida FB, Agnes G, et al. Antidepressant dose of taurine increases mRNA expression of GABAA receptor α2 subunit and BDNF in the hippocampus of diabetic rats. Behav Brain Res. 2015; 283:11–15. doi: 10.1016/j.bbr.2015.01.018
- Etemad A, Sheikhzadeh F, Asl NA. Evaluation of brain-derived neurotrophic factor in diabetic rats. Neurol Res. 2015;37(3):217–222. doi: 10.1179/1743132814Y.0000000428
- García-Barroso C, Ricobaraza A, Pascual-Lucas M, et al. Tadalafil crosses the blood-brain barrier and reverses cognitive dysfunction in a mouse model of AD. Neuropharmacology. 2013;64:114–123. doi: 10.1016/j.neuropharm.2012.06.052
- Cuadrado-Tejedor M, Hervias I, Ricobaraza A, et al. Sildenafil restores cognitive function without affecting β-amyloid burden in a mouse model of Alzheimer’s disease. Br J Pharmacol. 2011; 164(8):2029–2041.
- Paul C, Stratil C, Hofmann F, et al. cGMP-dependent protein kinase type I promotes CREB/CRE-mediated gene expression in neurons of the lateral amygdala. Neurosci Lett. 2010; 473(2):82–86. doi: 10.1016/j.neulet.2010.02.020
- Puerta E, Hervias I, Barros-Minones L, et al. Sildenafil protects against 3-nitropropionic acid neurotoxicity through the modulation of calpain CREB, and BDNF. Neurobiol Dis. 2010; 38(2):237-245. doi: 10.1016/j.nbd.2010.01.013