ABSTRACT
OBJECTIVE: In pain management, alternative medications are necessary due to the development of tolerance to traditional opioid analgesics. Literature data suggest that N-methyl-D-aspartate (NMDA) receptor antagonizing drugs can induce antinociception, and can reduce the opioid requirement. Ketamine is a non-competitive NMDA receptor antagonist drug and has well-known antinociceptive properties. The drug acts not only on NMDA receptors but also has effects on the monoaminergic system and non-NMDA glutamatergic receptors which have vital roles in the regulation of pain. This study was conducted to investigate the serotonergic and glutamatergic involvement in low-dose ketamine (20 mg/kg) analgesia in mice.
METHOD: The effects of serotonin were suppressed with two different ways; either the serotonin was depleted with p-chlorophenylalanine (pCPA, 150 mg/kg/d; 4 days) or the serotonin receptors were blocked with methiothepin (0.1 mg/kg), and α-amino-3-hydroxy-5-methyl-4-isoxazole propionic acid (AMPA) receptors were antagonized with GYKI-52466 (20 mg/kg). Fluoxetine (20 mg/kg; 7 days) was used to increase the serotoninergic activity. We used a hotplate (HP) test to measure pain reaction latencies. Furthermore, we tested sustained analgesic effects of ketamine for six consecutive times (1-hour break between each test).
RESULTS: In our experiment, ketamine treatment increased pain reaction latencies, yet it failed to increase the latencies when combined with antiserotonergic drugs, e.g. pCPA and methiothepin. The latencies were increased with AMPA receptor blockade, yet ketamine did not increase the analgesic effect of the AMPA receptor antagonist, i.e. GYKI-52466. In consecutive tests, ketamine was effective for 5 h, and the peak effect was seen at the 3rd-hour test.
CONCLUSION: Our data suggest that the activity of the serotonergic system and AMPA receptors are necessary for ketamine to produce antinociceptive effects. In pain management, ketamine can offer an alternative option to traditional analgesics and may be useful to reduce opioid tolerance.
Introduction
Traditional opioid analgesics are common and preferred therapies in pain management. However, the development of tolerance and hyperalgesia can discredit the analgesic potency of opioid drugs [Citation1]. Searching for alternative analgesic medications to be used alone or as adjunct therapies to reduce opioid usage and prevent tolerance development are priorities of research and drug development.
Previous studies have reported that a variety of N-methyl-D-aspartate (NMDA) receptor antagonist drugs can induce antinociception [Citation2,Citation3]. Ketamine is a non-competitive NMDA receptor antagonist with unique properties. It was introduced almost half a century ago to serve as a mono anesthetic drug providing analgesia, amnesia, loss of consciousness and immobility [Citation4]. However, reported significant side effects led to a diminishment in the role of ketamine in anesthesia [Citation5]. It was continuously studied in the desire to find an alternative therapy to treat chronic and perioperative pain. Current research indicated that low-dose ketamine could produce profound analgesia with fewer side effects [Citation6]. Despite that, the analgesic mechanism of action of ketamine is still not entirely clear.
A growing body of evidence suggests that glutamatergic mechanisms are involved in the production of pain [Citation7]. Administration of excitatory amino acid receptor agonists can induce pain in animal models [Citation8] while antagonizing these receptors can produce antinociception [Citation9].
Numerous studies suggest that perception of pain is closely related to serotonin. Alterations in serotonin levels in the brain lead to pain-altering effects [Citation10]. It is reported that ketamine increases serotonin-mediated transmission [Citation11]. Therefore, interactions of ketamine with the serotonergic system may be a way to explain its effects on pain.
The present study was performed to reveal the involvement of serotonergic and glutamatergic systems in ketamine analgesia and to test prolonged effects of ketamine on pain. A hotplate (HP) test was used to measure reaction latencies to the stress of heat.
Methods
Animals
104 male inbred BALB/c mice weighing 35–45 g and 10–12 weeks old were used in this experiment. The animals were housed in an animal colony facility for two weeks before the testing day and maintained at constant room temperature (22 ± 2) under standard laboratory conditions (12-h light/dark cycle, light onset at 07:00 h). All animals received tap water and food pellets ad libitum. The mice were naive about the procedure and tested individually. Experiments were performed between 09:00 and 15:00 h. All procedures in this study complied ethically according to The European Community Council Directive 86 (24 November 1986; 86/609/EEC) with the approval of the local Ethics Committee (DUHADEK, Approval date: 2014, Number:2014–12).
Experimental groups and drug administration
Drugs obtained from Sigma (St. Louis, MO, USA) were dissolved in saline and administered intraperitoneally (i.p.) in a volume of 0.1 ml/10 g body weight. Mice were separated into 14 groups (n = 8) and were treated with fluoxetine (20 mg/kg, 7 days) and a serotonin depleting agent; p-chlorophenylalanine (pCPA, 150 mg/kg, 4 days) or %0.9 saline. Drugs were administered once daily until the experiment day. A serotonin antagonist; methiothepin (0.1 mg/kg), an α-amino-3-hydroxy-5-methyl-4-isoxazole propionic acid (AMPA) receptor antagonist; GYKI-52466 (20 mg/kg), an analgesic; metamizole (500 mg/kg); and ketamine (20 mg/kg) were administered 60 mins before the test procedure. Doses of the drugs were selected according to previous studies. An HP test was performed for a single time for these groups. Three groups (vehicle, metamizole, and ketamine) were separated in order to test the sustained analgesic effect of ketamine. The HP test was performed for 6 consecutive times with a 1-hour break between each test [Citation12].
Hotplate test
The HP test was used to measure pain reaction latencies. Mice were individually placed into a square glass container on a hot plate (maintained at 55 ± 0.1 C). Mice were kept on the test apparatus for 60 s if no reaction were recorded. The time passing to lick the hind paws or jumping was recorded and considered as the index of pain reaction, and the test ended afterward.
Statistical analysis
The results of the single HP test were evaluated using one-way analysis of variance (ANOVA) followed by a post hoc Tukey test if significant differences were detected. To test the differences in the repeated trials of the same drug or vehicle treatment applied mice, we used one-way, within-subjects ANOVA. The data are presented as mean values and ± SEM. Differences were considered statistically significant if the p-value was less than 0.05.
Results
In the HP test, pain reaction latencies were increased with metamizole (p < 0.01), ketamine (p < 0.01), fluoxetine (p < 0.01), fluoxetine-ketamine (p < 0.05), GYKI-52466 (p < 0.05) and GYKI-52466-ketamine (p < 0.05) compared to control group (). Latencies were higher in GYKI-52466-ketamine combination compared to GYKI-52466 alone, but the difference was not statistically significant ().
Figure 1. The latency of the hind paw licking or jumping of groups on the hot plate test. Each column represents the mean ± SEM of 7–9 mice. *p < 0.05; **p < 0.01; compared with control group. One-way ANOVA; post hoc Tukey test was used for statistical analysis.
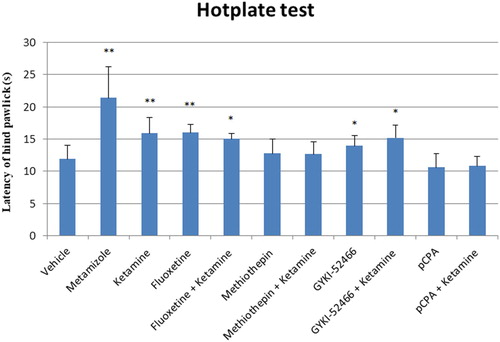
Figure 2. The latency of hind paw licking or jumping of groups on the hot plate test. Each column represents the mean ± SEM of 7–9 mice. *p < 0.05 compared with control group. One-way ANOVA; post hoc Tukey test was used for statistical analysis.
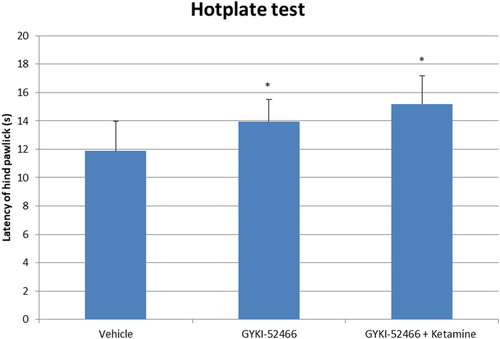
In consecutive tests, ketamine increased pain reaction latencies in the 1st, 2nd, 3rd, 4th and 5th-hour tests compared to vehicle-treated group (p < 0.05 for all). In the 6th hour test, the difference did not reach statistical significance. Metamizole increased reaction latencies in the 1st (p < 0.01), 2nd (p < 0.05) and 3rd (p < 0.05) hour tests. The difference did not reach statistical significance in the last 3 tests. In the 1st hour test, latencies were higher in the metamizole treated group while in the 2nd-hour and the following tests, latencies were lower in metamizole group compared to ketamine group, although the differences were not significant. We also examined pain latency differences between each hour test, although no significant difference was observed ().
Figure 3. The latency of the hind paw licking or jumping of groups on the hot plate test. Each column represents the mean ± SEM of 7–9 mice. *p < 0.05; **p < 0.01 compared with control group. One-way ANOVA; post hoc Tukey test was used for statistical analysis. To test the differences in the repeated tests of the same drug treatment applied mice, we used one-way, within-subjects ANOVA.
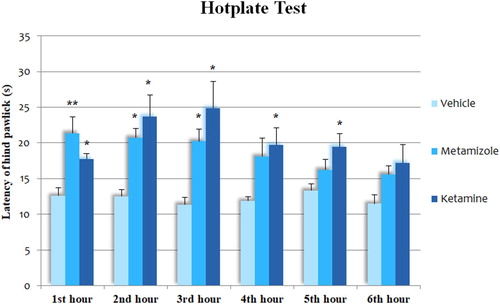
Discussion
In this study, pain reaction latencies in the HP test were increased with serotonin-enhancing drugs, e.g. ketamine and fluoxetine. On the other hand, ketamine failed to increase pain reaction latencies when combined with a serotonin receptor antagonist; methiothepin and a serotonin depleting agent; pCPA. These results indicate that ketamine analgesia is closely associated with the presence of serotonin and the activity of serotonin receptors.
Changes in serotoninergic transmission result in altered pain perception [Citation13]. Animal studies have noted that serotonin-enhancing drugs induce analgesia [Citation14] while suppression of the serotonergic system can inhibit the analgesic effects of the drugs [Citation15]. Studies also pointed out that ketamine inhibits serotonin reuptake and increases serotonin transmission in the brain [Citation11]. These changes appear to be necessary for ketamine to express its antinociceptive effects. In this manner, monoaminergic interactions of ketamine may be a way to explain its antinociceptive mechanism of action.
In the present study, GYKI-52466, a non-competitive AMPA receptor antagonist, exhibited antinociceptive effects while a combination with ketamine did not make a significant difference. An increasing body of evidence suggests that glutamatergic mechanisms and glutamate receptors are involved in the regulation of pain, i.e. pain transmission [Citation16]. Studies implied that glutamate acts as a nociceptive neurotransmitter. In animal and human studies, it is suggested that AMPA receptor agonist administration increases pain [Citation17] while antagonist drugs were reported to produce specific analgesic effects [Citation18]. Studies reported that ketamine administration increases glutamatergic transmission on AMPA receptors [Citation19]. Ketamine-induced antinociception that increases AMPA receptor activity indicates that different mechanisms are involved in antinociceptive effects of ketamine and GYKI-52466.
In our experiment, the antinociceptive effect of ketamine lasted for 5 h and the peak effect was seen at the 3rd-hour test. When the short plasma half-life of ketamine is considered, its pain-diminishing effects last much longer. The main metabolite, norketamine, has a more extended plasma half-life than ketamine [Citation20]. It is stated that the metabolites of ketamine (norketamine, dehydronorketamine, and hydroxynorketamine) may play an essential role in its sustained analgesia [Citation21].
Patients with chronic pain often have tolerance to traditional analgesic drugs due to constant use, and they suffer from enhanced pain sensitivity [Citation22]. Alternative medications with a different mechanism of action, such as NMDA and AMPA receptor antagonists [Citation23] may promise a new aspect to reduce or to replace opioid usage in pain management. Many studies have emphasized that ketamine might be beneficial for patients with conditions like acute pain, perioperative pain, chronic pain, and cancer-related pain [Citation24–26]. However, some studies reported that ketamine has little or no opioid-sparing effect [Citation27]. Several factors may contribute to variations in the results including differences in premedication, pathologic conditions, age, genetic polymorphism and dose range.
The neuropharmacology of ketamine is still complex. The drug interacts with a variety of binding sites, including NMDA, nicotinic [Citation28], muscarinic [Citation29], monoaminergic [Citation30] and opioid receptors [Citation31]. These various interactions may contribute to ketamine's anesthetic, analgesic, sedative, and mood-altering properties [Citation32].
Even though the antinociceptive effects of ketamine have been reported in various preclinical and clinical studies, physicians have an ongoing debate on its use in adult patients. Most cited concerns are its psychotic effects, restrictions and inadequate literature on adult use compared to children [Citation33]. Due to the conflict about its risk-benefit ratio, ketamine is still acknowledged as a controversial analgesic drug.
Limitation
We aimed to assess the potential involvement of serotonergic and glutamatergic systems after a single-dose S (+)-ketamine administration. Analgesic effects of repetitive ketamine administrations and tolerance development potential of the drug are yet not clear. In further studies, the effects of R (−)-ketamine and its metabolites along with different receptor blockers should be examined. The best dose and application frequency to relieve pain, along with its side-effects should be elaborately investigated in further studies.
Conclusion
Our results provide evidence that the antinociceptive effect of ketamine is closely associated with the existence of serotonin and the activity of serotonin receptors. If serotonin is depleted or its receptors are blocked, ketamine analgesia tends to disappear. AMPA receptor blockage was shown to reduce the sense of pain, yet a combination with ketamine did not induce more analgesia. With its long pain-relieving effects, ketamine can be considered as a good candidate for future pain therapy protocols.
With its public antinociceptive properties, ketamine may provide an alternative therapeutic option for the practitioner and the patient in pain management. Still, clinical benefits and risks should be clarified to consider its widespread use. More studies should be performed to reveal the underlying mechanisms of ketamine analgesia and the influence of its metabolites in this outcome. In light of the latest findings, indications of ketamine may need to be reviewed.
Disclosure statement
No potential conflict of interest was reported by the authors.
Additional information
Funding
References
- Roeckel LA, Utard V, Reiss D, et al. Morphine-induced hyperalgesia involves mu opioid receptors and the metabolite morphine-3-glucuronide. Sci Rep. 2017;7(1):10406.
- Hipólito L, Fakira AK, Cabañero D, et al. In vivo activation of the SK channel in the spinal cord reduces the NMDA receptor antagonist dose needed to produce antinociception in an inflammatory pain model. Pain. 2015;156(5):849–858.
- Niesters M, Dahan A. Pharmacokinetic and pharmacodynamic considerations for NMDA receptor antagonists in the treatment of chronic neuropathic pain. Expert Opin Drug Metab Toxicol. 2012;8(11):1409–1417.
- Rolly G. Use of ketamine as monoanesthetic in clinical anesthesia, acid-base status and oxygenation. In: Kreuscher H, editors. Ketamine. Berlin Heidelberg: Springer; 1969. p. 117–129.
- Goulart BK, De Lima MNM, De Farias CB, et al. Ketamine impairs recognition memory consolidation and prevents learning-induced increase in hippocampal brain-derived neurotrophic factor levels. Neuroscience. 2010;167(4):969–973.
- Ahern TL, Herring AA, Stone MB, et al. Effective analgesia with low-dose ketamine and reduced dose hydromorphone in ED patients with severe pain. Am J Emerg Med. 2013;31(5):847–851.
- Chiechio S, Nicoletti F. Metabotropic glutamate receptors and the control of chronic pain. Curr Opin Pharmacol. 2012;12(1):28–34.
- Castrillon EE, Cairns BE, Wang K, et al. Comparison of glutamate-evoked pain between the temporalis and masseter muscles in men and women. Pain. 2012;153(4):823–829.
- Chen SR, Samoriski G, Pan HL. Antinociceptive effects of chronic administration of uncompetitive NMDA receptor antagonists in a rat model of diabetic neuropathic pain. Neuropharmacology. 2009;57(2):121–126.
- Ossipov MH, Dussor GO, Porreca F. Central modulation of pain. J Clin Invest. 2010;120(11):3779–3787.
- Yamamoto S, Ohba H, Nishiyama S, et al. Subanesthetic doses of ketamine transiently decrease serotonin transporter activity: a PET study in conscious monkeys. Neuropsychoph. 2013;38(13):2666–2674.
- Carbone ET, Lindstrom KE, Diep S, et al. Duration of action of sustained-release buprenorphine in 2 strains of mice. J Am Assoc Lab Anim Sci. 2012;51(6):815–819.
- Ozdemir E, Gursoy S, Bagcivan I. The effects of serotonin/norepinephrine reuptake inhibitors and serotonin receptor agonist on morphine analgesia and tolerance in rats. J Physiol Sci. 2012;62(4):317–323.
- Villarinho JG, de Vargas Pinheiro K, de Vargas Pinheiro F, et al. The antinociceptive effect of reversible monoamine oxidase-A inhibitors in a mouse neuropathic pain model. Progress in Neuro-Psychopharmacology and Biological Psychiatry. 2013;44:136–142.
- Mallet C, Daulhac L, Bonnefont J, et al. Endocannabinoid and serotonergic systems are needed for Acetaminophen-induced analgesia. Pain. 2008;139(1):190–200.
- Vincent K, Wang S F, Laferrière A, et al. Spinal intracellular metabotropic glutamate receptor 5 (mGluR5) contributes to pain and c-fos expression in a rat model of inflammatory pain. Pain. 2017;158(4):705–716.
- Gibson W, Arendt-Nielsen L, Sessle BJ, et al. Glutamate and capsaicin-induced pain, hyperalgesia and modulatory interactions in human tendon tissue. Exp Brain Res. 2009;194(2):173–182.
- Lee HJ, Pogatzki-Zahn EM, Brennan TJ. The effect of the AMPA/kainate receptor antagonist LY293558 in a rat model of postoperative pain. J Pain. 2006;7(10):768–777.
- Tizabi Y, Bhatti BH, Manaye KF, et al. Antidepressant-like effects of low ketamine dose is associated with increased hippocampal AMPA/NMDA receptor density ratio in female Wistar–kyoto rats. Neurosci. 2012;213:72–80.
- Moaddel R, Venkata SLV, Tanga MJ, et al. A parallel chiral–achiral liquid chromatographic method for the determination of the stereoisomers of ketamine and ketamine metabolites in the plasma and urine of patients with complex regional pain syndrome. Talanta. 2010;82(5):1892–1904.
- Holtman JR, Jr., Crooks PA, Johnson-Hardy JK, et al. Effects of norketamine enantiomers in rodent models of persistent pain. Pharmacol Biochem Behav. 2008;90(4):676–685.
- Bannister K, Dickenson AH. Opioid hyperalgesia. Curr Opin Support Palliat Care. 2010;4(1):1–5.
- Wallace MS, Lam V, Schettler J. NGX426, an oral AMPA-kainate antagonist, is effective in human capsaicin-induced pain and hyperalgesia. Pain Med. 2012;13(12):1601–1610.
- Loftus RW, Yeager MP, Clark JA, et al. Intraoperative ketamine reduces perioperative opiate consumption in opiate-dependent patients with chronic back pain undergoing back surgery. Anesthesiology: J Am Soc of Anesthesiologists. 2010;113(3):639–646.
- Eldufani J, Nekoui A, Blaise G. Nonanesthetic effects of Ketamine: a review article. Am J Med. 2018;131(12):1418–1424.
- Currow DC, Hardy J, Quinn S, et al. A randomized, double-blind, placebo-controlled study to assess the efficacy and toxicity of subcutaneous ketamine in the management of cancer pain. J Clin Oncol. 2012;30(15):2604–2604.
- Sveticic G, Farzanegan F, Zmoos P, et al. Is the combination of morphine with ketamine better than morphine alone for postoperative intravenous patient-controlled analgesia? Anesth Analg. 2008;106(1):287–293.
- Udesky JO, Spence NZ, Achiel R, et al. The role of nicotinic inhibition in ketamine-induced behavior. Anesth Analg. 2005;101(2):407–411.
- Hirota K, Hashimoto Y, Lambert DG. Interaction of intravenous anesthetics with recombinant human M1-M3 muscarinic receptors expressed in Chinese hamster ovary cells. Anesth Analg. 2002;95(6):1607–1610.
- Engin E, Treit D, Dickson CT. Anxiolytic-and antidepressant-like properties of ketamine in behavioral and neurophysiological animal models. Neurosci. 2009;161(2):359–369.
- Kosson D, Klinowiecka A, Kosson P, et al. Intrathecal antinociceptive interaction between the NMDA antagonist ketamine and the opioids, morphine and biphalin. Eur J Pain. 2008;12(5):611–616.
- Zhang G F, Wang J, Han J F, et al. Acute single dose of ketamine relieves mechanical allodynia and consequent depression-like behaviors in a rat model. Neurosci Lett. 2016;631:7–12.
- Richards JR, Rockford RE. Low-dose ketamine analgesia: patient and physician experience in the ED. Am J Emerg Med. 2013;31(2):390–394.