Abstract
Without pure water, it is impossible to survive for any living beings. The ratio of freshwater on our planet is very poor and the demand is increasing with time for the growing population. Furthermore, water is being contaminated by industrial and agricultural activities, pharmaceuticals, technocratic civilization, pesticides, garments, global changes etc. In addition to this, environmental pollution and global warming are swelling due to the greenhouse and harmful gases generated from the dumping and burning of fossil fuel. Addressing these problems, it is necessary to find out the cost-effective and environmental friendly processes to purify the contaminated water and air. Activated carbons (ACs) are one of the best solutions for removing the pollutants from aqueous and atmosphere as it is the carbonaceous materials with a high degree of porosity, well-developed surface area, and distinguished functional groups which are required for elimination of contaminants. The preparations of activated carbon are easy and safe processes, mainly from the pyrolysis or gasification of biomass with heat and/or chemicals. The recycling and regeneration of activated carbon after use are also essential for resource maintenance and environmental safety. Thus, AC can protect the ecosystem in a double direction by purifying the water and air from the pollutants.
1. Introduction
As the world’s population continues to grow, the demand for water increasing by 1% annually (WWAP/UN-Water, 2018). The population increase corresponds to the economic growth and development leading to rise in the use of chemical compounds, industrial compounds, agricultural wastes which pose the risk of polluting the existing water sources (Mohammad Razi, Al-Gheethi, Al-Qaini, and Yousef, Citation2018; Oladipo, Ifebajo, Nisar, and Ajayi, Citation2017; Qaisrani, Shams, Zhenren, Reza, and Zainuddin, Citation2018). It has reported that 663 million people have lacked access to clean water and 2.2 million deaths occurring due to diarrhea caused by inadequate sanitation and ingestion of contaminated potable water (Huang et al., Citation2018). The river, lake, and groundwater sources are contaminated due to fertilizers, pesticides, antibiotics, dyes, heavy metals from the industry which results in diseases like cancer, skin defect, kidney damage, liver problems, etc. (Ahmad and Danish, Citation2018). On the other hand, the burning of fossil fuel generates the greenhouse (GHG) and harmful gases like CO2, CH4, H2S, NO2 to the environment which are increasing with current energy demand for the rapid economic development (Ahmed, Abu Bakar, Azad, Sukri, and Mahlia, Citation2018a). Therefore, it is highly desirable to find the cheap and environmentally friendly adsorbent to get rid of the pollutants from water and air. Different scientific techniques have been developed to purify the water contamination and the most widely used of them are listed in .
Table 1. Some of the commonly used water purification methods.
Of the above techniques, adsorption is one of the most popular methods used for the elimination of contaminants from wastewater due to its features of cost-effective, easy to operate, environment-friendly, low health risk, and non-destructive process (Balasundram et al., Citation2017; Oladipo and Gazi, Citation2015; Oladipo and Ifebajo, Citation2018). The addition of activated carbon (AC) in the adsorption process aids in the removal of a wide range of contaminants and carcinogenic compounds such as pharmaceuticals, metallic and non-metallic pollutants, dye and even taste and odor from aqueous solutions (Din, Ashraf, & Intisar, Citation2017). In comparison with other adsorbents like zeolite, clays, and polymers, AC shows better performance and stability in terms of adsorption (Regti, Laamari, Stiriba, and El Haddad, Citation2017). Recently, the pollutant gas adsorption by activated carbon has been recognized as a promising technology for the attraction mechanism between the pollutant and the surface functional groups (Le-Minh, Sivret, Shammay, and Stuetz, Citation2018). Production of AC from biomass can be seen as advantageous in two ways, firstly, it can prevent the production of CO2 by fixing the carbon and secondly, the AC can go into the soil naturally (Danish and Ahmad, Citation2018).
AC is carbonaceous material with an amorphous structure solid which has a high degree of porosity and well-developed surface area with numerous oxygenated functional groups such as carboxylic acids, phenols, carbonyls and lactones (Benedetti, Patuzzi, and Baratieri, Citation2018). The pores present on the AC surface are of significant importance and they exist in three forms: microspores, mesopores, and macropores (Jodeh, Abdelwahab, Jaradat, Warad, and Jodeh, Citation2016). Except for macropores that contribute the least contribution, the other pores contribute to the increase of the surface area and their presence on carbons is vital as they are the main source of driving the adsorption of gases and. In addition, functional groups also help promote adsorption capabilities (Elsayed et al., Citation2017). These distinctive characteristics make AC a versatile substance material that can be used not only as an adsorbent in water and gases but also as a catalyst or co-catalyst for the removal of pollutants from gases, liquids as well as the recovery of chemicals (Afif et al., Citation2019). Most studies have shown satisfactory results of ACs, specifically the effectiveness of removing organic compounds like dye, phenolic compounds, and inorganic compounds. They are also used in the field of the pharmaceutical industry to get rid of ingested toxins from the human body, recovery of gold, silver, and other metals (Vences-Alvarez et al., Citation2017). They are used as catalysts and co-catalyst in the gas mask filter company, food industries, chemical industries and automobile pollution control companies (Danish and Ahmad, Citation2018).
Usually, ACs are produced from finite resources such as coal, lignite, peat, petroleum residue materials that are expensive and required intensive regeneration (Yahya et al., Citation2018). It is reported that the demand for AC is estimated to increase by up to 2.1 million metric tons by the year 2018 (Maneerung et al., Citation2016). The high demand and the necessities have led to the discovery of cheaper, environmental-friendly and sustainable resources for the production of AC like biochar from thermal treatment of biomass. The primary sources of AC are agricultural waste, sewage, municipal waste, industrial waste, forestry residue, acacia species, Imperata cylindrica(Ahmed et al., Citation2018a, Ahmed, Parvaz, Johari, and Rafat, Citation2018b; Danish and Ahmad, Citation2018 ). Acacia species are known as the most invasive and they have adverse effects on other trees, and thus, threatens the biodiversity seriously (Hidayat, Abu Bakar, Yang, Phusunti, and Bridgwater, Citation2018; Islam, Mohamad, and Azad, Citation2019). A total of 1350 different types of acacia trees are known to exist in the world, which can grow in any climate (Ahmed, Hidayat, et al., Citation2018c; Radenahmad, Rahman, Morni, and Azad, Citation2018; Reza et al., Citation2019 ). Improvements in biofuel technology have developed the new technique to manage these invasive acacia trees to convert them into biochar (Alhinai, Azad, Bakar, and Phusunti, Citation2018) which can produce activated carbon.
For activated carbon, biochar is the primary source which can be produced from the thermochemical conversion of biomass like pyrolysis or gasification (Odetoye, Abu Bakar, and Titiloye, Citation2019; Radenahmad et al., Citation2020). Through slow pyrolysis, a higher amount of biochar can be produced from various types of precursors, typically biomass as a feedstock under a range of processes and operating conditions (Reza et al., Citation2020). Pyrolysis/gasification is the process to generate biochar, bio-oil, and biogas from biomass which has different properties than the parent biomass (Abu Bakar, Citation2013; Odetoye, Onifade, AbuBakar, and Titiloye, Citation2013). Bio-oil can be used as energy after refinement (Odetoye, Afolabi, Abu Bakar, and Titiloye, Citation2018). Biogas is the combination of different gases that can be used as fuel in a fuel cell (Abdalla et al., Citation2018; Afif et al., Citation2016; Afroze et al., Citation2020b, Citation2020a, Citation2019; Radenahmad et al., Citation2016). Finally, the solid residue is the biochar which can be converted into activated carbon (Morni et al., Citation2018). Using the same precursor, different types of ACs can be produced by activating agents under different operating conditions (Yusuff, Citation2019). Activated carbon can be produced by physical, chemical, physiochemical and microwave-assisted activation of biochar and biomass. Physical activation consists of heat and gas (steam, CO2, N2 or mixture), chemical activation is done by chemical agents (acid, base, metal oxide, alkaline metal), physiochemical activation involves heat and chemical and microwave-assisted activation is by microwave radiation (Ao et al., Citation2018). The activation depends on particle size, retention time, impregnation ratio, procedure configuration, activation period, properties of the precursor, and chemical substances. To further improve the features of AC, researchers have been performing research studies for producing much better AC via loading nanoparticles on the surface of this material which may be used in the removal of organic and inorganic compounds from aqueous solutions more efficiently (Lakshmi et al., Citation2018).
The present paper reviews the efficiency of different types of activation processes of activated carbon from biomass. The adsorption capacity of contaminants and pollutants from water and air has been described elaborately to get pure water with clean air. The recycling/regeneration and handling process of activated carbon is also illustrated.
2. Preparation of activated carbon
For the preparations of activated carbon, two basic steps are maintained. The first one is the carbonization and the second one is the activation. Carbonization is done through the pyrolysis/gasification at a higher temperature in an inert atmosphere to produce the biochar (Odetoye et al., Citation2019). In this stage, the carbon content of the carbonaceous substance was prepared by removing the volatile matter through thermal degradation (Radenahmad et al., Citation2020). The temperature, the heating rate, the nitrogen gas flow rate and the residence time are the significant parameters in this stage. As the achieved biochar reveals low adsorption ability, an activation process is essential to improve the pore volume, the pore diameter and the surface area (X. Yang et al., Citation2019). In the activation process, initially, the disorganized carbon was eliminated, exposed the lignin to the activating agents and developed the microporous structure. Finally, the existing pores are widened to a large size by burning of the walls between the pores. This raises the intermediate pores and macro-porosity which reduces the volume of micro-pores. Depending on the type of activation, activation can be a process prior to carbonization or subsequent to carbonization for the elimination of deposited tarry substances in biochar that can help to enhance the porosity and to provide high surface areas for the ACs (Ukanwa et al., Citation2019). The primary activation process by which the activated carbon was prepared from biomass has shown in .
Activation of carbon starts with the elimination of tarry substances to remove deposited tars that results in blockage of pores and to help facilitate the activating agent for reaction with biochar later (Yahya et al., Citation2015). Subsequently, carbon particles in biochar are burnt and then oxidized with an activating agent. For porous structure development, activation temperature and time become the two most important parameters to be taken into account. It is reported that these parameters correspond to the pore volume, but are inversely correlated with the yield of AC. The first step to prepare AC is the carbonization of biomass where it is necessary to control and limit the high temperature, as high-temperature activation could cause the formation of unwanted substances that can occur during thermal decomposition of the material (González-García, Citation2018).
2.1. Carbonization / pyrolysis
Carbonization or pyrolysis is the thermal decomposition of raw materials in a furnace under N2 purge in an inert atmosphere in order to remove volatilely, non-carbon species like nitrogen, oxygen, and hydrogen and to enhance fixed carbon content in order to produce biochar (Radenahmad et al., Citation2020). During the devolatilization process, narrow pore structures of precursors start to develop which leads to the deposition of tarry substances formed as the temperature rises (Alhinai et al., Citation2018). In some cases, this deposition may cause a collision of some tarry substances and the collapse of walls of pores that can result in hydrocracking and carbon deposition (Odetoye et al., Citation2018). has shown a summary of the carbonization process in four stages (Chowdhury et al., Citation2013).
Table 2. Four stages of the carbonization process.
The carbonization parameters significantly affect the process and highly influence the quality of final products, hence, a careful selection of parameters is of importance (Dhyani and Bhaskar, Citation2018). Carbonization temperature is of utmost importance as it has the most significant effect on the process, followed by a heating rate of reactions, amount of inert gas and its flow rate and lastly the carbonization residence or holding time, as shown in . Generally, when the temperature is increased, more volatile species are released along with the rise of fixed carbon content as well as ash content. However, this reduces the yield of biochar (González-García, Citation2018). The decrease in the yield is thought to be caused by the primary decomposition (de-volatilization) of biomass at high temperatures and the secondary decomposition (cracking) of biochar residue. Consequently, higher temperature produces a better quality biochar (Reza et al., Citation2020).
Figure 2. Thermo-chemical conversion of biomass into bio-oil, biochar, and gases (Mohan et al., Citation2014).
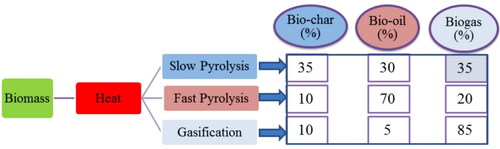
For instance, in a research study done by Wang et al. (Wang et al., Citation2010), the effects of carbonization temperature and carbonization holding on the performance and quality of AC has been investigated. When carbonization temperature was increased in the range of 300–600 °C, the adsorption capacity of AC increased from 756.42 mg/g to 933.84 mg/g but the yield of AC decreased from 41.23% to 32.79%. However, in a higher temperature range from 400 °C to 600 °C, adsorption capacity dramatically decreased from 933.84 mg/g to 538.36 mg/g. When the temperature was kept constant at 500 °C for two different holding times (1 h and 2 h), the adsorption capacities and yields were reported to be 756.04 mg/g and 37.63%, 887.35 mg/g and 38.12%, respectively.
2.2. Physical activation
Physical activation can be either a single-step or a dual-step process (Ao et al., Citation2018). Typically, physical activation is a dual step process, where carbonization process of dried samples happens at 400-700 °C to form biochar which is followed by activation using oxidizing gas like steam, air, CO2 or their mixtures (Menya, Olupot, Storz, Lubwama, & Kiros, Citation2018) at a high temperature of about 800-1100 °C for some burn off (Rashidi and Yusup, Citation2017). The biochar obtained from carbonization typically has a surface area of less than 300 m2/g (Rashidi and Yusup, Citation2017). This low surface area and adsorption capacity are ascribed to the pores locked by tarry substances, which can be removed by activation(Yahya et al., Citation2015). As a single-step process, carbonization and activation occur simultaneously where the temperature is kept in the range of 600-800 °C (Lee et al., Citation2018). Similar to the dual-stage process, in the single-stage process, the dried sample is carbonized until a specified temperature, but biochar can be further heated for a desired duration just by switching inert gas to oxidizing gas. Despite having similar procedures, the single-step process is much more feasible as it skips the cooling phase after the carbonization which can reduce the physical effort, electrical consumption, cost and operating time (Rashidi and Yusup, Citation2017).
During the physical activation, apart from widening the narrow pores developed on the surface of biochar, new pores are produced in the meantime, thereby the porosity and surface area of the carbonaceous porous structure can be enhanced (González-García, Citation2018). However, physical activation tends to give a relatively lower carbon yield and quality of AC than chemical activation could provide due to higher activation temperature and extended activation time spent in the process (Ao et al., Citation2018).
In physical activation, steam and CO2 are commonly used as activating agents as shown in . Air (oxygen) is less preferable as an agent for activation due to the exothermic nature of the reactions which can cause excessive burnings, both on the internal and external surfaces of the biochar which can result in reduced yield (Chowdhury et al., Citation2013) As can be seen in , steam and CO2 appear to give similar, maximum BET surface areas, both are able to react with carbon to provide maximum surface areas that can reach 1000 m2/g and even higher values. Although the procedures are similar, their mechanism and reaction are slightly different. Rafsanjani et al. (Rafsanjani et al., Citation2013) claimed that due to the smaller size of H2O than CO2, water molecules are able to diffuse into pores of biochar more easily and consequently, gives a faster reaction than that of carbon with CO2 and thus, develop new pores (Alvarez et al., Citation2015). At a near activation temperature, steam is reported to react four times faster with carbon than CO2 (Rashidi and Yusup, Citation2017). Hence, through steam activation, AC is able to reach a high surface area in a short period (Wong, Ngadi, Inuwa, & Hassan, Citation2018). CO2 activation prioritizes the development of new pores over the widening of narrow pores while steam activation favors the widening of micro-pores towards developing mesopores and macropores from the beginning of the activation process at a standard heating rate. This ensures a broader pore distribution on the resulting ACs (González-García, Citation2018). Even at a higher activation rate, it would result in higher percentage mesoporous and micro-porous structures for steam activation and CO2 activation respectively (Rashidi and Yusup, Citation2017). In some cases, CO2 activation appears to be a preferred choice since it is cleaner, easy to handle the gas and has control of the activation process even at high temperatures up to 800 °C because of its slow reaction rate (El-Naggar et al., Citation2019).
Table 3. Physical activation of precursors for the production of ACs.
2.3. Chemical activation
Chemical activation can be a single-stage or a dual-stage process. Both processes are similar, except for the carbonization process (González-García, Citation2018). In the single-stage process, carbonization does not exist and in the main step, the dried sample is activated by interacting with dehydrating agents like NaOH, KOH, ZnCl2, and H3PO4 (Yahya et al., Citation2018). In the dual-stage process, the dried sample is carbonized to form biochar in the temperature range of 400 – 600 °C, before chemical activation (Ao et al., Citation2018). A compilation of chemical activation data is summarised in . Chemical activations can be applied in three forms: (i) Basic activation, (ii) Acidic activation, and (iii) Neutral activation (Din et al., Citation2017).
Table 4. Chemical activations of precursors for the production of ACs.
The data summarised in denote that carbonization and activation conditions, choice of activators, and impregnation ratio can affect the textural properties and also the quality of the AC (Yahya et al., Citation2015). Depending on the type of dehydrating agents used, different precursors react differently to give a variety of surface areas, pore volumes, and yield (Mu’azu et al., Citation2017). In general, the basic activation of biomass with metal alkaline hydroxides such as KOH and NaOH produces ACs of very high surface areas that can reach up to 2000 m2/g (). However, the utilization of metal alkaline hydroxides is limited by the type of precursors. A study by Cao et al. (Cao et al., Citation2006) reported that metal alkali hydroxides are usually applied in the dual-stage process as they work more efficiently with biochar. Char formed by carbonization of precursors contains a certain number of pores that are feasible for the diffusion of dehydrating agents and react with carbons and ACs of high surface areas and high porosity are produced eventually (González-García, Citation2018). Metal alkali hydroxides might have difficulties penetrating into precursors as the porosity of raw materials is not prominent (Chowdhury et al., Citation2013). Impregnation alone is insufficient to produce a porous active carbon and hence would require a dual-stage chemical activation. For instance, in a research work done by Isoda et al. (Isoda et al., Citation2014) on rice husk using NaOH, the surface areas are activated by interacting with are reported as 280 m2/g and 660 m2/g, respectively. It is also reported that biochars are relatively more susceptible to KOH activation that could help produce ACs of high-quality surface properties (Zaini and Kamaruddin, Citation2013).
Among all the activators shown in , it is noticed that the most commonly used activator is H3PO4. This could be due to the essential activation being less economically favorable than acidic activation because of its higher cost. Acidic activation using H3PO4 as a more economical method can result in a higher percentage of yields ( Din et al., Citation2017) alters the total number of micropores and mesopores leading to significant changes in the surface area, porosity, and reactivity of the end product (Caglar et al., Citation2013) In another study performed by Arami-Niya et al. (Citation2010, Citation2011) the effects on the textural properties of the acidic activation and neutral activation of oil palm shell using H3PO4 and ZnCl2. Both the surface area and pore volume obtained with acidic activation are reported to be higher than those obtained with the neutral activation. It was justified that the differences in the textural properties are due to the rate of activation, which is higher for H3PO4 with an 8.86% burn-off per hour while it is lower for ZnCl2 with a 22% burn-off per hour (Arami-Niya et al., Citation2011). Nevertheless, from the environmental point of view, metal alkali hydroxides and acids are less preferable as activators since they are corrosive, toxic, hazardous and have high chances of causing an explosion where precaution measures are required (Din et al., Citation2017). Therefore, more reliable dehydrating agents such as K2CO3 is used alternatively in single-stage activation to produce activated carbons and to ease the problem of basic and acidic chemicals contributing to secondary waste disposal (Chowdhury et al., Citation2013; Rashidi and Yusup, Citation2017). As compared to physical activation, chemical activation is a preferable choice for activation in terms of the development of the surface area, micro-pores, higher carbon yield and it is economically viable due to lower activation temperature and shorter activation time (Din et al., Citation2017). However, its drawback is to have chemical agents remaining on char, and a further washing stage is required to be removed entirely (Chowdhury et al., Citation2013) or followed by physical activation of char (physiochemical activation) (Rashidi and Yusup, Citation2017).
2.4. Physiochemical activation
Apart from physical activation and chemical activation, activation can be carried out simultaneously through physiochemical activation (Chowdhury et al., Citation2013). Two methods can be carried out in producing ACs with physiochemical activation: (i) chemical treatment prior to carbonization (pre-carbonization) and (ii) chemical treatment subsequent to carbonization (post-carbonization) (Rashidi and Yusup, Citation2017). The first method has the precursors undergoing carbonization which is followed by impregnation of biochar, and then thermal treatment in the presence of oxidizing gas or carbonization in an inert environment and switching to oxidizing gas for physical activation at an elevated temperature in the range 600-850 °C (Rashidi and Yusup, Citation2017), while the second method has precursors undergoing chemical treatment before thermal treatment and physical activation (Mohd Din et al., Citation2009). Lee et al. (Citation2014) mentioned that the sequence of chemical activation in this process does affect the quality and textural properties of the obtained ACs.
In the studies given in the pre-carbonized sample and post-carbonized char for chemicals, activation was compared in several aspects. These findings revealed that a chemically treated pre-carbonized sample shows the surface area of 990 m2/g with the pore volume of 0.42 cm3/g while chemically treated post-carbonized char shows a lower surface area of 680 m2/g with a pore volume of 0.30 cm3/g. The lower surface area of the post-carbonized sample is explained by the pore-blocking effect of the dehydrating agent (Min, Abbas, Kanthasamy, Abdul Aziz, & Tay, Citation2017). Accordingly, physical activation could be carried to clear the blockage. In addition, (Chowdhury et al., Citation2013) have explained that the application of physiochemical activation could be carried out when dehydrating agent remains on the surface of biochar after washing the stage that results in the blockage of pores. Hence, additional physical activation step is necessary to enhance the porosity of biochar (Danish, Hashim, Ibrahim, Rafatullah, & Sulaiman, Citation2012).
Table 5. Physiochemical activation conditions of precursors for the production of ACs.
Several studies comparing physiochemical and chemical activation processes report that double-step activation produces ACs with different yet better textural properties and quality. Arami-Niya et al. (Arami-Niya et al., Citation2012) claimed that ACs produced through physiochemical activation, have well-developed and evenly distributed pore structures. They report in another study that the surface areas changed in the range of 1035-1653 m2/g and 554-1213 m2/g for H3PO4 and CO2 activation and sole CO2 activation respectively, even for only CO2 activation that has 2 h of longer optimum holding time (7 h) than the double-step activation (5 h) (Arami-Niya et al., Citation2011). Moreover, the surface area of AC obtained through sole CO2 activation also was reported being 632 m2/g with the pore volume of 0.26 cm3 /g (Lee et al., Citation2014). Tseng et al. (Tseng et al., Citation2006) also mentioned in their study that when carbons are impregnated by KOH, only micro-pore structures are formed on the surface. Meanwhile when impregnated carbon is subjected to heat treatment with CO2 gas for physiochemical activation, the ratio of macropores to mesopores increases which explains the increase in porosity and in mass transfer for better adsorption capacity (Danish and Ahmad, Citation2018).
2.5. Microwave-assisted activation
Microwave (MW) activation has turned out to be a realistic substitute to conservative approaches in producing activated carbon, due to its exceptional features such as selectively, quickly, even and volumetric heating, indirect interaction between the heating foundation and heated resources, and prompt and precise regulator (Hoseinzadeh Hesas et al., Citation2013). The major working constraints for the microwave supported activation are procedure configuration, MW radiation strength, activation period, properties of precursors, interactions between MW and chemical substances (Ao et al., Citation2018). In MW heating, the energy is easily distorted in to heat inside the molecules by dipole orientation and ionic transmission. The particles with induced dipole moment or permanent dipole moment are allied in the opposing course from the applied force when given a high-frequency voltage (Alslaibi, Abustan, Ahmad, & Foul, Citation2013). Therefore, a large temperature gradient progresses from the inner of the example molecule to its cool external, which makes it more operative and efficient (Pathak and Mandavgane, Citation2015). MW assisted activation is the combination of physical and/or chemical activation which can produce higher quality activated carbon by one-step (Deng et al., Citation2010) or two-step (Abbas and Ahmed, Citation2016) activation processes as shown in .
Figure 3. Schematic diagram of AC preparation from biomass by Microwave (MW) (Ao et al., Citation2018).
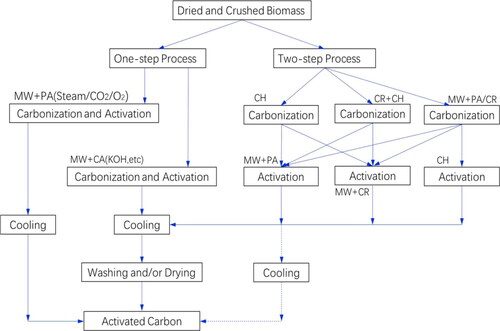
Carbonization and activation are the two major procedures for one-step microwave activation with a reactor (Li et al., Citation2016). The benefit of this activation is seen to get the AC with stable arrangement by a simple procedure. Two-step microwave activation involves the carbonization and activation of biochar under microwave radiation (Li et al., Citation2008). The BET surface area in this process depends on the biomass sources, the temperature of the pyrolysis, power of radiation, running time and the additives (Ao et al., Citation2018). Microwave activation is a versatile procedure for exceptionally manageable factors, and for producing ACs with a better pore distribution and textural features (Angın et al., Citation2013).
3. Applications of activated carbon
As a consequence of the limited supply of water, AC has been increasingly used in water purification for the removal of pollutants and contaminants (Wong et al., Citation2018). It is reported that around 80% of the ACs around the world is being applied in liquid-phase or aqueous solution (Rivera-Utrilla et al., Citation2013). ACs have been proven to be one of the most critical and effective adsorbents for organic and inorganic pollutants. The versatility of adsorption capacity, surface area and porous structure of ACs are the main grounds for its application (Briones et al., Citation2011). When carbons come in contact with an aqueous solution, the surfaces functional group of ACs dissociate or attract the ions in solution hence generating electric charges. The dissociation or strength of attraction depends on the pH of the solution and surface characteristics of adsorbents (González-García, Citation2018).
The use of green synthesis nano-composites to remove of pollutants and contaminants from water, with the technology of loading of nanoparticles on ACs for the enhancement of adsorption efficiencies and uptake capacities has been acknowledged by many researchers recently (Fu et al., Citation2015; Jamshidi et al., 2016). In this context, tungsten oxide nanoparticles (Anfar et al., Citation2018), Zero-valent iron nanoparticles (Khosravi et al., Citation2018), iron oxide nanoparticles (Sahu et al., Citation2017) and Ag nanoparticles (Tuan et al., Citation2011) were loaded onto ACs, which are mainly used in the applications of dye and metallic pollutants removal. The pH of the solution is one of the key parameters determining both the surface charge density and cationic or anionic nature of the surface. Thus, the solution with low pH will attract anions and at high pH values, it will attract cations. Therefore, the uptake of the charged inorganic groups can be controlled by arranging the pH of the solution. The acidic carbons will be more suitable for retaining cations, and basic carbons become effective towards anion removal (Rivera-Utrilla et al., Citation2013).
3.1. Inorganic pollutants from water
3.1.1. Metallic pollutant removal
Metallic pollutants such as heavy metals and metalloids are hazardous to water contaminants subjected to biomagnification in the food chains and non-degradable. Their existence is detrimental and can accumulate in the human body or living organisms causing long-term health effects and disease (Burakov et al., Citation2018). Moreover, they have small and complex structures that are of significant concern as that are difficult to be removed. These contaminants are biomagnification in the food chain that can accumulate in the body, causing long-term health effects. The main sources of these metals come from agricultural and industrial activities such as mining, usage of fertilizers, electroplating, and car manufacturing (Azimi et al., Citation2017). Many studies have reported the use of ACs for the low-cost and easy removal of the metallic pollutants (Burakov et al., Citation2018). Therefore ACs are widely used in purifying water containing metals such as cadmium, lead (II), copper, iron, and chromium (VI) (Yusuff, Citation2019). The removal of the metallic pollutant from water is a direct process because of its small size and being charged in solution repeatedly which creates the major communications with AC in the adsorption process of electrostatic behavior (X. Yang et al., Citation2019). The factors that affect the adsorption process on activated carbon are, the interaction of the metal ion and its structure, the pH of the solution and the point of zero charge, the porosity and surface area, the surface oxygen functionality and the size ions (typically 1.0–1.8 nm), with substantial volumes of tapered microporosity (Bian et al., Citation2018). The interactions between the adsorbent and adsorbate are dependent on the electrostatic forces, non-electrostatic forces and dispersive forces between them and these forces are being played an important role in the adsorption process (X. Yang et al., Citation2019).
summarizes the data obtained on different types of adsorbents used for the removal of metallic pollutants with referring to their adsorption capacities and experimental conditions. The removal efficiency of chromium with Acacia mangium AC was found as 37.16 mg/g whereas with Acacia nilotica Bark AC it was found as 93.1% (Danish et al., Citation2012; Rani, Gupta, & Yadav, Citation2006). Lead is one of the most important materials widely used in industry for the manufacturing of pipes, in accumulators and as additives in fuels and paints (Afroze et al., Citation2019). As lead is used in piping, there have been reports of high concentrations of lead up to 2 mg/l being released to the water in household pipes. It is reported that lead has the tendency to accumulate in living organisms and shows toxicity symptoms in the human body (Chowdhury et al., Citation2013) Hence, in this case, this hazardous pollutant that should be removed from water should be analyzed. The solution used for the adsorption of lead has an average of pH 5 except for Tamarind wood AC in solution pH 10. The highest surface area of AC is reported to be 1322 m2/g with Tamarind wood AC produced from ZnCl2 activation (González-García, Citation2018) and the highest adsorption capacity obtained is 114.8 mg/g with Tropical almond shells AC synthesized from the mixture of CO2 and steam activation (Largitte, Brudey, Tant, Dumesnil, & Lodewyckx, Citation2016). Meanwhile, lead is reported to be obliterated (100%) with watermelon peel AC and Oil palm shell AC follows closely for a 99.8% removal efficiency (Min et al., Citation2017).
Table 6. Applications of ACs for removal of metallic pollutants.
Cadmium is another threat to highly toxic water (Wan Ibrahim et al., Citation2019). Its existence is detrimental to living organisms and aquatic ecosystems even at a deficient concentration hence is only permitted to have a concentration of 5-10 ppm in water (Wang et al., Citation2010). Cadmium contaminants mainly come from battery production or inappropriate disposal of battery, from cadmium plating and the use of cadmium-rich fertilizers. Waste effluents from these industries pollute the water resource and environment (Burakov et al., Citation2018). Oil palm shell AC was used for the removal of cadmium with a high removal efficiency of 99.5% (Min et al., Citation2017). Chemically activated date pits ACs were able to remove cadmium with adsorption capacities in the range of 118.1 mg/g to 127.0 mg/g, at around pH 6 at temperature 25-30 °C (Ahmed, Citation2016a). Mercury generally has a deficient concentration in water with a weight of 1 g/l (Attari et al., Citation2017). Similar to other lead and cadmium, it occurs in aqueous waste from industries. NH4NO3 activated Pistachio wood wastes AC has a high surface area of 1448 m2/g that is able to adsorb mercury at a high capacity of 201.095 mg/g at pH 6 (Sajjadi et al., 2018), as well as for steam activation B. vulgaris striata AC with a high adsorption capacity of 248.05 mg/g in a much more acidic solution of pH 3 (González-García, Citation2018).
Physically activated Fe-supported walnut shell AC (Derdour, Bouchelta, Khorief Naser-Eddine, Medjram, & Magri, Citation2018) and Ceiba pentandra hulls AC (González-García, Citation2018) prepared for the removal of chromium appeared to have very low adsorption capacities that are < 30 mg/g meanwhile chemically activated date pits AC (Ahmed, Citation2016a) and chestnut oak shells AC (Niazi, Lashanizadegan, & Sharififard, Citation2018) have higher adsorption capacities of 120.5 mg/g and 85.47 mg/g. This shows that for the removal of chromium, chemically activation is preferable to optimize the adsorption process. Likewise, physically activated date pits AC can hardly remove iron, copper, and aluminum as shown from their low adsorption capacities (Ahmed, Citation2016a). It is only with chemically activated watermelon peel AC that iron and copper can be removed almost entirely at an efficiency of 91% and 99% respectively (Min et al., Citation2017).
Khosravi et al. (Citation2018) compared the results of the experiment between GnZVI/PAC, PAC, and ACs from other precursors. Despite a lower surface area than PAC, maximum adsorption of GnZVI/AC obtained is 53.48 mg/g considered to be a better adsorbent than PAC (18.18 mg/g), as well as other adsorbents which are only able to remove < 50 mg/g of chromium from aqueous solution. They concluded the reasons for this result were due to the loading of zero-valent iron particles that have caused the alteration of functional groups and hence the increase in feasibility between adsorbent and pollutants. Therefore, nanoparticles have a significant contribution to the adsorption of chromium from the solution. A similar trend is seen in another study where Fe3O4/CSAC is used as an adsorbent has proven to be superior to CSAC alone for the removal of As (V) and As (III) (Sahu et al., Citation2017).
3.1.2. Non-metallic pollutant removal
Although anions can be beneficial and are part of the essentials in human bodies, the excessive presence of these anions such as fluoride, Molybdate, and phosphate can be a threat and are detrimental to living organisms. Millions of people have suffered from fluorosis due to the high concentrations of fluoride in potable water, especially in developing countries (Alagumuthu and Rajan, Citation2010). For instance, Viswanathan et al. (Citation2009) accessed the fluoride ions in water and it was found to be excessive (3.24 ppm) and in their report, they explained the positive correlation of high fluoride level in the water to fluorosis in children. Likewise, molybdate in its anion form is readily absorbed by grazing livestock and appears in the food chain and water that can be harmful to grazers and carcinogenic to human bodies (Namasivayam and Sangeetha, Citation2006). Hence, the concentration of anions should be examined regularly and strictly controlled to ensure a permissible amount of concentration in the water.
In , three different research studies, Namasivayam and Sangeetha (Citation2006, Citation2005, Citation2004) examined the use of agriculture waste coir pith with ZnCl2 activation for the removal of anion pollutants in water like thiocyanate, phosphate, and molybdate. Maximum adsorption capacities are in the range of 16-18 mg/g at an optimum pH of 3.2 and in the range of 4-8 respectively for removal of thiocyanate and molybdate while for phosphate is relatively lower with a value of 5.1 mg/g at pH in the range of 3 - 10. They concluded that despite being a low-cost adsorbent, coir pith can be a good precursor use to produce ACs for removal of anion pollutants and that chemisorption and ion exchange on the surface of ACs have effects on the adsorption capabilities. Walnut shell AC has also proven to be a suitable adsorbent with a high surface area and adsorption capacity of 484.26 m2/g that is able to remove 52.67 mg/g of sulfur from aqueous solution. Alagumuthu and Rajan (Alagumuthu and Rajan, Citation2010) compared zirconium impregnated cashew nutshell AC and cashew nut shell AC that were both chemically activated with H2SO4. Zirconium impregnated Cashew nutshell AC (ZICNSC) shows a better removal efficiency of 80.33% than sole cashew nutshell AC (72.67%), proving the potential of a modified AC for removal of fluoride ions.
Table 7. Applications of ACs for removal of non-metallic pollutants.
3.2. Organic pollutants from water
3.2.1. Color/dye removal
Dyes have extensive usage and application in industries such as paints, cosmetics, textiles, and paper products. From an environmental point of view, the textile industry is considered to be the primary source of water pollution (Oladipo and Ifebajo, Citation2018). Among 3600 kinds of dyes used in the industry (Bernhardt and Gysi, Citation2016), about 2-20% of dyes are lost in the effluent discharge for every production (Ahmed et al., Citation2012). Hence, dyes constitute one of the largest and heaviest pollutants in wastewater (Oladipo et al., Citation2017). Effluents discharged from these industries contain dyes that can pollute the river and other water resources as they are toxic and carcinogenic (Ahmed, Citation2016a). Their presence in water can reduce photosynthesis of aquatic flora and fauna which leads to the destruction of the marine ecosystem. Moreover, the ingestion of dyes can be detrimental as it causes mutations to the human body (Wong et al., Citation2018).
Activated carbon has great potentiality for the removal of dyes from water solution. The removal capability depends on the surface area, pore size, functional groups of AC and the polarity, solubility and molecular size of the dyes and the pH, other ions in solution (Sharma et al., Citation2011). ACs were achieved by the conservative steam-activation technique, and the pre-treatment process has the adsorption characteristics of organic compounds (dye) from the aqueous solution which has abundant mesopores and high adsorption capacity especially for big molecules (Katheresan et al., Citation2018). is a compilation of data consisting of the different types of adsorbents use for the removal of dyes with their adsorption capacities and conditions. Activated carbon from Acacia mangium and Acacia nilotica have proven their capability for the removal of different dyes. These AC can eliminate the toxic textile Methyl orange dye 90.5% (Danish, Hashim, Mohamad Ibrahim, & Sulaiman, Citation2014) and Methylene Blue by 250 mg/g (Dass and Jha, Citation2015).
Table 8. Applications of ACs for removal of color/dye pollutants.
It can be seen from the table above that Methylene Blue is the most common dye to be removed from wastewater. Most of the adsorption capacities are reported to be more than 100 mg/g. Among all the ACs used for the adsorption of methylene blue, the highest adsorption is obtained from KOH activated distillers grains AC, which has a high surface of 1430 m2/g that is capable to adsorb 934.579 mg/g of methylene blue from aqueous solution. The adsorption was performed at 55 °C at pH 5.8 (Wang et al., Citation2018). Ramulus mori and rice straw were activated with N2H9PO4 to produce AC for adsorption of methylene blue. Their surface areas were 1061 m2/g and 1154 m2/g with adsorption capacities of 314.1 mg/g and 129.5 mg/g respectively (Ahmed, Citation2016b).
Date pits have been widely used for the production of AC with both physical and chemical activations for the dye removal including methylene blue, methyl orange, remazol yellow and maxilon blue. Date pits AC that is produced from activation with ZnCl2 worked best with adsorption of methyl orange and the maximum adsorption observed was 434.8 mg/g, followed by KOH activated dates pits AC for removal of methylene blue that adsorbed 316.1 mg/g of dye from aqueous solution (Ahmed, Citation2016a). AC produced from bamboo cane powder has proven its capability for the removal of lanaysn orange, with a very high adsorption capacity of 2600 mg/g from aqueous solution (Wong et al., Citation2018). ACs produced from B. aethiopum flower and rambutan peels with chemical activation were used for the adsorption of malachite green. The results showed a significant difference in adsorption capacities where B. aethiopum flower AC adsorbed48.23 mg/g of dye while rambutan peel adsorbed 418.6 mg/g of dye from aqueous solution (González-García, Citation2018).
Tuan et al. (Citation2011) and Anfar et al. (Citation2018) examined the use of green nanocomposites from the synthesis of coconut husk and almond shell with Ag nanoparticles and WO3 nanoparticles respectively for the removal of dye (methylene blue and rhodamine B) from aqueous solution. The adsorption capacity of Rhodamine B with WO3/AC shows a high value of 1666.67 mg/g while methylene Blue removal with AgAC shows a relatively low value of 240 mg/g. Anfar et al. concluded that the high value is due to the loading of WO3 nanoparticles that have affected the adsorption mechanisms for better adsorption. This can be compared with okra waste AC that only has an adsorption capacity of 321.5 mg/g (Wong et al., Citation2018). On the contrary, Tuan et al. explained the low value of AgAC adsorption could be due to nanoparticles having only a slight influence on the adsorption capability of AC. They concluded that nanoparticles had no effect on size, structure and adsorption capability of AC. From the data compiled in , it can be concluded that chemical activation and neutral activation produced ACs work better for the removal of dye than ACs produced from physical activation. The findings also concluded the importance of surface areas and the functional groups of ACs for their interactions with the adsorbates. Additionally, it is not on all occasions that nanoparticles could help in improving the adsorption capacity.
3.2.2. Phenolic compounds removal
Effluents containing phenolic compounds mainly come from chemical and petrochemical industries (Hammani et al., Citation2017). These pollutants are highly toxic, carcinogenic and are being considered as a serious threat to the aquatic ecosystem and the living organisms (Ahmed and Theydan, Citation2012). Therefore, environmental and health protection agencies have strictly limited the concentration of phenolic compounds in water bodies and these pollutants are given priority to remove (Chowdhury et al., Citation2013). Environmental Protection Agencies (EPA) has limited the concentration of these pollutants by the maximum of 0.1 mg/L in industrial effluents while World Health Organisation (WHO) has set a maximum permissible concentration of 0.001 mg/L for phenols in drinking water (Chowdhury et al., Citation2013). For the phenolic compound adsorption by activated carbon, surface chemistry is more significant than porosity because the surface functionality rises considerably comparative to the pore size arrangement. The carboxyl and hydroxyl groups of the AC reserved the adsorption of phenol which increased the attraction of the AC to water directed by π–π bond between the surface of the AC and the adsorbent (González-García, Citation2018). The elimination of phenol and the phenolic compound by AC has maintained the donor-acceptor mechanism where the surface oxygen groups are the electron donor and the aromatic rings of the solute are the acceptor (Mu’azu et al., Citation2017). The pH of the solution affects the phenol adsorption where the adsorption quantity decreases for both high and low values of the pH. The phenols have high pH which is mostly anionic and do the repulsion between the carbon surface and the anionic phenol result in less adsorption. The lower pH values in acidic solutions create protons, which striven with carbonyl groups results in decreased adsorption (El Gamal et al., Citation2018). has shown a list of the data including the different types of adsorbents used for the removal of phenolic compounds with their adsorption capacities and conditions.
Table 9. Applications of the ACs in phenolic compounds removal.
Zbair et al. (Citation2018) studied the adsorption of Bisphenol A using argan nutshell AC. The maximum adsorption capacity is reported to be 1250 mg/g at 293 K. The nutshell AC showed a better performance in adsorption in comparison with the commercial ACs. Another precursor used for this adsorption is durian peel. However, the adsorption efficiency observed with durian peel AC is very low (4.2 mg/g) with a removal efficiency of 69%, although the adsorption process lasted for a day (Min et al., Citation2017). Lignocellulosic biomass such as date pits, avocado kernel, kernaf rapeseed, baobab wood, and Acacia seeds have been used to obtain AC for the adsorption of phenols. Among these ACs, the best adsorbent was reported being the H3PO4 activated baobab wood AC. Maximum adsorption was obtained in 24 h, at an optimal pH of three at 50 °C. Bansal et al. (Bansal et al., Citation2002) mentioned in their study that acidic surface functional groups on ACs could suppress the adsorption efficiencies of AC, while non-acidic or basic functional groups enhance the adsorption. This analysis is supported by another study, where date pits AC were chemically activated with both H3PO4 and KOH for use in the removal of phenolic compounds (o-nitrophenol and p-cresol). Basic-treated AC promoted better adsorption of phenolic compounds with 322.5 mg/g whereas acidic-treated AC was only able to remove 142.9 mg/g from aqueous solution (Ahmed, Citation2016a).
3.2.3. Pesticides removal
Pesticides are essential in modern agriculture, but the intensive use of pesticides can deteriorate the water quality. Bentazon, carbofuran and 2,4-dichloro-phenoxyacetic acid are the commonly used pesticides in agriculture and gardening. Due to their mutagenicity and carcinogenicity, they are considered as threats to the aquatic environment and human health, and their maximum concentrations in water are set at certain levels. The permissible concentrations in tap water and potable water are 0.05 mg/L, 0.09 mg/L and 0.1 mg/L for bentazon, carbofuran and 2,4-dichloro-phenoxyacetic acid, respectively (Salman and Hussein, Citation2014). Based on these results, adsorption as one of the most effective separation techniques being used for the removal of pesticides from water resources (Ahmed, Citation2016a). The adsorption of pesticides by the activated carbon depends on the fraction of organic matter and the continuous flow rate in the adsorption process as well as the carbon doses (Sharma et al., Citation2011).
Date stones were widely used as precursors for adsorption of pesticides as summarised in . El Bakouri, Usero, J., Morillo, J., Rojas, R., and Ouassini (2009) studied the adsorption of date stones AC on different types of drin pesticides namely aldrin, dieldrin and endrin from aqueous solution. Maximum adsorption capacities were obtained as 228.047, 295.305 and 373.228 mg/g for endrin, dieldrin, and aldrin, respectively, showing an increase from endrin to aldrin. It is reported that in this experiment, aldrin has the lowest solubility and therefore, it can be concluded that the increase of adsorption was due to the decrease in solubility of pesticides in water. Salman et al. (Citation2011) examined the adsorption capacities of physiochemical activated date seeds ACs. Date seeds were physiochemically treated with KOH and CO2 and the results obtained were 86.26 mg/g and 137.04 mg/g for adsorption of bentazon and carbofuran, respectively under the same conditions. They explained the difference in the adsorption capacities by the molecular size of smaller carbofuran than bentazon which leads to the easy diffusion and attachment onto the AC surface. The same experiment was repeated in another study and similar results were reported: 78.13 mg/g for bentazon and 175.4 mg/g for carbofuran. In addition, they included an experiment on adsorption of 2,4-dichloro-phenoxy acetic acid. It was shown in this study that AC was able to remove 175.4 mg/g of pesticides from aqueous solution (Salman and Hussein, Citation2014). Olive kernel, corn cob, soya stalks, and rapeseed stalks were physically activated to produce ACs for the removal of bromopropylate. This study reported the removal efficiencies of 90-100% for all the precursors used (Ioannidou, Zabaniotou, Stavropoulos, Islam, & Albanis, Citation2010).
Table 10. Applications of ACs for removal of pesticides.
3.2.4. Pharmaceuticals compounds removal
Pharmaceutical compounds constitute one of the emerging pollutants in the environment widely used in human, agriculture and aquaculture practices. Throughout the years, the increasing use of pharmaceuticals has led to continuous discharge to the aquatic ecosystem. Due to their high stability and hydrophilicity in water bodies, they can be persistent in water and therefore pose a threat to the environment. Although the concentration of pharmaceuticals is considerably low in the water, generally at a trace level, they can still induce adverse effects in the environment (Ahmed, Citation2017; Boudrahem et al., Citation2017; Mansour, Al-Hindi, Yahfoufi, Ayoub, & Ahmad, Citation2018). The elimination of pharmaceuticals compounds by AC depends on the bridging procedure, operational situations, the type of adsorbent, the type of precursor, and the activation technique and the properties of the adsorbate (pharmaceutical). Moreover, the operational technique such as adsorbent dose, adsorbate properties, operating temperature, solution pH, ionic capability and organic structure plays an important role in the adsorption of activated carbon (Chayid and Ahmed, Citation2015). Most of the scientists have proven that adsorption of the pharmaceuticals compound by AC has governed the Langmuir and Freundlich isotherm models (Mansour et al., Citation2018). Kinetic modeling was being used to measure the controlling rate of the adsorption process in the carbon surface (Tan and Hameed, Citation2017).
In , Ahmed et al. (2017) compiled the data obtained from a number of studied using different types of precursors for the removal of ibuprofen from aqueous solution. Olive stones, cork powder, mung bean husk, and mugwort leaves were physically and chemically activated to prepare ACs. Among these materials, steam activated cork powder reported to give the best result which was able to remove 378.1 mg/g of ibuprofen from water. This result can be compared with K2CO3 treated cork powder and indeed steam activated cork powder proved to be superior. CO2 activated olive stones also showed a relatively better result than the rest of the ACs, with the adsorption capacity of 282.6 mg/g while the other adsorbents reached the adsorption capacities < 200 mg/g. Waste apricot AC and olive waste AC can also be compared for the removal of naproxen. ZnCl2 treated waste apricot AC removed 106.4 mg/g of the pharmaceutical in 1 h while H3PO4 treated olive waste AC was only able to remove 39.5 mg/g after an adsorption period of 26 h. Olive waste was also compared for the adsorption of naproxen and ketoprofen. Using the same precursor (Olive wastes) of the same treatment (phosphoric acid), ACs were compared considering their adsorption capacities. The results showed that olive waste AC acted as a better adsorbent towards naproxen (39.5 mg/g) than for ketoprofen (24.7 mg/g). Clearly, olive waste AC was not a good adsorbent for pharmaceutical compounds as they did not give satisfying results. On the other hand, primary paper mill sludge AC proved to be a suitable adsorbent with a high adsorption capacity of 405 mg/g towards paroxetine (Wong et al., Citation2018) and exhibited high removal efficiencies by 75, 85 and 84% for SMX, CBZ, and PAR, respectively (Jaria et al., Citation2019). H3PO4 activated waste textiles (Cotton) AC can reduce the amounts of clofibricacid (AC), tetracycline (TC) and paracetamol (PC) by 83, 97.03 and 84%, respectively (Boudrahem et al., Citation2017). In another study, Lagenaria Vulgaris shell AC with 665 m2/g surface area showed very effective adsorption for ranitidine within the range of 93-99% whereas the removal of levofloxacin by date stones AC varied in the range 41.40-97.01 % with 100.4 mg in one-gram and cyclamen persicum AC which has the surface area of 799-880 m2/g exhibited favorable adsorption towards diclofenac (22.22%) (Mansour et al., Citation2018).
Table 13. Applications of ACs for gas adsorptions.
3.3. Other pollutants from aqueous solution
It has been shown in that activated carbon can be used for the removal of different pollutants like aniline, caffeine, suspended solids, etc. Aniline, a significant compound in the petrochemical industry is used for the synthesis of isocyanate. It is also used for the production of accelerators and antioxidants in the rubber industry, herbicides and pharmaceuticals industry (Kecira, Benturki, Daoud, & Benturki, Citation2018). Caffeine is an alkaloid used as a cardiac, cerebral and respiratory stimulant and is found in teas, coffees, soft drinks, chocolate and some kinds of candies. Its average consumption per person is around 200 mg per day and if ingested it is metabolized by the human body with about 1–10% is excreted by urine (Beltrame et al., Citation2018). For aniline removal by apricot stones AC the ZnCl2 shows better results than H3PO4 and the values are 147 mg/g and 115 mg/g with the corresponding surface areas of 1111 and 1382 m2/g, respectively (Kecira et al., Citation2018). Durian shell AC could remove toluene by 874 mg/g whereas orange peel AC could remove p-nitrotoluene by 20 mg/g and coconut shell AC could adsorb benzene by 212.77 mg/g and coffee residues AC could eliminate formaldehyde by 245 mg/g (González-García, Citation2018). On the other hand, coconut shell AC activated by KOH eliminated 39% of suspended solids by the corresponding surface area of 478 m2/g and steam activated bio-sorbent from oil palm mesocarp fiber AC removed suspended solid from palm oil mill effluent (POME) by 80%. In addition to this, KOH activated sugarcane bagasse AC and sea mango AC adsorbed NH3-N up to 46.65 and 79.77%, respectively (Wong et al., Citation2018). Aceh coffee grounds AC for activation with hydrochloric acid eliminated ammonia by 56% (Mariana, Mahidin, Mulana, & Aman, Citation2018) and sugarcane bagasse AC can take out naphthalene by 119 mg/g with the corresponding surface area of 692 m2/g at the pH level of four (Eslami, Borghei, Rashidi, & Takdastan, Citation2018). Pineapple leaves AC which was activated by phosphoric acid absorbed caffeine by 155.5 mg per gram with a reasonable surface area of 1031 m2/g (Beltrame et al., Citation2018).
3.4. Gas adsorptions
In addition to the liquid substances, activated carbon is also found effective in gas adsorptions as the burning of fossil fuel produces greenhouse gases (GHG) and polluted gases in the environment with the increase of energy demand ( Afroze et al., Citation2018; A. Ahmed et al., Citation2018a; Hossain et al., Citation2019 ). In , it has shown, AC is one of the promising solid adsorbents that can be utilized to adsorb CO2, CH4, H2S, NO2 and H2 gases due to its many advantages like low cost, easy regeneration, insensitivity towards moisture, high gas adsorption capacity at normal atmosphere, high surface area, sufficient pore size distribution, high mechanical stability as well as very low energy requirement (Ogungbenro, Quang, Al-Ali, & Abu-Zahra, Citation2017). The pollutant gases have been adsorbed on activated carbon through the H-H interactions, dipole-dipole bonds, and even by covalent bonds between the gas and functional groups, resulting in the enhancement of efficiency (Creamer and Gao, Citation2016). The adsorption of harmful gases by activated carbon depends on the surface area, adsorption capacity, the micro-pores structure of the carbon and the reproducibility, processability of the solution (Sharma et al., Citation2011). The adsorption CO2 is intensely complex to the textural properties and surface groups of the adsorbents (Bai et al., Citation2013) and the normal adsorption capacity by activated carbon is around 5 wt.% (298 K and 0.1 bar). The K+ ions of the functional groups played a significant role for CO2 adsorption through electrostatic communications (Zhao et al., Citation2012). The CO2 adsorption capability has increased significantly in correlation to the heating temperature up to 873 K. Meanwhile; the micropore has the highest effect on CO2 adsorption (1 bar) and the pore sizes have strong affection on the adsorption behavior at lower pressures (below 0.3 bar) (Lee and Park, Citation2015). Application of activated carbon in NO2 adsorption is motivated by the pattern of pores structure, by the type and chemical organization of the AC surface. Hydrogen sulfide (H2S) is a colorless, toxic, odorous and harmful gas for the health of humans and the environment with a threshold of 0.0047 ppm (Elsayed et al., Citation2009). Activated carbon is the most effective and well-known technique for H2S elimination where the modified carbon with caustic chemicals (KOH and NaOH) or oxidative chemicals (KI and KMnO4) are being used to elemental the hydrogen sulfide gas (Mohamad Nor et al., Citation2013). Recently the removal of SO2 and NOx has gained significant attention due to their toxicity, and ACs are more promising to eliminate these poisonous gases by better sorption capacity. It was found that the impregnation of metal oxides into activated carbon is effective for simultaneously removing SO2 and NOx (Le-Minh et al., Citation2018).
Sunflower seed AC shows better results than almond shell AC for adsorbing CO2 gas (4.6 mmol/g and 2.7 mmol/g, respectively) which is consistent with the corresponding surface areas of 1790 and 862 m2/g, respectively. The activating agents for sunflower seed AC and almond shell AC were CO2 and ZnCl2 for which the surface area was close to each other with the value 870 m2/g. In addition, oil palm shell AC with the surface area of 1213 m2, could eliminate CH4 up to 13 cm3/g whereas, Agiricus fungi AC, activated by KOH, with 2137 m2/g area could capture H2 by 4.7 wt% (González-García, Citation2018). Phoenix dactylifera seeds AC which were activated by N2 removed CO2 up to 141.14 mg/g (Ogungbenro et al., Citation2017) and coconut shell activated carbon took out H2S up to 88.8% (Min et al., Citation2017). For removal of NO2, walnut shell, AC and plum stone AC exhibited almost similar performances, with the values of 66.3 mg/g and 67 mg/g, respectively (Jeguirim, Belhachemi, Limousy, & Bennici, Citation2018). Oil palm shell AC can be activated by CO2, Na2CO3 and ZnCl2 with the resultant surface areas of 1213, 742.34 and 551.05 m2/g and they are found very effective for removal of methane and hydrogen sulfide (Rashidi and Yusup, Citation2017).
4. Recycling/regeneration and handling of AC
The recycling or regeneration of AC has great importance for environmental protection and resource preservation (Bian et al., Citation2018). Activated carbon can be recycled once the pollutants are removed from the used AC and can be recovered up to 80% with high efficiency (Shi et al., Citation2010). If the ACs are soft, the particles become smaller after recycling, but the hard AC can be recycled many times for the rigid part is retained well (Mu’azu et al., Citation2017). Generally, the activated carbon is recycled and regenerated by Thermal (Bhagawan et al., Citation2015), Steam (El Gamal et al., Citation2018) and chemical (Q. Li et al., Citation2015) process. The process details as,
Thermal recycling (Radi et al., Citation2017)
Initially, the used activated carbon is dried out.
The dried AC is heated to higher temperatures so that the pollutants can be carbonized.
Then the AC is regenerated within the temperature range from 700 to 1000 °C to retain the hollow shape in the pores.
Steam recycling (El Gamal et al., Citation2018)
The AC filter is back-flushed with warm water continuously.
The steam is then linked and enforced through the activated carbon within the temperature range from 120 to 130 °C to remove the pollutants.
Finally, the carbon is back-flushed to be re-used.
Chemical recycling (Q. Li et al., Citation2015)
Specific chemical reagents (acidic and alkaline) are being used to desorb or decompose the adsorbate from activated carbon.
The efficiency depends on the type of available pollutants onto activated carbon.
Once the pollutants are eliminated by chemicals, the reagents are also needed to be removed to regenerate the AC.
In the handing of AC, there is no significant human health effect occurring during the contact of activated carbon (“Carbon, Activated - Materials Handled - Flexicon Corporation,” n.d.). Only minor irritations can happen because of contacting AC with human skin, eyes, and respiration. As unused AC is nontoxic, it can be disposed of in the area of harmless wastes. On the other hand used activated carbon may have the characteristics of the pollutant and therefore, it should be discarded according to the applicable rules. Attention must be taken in the reuse of the activated carbon. The use of reactivated ACs for the treatment of drinking water should follow the specific regulations and standards (El Gamal et al., Citation2018). Activated carbons with dust handling should consider eye protection and dust masks with dust filters to reduce the amount of dust intake by the researchers. If the high concentrations of vapors are adsorbed in AC, the temperature may rise, so partially wet with water or reduction of concentration by additional air is necessary (“The Care and Handling of Activated Carbon - WCP Online,” n.d.). In a closed vessel, oxygen reduction may reach a dangerous level because the wet AC favorably removes oxygen from the air. If people work in the vessel which contains AC, appropriate safety and the necessities for lower oxygen must be maintained (“Safe handling of activated carbon | Desotec,” n.d.).
5. Recommendation
A great variety of activated carbons have shown to be the most promising candidates to eliminate any type of pollutants from contaminated water due to their capability, low production cost, and greater efficiency. The applications of these activated carbons for water treatment have already been proved on the lab scale. However, the challenge is faced in the applications in the real industry for commercialization. ACs are not performing well in pollutant removals that are not attracted by carbon like sodium, nitrates, fluoride, and pathogens like bacteria and viruses. So, the process for removing all types of pollutants by activated carbon should be focused. The service life of the ACs is also short when it is bonding or filled with contaminants. An assessment for long-term applications will demonstrate more reliable result wastewater purification. The costs for preparing activated carbon from corresponding raw material sources should be taken into account primarily because of low-cost ACs with reliable surface areas. The majority of the research studies have focused on the removal of metallic pollutants and dye and only a few publications have limited information on other pollutants particularly non-metallic pollutants and pesticides. From this perspective, further investigations will be useful for the development of AC.
6. Conclusion
The precursors of the activated carbon are low cost and available biomass which can be the replacement of commercially available sources. Pyrolysis/gasification is a practical approach to use the wastages and garbage in an environment-friendly technique to prepare the biochar for activated carbon. In biomass, acacia species are also a useful source of AC as they are invasive and abundant all over the world. For the production of ACs from the waste biomass and invasive plants, there are four different types of activation processes that are being used frequently. Among them, chemical activation methods are using frequently for the simplicity of the process, lower investment and less time-consuming in the preparation process. It is observed that in order to improve and optimize the adsorption capacities of ACs towards the removal of organic, inorganic pollutants from water and gas pollutants from the air, an appropriate selection of precursors, carbonization process and optimum activation conditions are the most significant parameter. For dye removal, the performance of chemical and physiochemical activations are much better than physical activation for the higher functional groups in the AC surface. Whereas for the bigger size of the pollutants from metallic, phenolic and pesticides the pore size and pore distribution have directed the efficiency. The removal of gas by AC depends on the attraction force between the gas chemical and carbon surface groups with the pressure. The handling, disposal, recycling and regeneration of the activated carbon before and after use should be maintained according to the standards regulation to uphold the safety and protect the resources and the environment.
Nomenclature | ||
TAd | = | Optimum adsorption temperature |
tAd | = | Adsorption duration at optimum temperature |
Qm | = | Maximum adsorption capacity (mg adsorbate/g adsorbent ) |
Vt | = | Total pore volume |
Vmic | = | Micropore volume |
TC | = | Carbonization temperature |
tc | = | Carbonization holding time |
TA | = | Activation temperature |
ta | = | Activation holding time |
SBET | = | Brunauer–Emmett–Teller (BET) equation surface area |
Y | = | Yield |
IR | = | Impregnation ratio (g activating agent/ g precursor) |
Acknowledgments
The author is very grateful to his supervisors and colleagues for providing encouragement and facilities to this work. We are grateful for the UBD grant no. BD/RSCH/URC/RG(b)/2018/002.
Disclosure statement
No potential conflict of interest was reported by the author(s).
. Applications of AC in the removal of pharmaceutical compounds.
Table 12. Applications of ACs for removal of other pollutants.
Additional information
Funding
References
- Abbas, A.F., & Ahmed, M.J. (2016). Mesoporous activated carbon from date stones (Phoenix dactylifera L.) by one-step microwave assisted K2CO3 pyrolysis. Journal of Water Process Engineering., 9, 201–207. doi:10.1016/j.jwpe.2016.01.004
- Abdalla, A.M., Hossain, S., Nisfindy, O.B., Azad, A.T., Dawood, M., & Azad, A.K. (2018). Hydrogen production, storage, transportation and key challenges with applications: A review. Energy Conversion and Management, 165, 602–627. doi:10.1016/j.enconman.2018.03.088
- Abu Bakar, M. (2013). Catalytic intermediate pyrolysis of Brunei rice husk for bio-oil production. PhD thesis, Aston University, United Kingdom.
- Afif, A., Radenahmad, N., Cheok, Q., Shams, S., Kim, J.H., & Azad, A.K. (2016). Ammonia-fed fuel cells: A comprehensive review. Renewable and Sustainable Energy Reviews, 60, 822–835. doi:10.1016/j.rser.2016.01.120
- Afif, A., Rahman, S.M., Tasfiah Azad, A., Zaini, J., Islan, M.A., & Azad, A.K. (2019). Advanced materials and technologies for hybrid supercapacitors for energy storage – a review. Journal of Energy Storage, 25, 100852. doi:10.1016/j.est.2019.100852
- Afroze, S., Binti Haji Bakar, A.N., Reza, M.S., Salam, M.A., & Azad, A.K. (2018). Polyvinylidene fluoride (PVDF) piezoelectric energy harvesting from rotary retracting mechanism: Imitating forearm motion. IET Conference Publications, Institution of Engineering and Technology, London, United Kingdom, 4pp. doi:10.1049/cp.2018.1591
- Afroze, S., Karim, A.H., Cheok, Q., Eriksson, S., & Azad, A.K. (2019). Latest development of double perovskite electrode materials for solid oxide fuel cells: A review. Frontiers in Energy, 13(4), 770–797. doi:10.1007/s11708-019-0651-x
- Afroze, S., Torino, N., Henry, P.F., Reza, M.S., Cheok, Q., & Azad, A.K. (2020a). Neutron and X-ray powder diffraction data to determine the structural properties of novel layered perovskite PrSrMn2O5 + δ. Data in Brief, 29, 105173. doi:10.1016/j.dib.2020.105173
- Afroze, S., Torino, N., Henry, P.F., Sumon Reza, M., Cheok, Q., & Azad, A.K. (2020b). Insight of novel layered perovskite PrSrMn2O5+δ: A neutron powder diffraction study. Materials Letters., 261, 127126. doi:10.1016/j.matlet.2019.127126
- Ahmad, T., & Danish, M. (2018). Prospects of banana waste utilization in wastewater treatment: A review. Journal of Environment Management, 206, 330–348. doi:10.1016/j.jenvman.2017.10.061
- Ahmed, A., Abu Bakar, M.S., Azad, A.K., Sukri, R.S., & Mahlia, T.M.I. (2018a). Potential thermochemical conversion of bioenergy from Acacia species in Brunei Darussalam: A review. Renewable and Sustainable Energy Reviews, 82, 3060–3076. doi:10.1016/j.rser.2017.10.032
- Ahmed, A., Abu Bakar, M.S., Azad, A.K., Sukri, R.S., & Phusunti, N. (2018b). Intermediate pyrolysis of Acacia cincinnata and Acacia holosericea species for bio-oil and biochar production. Energy Conversion and Management, 176, 393–408. doi:10.1016/j.enconman.2018.09.041
- Ahmed, A., Hidayat, S., Abu Bakar, M.S., Azad, A.K., Sukri, R.S., & Phusunti, N. (2018c). Thermochemical characterisation of Acacia auriculiformis tree parts via proximate, ultimate, TGA, DTG, calorific value and FTIR spectroscopy analyses to evaluate their potential as a biofuel resource. Biofuels, 7269, 1–12. doi:10.1080/17597269.2018.1442663
- Ahmed, M.J. (2016a). Preparation of activated carbons from date (Phoenix dactylifera L.) palm stones and application for wastewater treatments: Review. Process Safety and Environment Protection., 102, 168–182. doi:10.1016/j.psep.2016.03.010
- Ahmed, M.J. (2016b). Application of agricultural based activated carbons by microwave and conventional activations for basic dye adsorption. Journal of Environmental Chemical Engineering, 4(1), 89–99. doi:10.1016/j.jece.2015.10.027
- Ahmed, M.J. (2017). Adsorption of non-steroidal anti-inflammatory drugs from aqueous solution using activated carbons: Review. Journal of Environmental Management, 190, 274–282. doi:10.1016/j.jenvman.2016.12.073
- Ahmed, M.J., & Theydan, S.K. (2012). Equilibrium isotherms, kinetics and thermodynamics studies of phenolic compounds adsorption on palm-tree fruit stones. Ecotoxicology and Environmental Safety, 84, 39–45. doi:10.1016/j.ecoenv.2012.06.019
- Ahmed, S., Parvaz, M., Johari, R., & Rafat, M. (2018). Studies on activated carbon derived from neem (azadirachta indica) bio-waste, and its application as supercapacitor electrode. Materials Research Express, 5(4), 045601. doi:10.1088/2053-1591/aab924
- Ahmed, T.F., Sushil, M., & Krishna, M. (2012). Impact of dye industrial effluent on physicochemical characteristics of Kshipra River, Ujjain City, India. International Research Journal of Environmental Science, 1, 41–45.
- Alagumuthu, G., & Rajan, M. (2010). Equilibrium and kinetics of adsorption of fluoride onto zirconium impregnated cashew nut shell carbon. Chemical Engineering Journal and the Biochemical Engineering Journal, 158(3), 451–457. doi:10.1016/j.cej.2010.01.017
- Alhamed, Y.A. (2009). Adsorption kinetics and performance of packed bed adsorber for phenol removal using activated carbon from dates' stones. Journal of Hazardous Materials, 170(2/3), 763–770. doi:10.1016/j.jhazmat.2009.05.002
- Alhinai, M., Azad, A.K., Bakar, M.S.A., & Phusunti, N. (2018). Characterisation and Thermochemical Conversion of Rice Husk for Biochar Production. International Journal of Renewable Energy Sources, 8, 1648–1656.
- Alslaibi, T.M., Abustan, I., Ahmad, M.A., & Foul, A.A. (2013). A review: Production of activated carbon from agricultural byproducts via conventional and microwave heating. Journal of Chemical Technology & Biotechnology, 88(7), 1183–1190. doi:10.1002/jctb.4028
- Altıntıg, E., Onaran, M., Sarı, A., Altundag, H., & Tüzen, M. (2018). Preparation, characterization and evaluation of bio-based magnetic activated carbon for effective adsorption of malachite green from aqueous solution. Materials Chemistry and Physics., 220, 313–321. doi:10.1016/j.matchemphys.2018.05.077
- Alvarez, J., Lopez, G., Amutio, M., Bilbao, J., & Olazar, M. (2015). Physical activation of rice husk pyrolysis char for the production of high surface area activated carbons. Industrial & Engineering Chemistry Research, 54(29), 7241–7250. doi:10.1021/acs.iecr.5b01589
- Amin, M.T., & Alazba, A.A. (2017). Comparative study of the absorptive potential of raw and activated carbon Acacia nilotica for Reactive Black 5 dye. Environ. Earth Sci, 76, 581. 10.1007/s12665-017-6927-8.
- Anastopoulos, I., & Kyzas, G.Z. (2014). Agricultural peels for dye adsorption: A review of recent literature. Journal of Molecular Liquids., 200, 381–389. doi:10.1016/j.molliq.2014.11.006
- Anfar, Z., Zbair, M., Ahsaine, H., Ezahri, M., & Alem, N. (2018). Well-designed WO3/Activated carbon composite for rhodamin. removal: Synthesis, characterization, and modeling using response surface methodology. Fullerenes Nanotubes and Carbon Nanostructures, 26(6), 389–397. doi:10.1080/1536383X.2018.1440386
- Angın, D., Altintig, E., & Köse, T.E. (2013). Influence of process parameters on the surface and chemical properties of activated carbon obtained from biochar by chemical activation. Bioresource Technology, 148, 542–549. doi:10.1016/j.biortech.2013.08.164
- Ao, W., Fu, J., Mao, X., Kang, Q., Ran, C., Liu, Y., … Dai, J. (2018). Microwave assisted preparation of activated carbon from biomass: A review. Renewable and Sustainable Energy Reviews, 92, 958–979. doi:10.1016/j.rser.2018.04.051
- Arami-Niya, A., Daud, W.M.A.W., & Mjalli, F.S. (2010). Production of palm shell-based activated carbon with more homogeniouse pore size distribution. Journal of Applied Sciences, 10(24), 3361–3366. doi:10.3923/jas.2010.3361.3366
- Arami-Niya, A., Daud, W.M.A.W., & Mjalli, F.S. (2011). Comparative study of the textural characteristics of oil palm shell activated carbon produced by chemical and physical activation for methane adsorption. Chemical Engineering Research and Design., 89(6), 657–664. doi:10.1016/j.cherd.2010.10.003
- Arami-Niya, A., Wan Daud, W.M.A., S. Mjalli, F., Abnisa, F., & Shafeeyan, M.S. (2012). Production of microporous palm shell based activated carbon for methane adsorption: Modeling and optimization using response surface methodology. Chemical Engineering Research and Design., 90(6), 776–784. doi:10.1016/j.cherd.2011.10.001
- Attari, M., Bukhari, S.S., Kazemian, H., & Rohani, S. (2017). A low-cost adsorbent from coal fly ash for mercury removal from industrial wastewater. Journal of Environmental Chemical Engineering., 5(1), 391–399. doi:10.1016/j.jece.2016.12.014
- Azimi, A., Azari, A., Rezakazemi, M., & Ansarpour, M. (2017). Removal of heavy metals from industrial wastewaters: A review. ChemBioEng Reviews., 4(1), 37–59. doi:10.1002/cben.201600010
- Bai, B.C., Cho, S., Yu, H.R., Yi, K.B., Kim, K.D., & Lee, Y.S. (2013). Effects of aminated carbon molecular sieves on breakthrough curve behavior in CO2/CH4 separation. Journal of Industrial and Engineering Chemistry., 19(3), 776–783. doi:10.1016/j.jiec.2012.10.016
- Balasundram, V., Ibrahim, N., Kasmani, R.M., Hamid, M.K.A., Isha, R., Hasbullah, H., & Ali, R.R. (2017). Thermogravimetric catalytic pyrolysis and kinetic studies of coconut copra and rice husk for possible maximum production of pyrolysis oil. Journal of Cleaner Production, 167, 218–228. doi:10.1016/j.jclepro.2017.08.173
- Bansal, R.C., Aggarwal, D., Goyal, M., & Kaistha, B.C. (2002). Influence of carbon-oxygen surface groups on the adsorption of phenol by activated carbons. Indian Journal of Chemical Technology., 9, 290–296.
- Beltrame, K.K., Cazetta, A.L., de Souza, P.S.C., Spessato, L., Silva, T.L., & Almeida, V.C. (2018). Adsorption of caffeine on mesoporous activated carbon fibers prepared from pineapple plant leaves. Ecotoxicology and Environmental Safety, 147, 64–71. doi:10.1016/j.ecoenv.2017.08.034
- Benedetti, V., Patuzzi, F., & Baratieri, M. (2018). Characterization of char from biomass gasification and its similarities with activated carbon in adsorption applications. Applied Energy., 227, 92–99. doi:10.1016/j.apenergy.2017.08.076
- Bernhardt, A., & Gysi, N. (2016). World’s Wost Pollution Problems: The Toxics Beneath Our Feet. Fabrikstrasse 17 8005 Zurich, Switzerland.
- Bhagawan, D., Poodari, S., Ravi Kumar, G., Golla, S., Anand, C., Banda, K.S., … Vidyavathi, S. (2015). Reactivation and recycling of spent carbon using solvent desorption followed by thermal treatment (TR). Journal of Material Cycles and Waste Management, 17(1), 185–193. doi:10.1007/s10163-014-0237-y
- Bian, Y., Yuan, Q., Zhu, G., Ren, B., Hursthouse, A., & Zhang, P. (2018). Recycling of Waste Sludge: Preparation and Application of Sludge-Based Activated Carbon. International Journal of Polymeric Science., 2018, 1–17. doi:10.1155/2018/8320609
- Bolisetty, S., Peydayesh, M., & Mezzenga, R. (2019). Sustainable technologies for water purification from heavy metals: Review and analysis. Chemical Society Reviews, 48(2), 463–487. doi:10.1039/c8cs00493e
- Boudrahem, N., Delpeux-Ouldriane, S., Khenniche, L., Boudrahem, F., Aissani-Benissad, F., & Gineys, M. (2017). Single and mixture adsorption of clofibric acid, tetracycline and paracetamol onto Activated carbon developed from cotton cloth residue. Process Safety and Environment Protection., 111, 544–559. doi:10.1016/j.psep.2017.08.025
- Briones, R., Serrano, L., Younes, R., Ben, Mondragon, I., & Labidi, J. (2011). Polyol production by chemical modification of date seeds. Industrial Crops and Products., 34(1), 1035–1040. doi:10.1016/j.indcrop.2011.03.012
- Burakov, A.E., Galunin, E. V., Burakova, I. V., Kucherova, A.E., Agarwal, S., Tkachev, A.G., & Gupta, V.K. (2018). Adsorption of heavy metals on conventional and nanostructured materials for wastewater treatment purposes: A review. Ecotoxicology and Environmental Safety, 148, 702–712. doi:10.1016/j.ecoenv.2017.11.034
- Caglar, B., Afsin, B., Koksal, E., Tabak, A., & Eren, E. (2013). Characterization of unye bentonite after treatment with sulfuric acid. Química Nova, 36(7), 955–959. doi:10.1590/S0100-40422013000700006
- Cao, Q., Xie, K.C., Lv, Y.K., & Bao, W.R. (2006). Process effects on activated carbon with large specific surface area from corn cob. Bioresource Technology, 97(1), 110–115. doi:10.1016/j.biortech.2005.02.026
- Carbon, Activated - Materials Handled - Flexicon Corporation [www Document], n.d. URL https://www.flexicon.com/Materials-Handled/Carbon-Activated.html. (accessed 12.3.19).
- Chayid, M.A., & Ahmed, M.J. (2015). Amoxicillin adsorption on microwave prepared activated carbon from Arundo donax Linn: Isotherms, kinetics, and thermodynamics studies. Journal of Environmental Chemical Engineering., 3(3), 1592–1601. doi:10.1016/j.jece.2015.05.021
- Chowdhury, Z.Z., Abd Hamid, S.B., Das, R., Hasan, M.R., Zain, S.M., Khalid, K., & Uddin, M.N. (2013). Preparation of carbonaceous adsorbents from lignocellulosic biomass and their use in removal of contaminants from aqueous solution. BioResources, 8(4), 6523–6555. doi:10.15376/biores.8.4.6523-6555
- Creamer, A.E., & Gao, B. (2016). Carbon-based adsorbents for postcombustion CO2 capture: A critical review. Environmental Science & Technology, 50(14), 7276–7289. doi:10.1021/acs.est.6b00627
- Danish, M., & Ahmad, T. (2018). A review on utilization of wood biomass as a sustainable precursor for activated carbon production and application. Renewable and Sustainable Energy Reviews, 87, 1–21. doi:10.1016/j.rser.2018.02.003
- Danish, M., Hashim, R., Ibrahim, M.N.M., & Sulaiman, O. (2013a). Characterization of physically activated Acacia mangium wood-based carbon for the removal of methyl orange dye. BioResources, 8(3), 4323–4339. doi:10.15376/biores.8.3.4323-4339
- Danish, M., Hashim, R., Ibrahim, M.N.M., Rafatullah, M., Ahmad, T., & Sulaiman, O. (2011). Characterization of Acacia mangium wood based activated carbons prepared in the presence of basic activating agents. BioResources, 8(3), 3019–3033.
- Danish, M., Hashim, R., Ibrahim, M.N.M.N.M., & Sulaiman, O. (2013b). Effect of acidic activating agents on surface area and surface functional groups of activated carbons produced from Acacia mangium wood. Journal of Analytical and Applied Pyrolysis., 104, 418–425. doi:10.1016/j.jaap.2013.06.003
- Danish, M., Hashim, R., Ibrahim, M.N.M.N.M.M., Rafatullah, M., & Sulaiman, O. (2012). Surface characterization and comparative adsorption properties of Cr(VI) on pyrolysed adsorbents of Acacia mangium wood and Phoenix dactylifera L. stone carbon. Journal of Analytical and Applied Pyrolysis., 97, 19–28. doi:10.1016/j.jaap.2012.06.001
- Danish, M., Hashim, R., Mohamad Ibrahim, M.N., & Sulaiman, O. (2014). Response surface methodology approach for methyl orange dye removal using optimized Acacia mangium wood activated carbon. Wood Science and Technology, 48(5), 1085–1105. doi:10.1007/s00226-014-0659-7
- Dass, B., & Jha, P. (2015). Batch Adsorption of Phenol by Improved Activated Acacia nilotica branches char: Equilibrium, Kinetic and Thermodynamic studies. Artic. Int. J. ChemTech Res, 8, 269–279.
- Deng, H., Li, G., Yang, H., Tang, J., & Tang, J. (2010). Preparation of activated carbons from cotton stalk by microwave assisted KOH and K2CO3 activation. Chemical Engineering Journal and the Biochemical Engineering Journal., 163(3), 373–381. doi:10.1016/j.cej.2010.08.019
- Derdour, K., Bouchelta, C., Khorief Naser-Eddine, A., Medjram, M.S., & Magri, P. (2018). Removal of Cr(VI) from aqueous solution using activated carbon supported iron catalysts as efficient adsorbent. World Journal of Engineering, 15, 3–13. 10.1108/WJE-06-2017-0132.
- Dhyani, V., & Bhaskar, T. (2018). A comprehensive review on the pyrolysis of lignocellulosic biomass. Renewable Energy, 129, 695–716. doi:10.1016/j.renene.2017.04.035
- Dias, J.M., Alvim-Ferraz, M.C.M., Almeida, M.F., Rivera-Utrilla, J., & Sánchez-Polo, M. (2007). Waste materials for activated carbon preparation and its use in aqueous-phase treatment: A review. Journal of Environmental Management, 85(4), 833–846. doi:10.1016/j.jenvman.2007.07.031
- Din, M. I., Ashraf, S., & Intisar, A. (2017). Comparative study of different activation treatments for the preparation of activated carbon: A mini-review. Science Progress, 100(3), 299–312. doi:10.3184/003685017X14967570531606
- Dong, L., Liu, W., Yu, Y., Hou, L., Gu, P., & Chen, G. (2019). Preparation, characterization, and application of macroporous activated carbon (MAC) suitable for the BAC water treatment process. The Science of the Total Environment, 647, 1359–1367. doi:10.1016/j.scitotenv.2018.07.280
- El Bakouri, H., Usero, J., Morillo, J., Rojas, R., & Ouassini, A. (2009). Drin pesticides removal from aqueous solutions using acid-treated date stones. Bioresource Technology, 100(10), 2676–2684. doi:10.1016/j.biortech.2008.12.051
- El Gamal, M., Mousa, H.A., El-Naas, M.H., Zacharia, R., & Judd, S. (2018). Bio-regeneration of activated carbon: A comprehensive review. Separation and Purification Technology., 197, 345–359. doi:10.1016/j.seppur.2018.01.015
- El-Naggar, A., Lee, S.S., Rinklebe, J., Farooq, M., Song, H., Sarmah, A.K., … Ok, Y.S. (2019). Biochar application to low fertility soils: A review of current status, and future prospects. Geoderma, 337, 536–554. doi:10.1016/j.geoderma.2018.09.034
- Elsayed, A.M., Askalany, A.A., Shea, A.D., Dakkama, H.J., Mahmoud, S., Al-Dadah, R., & Kaialy, W. (2017). A state of the art of required techniques for employing activated carbon in renewable energy powered adsorption applications. Renewable and Sustainable Energy Reviews, 79, 503–519. doi:10.1016/j.rser.2017.05.172
- Elsayed, Y., Seredych, M., Dallas, A., & Bandosz, T.J. (2009). Desulfurization of air at high and low H2S concentrations. Chemical Engineering Journal and the Biochemical Engineering Journal, 155(3), 594–602. doi:10.1016/j.cej.2009.08.010
- Eslami, A., Borghei, S.M.S.M., Rashidi, A., & Takdastan, A. (2018). Preparation of activated carbon dots from sugarcane bagasse for naphthalene removal from aqueous solutions. Separation Science and Technology., 53(16), 2536–2549. doi:10.1080/01496395.2018.1462832
- Fan, J., Zhang, J., Zhang, C., Ren, L., & Shi, Q. (2011). Adsorption of 2,4,6-trichlorophenol from aqueous solution onto activated carbon derived from loosestrife. Desalination, 267(2/3), 139–146. doi:10.1016/j.desal.2010.09.016
- Fu, X., Yang, H., Lu, G., Tu, Y., & Wu, J. (2015). Improved performance of surface functionalized TiO2/activated carbon for adsorption–photocatalytic reduction of Cr(VI) in aqueous solution. Materials Science in Semiconductor Processing, 39, 362–370. doi:10.1016/j.mssp.2015.05.034
- González-García, P. (2018). Activated carbon from lignocellulosics precursors: A review of the synthesis methods, characterization techniques and applications. Renewable and Sustainable Energy Reviews, 82, 1393–1414. doi:10.1016/j.rser.2017.04.117
- Gupta, T.B., & Lataye, D.H. (2017). Adsorption of Indigo Carmine Dye onto Acacia Nilotica (Babool) Sawdust Activated Carbon. Journal of Hazardous, Toxic, and Radioactive Waste, 21(4), 04017013. doi:10.1061/(ASCE)HZ.2153-5515.0000365
- Hammani, H., Boumya, W., Laghrib, F., Farahi, A., Lahrich, S., Aboulkas, A., & El Mhammedi, M.A. (2017). Electrocatalytic effect of NiO supported onto activated carbon in oxidizing phenol at graphite electrode: Application in tap water and olive oil samples. Journal of the Association of Arab Universities for Basic and Applied Sciences, 24(1), 26–33. doi:10.1016/j.jaubas.2017.06.006
- Hidayat, S., Abu Bakar, M.S., Yang, Y., Phusunti, N., & Bridgwater, A.V. (2018). Characterisation and Py-GC/MS analysis of Imperata Cylindrica as potential biomass for bio-oil production in Brunei Darussalam. Journal of Analytical and Applied Pyrolysis., 134, 510–519. doi:10.1016/j.jaap.2018.07.018
- Hoseinzadeh Hesas, R., Wan Daud, W.M.A., Sahu, J.N., & Arami-Niya, A. (2013). The effects of a microwave heating method on the production of activated carbon from agricultural waste: A review. Journal of Analytical and Applied Pyrolysis., 100, 1–11. doi:10.1016/j.jaap.2012.12.019
- Hossain, M.A., Shams, S., Amin, M., Reza, M.S., & Chowdhury, T.U. (2019). Perception and barriers to implementation of intensive and extensive green roofs in Dhaka, Bangladesh. Buildings, 9(4), 79. doi:10.3390/buildings9040079
- Huang, T., Zhou, R., Cui, J., Zhang, J., Tang, X., Chen, S., … Liu, H. (2018). Fast and cost-effective preparation of antimicrobial zinc oxide embedded in activated carbon composite for water purification applications. Materials Chemistry and Physics, 206, 124–129. doi:10.1016/j.matchemphys.2017.11.044
- Ioannidou, O.A., Zabaniotou, A.A., Stavropoulos, G.G., Islam, M.A., & Albanis, T.A. (2010). Preparation of activated carbons from agricultural residues for pesticide adsorption. Chemosphere, 80(11), 1328–1336. doi:10.1016/j.chemosphere.2010.06.044
- Islam, M.A., Tan, I.A.W., Benhouria, A., Asif, M., & Hameed, B.H. (2015). Mesoporous and adsorptive properties of palm date seed activated carbon prepared via sequential hydrothermal carbonization and sodium hydroxide activation. Chemical Engineering Journal and the Biochemical Engineering Journal, 270, 187–195. doi:10.1016/j.cej.2015.01.058
- Islam, S.N., Mohamad, S.M.B.H., & Azad, A.K. (2019). Acacia spp.: Invasive trees along the Brunei Coast, Borneo. In: C. Makowski & C.W. Finkl (Eds.), Impacts of Invasive Species on Coastal Environments: Coasts in Crisis (pp. 455–476). Cham, Switzerland: Springer International Publishing AG. 10.1007/978-3-319-91382-7_14.
- Isoda, N., Rodrigues, R., Silva, A., Gonçalves, M., Mandelli, D., Figueiredo, F.C.A., & Carvalho, W.A. (2014). Optimization of preparation conditions of activated carbon from agriculture waste utilizing factorial design. Powder Technology, 256, 175–181. doi:10.1016/j.powtec.2014.02.029
- Jamshidi, M., Ghaedi, M., Dashtian, K., Ghaedi, A.M.M., Hajati, S., Goudarzi, A., & Alipanahpour, E. (2016). Highly efficient simultaneous ultrasonic assisted adsorption of brilliant green and eosin B onto ZnS nanoparticles loaded activated carbon: Artificial neural network modeling and central composite design optimization. Spectrochimica Acta. Part A, Molecular and Biomolecular Spectroscopy, 153, 257–267. doi:10.1016/j.saa.2015.08.024
- Jaria, G., Silva, C.P., Oliveira, J.A.B.P., Santos, S.M., Gil, M.V., Otero, M., … Esteves, V.I. (2019). Production of highly efficient activated carbons from industrial wastes for the removal of pharmaceuticals from water-A full factorial design. Journal of Hazardous Materials, 370, 212–218. doi:10.1016/j.jhazmat.2018.02.053
- Jeguirim, M., Belhachemi, M., Limousy, L., & Bennici, S. (2018). Adsorption/reduction of nitrogen dioxide on activated carbons: Textural properties versus surface chemistry – a review. Chemical Engineering Journal and the Biochemical Engineering Journal, 347, 493–504. doi:10.1016/j.cej.2018.04.063
- Jodeh, S., Abdelwahab, F., Jaradat, N., Warad, I., & Jodeh, W. (2016). Adsorption of diclofenac from aqueous solution using Cyclamen persicum tubers based activated carbon (CTAC). Journal of the Association of Arab Universities for Basic and Applied Sciences, 20(1), 32–38. doi:10.1016/j.jaubas.2014.11.002
- Katheresan, V., Kansedo, J., & Lau, S.Y. (2018). Efficiency of various recent wastewater dye removal methods: A review. Journal of Environmental Chemical Engineering., 6(4), 4676–4697. doi:10.1016/j.jece.2018.06.060
- Kecira, Z., Benturki, A., Daoud, M., & Benturki, O. (2018). Effect of chemical activation on the surface properties of apricot stones based activated carbons and its adsorptive properties toward aniline. In book:Proceedings of the third international symposium on materials and sustainable development, Springer International Publishing, Springer Nature Switzerland AG(pp. 228–240). 10.1007/978-3-319-89707-3_27.
- Khosravi, R., Moussavi, G., Ghaneian, M.T., Ehrampoush, M.H., Barikbin, B., Ebrahimi, A.A., & Sharifzadeh, G. (2018). Chromium adsorption from aqueous solution using novel green nanocomposite: Adsorbent characterization, isotherm, kinetic and thermodynamic investigation. Journal of Molecular Liquids., 256, 163–174. doi:10.1016/j.molliq.2018.02.033
- Kong, J., Gu, R., Yuan, J., Liu, W., Wu, J., Fei, Z., & Yue, Q. (2018). Adsorption behavior of Ni(II) onto activated carbons from hide waste and high-pressure steaming hide waste. Ecotoxicology and Environmental Safety, 156, 294–300. doi:10.1016/j.ecoenv.2018.03.017
- Kumar, A., & Jena, H.M. (2016). Preparation and characterization of high surface area activated carbon from Fox nut (Euryale ferox) shell by chemical activation with H3PO4. Results in Physics, 6, 651–658. doi:10.1016/j.rinp.2016.09.012
- Kumar, M., & Tamilarasan, R. (2013). Modeling studies for the removal of methylene blue from aqueous solution using Acacia fumosa seed shell activated carbon. Journal of Environmental Chemical Engineering, 1(4), 1108–1116. doi:10.1016/j.jece.2013.08.027
- Lakshmi, S.D., Avti, P.K., & Hegde, G. (2018). Activated carbon nanoparticles from biowaste as new generation antimicrobial agents: A review. Nano-Structures & Nano-Objects, 16, 306–321. doi:10.1016/j.nanoso.2018.08.001
- Largitte, L., Brudey, T., Tant, T., Dumesnil, P.C., & Lodewyckx, P. (2016). Comparison of the adsorption of lead by activated carbons from three lignocellulosic precursors. Microporous and Mesoporous Materials, 219, 265–275. doi:10.1016/j.micromeso.2015.07.005
- Lee, H.W., Kim, Y.-M., Kim, S., Ryu, C., Park, S.H., Park, Y.-K., & Info, A. (2018). Review of the use of activated biochar for energy and environmental applications Review Articles. Carbon Letters, 26, 1–10. 10.5714/CL.2018.26.001.
- Lee, S.Y., & Park, S.J. (2015). A review on solid adsorbents for carbon dioxide capture. Journal of Industrial and Engineering Chemistry, 23, 1–11. doi:10.1016/j.jiec.2014.09.001
- Lee, T., Zubir, Z.A., Jamil, F.M., Matsumoto, A., & Yeoh, F.-Y. (2014). Combustion and pyrolysis of activated carbon fibre from oil palm empty fruit bunch fibre assisted through chemical activation with acid treatment. Journal of Analytical and Applied Pyrolysis, 110, 408–418. doi:10.1016/j.jaap.2014.10.010
- Le-Minh, N., Sivret, E.C., Shammay, A., & Stuetz, R.M. (2018). Factors affecting the adsorption of gaseous environmental odors by activated carbon: A critical review. Critical Reviews in Environmental Science and Technology, 48(4), 341–375. doi:10.1080/10643389.2018.1460984
- Li, J., Dai, J., Liu, G., Zhang, H., Gao, Z., Fu, J., … Huang, Y. (2016). Biochar from microwave pyrolysis of biomass: A review. Biomass and Bioenergy, 94, 228–244. doi:10.1016/j.biombioe.2016.09.010
- Li, K., Ruan, H., Ning, P., Wang, C., Sun, X., Song, X., & Han, S. (2018). Preparation of walnut shell-based activated carbon and its properties for simultaneous removal of H2S, COS and CS2from yellow phosphorus tail gas at low temperature. Research on Chemical Intermediates, 44(2), 1209–1233. doi:10.1007/s11164-017-3162-6
- Li, L., Sato, Y., & Shimizu, T. (2015). Promoting effect of PKS ash on activated carbon preparation from cypress Sawdust Liuyun. International Proceedings of Chemical, Biological & Environmental Engineering, 51, 139–142. 10.7763/IPCBEE.
- Li, Q., Qi, Y., & Gao, C. (2015). Chemical regeneration of spent powdered activated carbon used in decolorization of sodium salicylate for the pharmaceutical industry. Journal of Cleaner Production, 86, 424–431. doi:10.1016/j.jclepro.2014.08.008
- Li, W., Zhang, L., Peng, J., Li, N., & Zhu, X. (2008). Preparation of high surface area activated carbons from tobacco stems with K2CO3 activation using microwave radiation. Industrial Crops and Products., 27(3), 341–347. doi:10.1016/j.indcrop.2007.11.011
- Lingamdinne, L.P., Koduru, J.R., & Karri, R.R. (2019). A comprehensive review of applications of magnetic graphene oxide based nanocomposites for sustainable water purification. Journal of Environmental Management, 231, 622–634. doi:10.1016/j.jenvman.2018.10.063
- Maguana, Y., El, Elhadiri, N., Bouchdoug, M., & Benchanaa, M. (2018). Study of the in fluence of some factors on the preparation of activated carbon from walnut cake using the fractional factorial design. Journal of Environmental Chemical Engineering, 6(1), 1093–1099. doi:10.1016/j.jece.2018.01.023
- Mahat, S.B., Omar, R., Idris, A., Mustapa Kamal, S.M., & Mohd Idris, A.I. (2018). Dynamic membrane applications in anaerobic and aerobic digestion for industrial wastewater: A mini review. Food Bioprod. Food and Bioproducts Processing, 112, 150–168. doi:10.1016/j.fbp.2018.09.008
- Maneerung, T., Liew, J., Dai, Y., Kawi, S., Chong, C., & Wang, C.H. (2016). Activated carbon derived from carbon residue from biomass gasification and its application for dye adsorption: Kinetics, isotherms and thermodynamic studies. Bioresource Technology, 200, 350–359. doi:10.1016/j.biortech.2015.10.047
- Mansour, F., Al-Hindi, M., Yahfoufi, R., Ayoub, G.M., & Ahmad, M.N. (2018). The use of activated carbon for the removal of pharmaceuticals from aqueous solutions: A review. Reviews in Environmental Science and Bio/Technology, 17(1), 109–145. doi:10.1007/s11157-017-9456-8
- Mariana, M., Mahidin, M., Mulana, F., & Aman, F. (2018). Utilization of activated carbon prepared from aceh coffee grounds as bio-sorbent for treatment of fertilizer industrial waste water. IOP Conference Series: Materials Science and Engineering, 358, 012027. doi:10.1088/1757-899X/358/1/012027
- Menya, E., Olupot, P.W.W., Storz, H., Lubwama, M., & Kiros, Y. (2018). Production and performance of activated carbon from rice husks for removal of natural organic matter from water: A review. Chemical Engineering Research and Design, 129, 271–296. doi:10.1016/j.cherd.2017.11.008
- Mestre, A.S., Pires, R.A., Aroso, I., Fernandes, E.M., Pinto, M.L., Reis, R.L., … Carvalho, A.P. (2014). Activated carbons prepared from industrial pre-treated cork: Sustainable adsorbents for pharmaceutical compounds removal. Chemical Engineering Journal and the Biochemical Engineering Journal., 253, 408–417. doi:10.1016/j.cej.2014.05.051
- Min, H.S., Abbas, M., Kanthasamy, R., Abdul Aziz, H., & Tay, C.C. (2017). Activated Carbon: Prepared From Various Precursors. Ideal International E – Publication Pvt. Ltd.
- Mohamad Nor, N., Lau, L.C., Lee, K.T., & Mohamed, A.R. (2013). Synthesis of activated carbon from lignocellulosic biomass and its applications in air pollution control - a review. Journal of Environmental Chemical Engineering, 1(4), 658–666. doi:10.1016/j.jece.2013.09.017
- Mohammad Razi, M.A., Al-Gheethi, A., Al-Qaini, M., & Yousef, A. (2018). Efficiency of activated carbon from palm kernel shell for treatment of greywater. Arab Journal of Basic and Applied Sciences, 25(3), 103–110. doi:10.1080/25765299.2018.1514142
- Mohan, D., Sarswat, A., Ok, Y.S., & Pittman, C.U. (2014). Organic and inorganic contaminants removal from water with biochar, a renewable, low cost and sustainable adsorbent-a critical review. Bioresource Technology, 160, 191–202. doi:10.1016/j.biortech.2014.01.120
- Mohd Din, A.T., Hameed, B.H., & Ahmad, A.L. (2009). Batch adsorption of phenol onto physiochemical-activated coconut shell. Journal of Hazardous Materials, 161(2-3), 1522–1529. doi:10.1016/j.jhazmat.2008.05.009
- Morni, N.A.H., Radenahmad, N., Abu Bakar, M.S., Sukri, R.S., Phusunti, N., & Azad, A.K. (2018). Potential of sewage sludge as energy recovery via gasification process. Paper presented at the 7th Brunei International Conference on Engineering and Technology 2018 (BICET 2018). Institution of Engineering and Technology, p. 31 (4pp.). doi:10.1049/cp.2018.1528
- Mu’azu, N.D., Jarrah, N., Zubair, M., & Alagha, O. (2017). Removal of phenolic compounds from water using sewage sludge-based activated carbon adsorption: A review. International Journal of Environmental Research and Public Health, 14(10), 1094. doi:10.3390/ijerph14101094
- Namasivayam, C., & Sangeetha, D. (2004). Equilibrium and kinetic studies of adsorption of phosphate onto ZnCl2 activated coir pith carbon. Journal of Colloid and Interface Science, 280(2), 359–365. doi:10.1016/j.jcis.2004.08.015
- Namasivayam, C., & Sangeetha, D. (2005). Kinetic studies of adsorption of thiocyanate onto ZnCl2 activated carbon from coir pith, an agricultural solid waste. Chemosphere, 60(11), 1616–1623. doi:10.1016/j.chemosphere.2005.02.051
- Namasivayam, C., & Sangeetha, D. (2006). Removal of molybdate from water by adsorption onto ZnCl2 activated coir pith carbon. Bioresource Technology, 97(10), 1194–1200. doi:10.1016/j.biortech.2005.05.008
- Niazi, L., Lashanizadegan, A., & Sharififard, H. (2018). Chestnut oak shells activated carbon: Preparation, characterization and application for Cr (VI) removal from dilute aqueous solutions. Journal of Cleaner Production, 185, 554–561. doi:10.1016/j.jclepro.2018.03.026
- Niksiar, A., & Nasernejad, B. (2017). Activated carbon preparation from pistachio shell pyrolysis and gasification in a spouted bed reactor. Biomass and Bioenergy, 106, 43–50. doi:10.1016/j.biombioe.2017.08.017
- Odetoye, T.E., Abu Bakar, M.S., & Titiloye, J.O. (2019). Pyrolysis and characterization of Jatropha curcas shell and seed coat. Nigerian Journal of Technological Development, 16(2), 71. doi:10.4314/njtd.v16i2.4
- Odetoye, T.E., Afolabi, T.J., Abu Bakar, M.S., & Titiloye, J.O. (2018). Thermochemical characterization of Nigerian Jatropha curcas fruit and seed residues for biofuel production. Energy, Ecology and Environment, 3(6), 330–337. doi:10.1007/s40974-018-0104-0
- Odetoye, T.E., Onifade, K.R., AbuBakar, M.S., & Titiloye, J.O. (2013). Thermochemical characterisation of Parinari polyandra Benth fruit shell. Industrial Crops and Products, 44, 62–66. doi:10.1016/j.indcrop.2012.10.013
- Ogungbenro, A.E., Quang, D. V., Al-Ali, K., & Abu-Zahra, M.R.M. (2017). Activated carbon from date seeds for CO2 capture applications. Energy Procedia., 114, 2313–2321. doi:10.1016/j.egypro.2017.03.1370
- Oladipo, A.A., & Gazi, M. (2015). Microwaves initiated synthesis of activated carbon-based composite hydrogel for simultaneous removal of copper(II) ions and direct red 80 dye: A multi-component adsorption system. Journal of the Taiwan Institute of Chemical Engineers., 47, 125–136. doi:10.1016/j.jtice.2014.09.027
- Oladipo, A.A., & Ifebajo, A.O. (2018). Highly efficient magnetic chicken bone biochar for removal of tetracycline and fluorescent dye from wastewater: Two-stage adsorber analysis. Journal of Environmental Management, 209, 9–16. doi:10.1016/j.jenvman.2017.12.030
- Oladipo, A.A., Ifebajo, A.O., Nisar, N., & Ajayi, O.A. (2017). High-performance magnetic chicken bone-based biochar for efficient removal of rhodamine-B dye and tetracycline: Competitive sorption analysis. Water Science and Technology : A Journal of the International Association on Water Pollution Research, 76(2), 373–385. doi:10.2166/wst.2017.209
- Ozbay, N., & Yargic, A.S. (2016). Comparison of surface and structural properties of carbonaceous materials prepared by chemical activation of tomato paste waste: The effects of activator type and impregnation ratio. Journal of Applied Chemistry 2016, 1–10. 10.1155/2016/8236238.
- Pathak, P.D., & Mandavgane, S.A. (2015). Preparation and characterization of raw and carbon from banana peel by microwave activation: Application in citric acid adsorption. Journal of Environmental Chemical Engineering, 3(4), 2435–2447. doi:10.1016/j.jece.2015.08.023
- Qaisrani, Z.N., Shams, S., Zhenren, G., Reza, M.S., & Zainuddin, Q. (2018). Quantitative analysis of marine debris along the sea beaches of Brunei Darussalam. IET Conference Publications. Institution of Engineering and Technology, p. 108 (4 pp.). 10.1049/cp.2018.1605.
- Radenahmad, N., Afif, A., Petra, P.I., Rahman, S.M.H., Eriksson, S.G., & Azad, A.K. (2016). Proton-conducting electrolytes for direct methanol and direct urea fuel cells – a state-of-the-art review. Renew. Sustain. Energy Rev, 10.1016/j.rser.2015.12.103.
- Radenahmad, N., Rahman, I.S.A., Morni, N.A.H., & Azad, A.K. (2018). Acacia-polyethylene terephthalate co- gasification as renewable energy resource. Int. J. Renew. Energy Res, 8, 1612–1620.
- Radenahmad, N., Tasfiah, A., Saghir, M., Taweekun, J., Saifullah, M., Bakar, A., … Kalam, A. (2020). A review on biomass derived syngas for SOFC based combined heat and power application. Renew. Sustain. Energy Rev, 119 109560. https://doi.org/10.1016/j.rser.2019.109560.
- Radi, D.B., Stanojevi, M.M., Obradovi, M.O., & Jovovi, A.M. (2017). Thermal analysis of physical and chemical changes occuring during regeneration of activated carbon. Thermal Science, 21, 1067–1081. 10.2298/TSCI150720048R.
- Rafsanjani, H.H., Kamandari, H., & Najjarzadeh, H. (2013). Study on pore and surface development of activated carbon produced from Iranian coal in a rotary Kiln reactor. Iran. J. Chem. Eng, 10, 27–38.
- Rani, N., Gupta, A., & Yadav, A.K. (2006). Removal of Cr (VI) form aqueous solutions by Acacia nilotica bark. Environmental Technology., 27(6), 597–602. doi:10.1080/09593332708618672
- Rashidi, N.A., & Yusup, S. (2017). A review on recent technological advancement in the activated carbon production from oil palm wastes. Chemical Engineering Journal and the Biochemical Engineering Journal., 314, 277–290. doi:10.1016/j.cej.2016.11.059
- Regti, A., Laamari, M.R., Stiriba, S.-E., & El Haddad, M. (2017). Potential use of activated carbon derived from Persea species under alkaline conditions for removing cationic dye from wastewaters. Journal of the Association of Arab Universities for Basic and Applied Sciences, 24, 10–18. 10.1016/j.jaubas.2017.01.003.
- Reza, M.S., Ahmed, A., Caesarendra, W., Abu Bakar, M.S., Shams, S., Saidur, R., … Azad, A.K. (2019). Acacia Holosericea: An invasive species for bio-char, bio-oil and biogas production. Bioengineering, 6(2), 33. doi:10.3390/bioengineering6020033
- Reza, M.S., Islam, S.N., Afroze, S., Bakar, M.S.A., Sukri, R.S., Rahman, S., & Azad, A.K. (2020). Evaluation of the bioenergy potential of invasive Pennisetum purpureum through pyrolysis and thermogravimetric analysis. Energy, Ecology and Environment, 5(2), 118–133. doi:10.1007/s40974-019-00139-0
- Rivera-Utrilla, J., Sánchez-Polo, M., Ferro-García, M.Á., Prados-Joya, G., & Ocampo-Pérez, R. (2013). Pharmaceuticals as emerging contaminants and their removal from water. A review. Chemosphere, 93(7), 1268–1287. doi:10.1016/j.chemosphere.2013.07.059
- Safe handling of activated carbon | Desotec [WWW Document], n.d. Retrieved from March 12, 2019, https://www.desotec.com/en/carbonology/carbonology-academy/safe-handling-activated-carbon.
- Sahu, U.K., Sahu, S., Mahapatra, S.S., & Patel, R.K. (2017). Cigarette soot activated carbon modified with Fe3O4 nanoparticles as an effective adsorbent for As(III) and As(V): Material preparation, characterization and adsorption mechanism study. Journal of Molecular Liquids., 243, 395–405. doi:10.1016/j.molliq.2017.08.055
- Sajjadi, S.-A., Mohammadzadeh, A., Tran, H.N., Anastopoulos, I., Dotto, G.L., Lopičić, Z.R., … Hosseini-Bandegharaei, A. (2018). Efficient mercury removal from wastewater by pistachio wood wastes-derived activated carbon prepared by chemical activation using a novel activating agent. Journal of Environmental Management, 223, 1001–1009. doi:10.1016/j.jenvman.2018.06.077
- Salman, J.M., & Hussein, F.H. (2014). Batch adsorber design for different solution volume/adsorbate mass ratios of bentazon, carbofuran and 2,4-D adsorption on to date seeds activated carbon. Journal of Environmental Analytical Chemistry, 2, 1–5. 10.4172/2380-2391.1000120.
- Salman, J.M., Njoku, V.O., & Hameed, B.H. (2011). Bentazon and carbofuran adsorption onto date seed activated carbon: Kinetics and equilibrium. Chemical Engineering Journal and the Biochemical Engineering Journal., 173(2), 361–368. doi:10.1016/j.cej.2011.07.066
- Sayğılı, H., & Güzel, F. (2018). Novel and sustainable precursor for high-quality activated carbon preparation by conventional pyrolysis: Optimization of produce conditions and feasibility in adsorption studies. Advanced Powder Technology., 29(3), 726–736. doi:10.1016/j.apt.2017.12.014
- Sharma, P., Kaur, H., Sharma, M., & Sahore, V. (2011). A review on applicability of naturally available adsorbents for the removal of hazardous dyes from aqueous waste. Environmental Monitoring and Assessment, 183(1–4), 151–195. doi:10.1007/s10661-011-1914-0
- Shen, Y., & Fu, Y. (2018). KOH-activated rice husk char via CO2 pyrolysis for phenol adsorption. Materials Today Energy, 9, 397–405. doi:10.1016/j.mtener.2018.07.005
- Shi, Q., Zhang, J., Zhang, C., Li, C., Zhang, B., Hu, W., … Zhao, R. (2010). Preparation of activated carbon from cattail and its application for dyes removal. Journal of Environmental Sciences, 22(1), 91–97. doi:10.1016/S1001-0742(09)60079-6
- Shrestha, D., Gyawali, G., & Rajbhandari, A.R. (2009). Preparation and characterization of activated carbon from waste sawdust from saw mill. Journal of Hazardous Materials, 165, 481–485. 10.1016/j.jhazmat.2008.10.011.
- Sivarajasekar, N., Srileka, S., Samson Arun Prasath, S., Robinson, S., & Saravanan, K. (2008). Kinetic modeling for biosorption of metylene blue onto H3PO4 activated Acacia arabica. Carbon Letters, 9(3), 181–187. doi:10.5714/CL.2008.9.3.181
- Sun, K., & Jiang, J. c. (2010). Preparation and characterization of activated carbon from rubber-seed shell by physical activation with steam. Biomass and Bioenergy, 34(4), 539–544. doi:10.1016/j.biombioe.2009.12.020
- Sun, K., Leng, C.Y., Jiang, J.C., Bu, Q., Lin, G.F., Lu, X.C., & Zhu, G.Z. (2017). Microporous activated carbons from coconut shells produced by self-activation using the pyrolysis gases produced from them, that have an excellent electric double layer performance. New Carbon Materials, 32(5), 451–459. doi:10.1016/S1872-5805(17)60134-3
- Tan, K.L., & Hameed, B.H. (2017). Insight into the adsorption kinetics models for the removal of contaminants from aqueous solutions. Journal of the Taiwan Institute of Chemical Engineers, 74, 25–48. doi:10.1016/j.jtice.2017.01.024
- Tchikuala, E.F., Mourão, P.A.M., & Nabais, J.M.V. (2017). Removal of phenol by adsorption on activated carbon from aqueous solution, In: Wastes: Solutions, Treatments and Opportunities (pp. pp. 1–3). Faculty of Engineering of the University of Porto, Porto, Portugal.
- Teow, Y.H., & Mohammad, A.W. (2019). New generation nanomaterials for water desalination: A review. Desalination, 451, 2–17. doi:10.1016/j.desal.2017.11.041
- The Care and Handling of Activated Carbon - WCP Online [WWW Document], n.d. URL http://wcponline.com/2005/06/22/care-handling-activated-carbon/. (accessed 12.3.19).
- Theydan, S.K., & Ahmed, M.J. (2012). Adsorption of methylene blue onto biomass-based activated carbon by FeCl3 activation: Equilibrium, kinetics, and thermodynamic studies. Journal of Analytical and Applied Pyrolysis, 97, 116–122. doi:10.1016/j.jaap.2012.05.008
- Tseng, R.-L., Tseng, S.-K., & Wu, F.-C. (2006). Preparation of high surface area carbons from Corncob with KOH etching plus CO2 gasification for the adsorption of dyes and phenols from water. Colloids and Surfaces A: Physicochemical and Engineering Aspects, 279(1–3), 69–78. doi:10.1016/j.colsurfa.2005.12.042
- Tsoncheva, T., Mileva, A., Tsyntsarski, B., Paneva, D., Spassova, I., Kovacheva, D., … Petrov, N. (2018). Activated carbon from Bulgarian peach stones as a support of catalysts for methanol decomposition. Biomass and Bioenergy, 109, 135–146. doi:10.1016/j.biombioe.2017.12.022
- Tuan, T.Q., Son, N., Van, Dung, H.T.K., Luong, N.H., Thuy, B.T., Anh, N.T., Van, … Hai, N.H. (2011). Preparation and properties of silver nanoparticles loaded in activated carbon for biological and environmental applications. Journal of Hazardous Materials, 192(3), 1321–1329. doi:10.1016/j.jhazmat.2011.06.044
- Ukanwa, P., Sakrabani, A. & Mandavgane, (2019). A review of chemicals to produce activated carbon from agricultural waste biomass. Sustainability, 11, 6204. doi:10.3390/su11226204
- Vences-Alvarez, E., Razo-Flores, E., Lázaro, I., Briones-Gallardo, R., Velasco-Martínez, G., & Rangel-Mendez, J.R. (2017). Gold recovery from very dilute solutions from a mine in closing process: Adsorption-desorption onto carbon materials. Journal of Molecular Liquids., 240, 549–555. doi:10.1016/j.molliq.2017.05.069
- Viswanathan, G., Jaswanth, A., Gopalakrishnan, S., & Siva Ilango, S. (2009). Mapping of fluoride endemic areas and assessment of fluoride exposure. The Science of the Total Environment, 407(5), 1579–1587. doi:10.1016/j.scitotenv.2008.10.020
- Wan Ibrahim, W.M.H., Mohamad Amini, M.H., Sulaiman, N.S., & Kadir, W.R.A. (2019). Powdered activated carbon prepared from Leucaena leucocephala biomass for cadmium removal in water purification process. Arab Journal of Basic and Applied Sciences, 26(1), 30–40. doi:10.1080/25765299.2018.1533203
- Wang, H., Xie, R., Zhang, J., & Zhao, J. (2018). Preparation and characterization of distillers’ grain based activated carbon as low cost methylene blue adsorbent: Mass transfer and equilibrium modeling. Advanced Powder Technology., 29(1), 27–35. doi:10.1016/j.apt.2017.09.027
- Wang, J., Wu, F.-A., Wang, M., Qiu, N., Liang, Y., Fang, S.-Q., & Jiang, X. (2010). Preparation of activated carbon from a renewable agricultural residue of pruning mulberry shoot. African J. Biotechnol, 9, 2762–2767. 10.5897/AJB2010.000-3101.
- Wong, S., Ngadi, N., Inuwa, I.M., & Hassan, O. (2018). Recent advances in applications of activated carbon from biowaste for wastewater treatment: A short review. Journal of Cleaner Production, 175, 361–375. doi:10.1016/j.jclepro.2017.12.059
- Wu, F.C., & Tseng, R.L. (2006). Preparation of highly porous carbon from fir wood by KOH etching and CO2 gasification for adsorption of dyes and phenols from water. Journal of Colloid and Interface Science, 294(1), 21–30. doi:10.1016/j.jcis.2005.06.084
- WWAP/UN-Water (2018). The United Nations World Water Development Report 2018: Nature-Based Solutions for Water. 7, Place de Fontenoy, 75352 Paris 07 SP, France.
- Yahya, M.A., Al-Qodah, Z., & Ngah, C.W.Z. (2015). Agricultural bio-waste materials as potential sustainable precursors used for activated carbon production: A review. Renewable and Sustainable Energy Reviews, 46, 218–235. doi:10.1016/j.rser.2015.02.051
- Yahya, M.A., Mansor, M.H., Zolkarnaini, W.A.A.W., Rusli, N.S., Aminuddin, A., Mohamad, K., … Ozair, L.N. (2018). A brief review on activated carbon derived from agriculture by-product. in: AIP Conference Proceedings. pp. 030023–1–030023–8. doi:10.1063/1.5041244
- Yang, X., Wan, Y., Zheng, Y., He, F., Yu, Z., Huang, J., … Gao, B. (2019). Surface functional groups of carbon-based adsorbents and their roles in the removal of heavy metals from aqueous solutions: A critical review. Chemical Engineering Journal and the Biochemical Engineering Journal, 366, 608–621. doi:10.1016/j.cej.2019.02.119
- Yang, Z., Feng, R., & Zhang, Z. (2019). A review on reverse osmosis and nanofiltration membranes for water purification. Polymers (Basel), 11, 1252. doi:10.3390/polym11081252
- Yusuff, A.S. (2019). Adsorption of hexavalent chromium from aqueous solution by Leucaena leucocephala seed pod activated carbon: Equilibrium, kinetic and thermodynamic studies. Arab Journal of Basic and Applied Sciences, 26(1), 89–102. doi:10.1080/25765299.2019.1567656
- Zaini, M.A.A., & Kamaruddin, M.J. (2013). Critical issues in microwave-assisted activated carbon preparation. Journal of Analytical and Applied Pyrolysis, 101, 238–241. doi:10.1016/j.jaap.2013.02.003
- Zbair, M., Ainassaari, K., Drif, A., Ojala, S., Bottlinger, M., Pirilä, M., … Brahmi, R. (2018). Toward new benchmark adsorbents: Preparation and characterization of activated carbon from argan nut shell for bisphenol A removal. Environmental Science and Pollution Research, 25(2), 1869–1882. doi:10.1007/s11356-017-0634-6
- Zhao, Y., Liu, X., Yao, K.X., Zhao, L., & Han, Y. (2012). Superior capture of CO2 achieved by introducing extra-framework cations into N-doped microporous carbon. Chemistry of Materials, 24(24), 4725–4734. doi:10.1021/cm303072n